BRAIN INJURY
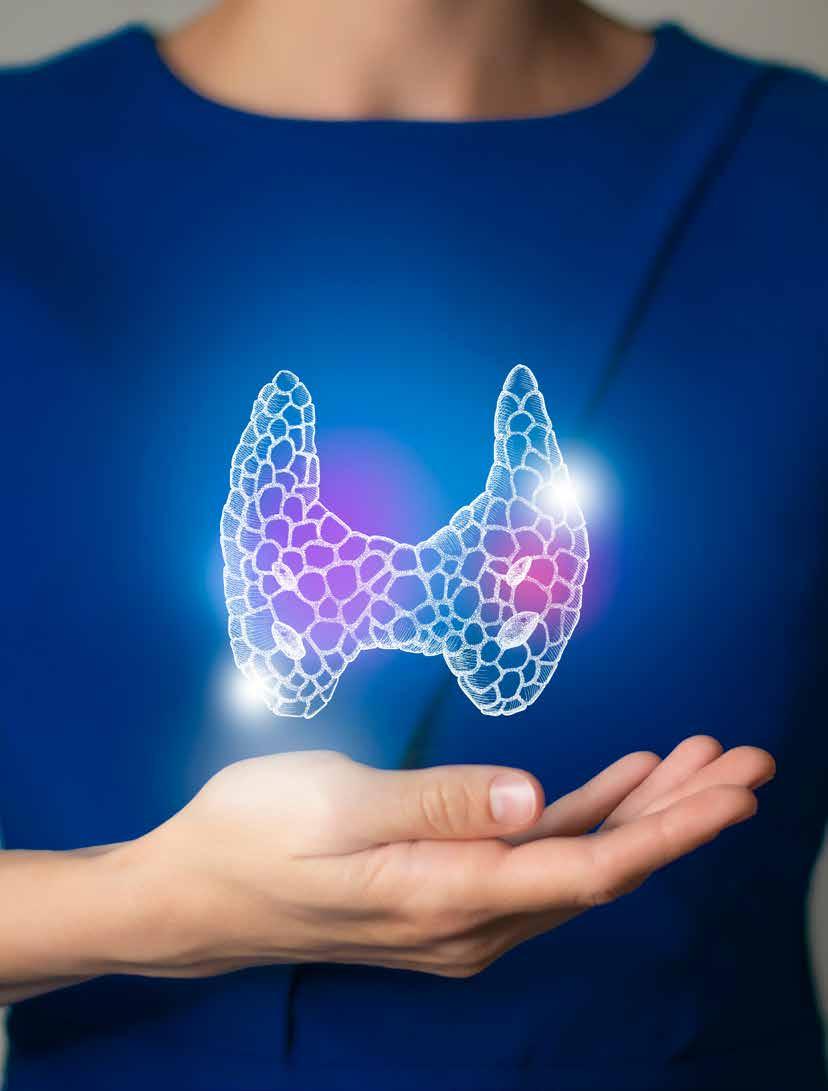
Endocrine Disorders After Traumatic Brain Injury
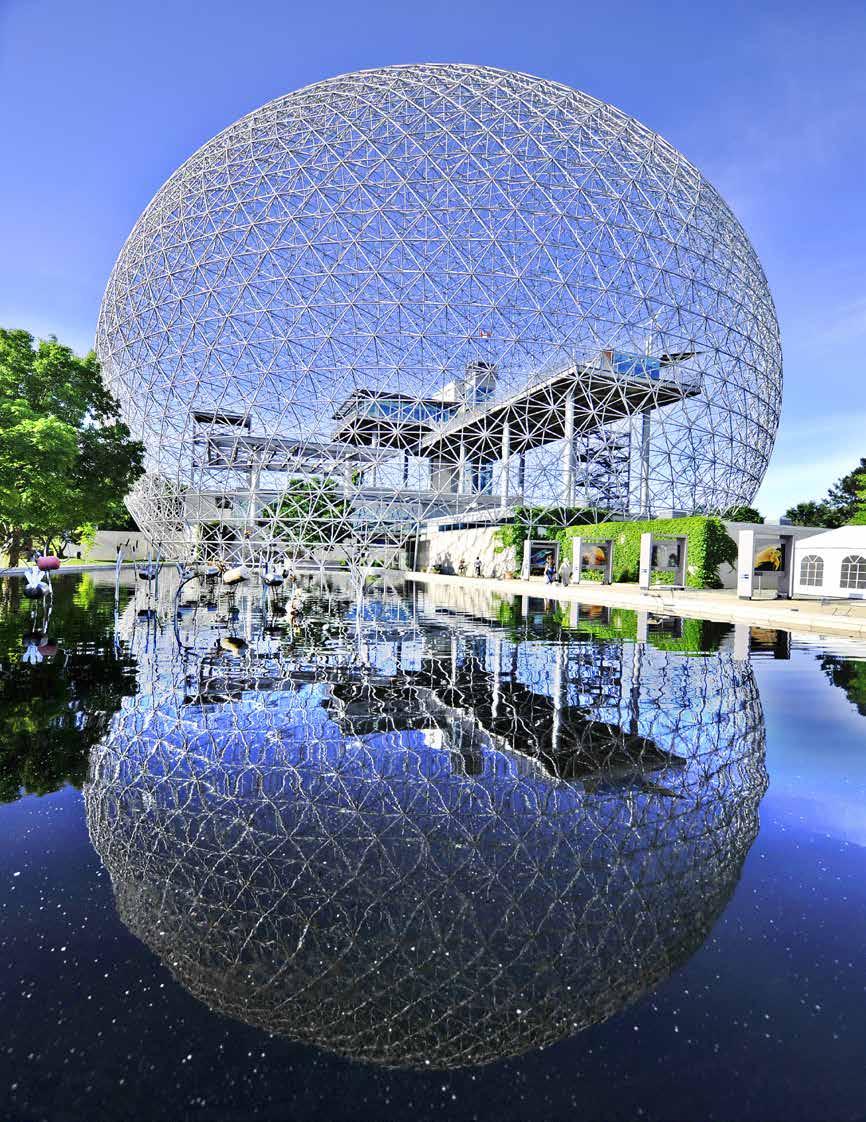
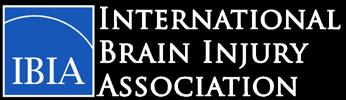
The Role of Pituitary Deficiencies in Delayed Recovery from Concussion: A Multidisciplinary Case Discussion
Tamara L. Wexler, MD, PhD • Chantel T. Debert, MD MSc FRCPC
Walter M. High, Jr., PhD • Brent E. Masel, MD • Alina Nico West, MD, PhD
Kevin C.J. Yuen, MD
Traumatic Brain Injury in Adults: An Often Forgotten yet Challenging Clinical Entity
Kevin C.J. Yuen, MD
Post-Traumatic Hypopituitarism (PTHP) in Military and Veteran Populations
Megan Herodes, BS, CSP • Jose Garcia, MD, PhD 22
Pediatric Traumatic Hypopituitarism (PTHP) Diagnostics: Where Are We Headed?
Alina Nico West, MD, PhD • Nadeem I. Shafi, MD
Alicia M. Diaz-Thomas, MD, MPH
Traversing the Landscape of Neuroendocrine Deficits Following Traumatic Brain Injury: The Role of a Neurorehabilitation Specialist
Chantel T. Debert, MD, MSc, FRCPC
The Effect of Growth Hormone on Cognition after Traumatic Brain Injury and Other Conditions
Walter M. High, Jr., PhD
Post Traumatic Hypopituitarism and Gut-Brain Dysbiosis in Chronic TBI: Incidence and Evaluation
Brent E. Masel, MD • Stefanie N. Howell, PhD
NORTH AMERICAN BRAIN INJURY SOCIETY
CHAIRMAN Mariusz Ziejewski, PhD
VICE CHAIR Debra Braunling-McMorrow, PhD
IMMEDIATE PAST CHAIR Ronald C. Savage, EdD
TREASURER Bruce H. Stern, Esq.
SECRETARY Brian Greenwald, MD
FAMILY LIAISON Skye MacQueen
EXECUTIVE DIRECTOR/ADMINISTRATION Margaret J. Roberts
EXECUTIVE DIRECTOR/OPERATIONS J. Charles Haynes, JD
MARKETING MANAGER Megan Bell-Johnston
BRAIN INJURY PROFESSIONAL
PUBLISHER J. Charles Haynes, JD
CO-EDITOR IN CHIEF Beth Slomine, PhD - USA
CO-EDITOR IN CHIEF Nathan Zasler, MD - USA
ASSOCIATE EDITOR Juan Arango-Lasprilla, PhD – Spain
TECHNOLOGY EDITOR Stephen K. Trapp, PhD - USA
EDITOR EMERITUS Debra Braunling-McMorrow, PhD - USA
EDITOR EMERITUS Ronald C. Savage, EdD - USA
DESIGN AND LAYOUT Kristin Odom
ADVERTISING SALES Megan Bell-Johnston
EDITORIAL ADVISORY BOARD
Nada Andelic, MD - Norway
Philippe Azouvi, MD, PhD - France
Mark Bayley, MD - Canada
Lucia Braga, PhD - Brazil
Ross Bullock, MD, PhD - USA
Fofi Constantinidou, PhD, CCC-SLP, CBIS - USA
Gordana Devecerski, MD, PhD - Serbia
Sung Ho Jang, MD - Republic of Korea
Cindy Ivanhoe, MD - USA
Inga Koerte, MD, PhD - USA
Brad Kurowski, MD, MS - USA
Jianan Li, MD, PhD - China
Christine MacDonell, FACRM - USA
Calixto Machado, MD, PhD - Cuba
Barbara O’Connell, OTR, MBA - Ireland
Lisandro Olmos, MD - Argentina
Caroline Schnakers, PhD - USA
Lynne Turner-Stokes, MD - England
Olli Tenovuo, MD, PhD - Finland
Asha Vas, PhD, OTR - USA
Walter Videtta, MD – Argentina
Thomas Watanabe, MD – USA
Alan Weintraub, MD - USA
Sabahat Wasti, MD - Abu Dhabi, UAE
Gavin Williams, PhD, FACP - Australia
Hal Wortzel, MD - USA
Mariusz Ziejewski, PhD - USA
EDITORIAL INQUIRIES
Managing Editor
Brain Injury Professional PO Box 131401, Houston, TX 77219-1401
Tel 713.526.6900 Email: mbell@hdipub.com Website: www.nabis.org
ADVERTISING INQUIRIES
Megan Bell-Johnston
Brain Injury Professional
HDI Publishers PO Box 131401, Houston, TX 77219-1401
Tel 713.526.6900 Email: mbell@internationalbrain.org
NATIONAL OFFICE
North American Brain Injury Society PO Box 1804, Alexandria, VA 22313
Tel 703.960.6500 / Fax 703.960.6603 Website: www.nabis.org ISSN 2375-5210
Brain Injury Professional is a quarterly publication published jointly by the North American Brain Injury Society and HDI Publishers. © 2023 NABIS/HDI Publishers. All rights reserved. No part of this publication may be reproduced in whole or in part in any way without the written permission from the publisher. For reprint requests, please contact, Managing Editor, Brain Injury Professional, PO Box 131401, Houston, TX 77219-1400, Tel 713.526.6900, Fax 713.526.7787,
Nathan D. Zasler, MD, DABPM&R, FAAPM&R, FACRM,
BIM-C, CBIST
Nathan Zasler, MD, is an internationally respected physician specialist in acquired brain injury (ABI) care and rehabilitation. He is CEO and Medical Director of the Concussion Care Centre of Virginia, an outpatient neurorehabilitation practice, as well as, the Medical Director of Tree of Life, a living assistance and transitional neurorehabilitation program for persons with acquired brain injury in Richmond, Virginia. He is board certified in Physical Medicine and Rehabilitation and fellowship trained in brain injury, as well as, Brain Injury Medicine certified.
Dr. Zasler is an Affiliate Professor of PM&R at VCU in Richmond, Virginia, as well as, a Visiting Professor of PM&R at the University of Virginia, Charlottesville, Virginia.
Dr. Zasler has lectured and written extensively on neurorehabilitation issues in ABI. He is active in national and international organizations dealing with acquired brain injury and neurodisability, serving in numerous consultant and board member roles.
I was very pleased that Tamara Wexler, MD, PhD, accepted my invitation to guest edit this issue on the topic of endocrine disorders after traumatic brain injury. Having known Dr. Wexler for a number of years and highly respecting her knowledge as well as standing in the field of endocrinology and neuroendocrinology, I think we are very fortunate to have her guest edit this issue along with her meritorious colleagues.
Readers of this issue of BIP will find the topics covered diverse and relevant to their brain injury practice ranging from pediatric to adult issues. As a clinician who has been in the field for many years, the evolution of knowledge in this area of brain injury medicine has been rather substantive and like many areas of our field crosses multiple disciplines. We certainly have come a long way in the last 30 years in regards to post-TBI neuroendocrinological assessment and treatment; however, there are still caveats and controversies to be addressed.
This issue of BIP provides a number of different perspectives from a dynamic group of multidisciplinary practitioners ranging from physicians (neurology, physiatry and endocrinology) to neuropsychology. A case presentation by Dr. Wexler puts these experts into dialogue and offers an example of how a patient may present and be helped by a multidisciplinary team, followed by an article on endocrinopathies following adult TBI by Dr. Yuen which includes commentary on who, when and how to screen. Dr. Garcia et al then examine post-traumatic hypopituitarism in military populations discuss the differential incidence and types from those that occur in civilian populations. The next article by Dr. Nico West et al provides a very nice review and some great tables dealing with pediatric posttraumatic hypopituitarism. A physiatrist perspective of neuroendocrine deficits following TBI by Dr. Debert provides readers with a neurorehabilitation overview of the topic again reminding us that these types of deficits can also occur even after milder injury such as concussion. An important but seldom discussed topic is covered by Dr. High in his article reviewing the cognitive impact of growth hormone following TBI (and other disorders). The last article in this issue of BIP addresses a particularly hot topic in the context of the relationship between gut brain dysbiosis and post-traumatic hypopituitarism in the context of the relatively recent discovery of brain injury associated fatigue and altered cognition (BIAFAC).
This issue of BIP will definitely spark discussion and get you cerebral circuits (and maybe even your hormones) revved up….thank you Dr. Wexler for all your hard work in putting this issue together and a special thanks to all who contributed their time and knowledge to this effort.
Nathan D. Zasler, MD, FAAPM&R, FACRM, BIM, CBIST Co-Chief Editor, Brain Injury Professional
Children’s Healthcare of Atlanta is Commission on Accreditation of Rehabilitation Facilities (CARF)-accredited for pediatric rehabilitation services.
We offer:
• An expansive Inpatient Rehabilitation Program
– A spinal cord system of care, brain injury and pediatric specialty programs that have received CARF specialty recognition
– A team of brain injury board-certified pediatric physiatrists
– Comprehensive care for young patients from birth to age 21
– Therapy seven days a week
– 28 private patient rooms
• A Day Rehabilitation Program to assist patients during recovery
• Technology-assisted therapy through our Center for Advanced Technology and Robotic Rehabilitation
• A full-service hospital with emergency services
Learn more or make a referral:
Tamara L. Wexler, MD, PhD
Tamara L. Wexler, MD, PhD, is a neuroendocrinologist whose work focuses on pituitary hormone dysfunction, including after acquired brain injury. She is a Clinical Professor in the Department of Rehabilitation Medicine at NYU and Adjunct Professor in the Department of Endocrinology, Diabetes and Metabolism at the University of Pennsylvania. She has served as an Attending Physician in Internal Medicine at the Massachusetts General Hospital and as the founding Director of the NYU Langone Medical Center Pituitary Center. While on staff at the MGH, Dr. Wexler spent four years at McKinsey & Company, where she is now a Senior External Advisor.
Dr. Wexler is a member of the University of Pennsylvania Institute for Diabetes, Obesity, and Metabolism Leadership Council, and recently completed a term on the Endocrine Society Clinical Guidelines Committee. She is a reviewer or peer editor for several publications, and her own publications appear in the Journal of Clinical Endocrinology & Metabolism and Journal of Neurotrauma, among other journals. Dr. Wexler founded Neuroendocrine Associates to provide education and clinical care regarding post-brain injury neuroendocrine issues.
I was delighted to be asked to edit this edition highlighting the importance of pituitary hormone deficiencies arising from traumatic brain injury (TBI).
This is an important area, and one that offers great opportunity: Hypopituitarism impacts health and quality of life, and, untreated, is associated with increased mortality as well as morbidity. Increased awareness of post-traumatic hypopituitarism (PTHP) - and thus education alone - can make a difference in the lives of many patients.
While there is finally increasing attention being paid to the health effects of brain injury, the role that pituitary hormone deficiencies may play in patients’ health after traumatic brain injury (TBI) remains underappreciated. Pituitary deficiencies are seen at a high rate in patients who have sustained TBI, with reports of chronic pituitary dysfunction in 15-60% of adults after TBI, and up to 42% of children and adolescents. Pituitary deficiencies include adrenal insufficiency, centrally mediated hypothyroidism, hypogonadism, and growth hormone deficiency (GHD, the most common deficiency reported after 12 months post-TBI).
Notably, pituitary deficiencies may appear soon after injury, or at a delay of months to years, and after mild concussions as well as more severe injury. Given the number of people who have sustained a TBI, it is important to address whom to screen. With no clinically applicable associations with imaging characteristics, types of injury, or biomarkers consistently identified in research to date, we rely on signs and symptoms—on clinical judgment. Symptoms from hypopituitarism include cognitive, physical, and emotional effects, and overlap with symptoms from other etiologies including TBI itself. Clinical sequelae may include difficulties with executive function, increased anxiety and irritability, irregular menses, sexual side effects, fatigue, and changes in bone strength and body composition, and delay physical and neurorehabilitation efforts. It is particularly important to be aware of patterns that suggest pituitary deficiencies in patients with persistent symptoms after TBI, as replacement of deficient hormones can reverse the effects of hypopituitarism.
I am especially honored to be able to present articles from leaders in this field, from multiple disciplines. Adult endocrinologists Kevin C.J. Yuen and Jose Garcia, pediatric neurocritical care researcher Alina Nico West, physiatrist Chantel T. Debert, neuropsychologist Walter M High, Jr, and neurologist Brent Masel are leaders in this field, and they, with their colleagues, emphasize in their articles the importance of screening for PTHP in appropriate cases, and the opportunity--and need—for further rigorous research.
We begin with a case illustrating the importance of multidisciplinary perspectives for these patients, bringing into dialogue experts in physiatry, adult and pediatric endocrinology, neurology, critical care, and psychology. Kevin Yuen highlights some of the challenges in diagnosis of PTHP. Jose Garcia and Megan Herodes address the important differences specific to a military population, stressing the importance of obtaining a thorough history including evidence of exposure to conflict. Alina Nico West, with Nadeem Shafi and Alicia Diaz-Thomas, discuss pediatric post-TBI hypopituitarism, emphasizing the importance of presentation and labs and the need for further research. Chantel T. Debert outlines the essential role of the physiatrist in recognizing and initiating the evaluation for PTHP, stressing the importance of screening questions in patients who may not share important symptoms, and clinical judgment. Walter M. High, Jr, presents a compelling review of the known role of GH in neurocognitive health after TBI, emphasizing the importance of treating GHD from TBI with as much attention as GHD from other causes. Brent Masel, with Stephanie Howell, provides an overview of the anatomy and pathophysiology of PTHP, and discusses the role of growth hormone and gut communication in patients after TBI.
We hope this edition provides an overview of the importance of pituitary hormones in patient health after TBI, and drives additional research and clinical attention to this area.
Tamara L. Wexler, MD, PhD
Chantel T. Debert, MD MSc FRCPC
Walter M. High, Jr., PhD
Brent E. Masel, MD
Alina Nico West, MD, PhD
Kevin C.J. Yuen, MD
Experts from different disciplines were invited to provide their perspectives on a case. The responses highlight the different perspectives that may exist regarding post-TBI pituitary deficiencies and their importance in post-TBI health and quality of life, and the importance of clinical judgement and multidisciplinary collaboration and communication.
Further information on this topic from the perspectives of Neurology (p. 36), Physiatry (p. 28), Neuropsychology (p. 32), Pediatric Endocrinology (p. 22), and Adult Endocrinology (pp. 10 and 28) can be found in this journal edition. Military cases are thought to be sufficiently distinct that commentary was not included on this non-military case; a full perspective and review on pituitary sequelae on TBI in Veterans is provided on page 16.
A 28 yo female with a series of concussions sustained during soccer (most recently 6 months ago) presents at your office, noting that she is not recovering as she did from prior concussions. She has had trouble focusing and recalling words and events, and her productivity at her government job has declined due to difficulty sustaining work for more than several hours. She was told all testing was normal, but her speech and cognitive processing seem slower to her family and partner. On exam, she also describes new loss of libido and menstrual irregularity since the recent concussion; she ascribed these to stress, as she has also felt more anxious than usual. She has stopped playing soccer or exercising due to fatigue.
Brent Masel, Neurology: This young lady has had multiple concussions and has not recovered from her last blow 6 months ago. She now has cognitive and executive functioning issues. She has anxiety, altered menses, and extreme fatigue. Although the “work up” has been negative, unfortunately, “work ups” frequently lack a neuroendocrine evaluation.
Chantel T. Debert, Physiatry: This athlete has sustained multiple concussions in the past, recovering from each in the appropriate time period. However, following the most recent concussion, she has a protracted and halted recovery. The lack of consistent improvement, cognitive difficulties, altered libido and menses irregularities should cue the neurorehabilitation specialist that a deeper look into neuroendocrine abnormalities is warranted.
Walter High, Neuropsychology: A person can score well within the normal range for any given neuropsychological test and still be experiencing a significant decrement in their pre-injury cognitive performance. It is difficult to estimate expected pre-injury performance with precision without pre-injury testing which is rarely available. Even if testing were available, performance on any given test can vary day-to-day, or year-to-year.
Decrements in functioning due to either concussion or growth hormone deficiency are often relatively small and difficult to detect because they fall within the same range as test-retest or intersubject variability. The net result is that the person experiencing the decrement in cognitive function feels like they are having a ‘bad’ or ‘sluggish’ day, every day. This may be especially noticeable for persons working in cognitively demanding jobs or studies where even a small decrement in performance may result in greater difficulty or even inability to complete a given task.
The patient is referred to an endocrinologist. Initial screening tests (8a morning fasting cortisol, TSH and free T4,* estradiol with LH/ FSH/prolactin,* and IGF-1*) appear normal, with the IGF-1 level in the lower part of the normal range.
Kevin Yuen, Endocrinology: Based on her history and symptomatology, a full neuroendocrine work up is recommended. If there are any non-GH pituitary hormone deficiencies detected, these deficiencies should be optimally replaced first before rescreening this patient again for possible GH deficiency with a serum IGF-I measurement. If her serum IGF-I level continues to be low or low-normal together with the presence of symptoms, then GH stimulation testing either with a GST or an ITT is recommended. The GST is generally the preferred test because it does not require direct and close medical supervision and can be performed in an office setting, unlike the ITT. Libido issues are difficult to ascertain regarding cause and in the context of a patient with possible TBI-induced hypopituitarism, tend to be related to secondary hypogonadism or the injury itself rather than GHD.
B. Masel, Neurology: In this scenario, we will assume that her imaging and pituitary axis testing are normal, and her IGF-1 is low normal. Based on what you will read (p. 36), you would agree that the most likely diagnosis is BIAFAC, and she should be referred to an Endocrinologist for provocative (and definitive) testing of the GH axis. If she is “lucky”, her testing will be abnormal and she can be started on growth hormone replacement which may markedly improve her symptoms.
The endocrinologist, aware that normal-range IGF-1 levels can be seen in the presence of failed provocative testing for growth hormone deficiency (GHD), ordered a glucagon stimulation test (GST). Peak GH on the test was 2.9 mg/mL in this patient with normal weight (BMI 23 kg/m2).
