Abigail Araia
Senior Thesis | 2024
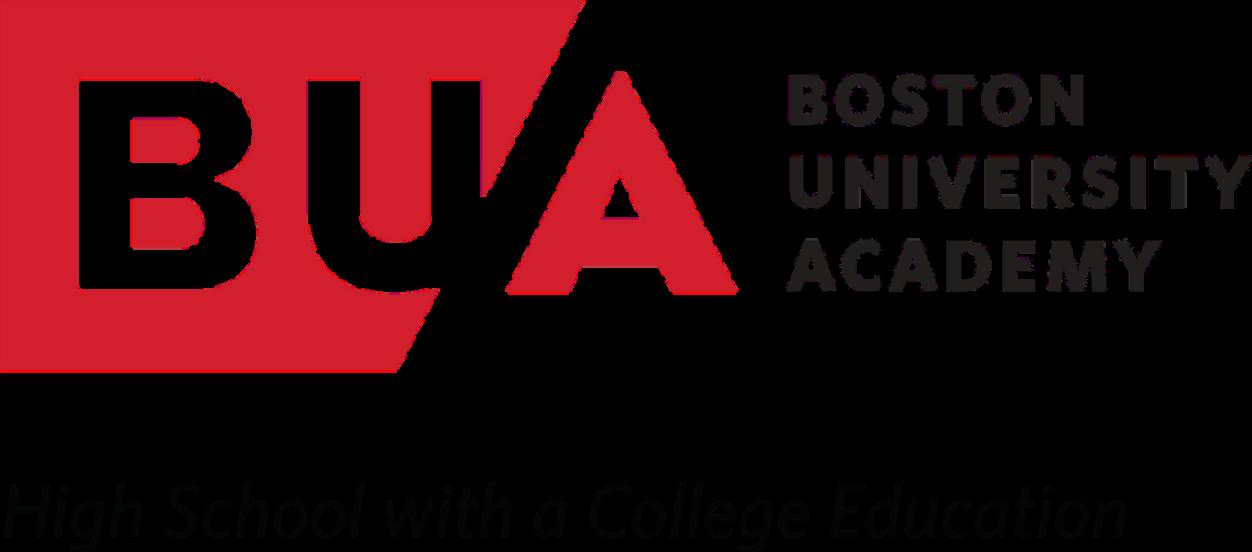
Abigail Araia
Dr. Krivacek
March 7, 2024
The History and Ethics of CRISPR
CRISPR, a rapidly developing genetic modification technique, is the topic of conversations ranging from potential cures of neurodegenerative diseases to harmful alteration of children’s genomes. There is much excitement about the, yet to be determined, capabilities of CRISPR. When a scientific technique makes the cure to incurable diseases seem possible and near, there is sure to be endless amounts of conversation and research conducted with it at the center. CRISPR is commonly discussed as a potential cure for cancer and neurodegenerative disease; however, there would be no incurable illness if CRISPR can be developed to work as well as scientists hope it will.
CRISPR has the potential to be an extremely powerful tool and for that reason, there are many concerns about proper usage.
CRISPR could be the tool used to bring about cures to incurable diseases, and on the other hand, it could be the tool that causes great harm not only to individuals, but greater populations if used improperly. CRISPR is a controversial tool sparking much interest among researchers attempting to both develop the tool further and determine the risks associated with CRISPR. When discussing the way CRISPR would be able to be used when discussing the use of gene modification tools to eliminate undesirable traits. When placing a focus on the ethical implications of CRISPR, it becomes difficult, if not impossible, to avoid the topic of eugenics. First, this paper will explain the mechanics of CRISPR as a gene editing technique and then it will explore both the scientific and moral conversations surrounding it. In this paper, the development of CRISPR as well as the technical and ethical complexities
surrounding the controversial technique will be discussed via a review of literature written about.
Introduction
Scientists first began to explore the possibility of DNA manipulation in test tubes as recently as the late 1970s and the precise alteration of DNA in living eukaryotic cells came decades later. Scientists have been experimenting and pushing the limits of genetic manipulation since the possibility arised; however, the experiment that brought about the discovery of CRISPR was unrelated to gene manipulation. It began with a study being conducted on Escherichia Coli (E. coli) when an unusual repetitive DNA sequence was discovered in the genome. This sequence is now known as CRISPR.