K. Yuen, Endocrinology: The cut-offs for GST for diagnosing adult GHD are as follows:
- peak GH ≤ 3.0 mg/L in normal-weight (BMI < 25 kg/m2) patients
- peak GH ≤ 3.0 mg/L in overweight (BMI 25-30 kg/m2) patients with a high pre-test probability
- peak GH ≤ 1.0 mg/L in overweight (BMI 25-30 kg/m2) patients with a low pre-test probability
- peak GH ≤ 1.0 mg/L in obese (BMI > 30 kg/m2) patients
If the patient instead had a BMI that is < 25 kg/m2 with a GH peak of 3 mg/L, then the patient, as per guidelines, is not GH deficient. This is because several GST studies have shown that BMI inversely correlated with peak GH levels. In addition, I would put this patient at low pre-test probability based on an IGF-I in the lower half of normal range and no other pituitary hormone deficiencies.
B. Masel, Neurology: Indeed, her peak GH level is 2.9, and she would be considered GH deficient and appropriate for therapy. However, what if her peak was 3.5? Abnormal is less than 3 according to most guidelines.
In view of her clear BIAFAC symptomatology, many physicians who treat people with this problem would consider her to be GH insufficient, as many patients with a peak between 3 and 10 do indeed respond to GH replacement. The Endocrinologist will most likely have to do some convincing to get the insurance company to pay—but with perseverance, it can be accomplished.
Although treatment is life-long and can be expensive, most likely she will see improvement and possibly resolution in her symptoms. Using a phrase we hear from many patients after starting treatment, she may be able to say: “I got my life back”.
W. High, Neuropsychology: The expected decrement in cognitive performance from concussion falls within the same range as the expected decrement associated with growth hormone deficiency. Therefore, the cognitive effects from growth hormone deficiency can be easily confounded with the effects of concussion. The symptom of increased fatigue due to growth hormone deficiency or concussion may be similarly confounded. Increased fatigue and decrements in cognitive performance are probably interrelated.
If the case were identical other than age, with the patient presenting at age 15, how might the evaluation and treatment differ?
Nico West, Pediatric Critical Care: Considering the patient’s age and pubertal status, endocrinology and/or adolescent Ob/Gyn referrals would be appropriate regarding her menstrual irregularities. Neurology, clinical psychology or neuropsychology (depending on availability) could be consulted regarding her neurocognitive deficits, slowed speech, and increased anxiety. Brain imaging may be indicated preliminarily for neurocognitive and neuropsychological changes. If this patient is referred to endocrinology, auxological measurements, her past medical and concussion history (especially timing of concussions related to age and pubertal status), and physical examination would determine if growth hormone testing is warranted.
Tamara L. Wexler, MD, PhD, a neuroendocrinologist, is a Clinical Professor in the Department of Rehabilitation Medicine at NYU, and Adjunct Professor in the Division of Endocrinology, Diabetes, and Metabolism at the University of Pennsylvania. See full bio on page 7.
Chantel T. Debert, MD, MSc, FRCPC, is an associate professor and clinician scientist in the Department of Clinical Neurosciences, division of physical medicine and rehabilitation and member of the Hotchkiss brain Institute at the University of Calgary. See full bio on page 31.
Brent E. Masel, MD, is a Board Certified Neurologist, Clinical Professor of Neurology at UTMB and Executive VP for Medical Affairs at the Centre for Neuro Skills. See full bio on page 38.
Walter M. High, Jr., PhD, is a neuropsychologist currently in private practice in New Mexico. See full bio on page 35.
Kevin C.J. Yuen, MD, is a Professor of Medicine at the University of Arizona College of Medicine and Creighton School of Medicine, and Medical Director of the Barrow Pituitary Center at the Barrow Neurological Institute in Phoenix, AZ. See full bio on page 15.
Alina Nico West, MD, PhD, is an Assistant Professor of Pediatrics and Clinical Researcher at the University of Tennessee Health Science Center (UTHSC) in the Division of Pediatric Critical Care Medicine in Memphis, Tennessee, USA. See full bio on page 26.
* TSH = thyroid-stimulating hormone, produced by the pituitary; T4 = thyroxine, produced by the thyroid gland; LH = luteinizing hormone, produced by the pituitary; FSH = follicle-stimulating hormone, produced by the pituitary; IGF-1 = insulin-like growth factor-1, produced by the liver in response to growth hormone.
Kevin C.J. Yuen, MD
Abstract
Traumatic brain injury (TBI) results in substantial health and economic burden, and it is one of the leading causes of death and disability globally. Clinical manifestations can range from no symptoms to mild alterations of consciousness to a comatose state and death, with survivors experiencing varying degrees of physical and emotional deficits. Numerous studies have described the risk of developing endocrinopathies, particularly growth hormone deficiency; hence, post-TBI endocrinopathies should no longer be considered a rare sequela. Nevertheless, the reported prevalence remains variable across studies, depending on the time frame after injury, time and type of testing, and variability in hormonal values considered normal across different studies. Furthermore, this clinical entity is challenging and often ignored due to the presenting symptoms that may be mistaken as part of the TBI itself, the severity of other life-threatening comorbidities that take clinical precedence, the lack of consensus guidelines of when to perform hormonal testing, and the challenges in conducting dynamic endocrine testing. The present review reports evidence on the post-TBI endocrinopathies, including clinical consequences if untreated, challenges in screening and subsequent diagnostic workup, and principles of therapeutic management. It is essential for healthcare providers managing TBI patients to recognize and to be aware of this challenging clinical entity. There remains an unmet need for developing stronger evidence, and for consensus guidelines establishing recommendations for screening and diagnosis so that they can be incorporated as standard of care in the management of TBI patients.
Traumatic brain injury (TBI) incurs substantial health and economic burden, and is one of the leading causes of death and disability affecting an estimated 64-74 million people worldwide yearly.1 In the past decade, endocrinopathies after TBI have been increasingly described in numerous studies that suggest that this complication is not so rare after all.2-4 However, the reported prevalence remains highly variable as it largely depends on the time frame of injury, time and type of endocrine testing, changes in hormonal levels over time after the TBI, and variable hormonal values and cut-points used to define various endocrinopathies. Pooled prevalence of endocrinopathies is estimated to range between 27.5-32%, and 19.8-25.3% of patients with TBI who develop single pituitary axis involvement, while multiple axes involvement is less frequent in the order of 6.7-7.7%. 2, 4 The symptoms can be mild and nonspecific, and may remain unscreened in very ill patients and those with other significant comorbidities after the TBI, leading to either the condition remaining undiagnosed or a delay in diagnosis. In this review, we report the increasing evidence for post-TBI endocrinopathies, and highlight the challenges of detecting and managing this condition, including identifying which patients to screen, timing of screening, challenges in conducting dynamic endocrine tests, and the limitations of biochemical testing, particularly the growth hormone (GH) stimulation tests.
Several methods are now used to classify TBI into mild, moderate, or severe categories, including measurements of duration of loss of
consciousness, post-traumatic amnesia, alteration of consciousness, and neuroimaging5, with the Glasgow Coma Scale (GCS) scoring system being the most frequently used measure of primary injury in the pre-hospital and acute care setting.6 The GCS is a 15-point scale used to assess alertness, level of consciousness, and motor response; scores of 13-15, 9-12, and 3-8 indicate mild, moderate, and severe TBI, respectively.6 The score reflects the primary event of direct brain injury to the parenchyma. The limitation of the GCS score, however, is that it may not accurately reflect the level of consciousness of the patient, a critical indicator of TBI severity.7 Recent studies have investigated the predictive value of the initial GCS for morbidity and long-term injury outcomes after TBI. Although there was a correlation between score and outcomes, other factors can influence outcomes, such as injury severity scores, associated hypoxic-ischemic injury, and provision of emergency care provided at a trauma versus non-trauma center.8, 9
Endocrinopathies after TBI can present with variable and nonspecific clinical features, which may overlap with those resulting from the injury. The clinical picture includes a very large spectrum determined by the type, number and severity of pituitary hormone deficiencies, and may range from mild and non-specific complaints to life-threatening conditions.10 Moderate to severe TBI itself can result in severe loss of cognitive function in a wide range of tasks including communication skills, motor skills, psychosocial adjustment, and emotional stability that might overlap with symptoms of endocrinopathies.11 Like those with moderate to severe TBI, patients with mild TBI (mTBI) with endocrinopathies may also exhibit physiological changes and cognitive and behavioural alterations,12 and the challenge is not to miss the diagnosis in these individuals, as many are not aware that they need to seek medical care.
In the acute phase of TBI, assessment for endocrinopathies can be challenging, as hormonal changes can occur as part of the stress response, and are also affected by medications and surgery. Furthermore, any change in mental status and muscle strength is challenging to detect in patients with altered consciousness. Refractory hypotension that is thought to be due to acute blood loss and circulatory hypovolemia can be due to adrenal insufficiency and arginine vasopressin (AVP)-deficiency, whereas gonadotropin, TSH and GH deficiencies might not even be clinically evident at this point and testing is not required. During this critical period, adrenal insufficiency and AVP-deficiency requires prompt treatment to prevent life-threatening adrenal crisis and hypovolemic electrolyte abnormalities. The chronic phase—defined as at least 3 months after injury-- is when GH deficiency (GHD) and gonadotrophin deficiency have been reported to develop, less often with hypothyroidism and adrenal insufficiency.10 Growth hormone deficiency can result in increased insulin resistance, hyperglycemia, increased abdominal fat deposition, dyslipidemia, and neurocognitive changes such as those involving executive function, concentration, problem-solving ability, memory, and speech.13 Hypogonadism and hypothyroidism can affect cognition and memory performance, while adrenal insufficiency is associated with increased fatigue, inadequate response to stress, decreased memory, and mood disorders.14 Thus, the symptoms of endocrinopathies after TBI can be non-specific, but contribute to the impairment in quality of life and delay the rehabilitative process of these patients; while some of the early endocrine abnormalities are transient with complete recovery, others can evolve over time and only become detectable several months after the injury.15
Growth hormone is secreted from the somatotroph cells in the anterior pituitary gland that receives its blood supply from long portal vessels, predisposing these cells to injury and early loss of function after TBI.15 Growth hormone deficiency is the most common endocrinopathy that can develop after 1, 3, and 5 years post-TBI.16 However, the prevalence of GHD varies across studies, depending on the severity of injury, age, time, and method of assessment, from 2-30% in the acute stage17, 18 and persisting in 10–63.6% in the chronic stage.19 Although most pituitary hormone deficiencies improve over time, the prevalence of GHD at 12 months post-TBI is still relatively high at 38%.18 Plasma insulin growth factor I (IGF-I) level is usually performed as a screening test; if low or low normal, further confirmation of the diagnosis is needed in the form of a GH stimulation test, unless there are at least three other pituitary hormone deficiencies simultaneously present.13 However, in acute trauma with underlying illness, IGF-I levels may not be reliable. Variability of IGF-I assays can also affect the accuracy of the test.
The evaluation for GHD is recommended at least ~3 months after TBI because of the possible spontaneous recovery in the post-acute phase19. Some authors proposed to postpone the evaluation until 1 year after TBI in adults, while children may require an earlier assessment.20 The choice of one test over another is usually based on patient characteristics, availability of GH secretagogues, and center experience. The insulin tolerance test (ITT) is considered the gold standard test for non-TBI patients with GHD but it can be only be used after excluding contraindications, such as history of seizures and cardio/cerebrovascular disease.13 Because it carries a risk of dangerous and unpleasant neuroglycopenia and seizures, the test is less favorable to perform in TBI patients.21 GH releasing hormone (GHRH) plus arginine can be safely used as the alternative test for ITT, but after EMD Serono discontinued manufacturing the GHRH analog (Geref@) in November 2008, this test is now rarely used in the United States.22 To fill this gap, the glucagon stimulation test (GST) then gained popularity in the United States as the alternative test to the ITT.28 In December 2017, the United States FDA approved oral ghrelin analogue macimorelin for use as a diagnostic test for adult GHD and mandated a GH cut-point of 2.8 mg/L to differentiate patients with normal GH secretion from those with GHD.23 Main advantages of macimorelin are that the drug is orally administered, has no risk of causing hypoglycaemia, and the test is shorter (90 minutes with 3-4 blood sample collections) in contrast to the higher number of blood sample collections over 2 hours for the ITT and 3-4 hours for the GST.24, 25 The macimorelin test is unaffected by age, BMI, or sex, indicating robustness for diagnosing adult GHD.26 The most commonly reported side effect is mild dysgeusia, generally mild and tolerable.24, 25 One drug-related serious adverse event was reported in a subject with an asymptomatic QT interval prolongation on the electrocardiogram that resolved spontaneously within 24 h.25 Thus, careful assessment of the patient’s concurrent medications is recommended to avoid drug-to-drug interactions. Wider use was precluded in the United States only by the high cost of macrimorelin itself ($4,500 per packet). However, in August 2022, a press announcement was released stating that Novo Nordisk Healthcare AG was planning to terminate the amended development and commercialization license agreement for macimorelin in the United States27
From May 2023 onwards, Aeterna Zentaris regained its full rights in the United States to macimorelin but because it has yet to find a partner in the United States, sales of macimorelin are temporarily discontinued until an alternate commercialization partner is found.
Due to the lack of availability of the GHRH-arginine and the macimorelin tests, the only alternative test to the ITT now available in the United States is the GST. Advantages of the GST are reproducibility, safety, and lack of influence by gender and hypothalamic GHD.28 Disadvantages include the lengthy test duration (3-4 hours), and the side-effects, including nausea, vomiting, and headaches reported in < 10%29 to 34%29 (mainly between 60-210 min into the test and resolved by 240 min). Furthermore, the side-effects are more pronounced in elderly subjects, in whom symptomatic hypotension (9.5%) and seizures (2.4%) have been observed.30
The GST is not without other caveats. Several studies have shown that the fixed-dose GST’s GH cut-point of 3 mg/L overdiagnoses adult GHD in a substantial number of overweight/ obese subjects and those with glucose intolerance. In two large retrospective studies, Toogood et al.31 and Yuen et al 32 found an inverse correlation between BMI and peak GH during the GST; this relationship appeared to be strongest with BMIs between 30 and 40 kg/m2 and to plateau at BMIs > 40 kg/m.31 Dichtel et al 33 evaluated 3 groups of overweight/obese men--i.e., controls who were younger than the patients, patients with 3-4 pituitary hormone deficits, and patients with 1-2 pituitary hormone deficits-- and found that the GH cut-point of 0.94 mg/L provided the optimal sensitivity (90%) and specificity (94%) in non-controls, and that BMI and amount of visceral adipose tissue inversely correlated with peak GH levels in controls. Diri et al 34 evaluated 216 patients with pituitary disease and 26 healthy controls and compared the GST to the ITT. These investigators used a GH cut-point of 3.0 mg/L for the ITT and two GH cut-points of 3.0 mg/L and 1.07 mg/L for the GST, yielding the diagnosis of adult GHD in 86.1%, 74.5%, and 54.2 % patients, respectively. Patient age, BMI, and number of pituitary hormone deficits correlated with IGF-I and peak GH levels. Twelve out of 26 (46.2 %) healthy subjects failed the GST using a GH cut-point of 3.0 mg/L, but none when the cut-point was lowered to 1.07 mg/L. Wilson et al. 35 studied 42 patients with a high pre-test probability of adult GHD, and these investigators found that body weight negatively correlated with GH area under the curve (AUC) (R = -0.45; P = 0.01) and peak GH response (R = -0.42; P = 0.02), and positively correlated with nadir blood glucose levels (R = 0.48; P < 0.01). Nadir blood glucose levels during GST inversely correlated with GH AUC (r= -0.38; p=0.03) and peak GH (r= -0.37; p=0.04), implying that patients with diabetes mellitus tended to have a lower glucagon-induced GH response. Hamrahian et al 36 compared the fixed-dose GST (1 mg or 1.5 mg in patients > 90 kg body weight) and weight-based GST (WB-GST: 0.03 mg/kg) with the ITT using a GH cut-point of 3.0 mg/L. Patients with hypothalamic-pituitary disease and 1-2 (n = 14) or ≥ 3 (n = 14) pituitary hormone deficiencies, and control subjects (n = 14) matched for age, sex, estrogen status and BMI, undertook the ITT, GST and WB-GST in random order. Using ROC analyses, the optimal GH cut-point was 1.0 (92% sensitivity, 100% specificity) for fixed-dose GST and 2.0 mg/L (96% sensitivity and 100% specificity) for WB-GST. Therefore, the investigators concluded that lowering the GH cut-point from 3 mg/L to 1 mg/L is important in overweight (BMI 25-30 kg/m2) patients with a low pretest probability and in obese (BMI > 30 kg/m2) patients, to reduce misclassifying adult GHD.
Nevertheless, it remains unclear whether hyperglycemia influences peak GH responses to glucagon stimulation, independent of central adiposity. Studies by Yuen et al 32 and Wilson et al 35 demonstrated that higher fasting (range 90-316 mg/dL), peak (range 156-336 mg/ dL), and nadir (range 52-200 mg/dL) blood glucose levels during the GST were associated with lower peak GH responses. Therefore, stratification of GH responsiveness by the degree of glycemia would be helpful to clinicians in interpreting the GST results in patients with impaired glucose tolerance and diabetes mellitus. It is also noteworthy that there have been no prospective studies examining the accuracy and reliability of any GH stimulation tests in a TBI cohort per se. Because these data are currently unavailable, caution should be exercised within the appropriate clinical suspicion for each patient when interpreting the GH stimulation test results, particularly the GST, in TBI patients.
The absence of lesions in imaging modalities such as head computed tomography and head MRI do not rule out postTBI endocrinopathies, while screening every TBI patient is also neither practical nor feasible. During the acute phase of TBI, stress response and drugs such as etomidate, propofol, and pentobarbital can result in hormonal changes. Patients with mild TBI are not routinely evaluated for endocrinopathies in the acute stage, unless suggestive symptoms are present. Furthermore, as there is no evidence that GH, thyroid and gonadotropin replacement benefits in the early period after TBI, patients do not need to be routinely screened for these hormones. Conversely, any patient with signs of adrenal insufficiency, TSH deficiency and AVP deficiency (refractory hypotension, hypoglycemia, hyponatremia, hypernatremia and polyuria) should be screened and promptly treated to avoid lifethreatening complications, such as adrenal crisis and electrolyte abnormalities.
The presence of diffuse axonal injury, skull fracture, basal skull fracture, diffuse brain swelling, evacuated hematoma, and multiple contusions may be risk factors for long-term endocrinopathies3 and should be considered for screening. Patients with moderateto-severe TBI and clinical signs or symptoms of endocrinopathies should be screened.37 Patients with complicated mild TBI (i.e., requiring hospitalization for > 24 hours, requiring intensive care unit admission, older age, an abnormal head CT scan demonstrating brain swelling, basal skull fracture, epidural/subdural hematomas, cranial vault fracture, evidence of hypoxia/hypotension, history of repetitive injuries, and/or polyuria/polydipsia during the acute phase) should be screened.38 Patients not admitted, or admitted for < 48 hours, who demonstrate features of endocrinopathies also require screening.39 Screening can be performed ~3 months and 1 year post-TBI, and further endocrinological screening after 1 year can be performed if symptoms persist.14
Some investigators have suggested measuring morning cortisol values for the first 7 days after TBI,40 while others have recommended measurement of cortisol on days 1–4 and on days 5–10 post injury in the setting of clinical suspicion.38
TBI patients presenting with features suggestive of adrenal insufficiency or water/electrolyte abnormalities
ACUTE PHASE
• Electrolytes and 8 AM ACTH and 8 AM cortisol
• If suspicious of AVP-deficiency, check electrolytes, serum and urine osmolality, initiate symptomatic treatment
• Refer to endocrinology for further testing if necessary and/or if there is clinical suspicion of endocrinopathies
CHRONIC PHASE 3-6 MONTHS POST-INJURY
• Any TBI patient admitted > 48 hours or symptomatic patients (fatigue, low mood, poor appetite, poor motivation, weight gain, sexual dysfunction in men, menstrual disturbances in women) who were not admitted or admitted < 48 hours should be evaluated
• Electrolytes, free T4, TSH, 8 AM ACTH, 8 AM cortisol, LH, FSH, testosterone (men), estradiol (women)
• If suspicious of AVP-deficiency, check electrolytes, serum and urine osmolality, initiate symptomatic treatment
• Refer to endocrinology for further testing if necessary and/or if there is clinical suspicion of endocrinopathies
• Electrolytes, free T4, TSH, 8 AM ACTH, 8 AM cortisol, IGF-I, LH, FSH, testosterone (men), estradiol (women)
• If suspicious of AVP-deficiency, check electrolytes, serum and urine osmolality, initiate symptomatic treatment
• Refer to endocrinology for further testing if necessary and/or if there is clinical suspicion of endocrinopathies
• If hypopituitarism present and GHD suspected, optimize other hormone deficiencies for 3-6 months, then perform GH stimulation test and if GHD is confirmed, then start GH replacement
• Symptomatic patients or those diagnosed with endocrinopathies, reassess annually for 5 years
They subsequently recommend retesting at discharge (at least 2 weeks post-TBI), at 6 months, and again at 12 months. If hormonal deficiency persists at 1 year, yearly reassessment until 5 years is suggested.38 By contrast, the British Neurotrauma group, a group of neurosurgeons, recommended screening after 1 year only if patients are symptomatic; otherwise, no further action is required.39 For GHD and ACTH deficiency, dynamic endocrine tests are often required to confirm diagnosis (Figure 1). Thyrotropin and gonadotropin deficiencies are diagnosed by measuring TSH, free thyroxine, luteinizing hormone, follicle stimulating hormone, estradiol for females and testosterone for males, while the diagnosis of AVP deficiency is made by low values of serum osmolality and urine osmolality in the presence of polyuria (urine output > 3-4L/day or 50 mL/kg/day) (Figure 1). In the chronic phase, patients with moderate–severe TBI and symptomatic patients with mild TBI should be screened41, 42 (Figure 1). The ideal timing for screening for endocrinopathies is controversial, but can be between 3 and 6 months in the chronic phase.42 Patients with abnormal tests at this time point should be referred to endocrinology for further hormonal assessment and any needed dynamic testing.42 The next time point for screening should be at 12 months after TBI,15 as, by this time, transient alterations may be corrected and new deficiencies may be identified.
Given the lack of consensus on the timing of screening, multidisciplinary evidence-based guidelines on the diagnosis and management of post-TBI endocrinopathies are urgently needed and should include indications, timing, and methods for screening and testing as well as providing objective measurements of clinical treatment response.
An algorithm would be helpful to provide to primary care, family medicine and emergency care physicians, neurologists, physical medicine and rehabilitation specialists, and other clinicians to identify patients on who and how TBI patients should be screened.
The occurrence of endocrinopathies should no longer be a forgotten clinical entity, with accumulating data identifying endocrinopathies as an important contributing factor affecting the prognosis and quality of life of post-TBI patients. Therefore, it is imperative that health care providers think about this complication as symptoms can range from subtle to severe and can be mistakenly thought to be due to the TBI itself.
Notwithstanding the challenges associated with screening and diagnosing post-TBI endocrinopathies, there is also an unmet need for consensus guidelines for screening methodologies to be implemented as standard of care in all TBI patients so that correct detection and appropriate treatment can be implemented.
1.Dewan MC, Rattani A, Gupta S, et al. Estimating the global incidence of traumatic brain injury. J Neurosurg. 2018;130:1080-1097.
2.Emelifeonwu JA, Flower H, Loan JJ, McGivern K, Andrews PJD. Prevalence of anterior pituitary dysfunction twelve months or more following traumatic brain injury in adults: a systematic review and meta-analysis. J Neurotrauma. 2020;37:217-226.
3.Lauzier F, Turgeon AF, Boutin A, et al. Clinical outcomes, predictors, and prevalence of anterior pituitary disorders following traumatic brain injury: a systematic review. Crit Care Med. 2014;42:712-721.
4.Schneider HJ, Kreitschmann-Andermahr I, Ghigo E, Stalla GK, Agha A. Hypothalamopituitary dysfunction following traumatic brain injury and aneurysmal subarachnoid hemorrhage: a systematic review. JAMA. 2007;298:1429-1438.
5.Branch AFHS. Traumatic Brain Injury (TBI): DoD Standard Surveillance Case Definition for TBI adapted for AFHSB Use 2015. .
6.Chou R, Totten AM, Carney N, et al. Predictive utility of the total Glasgow Coma Scale versus the motor component of the Glasgow Coma Scale for identification of patients with serious traumatic injuries. Ann Emerg Med. 2017;70:143-157 e146.
7.Bodien YG, Barra A, Temkin NR, et al. Diagnosing level of consciousness: the limits of the Glasgow Coma Scale total score. J Neurotrauma. 2021;38:3295-3305.
8.Cicero MX, Cross KP. Predictive value of initial Glasgow coma scale score in pediatric trauma patients. Pediatr Emerg Care. 2013;29:43-48.
9.Nesiama JA, Pirallo RG, Lerner EB, Hennes H. Does a prehospital Glasgow Coma Scale score predict pediatric outcomes? Pediatr Emerg Care. 2012;28:1027-1032.
10.Caputo M, Mele C, Prodam F, Marzullo P, Aimaretti G. Clinical picture and the treatment of TBI-induced hypopituitarism. Pituitary. 2019;22:261-269.
11.Rabinowitz AR, Levin HS. Cognitive sequelae of traumatic brain injury. Psychiatr Clin North Am. 2014;37:111.
12.McInnes K, Friesen CL, MacKenzie DE, Westwood DA, Boe SG. Mild Traumatic Brain Injury (mTBI) and chronic cognitive impairment: A scoping review. PLoS One. 2017;12:e0174847.
13.Yuen KCJ, Biller BMK, Radovick S, et al. American Association of Clinical Endocrinologists and American College of Endocrinology Guidelines for Management of Growth Hormone Deficiency in Adults and Patients Transitioning from Pediatric to Adult Care. Endocr Pract. 2019;25:1191-1232.
14.Gray S, Bilski T, Dieudonne B, Saeed S. Hypopituitarism after traumatic brain injury. Cureus. 2019;11:e4163.
15.Gasco V, Cambria V, Bioletto F, Ghigo E, Grottoli S. Traumatic brain injury as frequent cause of hypopituitarism and growth hormone deficiency: epidemiology, diagnosis, and treatment. Front Endocrinol (Lausanne). 2021;12:634415.
16.Tanriverdi F, De Bellis A, Ulutabanca H, et al. A five year prospective investigation of anterior pituitary function after traumatic brain injury: is hypopituitarism long-term after head trauma associated with autoimmunity? J Neurotrauma. 2013;30:1426-1433.
17.Olivecrona Z, Dahlqvist P, Koskinen LO. Acute neuro-endocrine profile and prediction of outcome after severe brain injury. Scand J Trauma Resusc Emerg Med. 2013;21:33.
18.Tanriverdi F, Senyurek H, Unluhizarci K, Selcuklu A, Casanueva FF, Kelestimur F. High risk of hypopituitarism after traumatic brain injury: a prospective investigation of anterior pituitary function in the acute phase and 12 months after trauma. J Clin Endocrinol Metab. 2006;91:2105-2111.
19.Kgosidialwa O, Agha A. Hypopituitarism post traumatic brain injury (TBI): review. Ir J Med Sci. 2019;188:1201-1206.
20.Gilis-Januszewska A, Kluczynski L, Hubalewska-Dydejczyk A. Traumatic brain injuries induced pituitary dysfunction: a call for algorithms. Endocr Connect. 2020;9:R112-R123.
21.Vespa PM, Nuwer MR, Nenov V, et al. Increased incidence and impact of nonconvulsive and convulsive seizures after traumatic brain injury as detected by continuous electroencephalographic monitoring. J Neurosurg. 1999;91:750-760.
22.Gordon MB, Levy RA, Gut R, Germak J. Trends in growth hormone stimulation testing and growth hormone dosing in adult growth hormone deficiency patients: results from the ANSWER Program. Endocr Pract. 2016;22:396-405.
23.Macrilen FDA approval history. https://wwwdrugscom/history/macrilenhtml. Accessed December 10, 2023.
24.Garcia JM, Biller BMK, Korbonits M, et al. Macimorelin as a diagnostic test for adult GH deficiency. J Clin Endocrinol Metab. 2018;103:3083-3093.
25.Garcia JM, Swerdloff R, Wang C, et al. Macimorelin (AEZS-130)-stimulated growth hormone (GH) test: validation of a novel oral stimulation test for the diagnosis of adult GH deficiency. J Clin Endocrinol Metab. 2013;98:2422-2429.
26.Garcia JM, Biller BMK, Korbonits M, et al. Sensitivity and specificity of the macimorelin test for diagnosis of AGHD. Endocr Connect. 2021;10:76-83.
27.Global News Wire. https://wwwglobenewswirecom/en/news-release/2022/08/29/2505902/0/en/ Aeterna-Zentaris-Set-to-Regain-Full-Rights-to-Macrilen-Macimorelin-in-U-S-and-Canada-from-NovoNordiskhtml. Accessed August 6, 2023.
28.Yuen KC, Biller BM, Molitch ME, Cook DM. Clinical review: Is lack of recombinant growth hormone (GH)-releasing hormone in the United States a setback or time to consider glucagon testing for adult GH deficiency? J Clin Endocrinol Metab. 2009;94:2702-2707.
29.Berg C, Meinel T, Lahner H, Yuece A, Mann K, Petersenn S. Diagnostic utility of the glucagon stimulation test in comparison to the insulin tolerance test in patients following pituitary surgery. Eur J Endocrinol. 2010;162:477-482.
30.Tavares AB, Seixas-da-Silva IA, Silvestre DH, Paixao CM, Jr., Vaisman M, Conceicao FL. Potential risks of glucagon stimulation test in elderly people. Growth Horm IGF Res. 2015;25:53-56.
31.Toogood A, Brabant G, Maiter D, et al. Similar clinical features among patients with severe adult growth hormone deficiency diagnosed with insulin tolerance test or arginine or glucagon stimulation tests. Endocr Pract. 2012;18:325-334.
32.Yuen KC, Biller BM, Katznelson L, et al. Clinical characteristics, timing of peak responses and safety aspects of two dosing regimens of the glucagon stimulation test in evaluating growth hormone and cortisol secretion in adults. Pituitary. 2013;16:220-230.
33.Dichtel LE, Yuen KC, Bredella MA, et al. Overweight/obese adults with pituitary disorders require lower peak growth hormone cutoff values on glucagon stimulation testing to avoid overdiagnosis of growth hormone deficiency. J Clin Endocrinol Metab. 2014;99:4712-4719.
34.Diri H, Karaca Z, Simsek Y, et al. Can a glucagon stimulation test characterized by lower GH cut-off value be used for the diagnosis of growth hormone deficiency in adults? Pituitary. 2015;18:884-892.
35.Wilson JR, Utz AL, Devin JK. Effects of gender, body weight, and blood glucose dynamics on the growth hormone response to the glucagon stimulation test in patients with pituitary disease. Growth Horm IGF Res. 2016;26:24-31.
36.Hamrahian AH, Yuen KC, Gordon MB, Pulaski-Liebert KJ, Bena J, Biller BM. Revised GH and cortisol cutpoints for the glucagon stimulation test in the evaluation of GH and hypothalamic-pituitary-adrenal axes in adults: results from a prospective randomized multicenter study. Pituitary. 2016;19:332-341.
37.Agha A, Rogers B, Mylotte D, et al. Neuroendocrine dysfunction in the acute phase of traumatic brain injury. Clin Endocrinol (Oxf). 2004;60:584-591.
38.Tanriverdi F, Schneider HJ, Aimaretti G, Masel BE, Casanueva FF, Kelestimur F. Pituitary dysfunction after traumatic brain injury: a clinical and pathophysiological approach. Endocr Rev. 2015;36:305-342.
39.Tan CL, Alavi SA, Baldeweg SE, et al. The screening and management of pituitary dysfunction following traumatic brain injury in adults: British Neurotrauma Group guidance. J Neurol Neurosurg Psychiatry. 2017;88:971-981.
40.Glynn N, Agha A. Which patient requires neuroendocrine assessment following traumatic brain injury, when and how? Clin Endocrinol (Oxf). 2013;78:17-20.
41.Ghigo E, Masel B, Aimaretti G, et al. Consensus guidelines on screening for hypopituitarism following traumatic brain injury. Brain Inj. 2005;19:711-724.
42.Tritos NA, Yuen KC, Kelly DF, Neuroendocrine A, Pituitary Scientific C. American Association of Clinical Endocrinologists and American College of Endocrinology Disease State Clinical Review: A neuroendocrine approach to patients with traumatic brain injury. Endocr Pract. 2015;21:823-831.
Dr. Kevin CJ Yuen is a Professor of Medicine at the University of Arizona College of Medicine and Creighton School of Medicine, and Medical Director of the Barrow Pituitary Center at the Barrow Neurological Institute in Phoenix, AZ. The focus of his clinical practice is on the diagnosis and treatment of pituitary and adrenal disorders, with a particular interest in acromegaly, Cushing's syndrome, growth hormone deficiency, and endocrinopathies caused by traumatic brain injury and immune checkpoint inhibitors.
Dr. Yuen leads the Pituitary section of the Endocrinology Fellowship program at his institution, and has conducted many growth hormone dosing and diagnostic studies, long-acting growth hormone formulation studies, and trials with new drug therapies for acromegaly and Cushing syndrome. He has published extensively, authored book chapters, and is a frequent guest speaker on various topics related to pituitary disorders. Dr. Yuen serves on several national and international committees and advisory boards on pituitary diseases. He is the current Editor-inChief of the Growth Hormone and IGF Research journal, and is also on the editorial boards of several other Endocrinology journals.
Vinland Center provides drug and alcohol treatment for adults with
Megan Herodes, BS, CSP • Jose Garcia, MD, PhD
Abstract
Diagnosis and treatment of pituitary hormone abnormalities following traumatic brain injury (TBI), a condition known as posttraumatic hypopituitarism (PTHP), poses several unique challenges in the military. When compared to civilian populations, service members and Veterans suffer from higher numbers of lifetime head injuries. These injuries very frequently are a result of a blast. Military populations suffer from notably more illnesses and disorders (PTSD, depression) than civilian populations with symptoms that overlap with both persistent post-concussion symptoms and symptoms of PTHP. This review summarizes the pathophysiology of blast TBI, the common comorbidities in military and Veteran populations, and the intersection and impact of these topics on PTHP.
Approximately 2.5 million Americans, less than 1% of the population of the USA, each year sustain a traumatic brain injury (TBI), an injury caused by an external force that disrupts normal function of the brain, and approximately 3.2-5.3 million people are living with a long-term disability due to this injury.1 Within the military, the incidence of TBI is much higher. Between 2000 and 2021, 1.5% of active duty service members (ADSM) (20,641) sustained a TBI each year, over three times the rate in civilian populations (Traumatic Brain Injury Center of Excellence.2 These individuals are more likely to be discharged from service due to a diagnosis of TBI than from any other types of injury, with an adjusted odds ratio of 3.94-3.99.3 Incidence of TBI among those ADSM that have served in combat is even higher with estimates ranging from 15-23%.4,5,6
These estimates of TBI sustained during combat are likely underreported. The tempo of combat theatre does not always allow for ADSM to report mild injuries or receive care at time of injury, as is more common in civilian TBI.7
Further, ADSM on combat deployment are frequently in highstress situations, both physiologically and emotionally, and are not receiving adequate sleep. These confounding factors likely impact how these service members both recognize that they have sustained a TBI and remember the details surrounding the injury.7 Military TBI tends to happen at a younger age and predominantly in men due to the age cut-offs for military service and the gender composition of the US military.8
Severity of TBI is typically divided into mild, moderate, and severe TBI, with greater severity reflecting lower Glasgow Coma Score (GCS), longer duration of loss of consciousness and of posttraumatic amnesia.9 In both civilian and military populations, the most common severity of TBI is mild, with approximately 75% of civilian head injuries and 83% of military head injuries classified as mild.1,2 The frequency of head injury, however, is notably higher among ADSM when compared to civilian populations. In a meta-analysis of recurrent traumatic brain injuries in civilian populations, Lasry and colleagues found great variability in recurrence risk (0.4-42%), and concluded that studies with the largest sample sizes reported 7-36% of people with an initial TBI would later go on to have at least one more TBI in their lifetime.10 Among ADSM, this risk rises. Sixty percent of ADSM who have sustained one TBI had at least one additional lifetime TBI, 47% had at least two additional lifetime TBIs, and 33% had at least three additional TBIs.11 ADSM are also frequently exposed to sub concussive head injuries primarily due to low-level blasts.5
These injuries are obtained in deployed settings through improvised explosive devices (IED) and training and, over time, can result in neurophysiological and neuropsychological changes.13-16
Mechanisms of injury differ greatly between civilian TBI populations and military populations. The greatest cause of TBI in civilian populations are falls (35%) followed by motor-vehicle accidents (17%), struck by/against events (17%), and assaults (10%).16 The vast majority of military TBI is due to explosions or blast TBI (bTBI). According to a survey of US soldiers returning from Operation Iraqi Freedom, 72% of TBI’s incurred during this deployment were blastrelated.17 The pathophysiology of bTBI tends to be more complicated than non-blast TBI and the injuries are rarely isolated to the blast itself.7 The initial blast comes from the detonation of high-energy explosives which produces overpressurization shock waves travelling at the speed of sound.18,19 Theories as to how these shock waves result in a brain injury are multitudinous and heavily debated including direct neural and axonal injury, vascular propagation, neuroinflammation, and a higher rate of acceleration/deceleration of the head.20,21,22,23 Following the initial explosion, additional injuries can result from flying debris (secondary), body displacement in which the ADSM is thrown due the force of the explosion striking other surfaces (tertiary), and other consequences (quaternary) such as crush injuries from structure collapse, burns, and radiation poisoning.7,19
The long-term medical consequences of military service and injury are extensive. The US Veterans Health Administration (VHA) reports that over 9 million Veterans receive healthcare in some capacity through the Veterans Affairs Health Care program.24 Veteran medical cases are typically more complicated with Veterans suffering from significantly poorer health and more severe symptoms than civilian populations25 In a retrospective record review of almost 4.5 million Veterans, over a quarter of these patients (1.15 million) were diagnosed with at least one mental health condition within 12 months of enrollment in VHA. Depression was most frequently diagnosed (14%) with 9.3% of Veterans diagnosed with posttraumatic stress disorder (PTSD), 8% diagnosed with a substance use disorder (SUD), 5% diagnosed with an anxiety disorder, and 4% diagnosed with a serious mental illness such as bipolar disorder (BPD) or schizophrenia.26 Veterans also have a high rate of cooccurrence of these disorders with depression co-occurring with other diagnoses at a rate of 30-55% and SUD co-occurring at least 22% of the time.26 Chronic pain (pain lasting greater than 3-6 months) is another common comorbidity in both ADSM and Veteran populations with an estimated prevalence of 24-37%27 and 25%,28 respectively. In a recent study of younger post-9/11 Veterans, Kennedy and colleagues found that Veterans with a history of TBI are at greater risk for early onset dementia; risk increases with greater TBI severity.29 Comorbidities are further complicated and, in some cases exacerbated by, additional symptoms due to TBI.30 These disorders impact ADSM and Veteran vocational and interpersonal outcomes and recovery from other disorders.31,32,33,34
PTHP, the inability of the pituitary to produce one or more pituitary hormones following traumatic brain injury35 has long been known as a sequela of TBI.36
Within the last 15 years, it has become clear that PTHP is more prevalent and less transient than originally believed.37 Military populations are no exception. The symptoms of PTHP are nonspecific and heavily overlap with those of TBI, persistent post concussion symptoms, and other common comorbidities in the military (Figure 1) likely delaying identification and treatment.37
Despite a high prevalence and symptom burden of PTHP in military populations, very little research has investigated the disorder in ADSM and Veteran populations. A prospective study comparing hormone abnormalities before and up to three years following deployment found that 48% of ADSM who had sustained a TBI while deployed may have at least one pituitary hormone deficiency. However, this study was limited as hormone levels were drawn from non-fasting banked samples without mention of time of sampling, provocative testing for growth hormone deficiency (GHD) and adrenal insufficiency (AI) were not performed, and normal ranges for prolactin, cortisol, and testosterone were not provided.38 In a separate, single-site, small study, Veterans who were 15-504 months post-injury, were slightly less likely to display PTHP with 38% of patients found to be deficient in at least one pituitary hormone.39 Blast TBI seems to account for many of these cases. When comparing prevalence of PTHP in ADSM with a history of bTBI to non-blast TBI, 31-32% of ADSM had PTHP following TBI while ADSM with a history of non-blast TBI had a prevalence of 3-15%40-41 Clinical presentation and treatment response for hormone deficiencies are further complicated in military populations. Many symptoms of common comorbidities in military and Veteran populations overlap with symptoms of hormone deficiencies including symptoms of PTSD, depression, anxiety, substance abuse, and chronic pain which may exacerbate these common symptoms (Figure 1).
GHD is the most common pituitary hormone abnormality following TBI in military and Veteran populations with reports of confirmed GHD ranging between 21%-25%39-41 While mechanisms are again heavily debated, the most accepted explanation is the location of the GH-secreting cells, somatotrophs, in the anterior pituitary. The long hypophyseal portal veins both connect the hypothalamus to the anterior pituitary and provide the majority of the blood supply to the anterior pituitary with the remaining blood to the pituitary supplied by the short portal vessels. As the name suggests, the long hypophyseal portal veins are long vessels that travel from the hypothalamus, through the infundibulum, to the anterolateral pituitary with no significant redundancies in the system to compensate for obstruction or damage to this vessel. Somatotrophs are located laterally and receive most of their blood supply from the long hypophyseal portal veins. During TBI, the infundibulum and the hypothalamus are very susceptible to ischemic injury resulting in anterior pituitary cell death, especially among somatotrophs.43 Imaging studies within the military support this theory. Castellano and colleagues found that ADSM with a history of mTBI had lower anterior pituitary volume than ADSM without a history of TBI and this reduced volume was associated with lower insulin-like growth factor-1 (IGF-1) values, one marker of GH secretion.44
ADSM with GHD following mTBI have been found to have worsened executive functioning, quality of life (QoL), and greater depression,42 and preliminary data on GHRT suggest that these may be responsive to treatment.
Low Exercise Tolerance
Low BMD Anemia
Loss of Body Hair
Muscle Loss
Increased Body Fat
Muscle Weakness
Hypotension
Hot Flashes
Oligomenorrhea/Amenorrhea
Infertility
Muscle Cramps
Constipation
Cold Intolerance
Breast Atrophy
Testicular Atrophy
PTHP Symptoms
Wrinkles around the Eyes and Mouth
Decreased Insulin Sensitivity
High Cholesterol
Poor Thermoregulation
Fever
Malaise
Hirsutism
Gynecomastia
Galactorrhea
Hypertension
Apathy
Sexual Dysfunction
Dry Skin
Low Quality of Life
Weight Gain
Social Isolation
Weight Loss
Pale Skin
Hypervigilance
Poor
Nausea
Dizziness
Post Concussion Symptoms
Balance
Coordination
Light Sensitivity
Noise
Sensitivity
Anxiety
Low Self-Esteem Depression
Poor Problem-Solving
Poor
Irritability
Cognitive Impairment
Headaches
Other Common Comorbidity Symptoms
Exaggerated Startle Response
Self-Destructive Behavior
Nightmares
Psychomotor Retardation
Pain Lasting Longer than 3-6 Months
Personality Changes
Paranoia
Suicidal Ideation
Restlessness
Tremors
Numbness and Tingling
Change in Taste and Smell
Vision Problems
Psychosis
Flashbacks
Muscle Tension
Anti-Social Behavior
American Psychological Association (APA), 2022a; APA, 2022b; Charmandari, Nicolaides, and Chrousos, 2014; Ciucă et al., 2023; Feldt-Rasmussen et al., 2022; Fine, 2011; Gudmundsdottir and Schlechte, 2002; Jabbour, 2003; Khera et al., 2016; Vanderploeg et al., 2015; Wand, 2003; West and Sharp, 2014
American Psychological Association (APA), 202245; APA, 202246; Charmandari, Nicolades, and Chrousos, 201468; Clucå et al., 202347; Feldt-Rasmussen et al., 202248; Fine, 201149; Gudmundsdottir and Schlechte, 200250; Jabbour, 200351; Khera et al., 201652; Vanderploeg et al., 201553; Wand, 200354
In addition to improved aspects of executive functioning and depression, studies have shown that military and Veteran populations who have been treated for GHD with GH replacement therapy (GHRT) display greater muscle strength and mass and improved cardiorespiratory capacity, memory, fatigue, and symptoms of PTSD.55-56 While these preliminary studies are uncontrolled and limited by small sample size, these results are encouraging and support the need for larger, more robust studies on the treatment of GHD in military TBI populations. One such multisite study in progress within the VA Cooperative Studies Program, Growth Hormone Replacement Therapy in Veterans with mTBI and AGHD: The GRIT Study, aims to identify and treat Veterans of Operations Enduring Freedom, Iraqi Freedom, and New Dawn (OEF/OIF/OND) who sustained an mTBI during combat and are now suffering from GHD. Following 6 months of treatment, efficacy, safety, and tolerance will be assessed including such domains as quality of life, body composition, sleep and mood disturbances, and cognitive functioning.57-58
If indicated by clinical presentation of GHD and low to normal basal IGF-1 at least one year following the most recent head injury, it is recommended that Veterans and ADSMs undergo provocative testing.59 There are a number of provocative test options including insulin tolerance test, growth hormone releasing hormone (GHRH) + arginine, GHRH + growth hormone releasing peptide-6, macimorelin, and glucagon stimulation test (GST).59, 63-64 While these tests have not been standardized in military populations, they have been used to confirm GHD in Veterans39, 55 and the standardization of macimorelin for the detection of GHD was composed, in part, by Veterans.62
GHD in this population is more likely to be partial, especially among ADSMs and Veterans with a history of exclusively mTBI and will likely need lower doses of GHRT as a result.65
Research on other anterior pituitary hormone deficiencies following TBI, especially military TBI, is scarce and focused largely on prevalence. The pathophysiology is presumed to be similar.
However, corticotrophs, gonadotrophs, lactotrophs, and thyrotrophs are located more centrally in the anterior pituitary68 and may be more protected from vascular and structural damage resulting in a lower prevalence of other anterior pituitary hormone deficiencies than of GHD.67 Secondary adrenal insufficiency (AI) is one such deficiency. Approximately 15-22% of ADSMs and Veterans with a history of TBI have been reported to have AI39-40 Symptoms of secondary AI are similar to primary AI but may be less severe and are less likely to result in adrenal crisis68. Symptoms of AI may include fatigue, weight loss, muscle weakness, dizziness, and dry skin.68
Prevalence of hyperprolactinemia in a military TBI population is slightly lower than GHD and secondary AI with estimates at 9-15%.39 - 40 Prolactin abnormalities related to PTHP are unique from other hormone deficiencies in that TBI may result in an increase in circulating prolactin levels. The hypothalamus controls prolactin secretion by releasing dopamine into the pituitary through the long hypophyseal portal veins running through the infundibulum which inhibits the release of prolactin from the anterior pituitary.