Clustered Regularly Interspaced Palindromic Repeats
(CRISPR) refers to a segment of genetic material found in the genome of prokaryotes, such as bacteria and archaea. This genetic
segment is a short sequence of nucleotides repeated at regular intervals. Between these sequences are nucleotide sequences originating from pathogens that previously infected the organism; these sequences are one way the body protects against future reinfection (Ishino et al. 2018).
Prior to the discovery of CRISPR, there were several genes believed to encode DNA repair proteins in hyperthermophilic archaea (archaea living in high-temperature environments); scientists later identified these as Cas genes. Cas genes also function as nucleases associated with CRISPR systems. A nuclease is an enzyme that catalyzes the process of cleaving chains of nucleotides into smaller segments (Oxford Languages, n.d.). As opposed to these genes encoding repair proteins, the CRISPR system utilizes both CRISPR and RNA segments and the enzymes it produces to identify and modify certain DNA sequences in the genome of other organisms.
There are many techniques besides CRISPR, such as TALENs and XFNs, used to alter gene expression, but CRISPR is set apart for its flexibility and efficiency. CRISPR is a cost efficient gene editing tool with a simple design; its greatest disadvantage is the lack of existing knowledge surrounding CRISPR functioning so research continues. With the potential of high accuracy and efficiency functioning, it is sure to be a powerful technique (Liu et al. 2021).
There are various names for the many versions of this system each programmed to perform a different function and CRISPR-Cas9 is the most common version. Oftentimes, the different CRISPR systems are simply referred to by the umbrella term, CRISPR. CRISPR-Cas9 is one of many CRISPR systems that function by cutting and removing an undesirable stretch of DNA, as shown in Figure 1. The attached RNA sequence guides the CRISPR system to an identical target DNA sequence. The
endonuclease then binds to the DNA and cuts the bonds to shut off the targeted gene and inhibit its functioning.
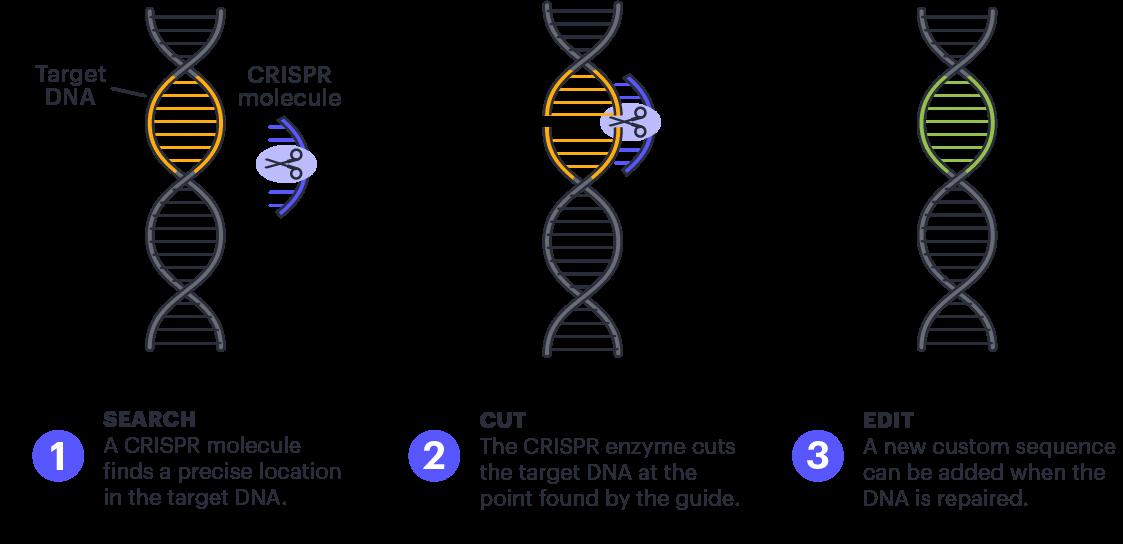
Figure 1 (What is CRISPR?, 2015). A diagram representation of one way in which a CRISPR system acts on DNA segments. Firstly, CRISPR molecules locate a segment in the target DNA which matches the segment of RNA guiding the CRISPR system. Secondly, the CRISPR associated enzyme cuts the target DNA at the target location. Lastly, the desirable DNA sequence is added and DNA is repaired.
Unlike other gene editing tools, CRISPR does not require a separate cleaving enzyme to cut DNA strands as the function is already integrated into the system though the Cas nuclease.