When the infundibulum and the hypophyseal portal veins are damaged, the amount of dopamine that reaches the anterior pituitary is decreased. As such, inhibition of prolactin release is decreased allowing the pituitary to release more prolactin.66 Symptoms are gender-specific and include galactorrhea, symptoms of hypogonadism such as menstrual abnormalities, sexual dysfunction, infertility, and hirsutism.69 More rarely, hypoprolactinemia has also been found but its true prevalence is unknown and likely underreported.70
Secondary hypogonadism and hypothyroidism appear to be much less common following military TBI, 3-8% and 0% respectively.39-40
Secondary hypogonadism is characterized by impaired release of luteinizing hormone (LH) and follicle-stimulating hormone (FSH) in the pituitary resulting in decreased production of testosterone and estrogen by the testes and ovaries, respectively.61 These can also be contributed at least in part by deficiencies in hypothalamic input.62 Secondary hypothyroidism is similar in that it is caused by decreased release of thyroid stimulating hormone (TSH) from the pituitary resulting in decreased production of thyroxine or T4 from the thyroid.63 In both cases, the levels of LH/FSH or TSH may be low or inappropriately normal, respectively. Key symptoms of secondary hypogonadism include menstrual abnormalities, sexual dysfunction, low libido, low exercise tolerance, poor quality of life, and mood disorders while secondary hypothyroidism is characterized by fatigue, depression, cognitive impairment, cold intolerance, weight gain, and sleep disturbances among others.74
At time of writing, there are no studies investigating symptom and treatment paradigms of AI, hyperprolactinemia, and hypogonadism and hypothyroidism among individuals with a history of TBI within the military. Additionally, studies on prevalence of individual hormone deficiencies following TBI in the military use different diagnostic criteria and are few and limited by small sample size. Diagnosis of the hormone abnormalities and current hormone replacement recommendations are the same as for hormone deficiencies as due to non-TBI causes despite the lack of evidence of the adequacy of this practice.68-69, 73, 71 These deficits tend to be partial and isolated.63
It is recommended that all patients with pituitary hormone abnormalities undergo MRI imaging of the sella turcica to rule out other causes of these abnormalities,61 however, there are some common contraindications within the military that may preclude the use of MRI such as presence of shrapnel and claustrophobia, among others. Other forms of imaging may be needed for these patients. Common military comorbidities should also be taken into consideration when treating PTHP. Clinical cases of military personnel are typically more complicated than civilian cases and include increased occurrence of PTSD, BPD, suicidal ideation, substance abuse, and early onset dementia.29, 25 Predicting how patients will respond to treatment is challenging as the interaction between comorbidities and hormone replacement therapy is unknown, and many drug studies exclude participants with some of these diagnoses and symptoms.
PTHP is prevalent in military and Veteran TBI populations. Research on this topic is limited. Large-scale studies within military populations are needed on prevalence, clinical presentation, diagnostic strategy and response to treatment in all hormone deficiencies but especially in GHD and partial secondary AI, the most common abnormalities.
Studies are also desperately needed to assess the impact and interaction of hormone replacement therapy and common military comorbidities that are not always included in clinical research trials such as PTSD, BPD, suicidality, substance abuse, and dementia as these comorbidities may limit the degree to which an ADSM or Veteran is able to respond to treatment. Patients with chronic pain, for example, may not experience the same changes in low exercise tolerance following HRT as other patients because chronic pain can limit the amount of exercise a patient is able to do. This information is lacking in the literature and will be an immense help to providers treating ADSM and Veterans. With the ever-increasing numbers of OEF/OIF/OND ADSM retiring from service, more studies are needed on the long-term effects of TBI, especially blast TBI, and its interaction with anteriorpituitary hormones. OEF, OIF, and OND saw unprecedented incidence of blast injury.75 As we proceed into the next decades, PTHP is likely to impact a greater number of Veterans. As this population ages, other common age-related comorbidities such as dementia and diabetes will become more prevalent and may interact with PTHP and its treatment.76-78 This research is needed now to anticipate the future burden of PTHP on Veterans and the VA healthcare system and to identify the best course of treatment in these complicated medical cases.
1. Centers for Disease Control and Prevention. (2015). Report to Congress on Traumatic Brain Injury in the United States: Epidemiology and Rehabilitation. National Center for Injury Prevention and Control; Division of Unintentional Injury Prevention. Atlanta, GA.
2. Traumatic Brain Injury Center of Excellence (TBI CoE). (2023). DOD TBI Worldwide Numbers. Military Health System. https://health.mil/Military-Health-Topics/Centers-of-Excellence/Traumatic-Brain-InjuryCenter-of- Excellence/DOD-TBI-Worldwide-Numbers
3. Gubata M.E. Packnett E.R. Blandford C.D., et al., Trends in the epidemiology of disability related to traumatic brain injury in the US Army and Marine Corps: 2005 to 2010. Journal of Head Trauma Rehabilitation. 29: 65-75, 2014.
4. Hoge C.W. McGurk D. Thomas J.L., et al., Mild traumatic brain injury in U.S. soldiers returning from Iraq. New England Journal of Medicine. 358: 453-463, 2008.
5. Schell T.L. Marshall GN: Survey of individuals previously deployed for OEF/OIF. In: Invisible Sound of War: Psychological and Cognitive Injuries, Their Consequences, and Services to Assist Recovery. TL Tanielian & LH Jaycox (Eds.) Rand Corporation, Santa Monica, CA. Pages 87-116. 2009.
6. Terrio H. Brenner L.A. Ivins B.J., et al., Traumatic brain injury screening: Preliminary Findings in a US Army brigade combat team. Journal of Head Trauma Rehabilitation. 24: 14-23, 2009.
7. Chapman J.C. Diaz-Arrastia R., Military Traumatic brain injury: A review. Alzheimers Dementia. 10: S97-104, 2014.
8. Department of Defense. (2022). Department of Defense Releases Annual Demographics Report – Upward Trend in Number of Women Serving Continues. https://www.defense.gov/News/Releases/Release/ Article/3246268/department-of-defense-releases-annual-demographics-report-upward-trend-in-numbe/
9. Malec J.F. Brown A.W. Leibson C.L., et al., The mayo classification system for traumatic brain injury severity. Journal of Neurotrauma. 24: 1417-1424, 2007.
10. Lasry O. Liu E.Y. Powell G.A., et al., Epidemiology of recurrent traumatic brain injury in the general population: A systematic review. Neurology. 89: 2198-2209, 2017.
11. Jannace K. Pompeii L Ruiz de Porras D.G., et al., Lifetime traumatic brain injury and risk of post-concussive symptoms in the Millennium Cohort Study. Journal of Neurotrauma. Online ahead of print, 2023.
12. Carr W. Polejaeva E. Grome A., et al., Relation of repeated low-level blast exposure with symptomatology similar to concussion. Journal of Head Trauma Rehabilitation. 30: 37-55, 2015.
13. Carr W. Stone J.R. Walilko T., et al. Repeated low-level blast exposure: A descriptive human subjects study. Military Medicine. 181: 28-39, 2016.
14. Stone J.R. Tutison N.J. Wassermann E., et al., Neuroimaging correlates of repetitive blast exposure in experienced military breachers. Journal of Neurotrauma. 30: A120-A121, 2013.
15. Tate C.M. Wang K.K.W. Eonta S., et al., Serum brain biomarker level, neurocognitive performance, and selfreported symptoms changes in soldiers repeatedly exposed to low-level blast: A breacher pilot study. Journal of Neurotrauma. 30: 1620-1630, 2013.
16. Centers for Disease Control. (2010). Traumatic Brain Injury in the United States: Emergency Department Visits, Hospitalization and Deaths 2002-2006. https://www.cdc.gov/traumaticbraininjury/pdf/blue_book.pdf
17. Wilk J.E. Thomas J.L. McGurk D.M., et al., Mild traumatic brain injury (concussion) during combat: Lack of association of blast mechanism with persistent postconcussive symptoms. Journal of Head Trauma Rehabilitation. 25: 9-14, 2010.
18. Cernak I. Noble-Haeusslein L.J., Traumatic brain injury: An overview of pathobiology with emphasis on military populations. Journal of Cerebral Blood Flow and Metabolism. 30: 255-266, 2010.
19. DePalma R.G. Burris D.G. Champion H.R., et al., Blast injuries. New England Journal of Medicine. 352: 1335-1342, 2005.
20. Bryden D.W. Tilghman J.I. Hinds II S.R., Blast-related traumatic brain injury: Current concepts and research considerations. Journal of Experimental Neuroscience. 13: 1179069519872213, 2019.
21. Davenport N.D. Lim K.O. Armstrong M.T., et al., Diffuse and spatially variable white matter disruptions are associated with blast-related mild traumatic brain injury. Neuroimage. 59: 2017-2024, 2012.
22. Goldstein L.E. Fisher A.M. Tagge C.A., et al., Chronic traumatic encephalopathy in blast-exposed military veterans and a blast neurotrauma mouse model. Science Translational Medicine. 4: 134ra60, 2012.
23. Przekwas A. Somayaji M.R. Gupta R.K., Synaptic mechanisms of blast-induced brain injury. Frontiers in Neurology. 7: 2, 2016.
24. Veterans Health Administration. (2023, November 8). About VHA. U.S. Department of Veterans Affairs. https://www.va.gov/health/aboutvha.asp#:~:text=The%20Veterans%20Health%20AdministrAdmin%20 (VHA,Veterans%20enrolled%20in%20the%20VA
25. Rogers W.H. Kazis L.E. Miller D.R., et al., Comparing the health status of VA and non-VA ambulatory patients: The veterans’ health and medical outcomes study. Journal of Ambulatory Care Management. 27: 249-262, 2004.
26. Trivedi R.B. Post E.P. Sun H., et al., Prevalence, comorbidity, and prognosis of mental health among US veterans. American Journal of Public Health. 105: 2564-2569, 2015.
27. Smith H.J. Taubman S.B. Clark L.L., A burden and prevalence analysis of chronic pain by distinct case definitions among active duty U.S. military service members, 2018. Pain Physician. 23: E429-E440, 2020.
28. Van Den Kerkhof E.G. Carley M.E. Hopman W.M., Prevalence of chronic pain and related risk factors in military veterans: A systematic review. JBI Database of Systematic Reviews and Implementation Reports. 12: 152-186, 2014.
29. Kennedy E. Panahi S. Stewart I.J., et al., Traumatic brain injury and early onset dementia in post 9-11 veterans. Brain Injury. 36: 620-627, 2022.
30. Klyce D.W. West S.J. Perrin P.B., et al., Network analysis of neurobehavioral and post-traumatic stress disorder symptoms one year after traumatic brain injury: A Veterans Affairs traumatic brain injury model system. Journal of Neurotrauma. 38: 3332-3340, 2021.
31. Asnaani A. Reddy M.K. Shea M.T., The impact of PTSD symptoms on physical and mental health functioning in returning veterans. Journal of Anxiety Disorders. 28: 310-317, 2014.
32. Kukla M. Rattray N.A. Salyers M.P., Mixed methods study examining work reintegration experiences from perspectives of veterans with mental health disorders. Journal of Rehabilitation Research and Development. 52:477-490, 2015.
33. Possemato K. Wade M. Andersen J., et al., The impact of PTSD, depression, and substance use disorders on disease burden and health care utilization among OEF/OIF veterans. Psychological Trauma: Theory, Research, Practice, and Policy. 2: 218, 2010.
34. Ramchand R. Rudavsky R. Grant S., et al., Prevalence of, risk factors for, and consequences of posttraumatic stress disorder and other mental health problems in military populations deployed to Iraq and Afghanistan. Current Psychiatry Reports. 17: 37, 2015.
35. Kałas M. Miksiewicz M. Kowalke A., et al., Post-traumatic hypopituitarism: A neglected consequence of traumatic brain injury. Neuroendocrinology. 113: 579-588, 2023.
36. Cyran E., Hypophysenschädigung durch Schädelbasisfraktur. Deutsche Medizinische Wochenschrift. 44: 1261-1270, 1918.
37. Quinn M. Agha A., Post-traumatic hypopituitarism – Who should be screened, when, and how?. Frontiers in Endocrinology. 9: 8, 2018.
38. Ciarlone S.L. Statz J.K. Goodrich J.A., et al., Neuroendocrine function and associated mental health outcomes following mild traumatic brain injury in OEF-deployed service members. Journal of Neuroscience Research. 98:1174-1187, 2020.
39. Lee J. Anderson L.J. Migula D., et al., Experience of a pituitary clinic for US military veterans with traumatic brain injury. Journal of the Endocrine Society. 5: bvab005, 2021.
40.Baxter D. Sharp D.J. Feeney C., et al., Pituitary dysfunction after blast traumatic brain injury: The UK BIOSAP study. Annals of Neurology. 74: 527-536, 2013.
41. Undurti A. Colasurdo E.A. Sikkema C.L., et al., Chronic hypopituitarism associated with increased postconcussive symptoms is prevalent after blast-induced mild traumatic brain injury. Frontiers in Neurology. 9: 72, 2018.
42. Ioachimescu A.G. Hampstead B.M. Moore A., et al., Growth hormone deficiency after mild combatrelated traumatic brain injury. Pituitary. 18: 535-541, 2015.
43.Kgosidialwa O. Hakami O. Zia-Ul-Hussnain H.M., et al., Growth hormone deficiency following traumatic brain injury. International Journal of Molecular Sciences. 20: 3323, 2019.
44. Castellano A.K. Powell J.R. Cools M.J., et al., Relationship between anterior pituitary volume and IGF-1 serum levels in soldiers with mild traumatic brain injury history. Medicine and Science in Sports and Exercise. 54:1364-1370, 2022
45. American Psychiatric Association. Anxiety Disorders. In: Diagnostic and Statistical Manual of Mental Disorders, Fifth Edition, Text Revision. American Psychiatric Publications Incorporated, Washington, D.C.https://doi.org/10.1176/appi.books.9780890425787.x05_Anxiety_Disorders, 2022.
46. American Psychiatric Association. Depressive Disorder. In: Diagnostic and Statistical Manual of Mental Disorders, Fifth Edition, Text Revision. American Psychiatric Publications Incorporated, Washington, D.C. https://doi.org/10.1176/appi.books.9780890425787.x04_Depressive_Disorders, 2022.
47. Ciucă Anghel D.M. Nițescu G.V. Tiron A.T., et al., Understanding the mechanisms of action and effects of drug abuse. Molecules. 28: 4969, 2023.
48. Feldt-Rasmussen U. Klose M. Feingold K.R., et al., Adult growth hormone deficiency – Clinical management. In: Endotext. B Anawalt, MR Blackman, A. Boyce, et al. (eds.) MDText.com, South Dartmout, MA. 2022.
49. Fine P.G., Long-term consequences of chronic pain: Mounting evidence for pain as a neurological disease and parallels with other chronic disease states. Pain Medicine. 12: 996-1004, 2011.
50. Gudmundsdottir A. Schlechte J.A., Central Hypothyroidism. The Endocrinologist. 12: 218-223, 2002.
51. Jabbour S.A., Cutaneous manifestations of endocrine disorders: A guide for dermatologists. American Journal of Clinical Dermatology. 4: 315-331, 2003.
52. Khera M. Broderick G.A. Carson III C.C., et al., Adult-Onset Hypogonadism. May Clinic Proceedings. 91: 908-926, 2016.
53. Vanderploeg R.D. Silva M.A. Soble J.R., et al., The structure of postconcussion symptoms on the Neurobehavioral Symptom Inventory: A comparison of alternative models. Journal of Head Trauma Rehabilitation. 30: 1-11, 2015.
54. Wand G.S. Diagnosis and management of hyperprolactinemia. The Endocrinologist. 13: 52-57, 2003.
55. Herodes M. Le N. Anderson L.J., et al., Metabolic and quality of life effects of growth hormone replacement in patients with TBI and AGHD: A pilot study. Growth Hormone & IGF Research. 71: 101544, 2023.
56. Mossberg K.A. Durham W.J. Zgaljardic D.J., et al., Functional changes after recombinant human growth hormone replacement in patients with chronic traumatic brain injury and abnormal growth hormone secretion. Journal of Neurotrauma. 34: 845-852, 2017.
57. ClinicalTrials.gov. (2023). Growth Hormone Replacement Therapy in Veterans with Mild Traumatic Brain Injury (mTBI) and Adult Growth Hormone Deficiency (AGHD) (GRIT). https://clinicaltrials.gov/study/ NCT04867317
58. VA Cooperative Studies Program. (2023). CSP #2018 Growth Hormone Replacement Therapy (GRIT). https://www.vacsp.research.va.gov/CSP_2018/Growth_Hormone_Replacement_Therapy_in_Veterans_with_ mild_Traumatic_Brain_Injury_mTBI.asp
59. Hacioglu A. Kelestimur F. Tanriverdi F., Pituitary dysfunction due to sports-related traumatic brain injury. Pituitary. 22: 322-331, 2019.
60. Molitch M.E. Clemmons D.R. Malozowski S., et al., Evaluation and treatment of adult growth hormone deficiency: An Endocrine Society clinical practice guideline. Journal of Clinical Endocrinology and Metabolism. 96: 1587-1609, 2011.
61. Yuen K.C.J. Tritos N.A. Samson S.L., et al., American Association of Clinical Endocrinologists and American College of Endocrinology Disease State clinical review: Update on growth hormone stimulation testing and proposed revised cut-point for the glucagon stimulation test in the diagnosis of adult growth hormone deficiency. Endocrine Practice. 22: 1235-1244, 2016.
62. Garcia J.M., Biller B.M.K., Korbonits M., et al. Macimorelin as a diagnostic test for adult GH deficiency. Journal of Clinical Endocrinology and Metabolism. 103: 3083-3093, 2018.
63. Ghigo E. Masel B., Aimaretti G., et al., Consensus guidelines on screening for hypopituitarism following traumatic brain injury. Brain Injury. 19: 711-724, 2005.
64. Guttikonda S. Ahmadi S. Urban R.J., Pituitary dysfunction after traumatic brain injury. Expert Review of Endocrinology & Metabolism. 6: 697-703, 2011.
65. Mercier L.J. Kruger N. Le Q.B., et al., Growth hormone deficiency testing and treatment following mild traumatic brain injury. Scientific Reports. 11: 8534, 2021.
66.Ben-Shlomo A. Melmed S.: Chapter 2 – Hypothalamic regulation of anterior pituitary function. In: ThePituitary (Fourth Edition). S Melmed (Ed.) Academic Press, Cambridge, MA. Pages 23-45, 2017.
67. Vennekens A. Vankelecom H., Traumatic brain injury and resultant pituitary dysfunction: Insights from experimental models. Pituitary. 22: 212-219, 2019.
68. Charmandari E. Nicolaides N.C. Chrousos G.P., Adrenal Insufficiency. Lancet. 383: 2152-2167,
59. Capozzi A. Scambia G. Pontecorvi A., et al., Hyperprolactinemia: Pathophysiology and therapeutic approach. Gynecological Endocrinology. 31: 506-510, 2015. 2014.
60. Herrmann B.L. Rehder J. Kahlke S., et al., Hypopituitarism following severe traumatic brain injury. Experimental and Clinical Endocrinology & Diabetes. 114: 316-321, 2006.
61. Richard-Eaglin R., Male and female hypogonadism. The Nursing Clinics of North America. 53: 395-405, 2018.
62. Crompton M.R., Hypothalamic lesions following closed head injury. Brain. 94: 165-172, 1971.
63. Garber J.R. Cobin R.H. Gharib H., et al., Clinical practice guidelines for hypothyroidism in adults: Cosponsored by the American association of Clinical Endocrinologists and the American Thyroid Association. Endocrine Practice. 18: 988-1028, 2012.
64. West T.A. Sharp S., Neuroendocrine dysfunction following mild TBI: When to screen for it. The Journal of Family Practice. 63: 11-16, 2014.
65. DePalma R.G. Cross G. Beck L.B., et al., Epidemiology of mTBI (mild traumatic brain injury) due to blast: History, DOD/VA data bases: Challenges and opportunities. In: Proceedings of the NATO RTO-MP-HFM-207 Symposium on a Survey of Blast Injury across the Full Landscape of Military Science. Pages 1-8, 2011.
66. Cheng Y.J. Imperatore G. Geiss L.S., et al., Secular changes in the age-specific prevalence of diabetes among US adults: 1988-2010. Diabetes Care. 36: 2690-2696, 2013.
67. Fann J.R. Riisgaard Ribe A. Schou Pedersen H., et al., Long-term risk of dementia among people with traumatic brain injury in Denmark: A population-based observational cohort study. The Lancet Psychiatry. 5: 424-431, 2018.
68. Newman A.B. Odden M.C. Cauley J.A.: Epidemiology of Aging. In: Handbook of Epidemiology. W Ahrens & I Pigeot (Eds.) Springer, New York, NY. Pages 1-37, 2023.
Megan Herodes, BS, CSP is a board-certified specialist in psychometry and has 10 years of clinical and research experience working with adults and children with various neurological conditions. Ms. Herodes has an extensive history in clinical research on TBI rehabilitation in military and Veteran populations including her current role as national study coordinator for CSP#2018 at the VA Puget Sound Health Care System where she focuses on investigating the use of growth hormone replacement therapy for Veterans with a history of TBI.
Jose Garcia, MD, PhD is Director of the Geriatric Research, Education and Clinical Center and Clinical Research Unit at the VA Puget Sound Health Care System (VAPSHCS) and a board-certified PhysicianScientist specializing in Diabetes, Endocrinology and Metabolism with over 20 years of clinical and research experience in the field of endocrinology. In his pituitary clinic at the APSHCS, he works closely with Veterans suffering from acquired hormone deficiencies especially those following TBI. His current clinical research focuses on diagnosis and treatment of acquired growth hormone deficiencies in the setting of traumatic brain injury and Gulf War Illness as well as treatment of wasting conditions. He is a professor in the Division of Gerontology & Geriatric Medicine at the University of Washington Department of Medicine and serves as associate editor of several peer-review journals.
2024
October
25 -26 - The 52nd Annual Meeting & International Conference of the Korean Academy of Rehabilitation Medicine (KARM), October 25 – 26, 2024, Seoul, Korea. For more information, visit karm2024.org.
31 October – 3 November: 101st ACRM Annual Fall Conference, October 31 –November 3, 2024, Dallas, Texas. For more information, visit acrm.org .
November
6- 10 - Annual AAPM&R Conference, November 6 – 10, 2024, San Diego, California. For more information, visit https://aapmr24.eventscribe.net/
22 – 23 - 7th International Physical & Rehabilitation Medicine Conference, November 22 – 23, 2023, Dusit Thani, Abu Dhabi, UAE. For more information, visit maarefah.eventsair.com/iprmc-2024 .
March
19 - 22 - 15th World Congress on Brain Injury, March 19-22, 2025, the Palais de Congres in Montreal, Canada. For information, please visit braininjurycongress.org.
May
19 – 22 – ISPRM 2025 – The 19th World Congress of the ISPRM, May 19 – 22, 2025, Dead Sea, Jordan. For more information, visit www.isprm2025.org.
Alina Nico West, MD, PhD • Nadeem I. Shafi, MD
Alicia M. Diaz-Thomas, MD, MPH
Abstract
Disruptions to the hypothalamic-pituitary axis occur following traumatic brain injury in children and adults, leading to a group of hormonal deficiencies called post-traumatic hypopituitarism (PTHP). PTHP leads to a constellation of symptoms that can overlap with TBI sequelae, making recognition difficult for providers. Variability in hormone testing also complicates diagnosis. In this paper, we describe current measures providers can take to recognize and diagnose pediatric patients who may have PTHP.
Pediatric post-traumatic hypopituitarism (PTHP) affects up to 82% of children1. For both children and adults, PTHP arises during injury recovery, leading to a constellation of symptoms arising from one or more anterior and posterior pituitary hormone deficiencies – central diabetes insipidus (now called arginine vasopressin deficiency), secondary adrenal insufficiency, non-thyroidal illness/secondary hypothyroidism, growth hormone deficiency, central precocious puberty, and/or secondary hypogonadotropic hypogonadism1-3 Although both children and adults are susceptible to PTHP, pediatric clinical presentation poses significant differences in diagnostics when compared with adults. Children also require additional monitoring over extended periods of time due to the potential for abnormalities in growth, development, and puberty. In this review, we discuss pediatric PTHP diagnosis as it relates to sequelae of TBI, the current state of PTHP diagnosis, and a proposal to advance the study of PTHP.
The highly vascularized pituitary gland, which sits in the sella turcica of the brain, is susceptible to structural damage either directly or indirectly via hypothalamic injury3-4.
Injuries may result in hypothalamic hemorrhage, posterior pituitary hemorrhage, and anterior pituitary infarction; shear injury and transection are also possible4
Pituitary volume decreases with increased intracranial pressure and/or necrosis and apoptosis5-7. Such hypoxic injury impacts the hypothalamic and pituitary cellular landscape, with somatotrophs and gonadotrophs affected more than thyrotrophs, corticotrophs, and axonal projections of magnocellular neurosecretory cells7-8 (Table 1). Hypoxic cellular injury and neuroinflammatory cascade activation potentiate hypothalamic-pituitary dysfunction in all severities of TBI9-12
Patient Characteristics
Overall, clinical suspicion for a PTHP disorder guides screening and diagnostics, but several knowledge gaps and confounders exist. There are no evidence-based diagnostic guidelines for children13. PTHP symptoms can often overlap with TBI-related sequelae during the chronic period, potentially delaying recognition for months to years1. Clinical considerations should include prior injuries, endocrine disorders unrelated to PTHP, and other neurocognitive, neuropsychological, and psychiatric disturbances. None are established as predictors of PTHP disorders1. Further complicating diagnosis, PTHP disorders have been reported to resolve spontaneously in adult and pediatric chronic TBI2, 14-17. Aspects of TBI include an overwhelming 3:1 male to female ratio with a bimodal peak at 0-4 years and 15-24 years of age18 concealing characteristics such as sex differences. Mechanisms and severity of injury, features of imaging, and ICU-related management have not been established as predictors of PTHP.
Pubertal status assessment is conducted in a majority of pediatric PTHP studies. Despite these assessments, there are no studies that have matched patients on pubertal status, confounding pediatric PTHP outcomes assessments, including those related to sex steroids and sex differences1.
When screening the hypothalamic-pituitary-gonadotropin axis for central precocious puberty and secondary hypogonadotropic hypogonadism, Tanner staging is performed. Abnormalities in breast development for females and testicular volume for males are used alongside laboratory testing, as discussed in the ‘Central Precocious Puberty’ and ‘Secondary Hypogonadotropic Hypogonadism’ sections later. However, pubertal development abnormalities have not been described as a diagnostic marker of other PTHP disorders.
Hypothalamic-pituitary dysfunction can emerge acutely or remotely after TBI. The acute TBI period is commonly regarded as the two weeks following initial injury, while the subacute and chronic periods are loosely defined as up to 3 months following the injury, and the subsequent years, respectively19-21. Because neuroinflammation and neuroendocrine dysfunction due to secondary injury can occur over an undefined and potentially prolonged period, PTHP in patients may go undiagnosed. Attempts to define the incidence and outcomes of PTHP within pediatric and adult studies have been affected by the unpredictable nature of TBI recovery. Most pediatric PTHP cases have been reported in chronic TBI and occur over yearss1-2. There are very few studies that provide prospective data from PTHP cases starting from the acute injury period, suggesting that there is not enough evidence to discern PTHP tempo during TBI recovery14-15. Moreover, PTHP can be either transient, without clear evidence of the point at which there is return of function, or it can be enduring, further complicating diagnosis and management22.
We previously evaluated retrospective and prospective pediatric PTHP studies to elucidate any sex differences1. We found that the diagnostic approaches are so varied for anterior and posterior PTHP disorders that they could potentially lead to significant variability in incidence/prevalence measurements.
Posterior pituitary dysfunction is most prevalent in acute TBI and decreases considerably in chronic TBI. Because pediatric patients are most likely to have challenges in chronic post-injury care, we will focus our attention in this review on the anterior pituitary dysfunction in chronic TBI. We provide an overview of each anterior pituitary disorder in chronic TBI by discussing: 1) incidence/ prevalence from previous studies to indicate the wide ranges in diagnostic rates; 2) signs and symptoms to highlight those that can overlap with TBI-related sequelae; and 3) variabilities in testing and diagnostic criteria for each disorder. Table 2 is presented as a guide for pediatric PTHP diagnostic strategies; however, consensus does not exist on diagnostic criteria and testing cutoffs. It is worth mentioning that the wide incidence/prevalence ranges likely reflect major differences in study design, different testing strategies, testing at inconsistent time points, and small sample sizes among a mix of retrospective and prospective observational studies.
Post-traumatic GHD is reported with an approximated range of 0-82%1. GHD can lead to short stature or decreased growth velocity following childhood TBI, along with decreased lean muscle mass and metabolism with increased abdominal fat 23 .
Children and adolescents may also experience neurocognitive and neuropsychological abnormalities such as poor memory and depression24-25, overwhelmingly an overlap of PTHP and TBI sequelae, altogether affecting a child’s overall quality of life.
Growth measurements during physical examination, together with radiological and biochemical markers, are used to make the diagnosis of GHD [Table 2]. It must be noted that IGF-1 and IGFBP-3 can be used either independently or as a ratio with moderate clinical sensitivity and specificity26. Both IGF-1 and IGFBP-3 have lower diagnostic accuracies when used alone versus in combination with stimulatory tests used to measure the potential release of GH27-28. Serum GH stimulation via the insulin tolerance test (ITT) is considered the clinical gold-standard29-30. However, the most commonly used test in clinical pediatric practice is arginine and levodopa stimulation29. Arginine can be used alone or in combination with other stimuli such as clonidine, glucagon, or growth hormone releasing hormone (GHRH). Glucagon has also been used alone or in combination with clonidine, betaxolol, or propranolol1. Sex steroid priming to increase GH responsiveness to GHRH is recommended for peripubertal children to improve GH secretion and decrease false positives for GHD23. Testing cutoffs for GH deficiency are incredibly variable, complicating diagnosis and prevalence assessments, and indicating the need for testing standardization1,31
Secondary adrenal insufficiency (SAI) is reported in 0-44% of children with all-severity TBI1, (Table 1). Although we do not discuss it here, SAI is reported in children with acute TBI as well. In SAI, disruption of the hypothalamic-pituitary-adrenal (HPA) axis results in decreased ACTH secretion and subsequent glucocorticoid deficiency. Effects of SAI include hypoglycemia, lethargy, poor feeding, nausea, and vomiting32. Generally, the diagnosis is made using an 8 a.m. cortisol and basal ACTH measurement; ACTH stimulation is preferred to make the definitive diagnosis of SAI but is not reliable in acute TBI. The ITT was originally considered the gold-standard; however, due to hypoglycemic seizure and cardiac event risk, low or high dose ACTH stimulation is recommended, with low dose (1μg) ACTH having a higher specificity. ACTH stimulation has variable testing cutoffs as well32-35
Secondary hypothyroidism occurs in chronic TBI with a prevalence of 0-64%, reflective of inconsistencies within pediatric PTHP study designs and testing methodologies1. (Nonthyroidal illness occurs during acute TBI and will not be discussed here.) Thyroid-stimulating hormone (TSH) and basal T4 and/or T3 comprise the thyroid panel; free T4 measurements are acceptable for diagnosis36. Expected laboratory results in secondary hypothyroidism are a low-normal free T4, with signs and symptoms including lethargy, temperature sensitivity, weight gain, dry skin, hair loss, muscle fatigue and aches, and depression. If TSH levels are tested, it is expected they are low to low-normal37.
Isosexual central precocious puberty is due to prematurely increased GnRH secretion and has an approximate prevalence of 0-8% in children with TBI1.
Table 1. Anterior Pituitary Cell Composi6on
Anterior Pituitary Cell Type
Pituitary Lobe Loca6on Pituitary Lobe Cellular Composi6on (%) Hormone
Somatotrophs Lateral anterior pituitary 35-45
Cor>cotrophs Central median anterior pituitary wedge 20
Thyrotrophs Anteromedial anterior pituitary 5
Gonadotrophs Heterogeneously-located 10-15
Table 2. General Pediatric PTHP Diagnos6c Approaches
PTHP Deficiency
GHD1
Diagnos6c Approach
Growth and Development Measurements (Physical Exam)
Growth Hormone (GH)
Adrenocor>cotropic Hormone (ACTH)
Thyrotropin S>mula>ng Hormone (TSH)
Luteinizing Hormone (LH) and Follicle S>mula>ng Hormone (FSH)
Outcome Measurement
Height
Height velocity (HV)
(Central) SAI3
Secondary Hypothyroidism4
Secondary Hypogonadotropic Hypogonadism and Central Precocious Puberty5,6
Radiological Assessments (including neuroimaging)
Auxiliary Serum Growth Markers
Bone age
Brain MRI for pituitary abnormali>es
IGF-1
IGFBP-3
GH S>mula>on Confirmatory Tes>ng ITT2
Arginine Clonidine Glucagon L-DOPA
Clinical signs and symptoms
Medical History and Physical Exam
Circula>ng serum hormones and ACTH s>mula>on tes>ng Cor>sol with basal ACTH
Clinical signs and symptoms
Medical History and Physical Exam Circula>ng serum hormones
Physical Exam
Free T4 (random)
Tanner Staging
Females: Tanner Stage 2 Breast Development
Males: Tes>cular volume ≥4mL
Basal circula>ng serum hormones and GnRH s>mula>on tes>ng LH FSH T E2
1Hage et al. 2021 2Gold Standard 3Bowden, 2023 4Rodriguez et al. 2022 5Chen, et al. 2015 6Klein et al. 2017
Most pediatric patients develop clinical signs months to years following their injury1. Pubertal changes are initially assessed via Tanner staging for both males and females38-39 (Table 2). Although basal gonadotropins have fluctuations in measurement, GnRH stimulation is a sensitive and reliable confirmatory test and considered the diagnostic gold standard for central precocious puberty.
Delayed puberty, the effect of secondary or post-traumatic hypogonadotropic hypogonadism, has been described in 0-13% of children who suffer TBI, but this is based on a few mixed retrospective-prospective reports which are small in sample size1. General diagnostic strategies are presented in Table 2. Longitudinal cohort studies after pediatric TBI are required to obtain a better estimate of the incidence of secondary hypogonadotropic hypogonadism.
Research studies have used a variety of diagnostic approaches over time that have contributed to an overall underdiagnosis of PTHP in children. Major challenges in the field include: (1) understudied PTHP predictors; (2) a lack of guidelines for appropriate testing time windows; (3) the male to female incidence ratio associated with TBI; (4) variability in PTHP testing strategies; (5) study issues such as loss of participant follow-up, lack of control measurements, lack of case matching by age, sex, pubertal status and injury related factors such as severity, and small sample sizes1. A better understanding of the time course of PTHP – as well as improvements in study designs – would help to capture the chronic symptoms that overlap with other TBI sequelae such as sleep disorders, behavioral issues, psychological disturbances, and psychiatric disorders. Advances in the aforementioned areas would provide needed standardization in the diagnosis and management of PTHP, the development of patient screening mechanisms including self-reporting tools, and the development of prospective research studies.
The existing pediatric PTHP literature does not reflect consensus in diagnostic approaches or treatment strategies. As a result, we lack insights into clinically relevant sex differences; the overlap of PTHP symptoms and TBI sequelae including neurocognition, psychiatric/ neuropsychological and sleep disturbances; and, potential predictors and outcomes. Furthermore, the relevance of social determinants of health and adverse childhood experiences remain unclear. Lastly, we cannot effectively design pre-clinical (animal) PTHP models based on current diagnostic data.
Our group has described that the path forward in studying PTHP must begin by establishing consensus on diagnostic strategies, and we have initiated this work as a modified Delphi to survey endocrinologists’ consensus of PTHP definitions and testing strategies. We expect diagnostic consensus will provide a basis upon which future pediatric PTHP research studies can be designed. Until these objectives have been successfully addressed for children, we recommend that clinicians keep very high indices of suspicion for PTHP disorders following pediatric TBI, and that patients be referred to endocrinology for further workup of suspected PTHP.
1. West, A. N., Diaz-Thomas, A. M., & Shafi, N. I. (2020). Evidence Limitations in Determining Sexually Dimorphic Outcomes in Pediatric Post-Traumatic Hypopituitarism and the Path Forward. Frontiers in Neurology, 11, 551923. https://doi.org/10.3389/fneur.2020.551923
2. Casano-Sancho, P. (2017). Pituitary dysfunction after traumatic brain injury: are there definitive data in children? Archives of Disease in Childhood, 102(6), 572–577. https://doi.org/10.1136/ archdischild-2016-311609
3. Reifschneider, K., Auble, B., & Rose, S. (2015). Update of Endocrine Dysfunction following Pediatric Traumatic Brain Injury. Journal of Clinical Medicine, 4(8), 1536–1560. https://doi.org/10.3390/jcm4081536
4. Benvenga, S., Campenní, a, Ruggeri, R. M., & Trimarchi, F. (2000). Clinical review 113: Hypopituitarism secondary to head trauma. The Journal of Clinical Endocrinology and Metabolism, 85(4), 1353–1361. https:// doi.org/10.1210/jcem.85.4.6506
5. De Marinis, L., Bonadonna, S., Bianchi, A., Maira, G., & Giustina, A. (2005). Primary empty sella. Journal of Clinical Endocrinology and Metabolism, 90(9), 5471–5477. https://doi.org/10.1210/jc.2005-0288
6. Dusick, J. R., Wang, C., Cohan, P., Swerdloff, R., & Kelly, D. F. (2012). Pathophysiology of hypopituitarism in the setting of brain injury. Pituitary, 15(1), 2–9. https://doi.org/10.1007/s11102-008-0130-6
7. Sav, A., Rotondo, F., Syro, L. V., Serna, C. A., & Kovacs, K. (2019). Pituitary pathology in traumatic brain injury: a review. Pituitary, 22(3), 201–211. https://doi.org/10.1007/s11102-019-00958-8
8. Makulski, D. D., Taber, K. H., & Chiou-Tan, F. Y. (2008). Neuroimaging in posttraumatic hypopituitarism. Journal of Computer Assisted Tomography, 32(2), 324–328. https://doi.org/10.1097/RCT.0b013e3181636ed4
9. Kelestimur, F. (2021). Antibodies against the pituitary and hypothalamus in boxers. Handbook of Clinical Neurology, 181, 187–191. https://doi.org/10.1016/B978-0-12-820683-6.00014-2
10. Kumar, R. G., Rubin, J. E., Berger, R. P., Kochanek, P. M., & Wagner, A. K. (2016). Principal components derived from CSF inflammatory profiles predict outcome in survivors after severe traumatic brain injury. Brain, Behavior, and Immunity, 53, 183–193. https://doi.org/10.1016/j.bbi.2015.12.008
11. Santarsieri, M., Kumar, R. G., Kochanek, P. M., Berga, S., & Wagner, A. K. (2015). Variable neuroendocrineimmune dysfunction in individuals with unfavorable outcome after severe traumatic brain injury. Brain, Behavior, and Immunity, 45, 15–27. https://doi.org/10.1016/j.bbi.2014.09.003
12. Tanriverdi, F., Unluhizarci, K., & Kelestrimur, F. (2010). Persistent neuroinflammation may be involved in the pathogenesis of traumatic brain injury (TBI)-induced hypopituitarism: Potential genetic and autoimmune factors. Journal of Neurotrauma, 27(2), 301–302. https://doi.org/10.1089/neu.2009.1102
13.Ben Abdeljelil, A., Freire, G. C., Yanchar, N., Turgeon, A. F., Beno, S., Bérubé, M., Stang, A., Stelfox, T., Zemek,R., Beaulieu, E., Gagnon, I. J., Gabbe, B., Lauzier, F., Labrosse, M., Tardif, P. A., Deshommes, T., G nanvi, J., & Moore, L. (2023). Pediatric Moderate and Severe Traumatic Brain Injury: A Systematic Review of Clinical Practice Guideline Recommendations. Journal of Neurotrauma. https://doi.org/10.1089/ neu.2023.0149
14. Einaudi, S., Matarazzo, P., Peretta, P., Grossetti, R., Giordano, F., Altare, F., Bondone, C., Andreo, M., Ivani, G., Genitori, L., De Sanctis ’, C., & Einaudi, S. (2006). Hypothalamo-hypophysial Dysfunction After Traumatic Brain Injury in Children and Adolescents: A Preliminary Retrospective and Prospective Study. Journal of Pediatric Endocrinology & Metabolism, 19, 691–703. https://extranet.uj.edu.pl/downloadpdf/j/ jpem.2006.19.5/jpem.
15. Kaulfers, A. M., Backeljauw, P. F., Reifschneider, K., Blum, S., Michaud, L., Weiss, M., & Rose, S. R. (2010). Endocrine dysfunction following traumatic brain injury in children. J Pediatr, 157(6), 894–899. https://doi. org/10.1016/j.jpeds.2010.07.004
16. Personnier, C., Crosnier, H., Meyer, P., Chevignard, M., Flechtner, I., Boddaert, N., Breton, S., Mignot, C., Dassa, Y., Souberbielle, J.-C., Piketty, M., Laborde, K., Jais, J.-P., Viaud, M., Puget, S., Sainte-Rose, C., & Polak, M. (2014). Prevalence of Pituitary Dysfunction After Severe Traumatic Brain Injury in Children and Adolescents: A Large Prospective Study. The Journal of Clinical Endocrinology & Metabolism, 99(6), 2052–2060. https://doi.org/10.1210/jc.2013-4129
17. Srinivas, R., Brown, S. D., Chang, Y. F., Garcia-Fillion, P., & Adelson, P. D. (2010). Endocrine function in children acutely following severe traumatic brain injury. Child’s Nervous System, 26(5), 647–653. https://doi. org/10.1007/s00381-009-1038-9
18. Centers for Disease Control and Prevention (2022). Surveillance Report of Traumatic Brain Injury-related Deaths by Age Group, Sex, and Mechanism of Injury—United States, 2018 and 2019. Centers for Disease Control and Prevention, U.S. Department of Health and Human Services.
19. Agha, A., Rogers, B., Mylotte, D., Taleb, F., Tormey, W., Phillips, J., & Thompson, C. J. (2004). Neuroendocrine dysfunction in the acute phase of traumatic brain injury. Clinical Endocrinology, 60(5), 584–591. https://doi.org/10.1111/j.1365-2265.2004.02023.x
20. Godbolt, A. K., Stenberg, M., Jakobsson, J., Sorjonen, K., Krakau, K., Stålnacke, B.-M., & DeBoussard, C.N. (2015). Subacute complications during recovery from severe traumatic brain injury: frequency and associations with outcome. BMJ Open, 5, e007208. https://doi.org/10.1136/bmjopen-2014- 007208
21. Tanriverdi, F., & Kelestimur, F. (2015). Pituitary dysfunction following traumatic brain injury: Clinical perspectives. Neuropsychiatric Disease and Treatment, 11, 1835–1843. https://doi.org/10.2147/NDT.S65814
22. Fernandez-Rodriguez, E., Bernabeu, I., Castro, A. I., Kelestimur, F., & Casanueva, F. F. (2010). Hypopituitarism following traumatic brain injury: Determining factors for diagnosis. In Frontiers in Endocrinology (Vol. 2, Issue AUG). https://doi.org/10.3389/fendo.2011.00025
23. Hage, C., Gan, H. W., Ibba, A., Patti, G., Dattani, M., Loche, S., Maghnie, M., & Salvatori, R. (2021). Advances in differential diagnosis and management of growth hormone deficiency in children. In Nature Reviews Endocrinology (Vol. 17, Issue 10, pp. 608–624). Nature Research. https://doi.org/10.1038/s41574021-00539-5
24. Szarka, N., Szellar, D., Kiss, S., Farkas, N., Szakacs, Z., Czigler, A., Ungvari, Z., Hegyi, P., Buki, A., & Toth, P. (2021). Effect of Growth Hormone on Neuropsychological Outcomes and Quality of Life of Patients with Traumatic Brain Injury: A Systematic Review. In Journal of Neurotrauma (Vol. 38, Issue 11, pp. 1467–1483).
25. Wamstad, J. B., Norwood, K. W., Rogol, A. D., Gurka, M. J., Deboer, M. D., Blackman, J. A., Buck, M. L., Kuperminc, M. N., Darring, J. G., & Patrick, P. D. (2013). Neuropsychological recovery and quality-of-life in children and adolescents with growth hormone deficiency following TBI: A preliminary study. Brain Injury, 27(2), 200–208. https://doi.org/10.3109/02699052.2012.672786
26. Shen, Y., Zhang, J., Zhao, Y., Yan, Y., Liu, Y., & Cai, J. (2015). Diagnostic value of serum IGF-1 and IGFBP-3 in growth hormone deficiency: a systematic review with meta-analysis. In European Journal of Pediatrics (Vol. 174, Issue 4, pp. 419–427). Springer Verlag. https://doi.org/10.1007/s00431-014-2406-3
27. Ho, K. K. Y., & Hoffman, D. M. (1995). Defining Growth Hormone Deficiency in Adults. Metabolism, 44(Suppl 4), 91–96. https://doi.org/10.1016/0026-0495(95)90227-9
28. Kim, H. J., Kwon, S. H., Kim, S. W., Park, D. J., Shin, C. S., Park, K. S., Kim, S. Y., Cho, B. Y., & Lee, H. K. (2001). Diagnostic value of serum IGF-I and IGFBP-3 in growth hormone disorders in adults. Hormone Research, 56(3–4), 117–123. https://doi.org/10.1159/000048103
29. Yau, M., & Rapaport, R. (2022). Growth Hormone Stimulation Testing: To Test or Not to Test? That Is One of the Questions. In Frontiers in Endocrinology (Vol. 13). Frontiers Media S.A. https://doi.org/10.3389/ fendo.2022.902364
30.Yuen, K. C. J., Biller, B. M. K., Radovick, S., Carmichael, J. D., Jasim, S., Pantalone, K. M., & Hoffman, A. R. (2019). American Association of Clinical endocrinologists and American College of Endocrinology guidelines for management of growth hormone deficiency in adults and patients transitioning from pediatric to adult care. Endocrine Practice, 25(11), 1191–1232. https://doi.org/10.4158/GL-2019-0405
31. Stanley, T. (2012). Diagnosis of Growth Hormone Deficiency in Childhood. Curr Opin Endocrinol Diabetes Obes., 19(1), 47–52. https://doi.org/10.1097/MEDMary Ann Liebert Inc. https://doi.org/10.1089/ neu.2020.7265
32. Bowden, S. A. (2023). Current Screening Strategies for the Diagnosis of Adrenal Insufficiency in Children. Pediatric Health, Medicine and Therapeutics, Volume 14, 117–130. https://doi.org/10.2147/phmt.s334576
33. Bancos, I., Hahner, S., Tomlinson, J., & Arlt, W. (2015). Diagnosis and management of adrenal insufficiency. Lancet Diabetes Endocrinol, 3(3), 216–226. ttps://doi.org/http://dx.doi.org/10.1016/ S22138587(14)70142-1
34. Kazlauskaite, R., Evans, A. T., Villabona, C. V., Abdu, T. A. M., Ambrosi, B., Atkinson, A. B., Cheung, H. C., Clayton, R. N., Courtney, C. H., Gonc, E. N., Maghnie, M., Rose, S. R., Soule, S. G., & Tordjman, K. (2008). Corticotropin tests for hypothalamic-pituitary-adrenal insufficiency: A metaanalysis. Journal of Clinical Endocrinology and Metabolism, 93(11), 4245–4253. https://doi.org/10.1210/jc.2008-0710
35. Rose, S. R., Lustig, R. H., Burstein, S., Pitukcheewanont, P., Broome, D. C., & Burghen, G. A. (1999). Diagnosis of ACTH deficiency. Comparison of overnight metyrapone test to either low-dose or high-dose ACTH test. Hormone Research, 52(2), 73–79. https://doi.org/10.1159/000023438
36. Rodriguez, L., Dinauer, C., & Francis, G. (2022). Treatment of hypothyroidism in infants, children and adolescents. In Trends in Endocrinology and Metabolism (Vol. 33, Issue 7, pp. 522–532). Elsevier Inc. https:// doi.org/10.1016/j.tem.2022.04.007
37. Lania, A., Persani, L., & Beck-Peccoz, P. (2008). Central hypothyroidism. Pituitary, 11(2), 181–186. https:// doi.org/10.1007/s11102-008-0122-6
38. Chen, M., & Eugster, E. A. (2015). Central Precocious Puberty: Update on Diagnosis and Treatment. Pediatric Drugs, 17(4), 273–281. https://doi.org/10.1007/s40272-015-0130-8 2006.19.5.691/,DanaInfo=www.degruyter.com,SSL+jpem.2006.19.5.691.pdf
39. Klein, D. A., Emerick, J. E., Sylvester, J. E., & Vogt, K. S. (2017). Disorders of Puberty: An Approach to Diagnosis and Management. Am Fam Physician, 96(9), 590–599. www.aafp.org/afp.