CRISPR associated nucleotides can also be modified in order to alter the function of the system. For example, the Cas9 nuclease cuts DNA, but can be modified so the system activates gene expression rather than deactivating it (Ishino et al. 2018).
CRISPR Cas systems have been categorized into two classes, Class 1 and Class 2, which are then subdivided into three types with varying subtypes. The different CRISPR systems are categorized into Class 1 or Class 2 based on the varying Cas enzymes associated with them. The specificity of each class and the respective subtypes are depicted in Figure 2. The main difference between the two classes is the amount of Cas proteins they employ. Class 1 CRISPR systems utilize multiple Cas proteins whereas class 2 systems utilize just one.
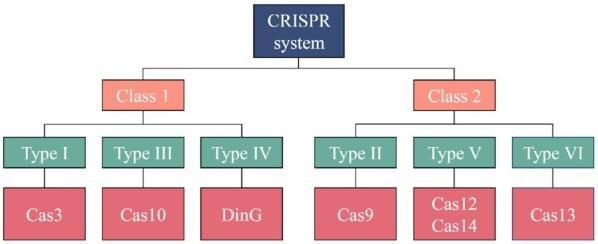
Figure 2. This chart represents the subdivisions of CRISPR at multiple levels of specificity.
Depicted on the chart are the two CRISPR classes, Class 1 and Class 2, and the three types within each class. The CRISPR types range from Type I to Type VI and each CRISPR endonuclease falls into one of the 6 types, a few of which are depicted above underneath their respective types.
Two of the most common CRISPR-Cas systems are CRISPR-Cas9 and CRISPR-Cas13. CRISPR-Cas9, the first and most researched nuclease, is able to perform gene insertion,
deletion, and base editing in DNA or RNA. It utilizes a base pair pattern in the guide RNA to recognize and edit matching sequences of target DNA. CRISPR-Cas9 also has the capability to perform base editing; base editing is a method of gene alteration that allows for precise point mutations in DNA/RNA by combining CRISPR components with other enzymes. However, there are a few limitations to the functionality of CRISPR-Cas9. One limitation being its inability to read long DNA sequences and another being the possibility of the system targeting a matching DNA sequence within an operon which would impact downstream gene expression.
In contrast to CRISPR-Cas9, the sole function of CRISPRCas13 is to edit base nucleotides within RNA. Six Cas13 protein families have been identified with the smallest being Cas13X and Cas13Y (775-803 amino acids). Their small size allows for easy application and versatile functioning. Cas-13X.1, a modified version of Cas13X, has advanced capabilities of mismatch
recognition as it is able to recognize single nucleotide mismatches and interfere with RNA sequences (Liu et al. 2021).
Limitations
Despite the mass amount of CRISPR-related research being conducted leading to an influx of new discoveries surrounding CRISPR functioning, CRISPR remains a largely new tool and there seems to be more questions than there are answers. The lack of a complete understanding of how genes function means that the implications of gene editing are not fully understood either. The looming possibility that gene editing is not as simple as we currently think it to be is real, as is the question of whether CRISPR technologies will work the way we hope since genes play an extremely complex role in the functioning of human beings (Ishino et a. 2018).
In addition to biological limitations, there are legal limitations slowing the progress of CRISPR. The first in vivo
clinical train was approved by the Food and Drug Administration in 2019 (Liu et al., 2021). and there are a number of clinical trials currently underway; however, there are extremely strict laws restricting the actions of the Administration in relation to CRISPR since the potential ramifications are yet to be fully understood. One restriction placed on the administration prohibits the evaluation of therapies involving the modification of human embryos; the major complications accompanying such experiments, namely a high probability of inaccuracy in CRISPR functioning, make them unjustifiable.
One of two components in CRISPR is the DNA cutting nuclease. Unfortunately, this nuclease can be rather unreliable. At times, the nuclease will act on the wrong stretches of DNA or the genetic repair equipment of the cell will scrabble adjacent nucleotides leading to accidental insertion or deletion of bases which has the potential to cause new harm. A group led by Kathy Niakan of the Crick reported that one study found that 16% of
human embryos altered by CRISPR suffered unintended editing outcomes at the targeted stretches of DNA (Alanis-Lobato et al., 2021). This problem becomes even more severe when factoring in the possibility for genetic screening of edited IVF embryos to miss these errors.