Alina Nico West, MD, PhD, is an Assistant Professor of Pediatrics and Clinical Researcher at the University of Tennessee Health Science Center (UTHSC) in the Division of Pediatric Critical Care Medicine in Memphis, Tennessee, USA. She is the Director of Pediatric Critical Care Fellowship Research at UTHSC. She is also co-chair of the Endocrine Society Research Affairs Core Committee. Dr. West’s research interests include pediatric neuroendocrine dysfunction following traumatic brain injury (TBI), social determinants of health in pediatric TBI, and adverse childhood experiences in pediatric critical care.
Nadeem I. Shafi, MD, is Associate Professor of Pediatric Critical Care Medicine at the University of Tennessee Health Science Center (UTHSC) and Medical Director of the Pediatric Intensive Care Unit at Le Bonheur Children's Hospital in Memphis, Tennessee, USA. His growing clinical and research interests include the use of artificial intelligence in pediatric intensive care,outcomes of pediatric neurocritical care patients, and the global burden of pediatric critical illness.
Alicia Diaz-Thomas, MD, MPH, is Professor of Pediatric Endocrinology and Associate Dean of Faculty Affairs at the University of Tennessee Health Science Center (UTHSC) and a board-certified pediatric endocrinologist at Le Bonheur Children's Hospital in Memphis, Tennessee, USA. She is also the chair of the Endocrine Society Committee on Diversity and Inclusion. Her research interests include health disparities in pediatric endocrinology and outcomes of pediatric endocrinology patients.
Comprehensive Interdisciplinary traumatic brain injury evaluation and treatment. Our experts include:
Nathan Zasler, MD, Medical Director Brain Injury Medicine
Jacqueline Theis, OD, FAAO Neuro-Optometry
Kendra Sheard, OTD, OTR/L Neuro-occupational Therapy
Sara Etheredge, PT, DPT, CCI Orthopedic and Neuro Physical Therapy
Medical and rehabilitative care for problems following concussion/brain injury, head trauma and whiplash
Post-trauma pain assessment and management
Medicolegal evaluations and peer reviews
Chantel T. Debert, MD, MSc, FRCPC
Worldwide, 69 million individuals are estimated to suffer a traumatic brain injury (TBI) annually.1 Following TBI, debilitating symptoms can occur, lasting months to years, and can be permanent. Neuroendocrine deficits are a prominent contributor to disability after TBI, occurring in approximately 28-32% of patients acutely and chronically.2; 3 Previous recommendation suggested only patients presenting with intracranial bleeds and/or meeting the criteria for moderate or severe TBI should be screened for hypothalamic pituitary axis (HPA) deficits, however 3; 4; 5 we now know neuroendocrine insufficiencies can arise with any severity of TBI, including mild TBI and sport-related concussions.6; 7; 8; 9; 10; 11 If left untreated, neuroendocrine deficits can increase morbidity and mortality, impede participation in rehabilitation and have long-term consequences on recovery. Therefore, it is essential neurorehabilitation specialists have a good understanding of HPA deficits following TBI, and to appropriately screen and treat neuroendocrine insufficiencies that can occur during a vulnerable and critical time of TBI recovery.
Most acute neuroendocrine dysfunctions following TBI are transient and will resolve within 6 months following injury.12 However, patients presenting with signs of electrolyte abnormalities or adrenal insufficiency should be screened. The most common acute neuroendocrine dysfunction includes injury to the posterior pituitary causing alterations in antidiuretic hormone (ADH), also called arginine vasopressin (AVP). Early in recovery, reported within 7-20 days following TBI, arginine vasopressin (AVP) deficiency (formerly known as diabetes insipidus) occurs in 22-26% of patients and reflects an underproduction of antidiuretic hormone (ADH) from the posterior pituitary, leading to signs of polyuria, nocturia and polydipsia.12 Diagnostic testing for AVP deficiency includes serum sodium > 145 mEq/l, urine osmolality>plasma osmolality, urinespecific gravity<1.005 and polyuria>3-4 l/day. AVP deficiency is most often transient but can persist in approximately 6.9% beyond 6 months.12 The syndrome of inappropriate
antidiuretic hormone secretion (SIADH) can also occur acutely, within the first three weeks of injury, in approximately 12% of patients with TBI and is the most common reason for hyponatremia during this phase.12
Importantly, during the acute phase after TBI, adrenal insufficiency (AI) can be life threatening and needs to be identified and treated when present. AI occurs when adrenocorticotropic hormone (ACTH) is unable to be released from the pituitary. Patients with all severities of TBI presenting with some or all of the following symptoms should be screened for AI: weakness, nausea, weight loss, loss of appetite, muscle and joint pain, dizziness, orthostatic hypotension, hypoglycemia, hyponatremia, eosinophilia and anemia. Diagnostic tests consistent with AI include morning cortisol <3.5 μg/dl; if the value is between 3-15 μg/dl a stimulation test is required. It is important to note, transient AI due to acute phase illness response should be considered, particularly in patients with moderate-severe TBI admitted to hospital, as this may not require treatment. In one study, repeat morning cortisol testing within 24 hours and then daily for the first 9 days following severe TBI revealed that 53% of patients had transient AI; they were deemed not to require treatment.13 Acute phase illness responses may lead to diagnostic uncertainty of AI in the acute period following TBI, with provocative testing being inaccurate within the first 6 weeks following injury. Importantly, acute AI does not predict chronic HPA dysfunction.13; 14; 15
Neurorehabilitation guidelines for TBI suggest an initial time of rest (24-72 hours) and then proceeding with focused rehabilitation as tolerated.16; 17; 18 Identification of neuroendocrine deficiencies is important, as symptoms of HPA deficits such as weakness, muscle and joint pain, dizziness, orthostatic hypotension and nausea can delay the onset of rehabilitation and limit critical therapies that focus on return to activities of daily living (dressing, feeding, eating, toileting and hygiene), work, school or sport. Important treatments such as vestibular therapy, physiotherapy and occupation therapy that target improvement of symptoms and return to function may be limited or non-existent because the patient is unable to tolerate the rehabilitation.
Legend: TBI traumatic brain injury, AI adrenal insufficiency, ACTH adrenocorticotropic hormone, DI diabetes insipidus (now known as arginine vasopressin deficiency), SIADH syndrome of inappropriate antidiuretic hormone, TSH thyroid stimulating hormone, LH luteinizing hormone, FSH follicle stimulating hormone, HPA hypothalamic pituatary axis.
The rehabilitation specialist can play an integral role in identifying neuroendocrine dysfunction in the acute and subacute phases of recovery. For example, patients unable to participate in therapies due to frequent voiding, exercise intolerance, dizziness or orthostatic hypotension should be evaluated for hormone deficiencies involving ADH. Similarly, patients with TBI presenting with additional symptoms of muscle pain, global weakness weight loss, loss of appetite and nausea evaluation of AI should be considered. For the rehabilitation specialist, awareness of common acute/subacute neuroendocrine deficits is essential; insufficiencies left untreated can delay initiation of rehabilitation and have significant impact on the patient’s recovery.
Patients with all severities of TBI can present with persistent or permanent symptoms that consist of headache, dizziness, fatigue, sleep disruptions, cognitive dysfunction, vision changes, sensory deficits, mood/behavioural changes, language impairments and motor dysfunction. These symptoms can be non-specific especially in mild TBI and can have significant overlap with symptoms of HPA deficits making diagnosis of neuroendocrine insufficiencies difficult. Therefore, screening HPA hormones in patients with chronic symptoms, even if non-specific during recovery, is recommended. If left untreated, persistence of neuroendocrine deficits can occur well into the chronic phase of TBI, hindering recovery and causing deleterious health consequences. Of neuroendocrine deficiencies, growth hormone deficiency (GHD) after TBI is the most common chronic hormone deficit with variable prevalence cited in the literature. 13; 19; 20; 21 The large variation in prevalence most likely reflects timing and method of testing, age, and injury severity.
Patients with GHD may present with significant fatigue,22; 23 poor sleep,22 cognitive dysfunction,22 decreased exercise tolerance,24 reduced muscle mass and strength,24; 25 dyslipidemia,26 anxiety,27 depression,23; 27 and osteoporosis.28 Timing of screening and treating GHD can be controversial. Some endocrinology centres will screen >3 months but treat between 6-12 months. Whereas many will wait until one year post-injury to screen and treat, as studies have shown recovery of GHD up to one year following TBI.29 Though previous guidelines recommended assessment with serum IGF-1,31 studies have found IGF-1 lacks specificity and sensitivity in patients with TBI and GHD.32 Specifically, Lithgow et al. evaluated 60 participants with persistent symptoms one year post-TBI and found there was no correlation between IGF-1 and dynamic testing results, suggesting that IGF-1 had no utility in diagnosing GHD in patients with TBI. Therefore, when GHD is suspected, we recommend referring to endocrinology for dynamic testing, such as glucagon stimulation testing or insulin tolerance testing, for a definitive diagnosis.
Deficits in other HPA hormones also occur. Damage of the anterior pituitary following TBI can alter levels of circulating sex steroids leading to gonadotropin deficiencies (GD). Acutely, 40-80% of the patients with TBI may display low gonadotropin levels,20; 32 but the prevalence declines to 2-32% in the chronic phase post-injury.33 Patients may present with loss of hair, sexual dysfunction, fatigue, decline in muscle mass, infertility, galactorrhea, cognitive changes, disrupted menses and breast atrophy. Diagnostic testing of sex hormones acutely following TBI is not recommended, as most patients recover. Previously published guidelines agree that patients should be tested for gonadotrophic dysfunction, including estradiol, progesterone, luteinizing hormone and follicular stimulating hormone at 3-6 months and 12 months post-injury. 3; 34; 35
Hypothyroidism can also occur following TBI, though it is reported to be less frequent than other HPA hormone deficiency. At 1-year post-injury the reported prevalence of hypothyroidism is 4.1-6.2% with patients presenting with fatigue, cold intolerance, weight gain, altered menses, hyperlipidemia, hyponatremia, low mood and constipation.2; 4 (See FIGURE 1 for chronic phase screening details.)
The neurorehabilitation specialist plays an important role in identifying and treating neuroendocrine deficiencies in the chronic phase following TBI. As the most common medical provider during this phase of recovery, rehabilitation specialists require a clear understanding of signs and symptoms of chronic HPA deficiencies, guidelines for evaluation, and when to refer and principles of treatment. Brain injury rehabilitation often requires a multidisciplinary team collaboratively working together to improve symptoms and function. Identification of HPA deficits may be easiest to determine when patients are engaged with a multidisciplinary neurorehabilitation team, as there is increased interaction with a variety of care providers, and subtle signs and symptoms are more evident. Neuroendocrine deficiency during the chronic phase of TBI can delay rehabilitation, slow recovery and hinder patients’ ability to reach optimum function. Symptoms following TBI can be non-specific (fatigue, sleep disruption, poor attention and memory, decrease mood and increased anxiety) and overlap with those presenting with neuroendocrine deficits. Implementing regular evaluation of screening questions and diagnostic testing during this phase of recovery is important ( FIGURE 1).
Neuroendocrine deficits can occur following all severities of TBI and the absence of intracranial pathology on structural neuroimaging (computer tomography or magnetic resonance brain imaging) does not eliminate the possibility of HPA deficits. Neuroendocrine deficiency after TBI is more common than once thought and can occur in all types of TBI, mild TBI and sport-related concussion included. In the acute phase, screening for AVP deficiency, SIADH and adrenal insufficiency in individuals with signs and symptoms suggestive of these deficiencies is recommended. However, particularly in moderate/severe TBI, a stress response and commonly used medications such as propofol may contribute to transient changes in HPA function and need to be considered. In patients with TBI admitted to the hospital we recommend morning cortisol assessments for day 1-5 and then at again at 2-weeks postinjury; if AI is diagnosed, repeat provocative testing at 2-3 months is recommended. It is not recommended to test for gonadotropin or growth hormone deficiency in the acute phase as there is limited evidence that treatment is beneficial.
When and whom to screen is controversial in the chronic phase. For example, Tanriverdi et al. recommended screening at 6 months and 12-months in patients with complicated mild TBI (evidence of intracranial bleed on neuroimaging) and moderate/severe TBI.3 However, Glynn et al recommend similar hormone screening at 3-6 months and 12 months but in moderate/severe TBI only.36 More recently, Mahajan et al. recommended screening at 3-6 months, 12 months, in patients with TBI admitted to hospital for >48 hours or symptomatic patients not admitted or those admitted for < 48 hours.37 We recommend screening for adrenal, gonadal and thyroid deficits at 3-6 months and 12 months post-injury after all severities of TBI, including mild TBI and sport-related concussion with clinical suspicion of hormone deficiency. If any hormone deficits are identified, referral to endocrinology is recommended. At 1-year post-injury, in all severities of TBI, if patients present with signs and symptoms of growth hormone deficiency, we recommend a
referral to endocrinology for dynamic testing. Opinions differ, but one group suggests if hormone deficits are identified at 12-months post-injury, annual repeat assessment for up to 5 years should be completed (Figure 1).36 All patients with TBI presenting with signs and symptoms of HPA deficits should be screened at the appropriate timepoints after injury to improve symptom burden and enhance recovery. If deficiencies are identified, it is important hormone replacement is not delayed as optimization of a patient’s wellbeing will enhance rehabilitation participation and subsequently improve symptoms and function. Neurorehabilitation teams members such as physiotherapists, occupational therapists, social workers, recreational therapists, nurses, and physical medicine and rehabilitation physicians provide care for patients with TBI at all stages of recovery. Identification and treatment of neuroendocrine deficits during TBI rehabilitation can provide the neurorehabilitation specialist with another important tool to optimize patient’s health.
1. DEWAN, M. C. et al. Estimating the global incidence of traumatic brain injury. J Neurosurg, v. 130, n. 4, p. 1080-1097, Apr 27 2018. ISSN 1933-0693. Disponível em: < https://www.ncbi.nlm.nih.gov/ pubmed/29701556 >.
2. EMELIFEONWU, J. A. et al. Prevalence of Anterior Pituitary Dysfunction Twelve Months or More following Traumatic Brain Injury in Adults: A Systematic Review and Meta-Analysis. J Neurotrauma, v. 37, n. 2, p. 217226, Jan 15 2020. ISSN 1557-9042. Disponível em: < https://www.ncbi.nlm.nih.gov/pubmed/31111791 >.
3. TANRIVERDI, F. et al. Pituitary dysfunction after traumatic brain injury: a clinical and pathophysiological approach. Endocr Rev, v. 36, n. 3, p. 305-42, Jun 2015. ISSN 1945-7189. Disponível em: < https://www.ncbi. nlm.nih.gov/pubmed/25950715 >.
4. LAUZIER, F. et al. Clinical outcomes, predictors, and prevalence of anterior pituitary disorders following traumatic brain injury: a systematic review. Crit Care Med, v. 42, n. 3, p. 712-21, Mar 2014. ISSN 1530-0293. Disponível em: < https://www.ncbi.nlm.nih.gov/pubmed/24247474 >.
5. SCHNEIDER, H. J. et al. Hypothalamopituitary dysfunction following traumatic brain injury and aneurysmal subarachnoid hemorrhage: a systematic review. JAMA, v. 298, n. 12, p. 1429-38, Sep 26 2007. ISSN 15383598. Disponível em: < https://www.ncbi.nlm.nih.gov/pubmed/17895459 >.
6. KELESTIMUR, F. et al. Boxing as a sport activity associated with isolated GH deficiency. J Endocrinol Invest, v. 27, n. 11, p. RC28-32, Dec 2004. ISSN 0391-4097. Disponível em: < https://www.ncbi.nlm.nih.gov/ pubmed/15754728 >.
7. TANRIVERDI, F. et al. Hypopituitarism due to sports related head trauma and the effects of growth hormone replacement in retired amateur boxers. Pituitary, v. 13, n. 2, p. 111-4, Jun 2010. ISSN 1573-7403. Disponível em: < https://www.ncbi.nlm.nih.gov/pubmed/19847653 >.
8. TANRIVERDI F, DE BELLIS A, BATTAGLIA M, BELLASTELLA G, BIZZARRO A, SINISI AA, BELLASTELLA A, UNLUHIZARCI K, SELCUKLU A, CASANUEVA FF, KELESTIMUR F. Investigation of antihypothalamus and antipituitary antibodies in amateur boxers: is chronic repetitive head trauma-induced pituitary dysfunction associated with autoimmunity? Eur J Endocrinol, v. 162, n. 5, p. 861-7, May 2010. ISSN 1479-683X. Disponível em: < https://www.ncbi.nlm.nih.gov/pubmed/20176736 >.
9. LANGELIER, D. M.; KLINE, G. A.; DEBERT, C. T. Neuroendocrine Dysfunction in a Young Athlete With Concussion: A Case Report. Clin J Sport Med, v. 27, n. 6, p. e78-e79, Nov 2017. ISSN 1536-3724. Disponível em: < https://www.ncbi.nlm.nih.gov/pubmed/28114247 >.
10. LITHGOW, K. et al. IGF-1 Level for Diagnosis of Growth Hormone Deficiency Following Traumatic Brain Injury. Canadian Journal of Diabetes, v. 41, n. 5, p. S35-S36, 2017. ISSN 1499-2671.
11. MERCIER, L. J. et al. Growth hormone deficiency testing and treatment following mild traumatic brain injury. Sci Rep, v. 11, n. 1, p. 8534, Apr 20 2021. ISSN 2045-2322. Disponível em: < https://www.ncbi.nlm.nih. gov/pubmed/33879807 >.
12. AGHA, A. et al. Neuroendocrine dysfunction in the acute phase of traumatic brain injury. Clin Endocrinol (Oxf), v. 60, n. 5, p. 584-91, May 2004. ISSN 0300-0664. Disponível em: < https://www.ncbi.nlm.nih.gov/ pubmed/15104561 >.
13. COHAN, P. et al. Acute secondary adrenal insufficiency after traumatic brain injury: a prospective study. Crit Care Med, v. 33, n. 10, p. 2358-66, Oct 2005. ISSN 0090-3493. Disponível em: < https://www.ncbi.nlm. nih.gov/pubmed/16215393 >.
14. KLOSE, M. et al. Acute and long-term pituitary insufficiency in traumatic brain injury: a prospective single-centre study. Clin Endocrinol (Oxf), v. 67, n. 4, p. 598-606, Oct 2007. ISSN 0300-0664. Disponível em: < https://www.ncbi.nlm.nih.gov/pubmed/17880406 >.
15. BENSALAH, M. et al. Cortisol evaluation during the acute phase of traumatic brain injury-A prospective study. Clin Endocrinol (Oxf), v. 88, n. 5, p. 627-636, May 2018. ISSN 1365-2265. Disponível em: < https:// www.ncbi.nlm.nih.gov/pubmed/29405355 >.
16. SCHNEIDER, K. J. et al. Targeted interventions and their effect on recovery in children, adolescents and adults who have sustained a sport-related concussion: a systematic review. Br J Sports Med, v. 57, n. 12, p. 771-779, Jun 2023. ISSN 1473-0480. Disponível em: < https://www.ncbi.nlm.nih.gov/pubmed/37316188 >.
17. LEDDY, J. J. et al. Rest and exercise early after sport-related concussion: a systematic review and metaanalysis. Br J Sports Med, v. 57, n. 12, p. 762-770, Jun 2023. ISSN 1473-0480. Disponível em: < https://www. ncbi.nlm.nih.gov/pubmed/37316185 >.
18. LENDRAITIENĖ, E. et al. The impact of physical therapy in patients with severe traumatic brain injury during acute and post-acute rehabilitation according to coma duration. J Phys Ther Sci, v. 28, n. 7, p. 2048-54, Jul 2016. ISSN 0915-5287. Disponível em: < https://www.ncbi.nlm.nih.gov/pubmed/27512262 >.
19. TANRIVERDI, F. et al. A five year prospective investigation of anterior pituitary function after traumatic brain injury: is hypopituitarism long-term after head trauma associated with autoimmunity? J Neurotrauma, v. 30, n. 16, p. 1426-33, Aug 15 2013. ISSN 1557-9042. Disponível em: < https://www.ncbi.nlm.nih.gov/ pubmed/23470214 >.
20. KGOSIDIALWA, O. et al. Growth Hormone Deficiency Following Traumatic Brain Injury. Int J Mol Sci, v. 20, n. 13, Jul 06 2019. ISSN 1422-0067. Disponível em: < https://www.ncbi.nlm.nih.gov/pubmed/31284550 >.
21. WAGNER, A. K. et al. Persistent hypogonadism influences estradiol synthesis, cognition and outcome in males after severe TBI. Brain Inj, v. 26, n. 10, p. 1226-42, 2012. ISSN 1362-301X. Disponível em: < https:// www.ncbi.nlm.nih.gov/pubmed/22571223 >.
22. BROD, M. et al. Impact of adult growth hormone deficiency on daily functioning and well-being. BMC Res Notes, v. 7, p. 813, Nov 18 2014. ISSN 1756-0500. Disponível em: < https://www.ncbi.nlm.nih.gov/ pubmed/25406443 >.
23. BROD M, BECK JF, HØJBJERRE L, BUSHNELL DM, ADALSTEINSSON JE, WILKINSON L, RASMUSSEN MH. Assessing the Impact of Growth Hormone Deficiency (GHD) in Adults: Interpreting Change of the Treatment-Related Impact Measure-Adult Growth Hormone Deficiency (TRIM-AGHD). Pharmacoecon Open, v. 3, n. 1, p. 71-80, Mar 2019. ISSN 2509-4254. Disponível em: < https://www.ncbi.nlm.nih.gov/ pubmed/29797004 >.
24. DÍEZ, J. J.; SANGIAO-ALVARELLOS, S.; CORDIDO, F. Treatment with Growth Hormone for Adults with Growth Hormone Deficiency Syndrome: Benefits and Risks. Int J Mol Sci, v. 19, n. 3, Mar 17 2018. ISSN 14220067. Disponível em: < https://www.ncbi.nlm.nih.gov/pubmed/29562611 >.
25. CUNEO, R. C. et al. Skeletal muscle performance in adults with growth hormone deficiency. Horm Res, v. 33 Suppl 4, p. 55-60, 1990. ISSN 0301-0163. Disponível em: < https://www.ncbi.nlm.nih.gov/ pubmed/2245969 >.
26. TWICKLER, T. B. et al. Adult-onset growth hormone deficiency: Relation of postprandial dyslipidemia to premature atherosclerosis. J Clin Endocrinol Metab, v. 88, n. 6, p. 2479-88, Jun 2003. ISSN 0021-972X. Disponível em: < https://www.ncbi.nlm.nih.gov/pubmed/12788843 >.
27. BROD, M. et al. Assessing the impact of growth hormone deficiency and treatment in adults: development of a new disease-specific measure. J Clin Endocrinol Metab, v. 99, n. 4, p. 1204-12, Apr 2014. ISSN 1945-7197. Disponível em: < https://www.ncbi.nlm.nih.gov/pubmed/24438372 >.
28. HOLMES, S. J. et al. Reduced bone mineral density in patients with adult onset growth hormone deficiency. J Clin Endocrinol Metab, v. 78, n. 3, p. 669-74, Mar 1994. ISSN 0021-972X. Disponível em: < https://www.ncbi.nlm.nih.gov/pubmed/8126140 >.
29. WEXLER, T. L. Neuroendocrine Disruptions Following Head Injury. Curr Neurol Neurosci Rep, v. 23, n. 5, p. 213-224, May 2023. ISSN 1534-6293. Disponível em: < https://www.ncbi.nlm.nih.gov/pubmed/37148402 >.
30. TANRIVERDI, F.; UNLUHIZARCI, K.; KELESTIMUR, F. Pituitary function in subjects with mild traumatic brain injury: a review of literature and proposal of a screening strategy. Pituitary, v. 13, n. 2, p. 146-53, Jun 2010. ISSN 1573-7403. Disponível em: < https://www.ncbi.nlm.nih.gov/pubmed/20037793 >.
31. LITHGOW, K. et al. Utility of serum IGF-1 for diagnosis of growth hormone deficiency following traumatic brain injury and sport-related concussion. BMC Endocr Disord, v. 18, n. 1, p. 20, Apr 02 2018. ISSN 1472-6823. Disponível em: < https://www.ncbi.nlm.nih.gov/pubmed/29609574 >.
32. TANRIVERDI, F. et al. High risk of hypopituitarism after traumatic brain injury: a prospective investigation of anterior pituitary function in the acute phase and 12 months after trauma. J Clin Endocrinol Metab, v. 91, n. 6, p. 2105-11, Jun 2006. ISSN 0021-972X. Disponível em: < https://www.ncbi.nlm.nih.gov/ pubmed/16522687 >.
33. HOHL, A. et al. Hypogonadism after traumatic brain injury. Arq Bras Endocrinol Metabol, v. 53, n. 8, p. 908-14, Nov 2009. ISSN 1677-9487. Disponível em: < https://www.ncbi.nlm.nih.gov/pubmed/20126842 >.
34. TAN, C. L. et al. The screening and management of pituitary dysfunction following traumatic brain injury in adults: British Neurotrauma Group guidance. J Neurol Neurosurg Psychiatry, v. 88, n. 11, p. 971-981, Nov 2017. ISSN 1468-330X. Disponível em: < https://www.ncbi.nlm.nih.gov/pubmed/28860331 >.
35. GLYNN, N.; AGHA, A. Which patient requires neuroendocrine assessment following traumatic brain injury, when and how? Clin Endocrinol (Oxf), v. 78, n. 1, p. 17-20, Jan 2013. ISSN 1365-2265. Disponível em: < https://www.ncbi.nlm.nih.gov/pubmed/22891644 >.
36. MAHAJAN, C.; PRABHAKAR, H.; BILOTTA, F. Endocrine Dysfunction After Traumatic Brain Injury: An Ignored Clinical Syndrome? Neurocrit Care, Feb 14 2023. ISSN 1556-0961. Disponível em: < https://www. ncbi.nlm.nih.gov/pubmed/36788181 >.
Dr. Chantel T. Debert is an associate professor and clinician scientist in the Department of Clinical Neurosciences, division of physical medicine and rehabilitation and member of the Hotchkiss brain Institute at the University of Calgary. She is the lead of the Calgary brain injury program and research lead of the Canadian association of physical medicine and rehabilitation.
Clinically, she sees patients across the age spectrum from adolescents to elderly with concussion and brain injury. Dr. Debert’s research interests include exploring the pathophysiology of concussion through a variety of imaging and fluid biomarkers and techniques, with a specific interest in hormones. She is also interested in evaluating novel treatments for patients struggling with symptoms following concussion, such as neuromodulation, exercise, nutraceuticals and pharmacological interventions.
Walter M. High, Jr., PhD
Incidence of TBI and anterior pituitary dysfunction following TBI.
Traumatic brain injury (TBI) is the leading killer and disabler of young adults under the age of 35. The annual cost of acute care and rehabilitation is estimated to be 40.6 billion dollars per year (CDC 2023). The reported incidence of pituitary dysfunction as a result of TBI has varied widely (Gasco et al., 2021). Two major meta-analyses show that the proportion of patients with some kind of pituitary dysfunction can be estimated to be approximately 27.5-32.0% (Schnieder et al., 2007, Emelifeonwu et at., 2020).
Pathophysiological basis of cognitive impairments following TBI.
TBI results in cognitive impairments in memory, executive functioning, and information processing speed. Impairments have been shown to be related to focal injury in the anterior temporal and frontal regions and diffuse axonal damage. (Levin et al., 1987; Levin et al., 1988; Levin and High, 1989; Levin et al., 1992). These impairments are stable after one year post-injury (Levin et al., 1990) and persistent even 10 years post injury (Draper and Ponsford, 2008). Few treatments are available for chronic impairments (High, 2005; High et al., 2006; Millis et al., 2001). Pharmacological (Levin et al.,1986; Plenger et al.; 1996; Poole and Agrawal; 2008) and cognitive interventions for persons with chronic TBI have met with marginal success (High et al., 2005; Cicerone et al., 2000; Cicerone et al., 2005; Sohlberg and Mateer, 1987).
Hypopituitarism following TBI.
Pituitary dysfunction caused by acute brain injury has been increasingly identified as a common sequela of TBI. The pathophysiology of pituitary dysfunction following TBI is likely related to the blood supply to the pituitary gland, particularly the anterior pituitary. (Kornblum and Fisher, 1969; Salehi et al., 2007; Maiya et al, 2008; Schneider et al., 2008). Shearing forces could impair blood flow through the long hypophyseal portal veins to the peripheral pituitary.
Kelly et al. (2000) found a 26% incidence of chronic GH deficiency and an approximately 20% occurrence of gonadotropin deficiency following TBI.
Lieberman et al. (2001) found an approximately 15% occurrence of chronic GH deficiency. Subsequent studies have shown an approximately 20% occurrence of chronic GH deficiency after moderate to severe TBI (Bondanelli et al., 2004; Tanriverdi et al., 2006; Klose et al., 2007; Aimaretti et al., 2004). Meta-analysis of high-quality studies indicated that the prevalence of chronic posttraumatic brain injury anterior pituitary dysfunction following TBI of any severity was 34% and GH deficiency was 22% (Emelifeonwu et. al., 2020). Surprisingly, little correlation between severity of TBI and increased risk of GH deficiency has been found (Bondanelli et al., 2004): Ioschimescu et al. (2015) found a 25% prevalence of GHD in veterans with mild TBI. In contrast, an association with age has been found; the highest incidence of GH deficiency after TBI is correlated with increased age (Bondanelli et al., 2004).
The time course for hypopituitarism after TBI was clarified by Aimaretti and colleagues (2005). They found that adults with panhypopitutarism 3 months after injury did not change their status at 12 months. However, adults with isolated anterior pituitary deficiency at 3 months were not consistently deficient at 12 months. After 12 months, all deficiencies found were permanent. These findings were confirmed by Klose et al. (2007).
Effects of GH deficiency and replacement on cognition for anterior pituitary disorders in etiology other than TBI.
Effects of GH deficiency and replacement on cognition following TBI have been studied relatively infrequently compared to other etiologies. (Beca et al., 2012). The effects of GH deficiency and replacement on cognition from causes other than TBI have been relatively well-studied in both persons with childhood-onset and adult-onset GH deficiency. Both childhood-onset GH deficiency (Lijffijt et al., 2003; van Dam et al., 2005) and adult GH deficiency (Baum et al., 1998; Deijen et al., 1996; Peace et al., 1998) have been associated with cognitive impairments in memory, attention/ concentration (working memory), and information processing speed. In addition, replacement of GH in persons with childhood onset GH deficiency has been shown to improve cognitive impairments in memory, attention/concentration (working memory), and information processing speed (Arwert et al., 2005). Adults with GH deficiency from reasons other than TBI show similar improvements in impaired cognition with replacement therapy (Almqvist et al., 1986; Deijen et al., 1998; Oertel et al., 2004; Sartorio et al., 1995). GH replacement has also been associated with improved quality of life (McGauley 1989; Wexler et al. 2009 1989).
The effects of GH deficiency and GH replacement on cognition have been the subject of several reviews and meta-analysis. Maruff et al. (2005) reviewed 17 studies of the effects of GH deficiency or GH replacement on cognition in persons who were GH deficient from causes other than TBI. Six out of nine cross-sectional studies reported that GH deficiency was associated with poorer cognition or replacement was associated with improved cognition. Three of the studies reported associations of GH with processing speed/ attention/working memory, and three reported associations with the ability to move information from short-term storage into longterm storage. Of the eight prospective non-TBI studies (not TBI) they reviewed, five reported associations between cognition and GH deficiency and/or replacement. Three studies reported associations with processing speed/attention/working memory, three reported associations with long-term memory, and one indicated an association with face recognition. Several studies showed associations of GH with more than one cognitive domain.
While the majority of studies showed an association between cognition and GH, several did not. However, many of the studies had relatively small sample sizes, raising the question of whether studies were powered to show any association. This problem of small sample sizes led Falleti et al. (2006) to conduct a meta-analysis of 340 GH deficient patients (non-TBI) across 14 studies. Studies of both GH deficiency and GH replacement were included. The neuropsychological domains included attention, memory, motor function, executive functioning (including working memory), spatial ability and language. For GH deficiency, each of the cognitive domains assessed (with the exception of language) showed moderate to large impairments when compared to matched controls. For the GH replacement studies, treated patients still performed moderately to largely below the levels of controls, but showed moderate improvements in cognitive performance, particularly in attention and memory, when compared to their own baselines. The investigators concluded that the analysis demonstrated the link between GH and cognitive performance and that GH treatment significantly improved cognitive performance.
The GH/IGF-1 somatotropic axis and cognitive decline associated with aging.
The mechanism for the effect of GH on cognition in humans is not well-understood. In animal literature, there is evidence that GH and IGF-1 play a role in modulating the NMDA receptor which is crucial in long-term potentiation underlying normal memory. Following CNS injury, IGF-1 has been found to increase progenitor cell proliferation and new neurons, oligodendrocytes, and blood vessels in the dentate gyrus of the hippocampus (Aberg et al., 2006). In contrast, deficiency in growth hormone and insulin-like growth factor-I decreases survival of dentate granule neurons (Lichtenwalner et al., 2006).
GH induces the production of IGF-1 in the liver. GH and IGF-1 can cross the blood-brain barrier. Both glial cells and neurons have GH receptors and IGF-1 receptors. The brain is also capable of synthesizing GH and IGF-1 (Creyghton et al., 2004). Binding sites for IGF-1 are found primarily in the hippocampus, amygdala, and parahippocampal gyrus (Adem et al., 1989). GH receptors are concentrated mainly in the choroid plexus, hippocampus, putamen and hippocampus. GH receptors are found in 2-4 times greater concentration in the hippocampus compared to other brain areas (Lai et al., 1991; 1993; Bennett and Robinson, 2000). These areas of the brain are extremely important for memory and information processing efficiency. Damage to these areas following TBI underlies many of the cognitive impairments following TBI.
These same cognitive functions are particularly vulnerable to the effects of normal aging, and to disease states associated with aging such as Alzheimer’s Disease (AD). This has led investigators to speculate that the GH/IGF-1 somatotropic axis may play a role in the cognitive decline associated with aging (Arwert et al., 2005; van Dam 2006). GH/IGF-1 serum levels (Strasburger et al., 2001) and receptors numbers in the brain (Lai et al., 1993; Kar et al., 1997) decline with age.
A significant number of important studies investigating the relationship between IGF-1 serum levels and cognition have now been published. Arwert et al.’s (2005) meta-analysis identified 13 studies that studied a total of 1981 subjects. One of the included studies had a particularly large cohort (Dik et al., 2003; n=1318). Arwert et al. conducted their meta-analysis for all 13 studies and then for the 12 studies excluding the large Dik et al. study. These studies showed an overall large effect size for the correlation of IGF-1 levels and cognitive functioning in healthy elderly measured primarily by the mini mental status examination (MMSE). Excluding the Dik et al. study, the effect size was moderate. Looking across the reported studies, 5 studies included measures of information processing speed and all five reported significant correlations with IGF-1 levels (Aleman et al., 1999; 2000; Dik et al., 2003; Papadakis et al., 1995; 1996). Two studies reported correlations with memory (Aleman et al., 2001; Morley et al., 1997), two reported correlations with attention (Arwert et al., 2006; Cherrier et al., 2004), and four studies included only the MMSE (Kalmijn et al., 2000; Paolisso et al., 1997; Rollero et al. ,1998; Watanabe et al., 2004). All 13 studies demonstrated a correlation between objective cognitive performance and IGF-1 level.
The large study by Dik et al. (2003) warrants special consideration for several reasons. The first is its large size (n=1318; aged 65-88 years). The second reason is the breadth of the cognitive measures (information processing speed, immediate recall, delayed recall, fluid intelligence, and the MMSE). The third is its prospective longitudinal design (subjects were retested after 3 years). Fourth, important putative confounders were measured including age, sex, education, BMI, serum albumin, number of chronic diseases, and a physical activity index. The study clearly demonstrated that low IGF-1 levels were associated with slower information processing speed and with greater decline in information processing speed over a three-year period compared to elderly with higher IGF-1 levels.
Arwert et al. (2005) investigated fMRI in adults with childhood onset of GH deficiency (non-TBI) while doing a working memory task compared to healthy controls. Memory speed was slower for the GH-deficient group and the GH-deficient group complained of more fatigue and less vigor. Imaging data showed that the patients had increased activity in dorsolateral/ventrolateral prefrontal cortex, anterior cingulate cortex, parietal cortex, and motor and supplementary cortex, as well as in the thalamus and precuneus area. The investigators interpreted their results as being consistent with compensatory recruitment of or dorsal prefrontal regions associated with increased effort to complete the working memory task. Arwert et al. (2006) followed up this finding with a subsequent study of fMRI pre- and post-GH replacement therapy in a doubleblind, placebo-controlled study. Six months of GH substitution resulted in improved memory functioning, for both long-term and working memory. Decreased activation in the ventrolateral prefrontal cortex was observed after GH treatment as compared with placebo treatment, indicating decreased effort and more efficient recruitment of the neural system involved.
This is consistent with decreased effort being required to complete the working memory task. The investigators concluded that GH substitution in GHD patients is beneficial for cognitive functioning, the effects of which can be visualized by means of neuroimaging.
The contribution of GH deficiency to cognitive impairments following TBI.
Leon-Carrion et al. (2007) were able to show that persons who were GH deficient after TBI (n=11), showed cognitive impairment in attention, executive functioning, memory and emotion compared to persons with TBI without GH deficiency. Such findings compelled High et al. (2010) to assess the effects of rhGH replacement on neuropsychological functioning in adults who were GH deficient following chronic moderate-to-severe TBI. This led to the first published double-blind, placebo-controlled study to show improvements in information processing speed, motor speed, and memory following GH replacement for GH deficiency following moderate-to-severe TBI. Twenty-three persons with TBI were randomized, evaluated (at baseline) with neuropsychological and functional measures, and treated for one year with active rhGH (n=12) or placebo (n=11). Both the participants and the evaluators were blinded concerning which treatment group the participants were assigned until after the one-year evaluations were complete. The two groups were comparable in age, education, initial severity of TBI, and level of persisting disability when the study started. The neuropsychological measures included measures of language, visual/spatial functioning, upper extremity motor functioning, information processing efficiency, working memory/ attention, learning and memory, executive functioning, intellectual functioning, and emotional functioning. Improved functioning in verbal memory, information processing speed, and upper extremity motor speed were observed after replacement of GH but not with placebo after one year of treatment.
Subsequent studies have confirmed these results. Reimunde et al. (2010) showed improved reasoning abilities in 11 persons with GH deficiency following chronic TBI of unspecified severity. Moreau et al. (2013) showed improved memory and quality of life following open label treatment with GH in 23 persons who were GH deficient following chronic severe TBI. Feeney et al. (2017) showed memory improvements after TBI were greater in patients with higher serum IGF-I at baseline. These findings suggest that the importance of the GH/IGF-I system as a potential therapeutic target following TBI.
In the context of the large literature showing the detrimental effects of GH and IGF-1 deficiency on memory and information processing speed, and the improvement following replacement after etiologies other than TBI, the commensurate findings following TBI are hardly surprising. What is surprising is the resistance among some clinicians to test for GH/IGF-1 deficiency following TBI and to replace it if deficient. While the consensus of the non-TBI literature and definitive meta-analyses is that GH replacement is beneficial for improved cognition, replacement following TBI is often met with skepticism. The large incidence of GH and IGF-1 deficiency for persons following TBI continues to be under-appreciated. Despite the clear evidence that large numbers of persons with TBI have GH deficiency, few have ever been screened for GH deficiency.
Why is this the case? We can only speculate. Historically, the establishment of a linkage between GH deficiency and cognitive impairment after TBI has been difficult to demonstrate given the context of often large amounts of structural brain damage, especially in moderate to severe TBI and small sample sizes. The symptoms of GH deficiency may be confounded by the effects of structural damage following TBI. More importantly, it should be noted that distinguishing cognitive impairment resulting from GH deficiency after TBI is inherently more difficult than making the association for causes other than TBI. This is because cognitive impairments typically seen after TBI, as the result of primary damage to the frontal and anterior temporal cortices and the underlying white matter connections, are completely confounded by the effects of GH deficiency on cognition. It is extremely plausible that the well-known cognitive impairments typical of moderate to severe TBI, that for decades have been attributed to the primary injury, might be at least partially mediated by a lack of GH in a substantial subset of persons with TBI, and therefore at least partially reversible.
We have assumed for decades that the devastating impairments in memory and attention following moderate to severe TBI are entirely due to structural damage to these cortical areas and the underlying white matter. This is not a reasonable assumption given what we know about the incidence of GH/IGF-1 deficiency following moderate to severe TBI and the important role of GH/ IGF-1 in attention and memory. GH replacement in persons who are deficient following TBI is one of the few treatments that have been shown to be effective. The question is whether the cost of treatment is worth the anticipated improvements in cognition and quality of life. At the very least, more research needs to be done in this area.
This review has centered primarily on GH deficiency and replacement following moderate to severe TBI. We know much less about GH deficiency and replacement following mild TBI. Approximately 80 percent of persons with TBI have sustained a mild TBI. The percentage of persons with mild TBI who also have GH deficiency in the total population of persons with mild TBI is not known. However, we do know that GH deficiency occurs with surprising frequency in military convenience samples sustaining blast injuries, rivaling rates seen following moderate to severe TBI (Wilkinson et. al., 2012). Persons are referred to clinic for persisting post-concussive symptoms that often parallel symptoms of GH deficiency, e.g. fatigue, decreased attention, and decreased memory. Clinicians often dismiss reported symptoms when no apparent structural damage is visualized on CT or MRI. However, in the context of no, or relatively minimal, structural damage to the brain per imaging studies, GH deficiency may play a very significant role in the persistence of symptoms which need to be investigated by the treating clinician (Mercier et al., 2021).
Adem A. Jossan S. d’Argy R. Insulin-like growth factor 1 (IGF-1) receptors in the human brain: Quantitative autoradiographic localization. Brain Research, 45, 299-303, 1989
Aimaretti G. Ambrosio MR. Di Somma C., et al., Residual pituitary function after brain injury-induced hypopituitarism: a prospective 12-month study. J Clin Endocrinol Metab. 2005.
Aimaretti G. Baldelli R. Corneli G., et al., GHRH and GH secretagogues: Clinical perspectives and safety. Pediatric Endocrinology Reviews, 2 (1), 86-92, 2004.
Aleman A. Verhaar HJ. de Haan EHF., et al., Insulin-like growth factor-I and cognitive function in healthy older men. Journal of Clinical Endocrinology and Metabolism, 84, 471-475, 1999.
Aleman A. Verhaar HJ. de Haan EHF., et al., Age-sensitive cognitive function, growth hormone and insulinlike growth factor 1 plasma levels in healthy older men. Neuropsychobiology, 41, 73-78, 2000.
Aleman A. de Vries WR. Koppeschaar HPF., et al., Relationship between circulating levels of sex hormones and insulin-like growth factor-I and fluid intelligence in older men. Experimental Aging Research, 27, 283291, 2001.
Almqvist O. Thoren M. Saaf M. et al., Effects of growth hormone substitution on mental performance in adults with growth hormone deficiency: A pilot study. Psychoneuroendocrinology, 11, 347-352, 1986.
Arwert LI. Deijen JB. & Drent ML. The relation between insulin-like growth factor I levels and cognition in healthy elderly: A meta-analysis. Growth Hormone & IGF Research, 15, 416-422,2005.
Arwert LI. Veltman DJ. Deijen JB., et al., Growth hormone deficiency and memory functioning in adults visualized by functional magnetic resonance imaging. Neuroendocrinology, 82, 32-40, 2005.
Arwert LI. Veltman DJ. Deijen JB., et al., Effects of growth hormone substitution therapy on cognitive functioning in growth hormone deficient patients: A functional MRI study. Neuroendocrinology, 83, 12-19, 2006.
Arwert LI, Deijen JB, Müller M, et al., Long-term growth hormone treatment preserves GH-induced memory and mood improvements: a 10-year follow-up study in GH-deficient adult men. Horm Behav. 47(3):343-349, 2005.
Baum HBA. Katznelson L. Sherman JC., et al., Effects of physiological growth hormone (GH) therapy on cognition and quality of life in patients with adult onset GH deficiency. Journal of Clinical Endocrinology and Metabolism, 83, 3184-3189, 1998.
Beca SG, High WM Jr, Masel BE, et al. What are critical outcome measures for patients receiving pituitary replacement following brain injury?. Pituitary. 15(1):10-19, 2012.
Bennett PA. & Robinson ICAF. The central nervous system as a direct target for growth hormone action. In Smith, R.G., Thorner, M.O. (Eds.), Human Growth Hormone: Basic and Clinical Research, (pp. 145-162). Totowa, NJ: Humana Press, 2000.
Bondanelli M. De Marinis L. Ambrosio MR., et al., Occurrence of pituitary dysfunction following traumatic brain injury. Journal of Neurotrauma, 21, 685-696, 2004.
Cherrier MM. Plymate S. Mohan S., et al., Relationship between testosterone supplementation and insulinlike growth factor-I levels and cognition in healthy older men. Psychoneuroendocrinology, 29, 65-82, 2004.
Cicerone KD. Dahlberg C. Kalmar K., et al., Evidence-based cognitive rehabilitation: Recommendations for clinical practice. Archives of Physical Medicine and Rehabilitation, 81, 1596-1615, 2000.
Cicerone KD. Dahlberg C. Malec JF, et al., Evidence-based cognitive rehabilitation: Updated review of the literature from 1998 through 2002. Archives of Physical Medicine and Rehabilitation, 86, 1681-1692, 2005.
Creyghton WM. van Dam PS. & Koppeschaar HPF. The Role of the somatotropic system in cognition and other cerebral functions. Seminars in Vascular Medicine, 4, 167-172, 2004.
Dik MG. Pluijm SMF, Jonker C., et al., Insulin-like growth factor I (IGF-I) and cognitive decline in older persons, Neurobiology of Aging, 24, 573-581, 2003.
Deijen JB. de Boer H. Blok GJ., et al., Cognitive impairments and mood disturbances in growth hormone deficient men. Psychoneuroendocrinology, 21, 313-322, 1996.
Deijen JB. de Boer H. & van der Veen, A. Cognitive changes during growth hormone replacement in adult men. Psychoneuroendocrinology, 23, 45-55, 1998.
Draper K. & Ponsford J. Cognitive functioning ten years following traumatic brain injury and rehabilitation. Neuropsychology, 22, 618-625, 2008.
Emelifeonwu JA, Flower H, Loan JJ, et al., Prevalence of Anterior Pituitary Dysfunction Twelve Months or More following Traumatic Brain Injury in Adults: A Systematic Review and Meta-Analysis. J Neurotrauma. 37(2):217-226, 2020.
Falleti MG. Maruff P. Burman P., et al., The Effects of growth hormone (GH) deficiency and GH replacement on cognitive performance in adults: A meta-analysis of the current literature. Psychoneuroendocrinology, 31, 681-691, 2006.
Feeney C, Sharp DJ, Hellyer PJ, et al. Serum insulin-like growth factor-I levels are associated with improved white matter recovery after traumatic brain injury. Ann Neurol. 82(1):30-43, 2017.
Gasco V, Cambria V, Bioletto F, et al. Traumatic Brain Injury as Frequent Cause of Hypopituitarism and Growth Hormone Deficiency: Epidemiology, Diagnosis, and Treatment. Front Endocrinol (Lausanne). 12:634415, 2021.
High Jr WM, Roebuck-Spencer T. Sander AM., et al. Early versus later admission to postacute rehabilitation: Impact on functional outcome after traumatic brain injury. Archives of Physical Medicine and Rehabilitation, 87, 334-342, 2006.
High Jr WM. Effectiveness of TBI rehabilitation programs. In: High Jr WM, Sander AM. Struchen MA, et al., Rehabilitation for Traumatic Brain Injury, New York: Oxford University Press, 2005.
High Jr WM. Sander AM, Struchen MA, et al., Rehabilitation For Traumatic Brain Injury. New York: Oxford University Press, 2005.
High Jr WM, Briones-Galang M, Clark JA, et al., Effect of Growth Hormone Replacement Therapy on Cognition after Traumatic Brain Injury. Journal of Neurotrauma, Sep; 27(9):1565-75, 2010.
Ioachimescu AG, Hampstead BM, Moore A, et al., Growth hormone deficiency after mild combat-related traumatic brain injury. Pituitary. 18(4):535-541, 2015.
Kalmijn S. Janssen JAMJ. Pols HAP, et al., A prospective study on circulating insulin-like growth factor I (IGF-I), IGF-binding proteins, and cognitive function in the elderly. Journal of Clinical Endocrinology and Metabolism, 85, 4551-4555,2000.
Kar S. Seto D. Dorè S., et al.. Insulin-like growth factors-I and –II differentially regulate endogenous acetylcholine release from the rat hippocampal formation. Proceedings of the National Academy of Science of the United States of America, 94, 14054-14059, 1997.
Kelly DF, Gonzalo IT, Cohan P., et al., Hypopituitarism following traumatic brain injury and aneurismal subarachnoid hemorrhage: A preliminary report. Journal of Neurosurgery, 93, 743-752, 2000.
Klose M. Juul A. Struck J., et al., Acute and long-term pituitary insufficiency in traumatic brain injury: A prospective single-centre study. Clinical Endocrinology, 67, 598-606, 2007.
Kornblum RN & Fisher RS. Pituitary lesions in craniocerebral injuries. Archives of Pathology, 88, 242248,1969.
Lai Z. Emtner M. Roos P., et al., Characterization of putative growth hormone receptors in human choroid plexus. Brain Research, 546, 222-226, 1991.
Lai Z. Roos P, Zhai O., et al. Age-related reduction of human growth hormone-binding sites in the human brain. Brain Research, 621, 260-266, 1993.
Leon-Carrion J. Leal-Cerro A. Cabezas FM., et al., Cognitive Deterioration Due to GH Deficiency in Patients With Traumatic Brain Injury: a Preliminary Report. Brain Injury, 21, 871-5, 2007.
Levin HS, Gary HE Jr, Eisenberg HM, et al. Neurobehavioral outcome 1 year after severe head injury. Experience of the Traumatic Coma Data Bank. J Neurosurg. 73(5):699-709, 1990.
Levin HS, & High Jr WM. Contributions of neuroimaging to neuropsychological research on closed head injury. Neuropsychology, 3, 243-254, 1989.
Levin HS. Mattis S. Ruff RM., et al., Neurobehavioral outcome following minor head injury: A three center study. Journal of Neurosurgery, 66, 234-243, 1987.
Levin HS, Peters BH, Kalisky Z., et al., Effects of oral physostigmine and lecithin on memory and attention in closed head injured patients. Central Nervous System Trauma, 3, 333342, 1986.
Levin HS. Williams DH. Crofford MJ., et al., Relationship of depth of brain lesions to consciousness and outcome after closed head injury. Journal of Neurosurgery, 69, 861-866, 1988.
Levin HS. Williams DH, Eisenberg HM, et al., Serial magnetic resonance imaging and neurobehavioral findings after mild to moderate closed head injury. Journal of Neurology, Neurosurgery and Psychiatry, 55, 255-262, 1992.
Lichtenwalner RJ. Forbes ME. Sonntag WE., et al., Adult-onset deficiency in growth hormone and insulinlike growth factor-I decreases survival of dentate granule neurons: Insights into the regulation of adult hippocampal neurogenesis. Journal of Neuroscience Research, 83, 199-210,2006.
Lieberman SA, Oberoi AL. Gilkison CR., et al. Prevalence of neuroendocrine dysfunction in patients recovering from traumatic brain injury. The Journal of Clinical Endocrinology & Metabolism, 86, 2752-2756. 2001.
Lijffijt M. van Dam PS. Kenemans JL. et al., Somatotropic-axis deficiency affects brain substrates of selective attention in childhood-onset growth hormone deficient patients. Neuroscience Letters, 353, 123-126, 2003.
Maiya S. Newcombe V. Nortje J. et al., Magnetic resonance imaging changes in the pituitary gland following acute traumatic brain injury. Intensive Care Medicine, 34, 468-475, 2008.
Maruff P. Fallet M. Cognitive function in gorwoth hormone deficiency and growth hormone replacement. Hormone Research, 64, 100-108, 2005.
McGauley GA Quality of life assessment before and after growth hormone treatment in adults with growth hormone deficiency. Acta Paediatrica Scandinavica, 356, 70-72, 1989.
Millis SR, Rosenthal M, Novack TA, et al. Long-term neuropsychological outcome after traumatic brain injury. J Head Trauma Rehabil. 16(4):343-355, 2001.
Mercier LJ, Kruger N, Le QB, et al., Growth hormone deficiency testing and treatment following mild traumatic brain injury. Sci Rep. 11(1):8534, 2021.
Moreau OK, Cortet-Rudelli C, Yollin E, et al., Growth hormone replacement therapy in patients with traumatic brain injury. J Neurotrauma. 30(11):998-1006, 2013.
Morley JE. Kaiser F. Raum WJ., et al. Potentially predictive and manipulable blood serum correlates of aging in the healthy human male: Progressive decreases in bioavailable testosterone, dehydroepiandrosterone sulfate, and the ratio of insulin-like growth factor I to growth hormone. Proceedings of the National Academy of Science, U.S.A., 94, 7537-7542, 1997.
Oertel H. Schneider HJ. Stalla GK., et al. The Effect of growth hormone substitution on cognitive performance in adult patients with hypopituitarism. Psychoneuroendocrinology, 29, 839-850, 2004.
Papadakis MA. Grady D. Tierney MJ., et al., Insulin-like growth factor 1 and functional status in healthy older men. Journal of the American Geriatric Society, 53, 1350-1355 1995.
Papadakis MA. Grady D. Black D., et al., Growth hormone replacementin healthy older men improves body composition but not functional ability, Annals of Internal Medicine, 124, 708-716, 1996.
Paolisso G. Ammendola S. Del Buono A., et al., Serum levels of insulin-like growth factor-I (IGF-I) and IGFbinding protein-3 in healthy centenarians: Relationship with plasma leptin and lipid concentrations, insulin action, and cognitive function. Journal of Clinical Endocrinology and Metabolism, 82, 2204-2209, 1997.
Peace KA. Orme SM. Padayatty SJ., et al. Cognitive dysfunction in patients with pituitary tumour who have been treated with transfrontal or transsphenoidal surgery or medication. Clinical Endocrinology, 49, 391396, 1998.
Plenger PM. Dixon CE. Castillo RM., et al., Subacute methylphenidate treatment for moderate to moderately severe traumatic brain injury: A preliminary double-blind placebo-controlled study. Archives of Physical Medicine and Rehabilitaiton, 77, 536-540, 1996.
Poole NA & Agrawal N. Cholinomimetic agents and neurocognitive impairment following head injury: A systematic review. Brain Injury, 22, 519-534, 2008.
Reimunde P, Quintana A, Castañón B, et al. Effects of growth hormone (GH) replacement and cognitive rehabilitation in patients with cognitive disorders after traumatic brain injury. Brain Inj. 25(1):65-73, 2011.
Rollero A. Murialdo G. Fonzi S., et al., Relationship between cognitive function, growth hormone and insulin-like growth factor I plasma levels in aged subjects, Neuropsychobiology, 38, 73-79, 1998.
Salehi F. Kovacs K. Scheithauer BW., et al., Pituitary tumor-transforming gene in endocrine and other neoplasms: A review and update. Endocrine-Related Cancer, 15, 721-743, 2008.
Sartorio A. Molinari E. Riva G., et al., Growth hormone treatment in adults with childhood onset growth hormone deficiency: Effects on psychological capabilities. Hormone Research, 44, 6-11, 1995.
Schneider M. Schneider HJ. Yassouridis A., et al., Predictors of anterior pituitary insufficiency after traumatic brain injury. Clinical Endocrinology, 68, 206-212, 2008.
Sohlberg MM. & Mateer CA. Effectiveness of an attention-training program. Journal of Clinical and Experimental Neuropsychology, 9, 117-130, 1987.
Strasburger CJ, Bidlingmaier M, Wu Z, et al., Normal values of insulin-like growth factor I and their clinical utility in adults. Horm Res. 55 Suppl 2:100-105, 2001.
Tanriverdi F. Senyurek H. Unluhizarci K., et al., High risk of hypopituitarism after traumatic brain injury: A prospective investigation of anterior pituitary functioning in the acute phase and 12 months after trauma. Journal of Clinical Endocrinology & Metabolism, 91, 2105-2111, 2006.
van Dam PS. Somatotropin therapy and cognitive function in adults with growth hormone deficiency. Treatments in Endocrinology, 5, 159-170, 2006.
van Dam PS. de Winter CF. de Vries R., et al., Childhood-onset growth hormone deficiency, cognitive function and brain N-acetylaspartate. Psychoneuroendocrinology, 30, 357-363, 2005.
Watanabe T. Yamamoto H. Idei T., et al. Influence of insulin-like growth factor-I and hepatocyte growth factor on carotid atherosclerosis and cognitive function in the elderly. Dementia and Geriatric Cognitive Disorders, 18, 67-74, 2004.
Wexler T, Gunnell L, Omer Z, et al. Growth hormone deficiency is associated with decreased quality of life in patients with prior acromegaly. J Clin Endocrinol Metab. 009;94(7):2471-2477. doi:10.1210/jc.2008-2671
Walter M. High, Jr., PhD, is a neuropsychologist currently in private practice in New Mexico. Dr. High has published extensively on neuropsychological and functional outcomes following TBI of all severity levels, growth hormone replacement following TBI, and the long term interactive effects of concussion from blast injuries and chronic posttraumatic stress disorder (PTSD) in veterans.
Brent E. Masel, MD • Stefanie N. Howell, PhD
The size of a pea, and weighing only 600 grams, the pituitary is known as the “master gland” due to its significant control over the neuroendocrine system. Its importance is stressed in a publication by the Institute of Medicine which states “… That hormonal alterations substantially modify the posttraumatic clinical course in the success of therapy and rehabilitation underscores
the need for the identification an appropriate timely management of hormone deficiencies to optimize patient recovery from head trauma, to improve quality of life and to avoid the long-term adverse consequences of untreated hypopituitarism”. 1
The pituitary gland is a two-lobed structure that sits within a bony cave, the sella turcica (Turk’s saddle), deep within the middle cranial fossa of the brain. It is attached to the hypothalamus by the slender 2-3 mm pituitary stalk. The hypothalamus synthesizes specific inhibiting and releasing hormones that are secreted directly into the portal vessels within the pituitary stalk, which then carry those hormones to the secretory cells within the anterior pituitary lobe. The pituitary stalk supplies pituitary hormones from the hypothalamus directly to the posterior pituitary lobe.
The superior hypophyseal arteries branch from the internal carotid, providing blood supply to the hypothalamus. The pituitary then receives its blood supply from the long and short hypophyseal vessels that form the hypothalamic portal circulation. Long hypophyseal portal veins arise from the median eminence and course downward to provide blood to the anterior lobe of the pituitary. These vessels provide the anterior lobe with 70-90% of its blood supply. As these vessels must traverse the diaphragma sella, which forms the roof of the pituitary fossa, they are more susceptible to direct mechanical trauma, increased intracranial pressure, low blood flow and pituitary swelling. The short portal veins have a less tenuous course, as they arise from below the diaphragma sella, and supply less than 30% of the anterior gland –predominantly to the medial portion.
The trophic hormone cells are located within specific areas of the anterior pituitary. This renders certain trophic hormones susceptible to vascular trauma, depending on the blood supply. The cells responsible for the secretion of growth hormone are located predominantly in the lateral wings of the anterior lobe, supplied by the long hypophyseal portal veins, and make up approximately 40% of the pituitary cells. The cells responsible for ACTH are central median in location and make up about 20% of the anterior pituitary. The cells responsible for thyroid stimulating hormone comprise 5% of the anterior pituitary and are located in the anterior medial region of the gland. The gonadotropin-secreting cells, located in the lateral wings, make up 10 to 15% of the anterior pituitary.
Incidence
The reported incidence of post-traumatic hypopituitarism (PTHP) has been variable, depending on the time since injury in each study, as well as the methods, laboratory assays, parameters and statistics used for testing. In general, it is felt that approximately 30-50% of individuals with moderate-severe TBI may have PTHP. Individuals with mild TBI who remain symptomatic after 12 months will have similar numbers.2 Growth hormone deficiency (GHD) is the most common problem, with rates of approximately 15%. Gonadotropin deficiencies will occur at approximately 10%. Thyroid and adrenocorticoid deficiencies are 4-12%. And of course, individuals may have more than one axis deficiency.2
Function
The pituitary gland functions as part of a feedback loop, analogous to our thermostat and our air-conditioning unit. As an example, the pituitary will produce thyroid stimulating hormone.
That hormone stimulates the thyroid gland to make thyroid hormone. If the hypothalamus detects that thyroid hormone is high, it will send an inhibiting hormone to the thyroid, and subsequently, the amount of thyroid stimulating hormone will decrease. If it detects that the amount of circulating thyroid hormone is low, it will increase production of thyroid stimulating hormone. A similar mechanism is in play with the other pituitary hormones and their target glands. The incidence of secondary hypothyroidism after a TBI has been estimated to be approximately 4%.2 Symptoms may include fatigue, hair loss, constipation, cold intolerance, and intellectual impairment.
In addition to thyroid dysfunction, many other hormone axes may be disrupted post-TBI.
Hypothalamic Pituitary Adrenal (HPA) Axis: In response to ACTH, the adrenal glands produce mineralocorticoids, glucocorticoids, and adrenal androgens, which are responsible for secondary sexual characteristics in females. Mineralocorticoids and glucocorticoids modulate our immune responses, blood pressure, vascular volume, and our stress response. Symptoms of adrenal insufficiency may include extreme fatigue, loss of appetite and abdominal pain.
Hypothalamic Pituitary Gonadal (HPG) Axis: The gonadotropin cells produce follicle stimulating hormone (FSH) and luteinizing hormone (LH). They are regulated by gonadotropin releasing hormone from the hypothalamus. Deficiencies in adult males may result in decreased libido and infertility, as well as muscular weakness, decreased muscle mass and loss of secondary sexual characteristics, and other symptoms. Women may exhibit decreased libido, infertility, decreased bone density, and secondary amenorrhea, among other symptoms.
Hypothalamic Pituitary Somatotropin (HPS) Axis: The growth hormone (GH) secreting cells make up approximately 40% of the total anterior pituitary. Secretion of GH is pulsatile, predominantly occurring during the beginning of slow wave sleep. GH has no direct effects on target tissues but stimulates the synthesis and release of insulin-like growth factor-1 (IGF-1) predominantly from the liver.
GH deficiency in children is characterized by increased fat and short stature. In adults, GH deficiency can produce an abnormal lipid profile, decreased strength, decreased bone density, and decreased lean body fat. Common symptoms may include fatigue, decreased muscle mass, reduced exercise performance, and impaired cognitive (poor memory and concentration) and psychological (increased anxiety and depression) functioning.
and the Gut-Brain Axis
Recently described, brain injury associated fatigue and altered cognition (BIAFAC) refers to a subset of individuals with mild TBIs who develop PTHP and other metabolic abnormalities, including altered gut microbiome and amino acid utilization.3
BIAFAC is characterized by profound fatigue (which does not resolve with sleep), altered cognition (predominantly short-term memory loss and executive function complaints), an abnormal GH stimulation test, and a positive response to growth hormone replacement.
Although these complaints are common to post-concussion syndrome, the symptoms of BIAFAC generally do not appear until over 6 months after the brain injury. With GH replacement, improvement in fatigue occurs in 3 months and cognition improves in approximately 5-6 months. When the GH is stopped, fatigue returns in approximately 3 months and cognition becomes impaired at 6 months.4
Amino acids are the building blocks of our bodies. There are 10 essential amino acids which must be ingested in our food. The ten non-essential amino acids (a misnomer, as they are “essential” to functions) are synthesized by our bodies. Amino acids are critical to protein formation. They also make up our neurotransmitters; therefore, hypoaminoacidemia, such has been discovered in TBI, can produce profound physical, cognitive and neurological dysfunction.5 For example, glutamate is the primary excitatory neurotransmitter in the CNS. Arginine is a precursor for nitric oxide. Tryptophan is a precursor to serotonin. Serotonin and nitric oxide are both tied to cognition and mood.6
Studies have shown that an altered gut microbiome may be responsible for amino acid abnormalities after TBI.7 Simply put, the bacteria in our gut decide what gets absorbed into the body, what gets flushed straight out in the feces, and what gets absorbed by bacteria for their own growth. A consistent balance of our gut microbiota is required for health.
The literature supports the concept that the gut brain axis is a functional organ system that includes amino acid signals and short chain fatty acids as part of what is a bidirectional conversation between the CNS and the gut microbiome. It appears that the vagus nerve and the autonomic nervous system in the spinal cord are the conduits for communication between the gut and the brain. As one might expect, gut dysbiosis has also been found in spinal cord injuries.8 A logical next step would be to correct the gut dysbiosis, perhaps with prebiotics, probiotics, or even fecal transplants.
When evaluating PTHP, the first step is a detailed history and physical examination, including a thorough neurologic examination. Assuming the main complaints are fatigue and cognitive issues, other sources of these symptoms must be sought. Is the patient depressed and/or anxious? Is there a history of drug and/or alcohol abuse? Are there symptoms suggesting a metabolic disease or sleep disturbance? Is the general physical and neurologic exam normal? The physician will most likely order screening labs such as a complete blood count, vitamin levels, and a comprehensive metabolic panel. If negative, the physician should intensify the evaluation looking for neuroendocrine dysfunction.
Although imaging frequently does not identify the source of hypopituitarism, an MRI with special attention to the hypothalamus and the pituitary is recommended.
Gonadotropin function should be tested by FSH and LH, as well as a 17-beta-estradiol in females or testosterone in males. The patient should have a TSH and free T4 testing for thyroid dysfunction.
The patient should have a morning prolactin level and a cortisol drawn for testing of the HPA axis. Due to its pulsatile nature and short half-life, random blood draws of GH are of little value. Therefore, one should also test for IGF-1 to screen for GHD. All these tests can be ordered by any provider and require one simple blood draw.
It is important to highlight that IGF-1 is merely a screening test for GHD and, unfortunately, has many false negatives. If the patient’s pituitary testing reveals abnormalities suggesting PTHP, the likelihood of having a problem with the GH axis is greater--an Endocrinology referral should be made for more definitive provocative testing. Providers should also use prior experience and intuition, referring a patient to an Endocrinologist regardless of the IGF-1 status.
With treatment for any post-TBI pituitary deficiencies identified, most patients will see improvement and possibly resolution in symptoms-- we often hear the phrase, “I got my life back.”
1. Institute of Medicine, Gulf War and Health, Volume 7: Long-term Consequences of Traumatic Brain Injury. Washington DC: The National Academies Press, 2009.
2. Schneider, HJ., Kreitschmann-Andermahr, I., Ghigo, E., Stalla, GK., Agha, A. (2007). Hypothalamopituitary dysfunction following traumatic brain injury and aneurysmal subarachnoid hemorrhage: A systematic review. JAMA, 298(12), 1429-1438.
3. Yuen, KCJ., Masel, BE, Reifschneider, KL., Sheffield-Moore, M., Urban, RJ., Pyles, RB. (2020). Alterations of the GH/IGF-I axis and gut microbiome after traumatic brain injury: A new clinical syndrome?. The Journal of Clinical Endocrinology & Metabolism, 105(9), 3054-3064.
4. Urban, R. (2020). A Treatable Syndrome in Patients with Traumatic Brain Injury. Journal of Neurotrauma, 37(8), 1124-1125.
5. Durham, WJ., Foreman, JP., Randolph, KM., Danesi, CP., Spratt, H., Masel, BD…. Masel, BE. (2017). Hypoaminoacidemia characterizes chronic traumatic brain injury. Journal of Neurotrauma 34(2), 385–390.
6. Armstrong, PA., Venugopal, N., Wright, TJ., Randolph, KM., Batson, RD., Yuen, KCJ…. Pyles, RB. (2023). Traumatic brain injury, abnormal growth hormone secretion, and gut dysbiosis. Best Practice & Research Clinical Endocrinology & Metabolism, 37(6), 1018-1041.
7. Urban, RJ., Pyles, R., Stewart, C., Ajami, N., Randolph, K., Durham, WJ…. Sheffield-Moore, M. (2020). Altered Fecal Microbiome Years After Traumatic Brain Injury. Journal of Neurotrauma, 37(8), 10371051.
8. Jing, Y., Bai, F., Yu, Y. (2021). Spinal cord injury and gut microbiota: A review. Life Sciences, 266.
9. Hoffman, DM., O’Sullivan, O., Ho, K. (1994). Diagnosis of growth-hormone deficiency in adults. The Lancet, 343(8905), 1064-1068.
Brent E. Masel, MD, is a Board Certified Neurologist, Clinical Professor of Neurology at UTMB and Executive VP for Medical Affairs at the Centre for Neuro Skills. He is the National Medical Director for the Brain Injury Association of America. He has published extensively on pituitary dysfunction after TBI.
Stefanie N. Howell has her Ph.D. in Cognitive and Behavioral Neuroscience and serves as Senior Neuroscientist at the Centre for Neuro Skills. Her research is focused on recognizing and addressing underrecognized comorbidities of brain injury, including sleep-wake disturbances and neuroendocrine dysfunction.
NEUROREHABILI TATION & RESEARCH HOSPITAL