Off-target effects could have disastrous impacts on the human body. Though many factors make CRISPR a promising method of gene alteration, it is also accompanied by a higher likelihood of causing off-target effects. One example is the mismatching of chromosomes which would alter the genomic stability and the basic genomic functioning as a result. Another possibility is the misrecognition of shorter target sequences by the Cas protein, since it is a monomer, whereas dimeric gene editing systems don’t have the same likelihood of inaccuracy.
Whole Genome Sequencing (WGS) is a direct approach of evaluating mutations. WGS provides a useful option to measure the off target effects with a high frequency in clones and single
cells; however, the sensitivity of WGS is not high enough to detect off target effects in bulk populations. Alternatively, the high sensitivity of DISCOVER-Seq allows for the identification of off target effects with low false positive rates. DISCOVER-Seq is a method which locates MRE11, A DNA repair factor found at Cas9 double strand breaks, in order to detect off target effects (RS Zou et al., 2023). DISCOVER-Seq has been used in vivo to genetically alter the adenovirus CRISPR vector, which is a viral vector used to introduce Cas9 and specific gRNA sequences to various mammalian cell types. The target cell must simultaneously express Cas9 and the specific gRNA sequence in order to achieve successful CRISPR mediated gene alteration and the adenovirus makes this possible by expressing both within one vector (Liu et al. 2021). Post transmission, the adenovirus vector does not integrate itself into the genome of the host, but remains within the host as episomal DNA (Adenovirus CRISPR Vector, n.d.).
There is much that is known about CRISPR and so much that is not. The searches to find answers to the multitude of unknowns are only accompanied by another set of questions. For example, one difficulty facing CRISPR research is that of translating findings from animal studies to conclusions about humans. CRISPR, being the new and volatile field it is, is often tested on animal models. A common study modifies the stem cells which give rise to sperm cells in mice; the modification of sperm stem cells in mice has resulted in offspring with the desired changes in DNA, but there is nothing to say it will work the same way in humans. It is unclear how to maintain spermatogonial stem cells in culture the way that it is done for animals. Spermatogonial stem cells only survive when placed into newborn mice which is an unrealistic process for humans (Cohen, 2022). This is but one example of an obstacle in CRISPR research; however, similar difficulties are omnipresent.
Potential Use
A promising side of CRISPR research is the potential it has uncovered. CRISPR is such a wide unknown field, but there is so much that could be known and has begun to be explored. CRISPR has proven to be more than a tool to be used in labs; CRISPR can be the tool that cures currently untreatable diseases by correcting gene variants causing them. The correction of such gene pathologies would cure subsequent genetic diseases; the most pressing ones being inherited blood disorders, and eye diseases; genetic muscular, liver, and lung diseases; genetic deafness and neurological disorders; cancer. There are many studies that have proven the potential for CRISPR to be used as a treatment for the initiation or progression of many cancer types by applying varying CRISPR systems to target genes (Liu et al., 2021).
In 2018, the first ex vivo clinical trial using CRISPR edited cells was approved as a cancer treatment, the treatment uses the CRISPR-Cas9 system and focuses on cancer immunotherapy. The
first ex vivo clinical experiment was performed in West China hospital of China to treat metastatic non small-cell lung cancer. The study targeted the PD-1 gene in T cells of peripheral blood drawn out of patients; the electrophoration of sgRNA plasmid and Cas9 is used to target the cells. Afterwards, the peripheral blood is reinjected into the patients' bodies. However, while results have shown some level of therapeutic benefits, the results from this trial are yet to show high efficiency; additionally, the safety of this technique is not completely certain so further testing must be conducted (Liu et al. 2021)
2018 marked another major milestone in CRISPR research: the announcement of the first babies with CRISPR altered genomes. The babies are now nicknamed as the first “CRISPR babies”. The experiment was largely conducted underground by Jiankui He as it violated existing laws limiting embryonic genome editing in China. He’s goal in the experiment was to modify the genome of two embryos so as to create HIV immunity in the
babies prior to birth. Following the birth of the babies, Jiankui He posted a youtube video announcing the success of his experiment and detailed the way in which it was carried out (He, 2018). He describes the typical IVF process with one difference: gene alteration resulting from the introduction of protein and a guide sequence to the genome. He claimed that the surgery removed the entryway for HIV in their genomes. Following the surgery, He claims to have examined the results of the surgery through whole genome sequencing as well as ultrasounds and blood tests to determine that the mother’s pregnancy was normal; the only alteration was the gene altered to prevent HIV infection (He, 2018). Jiankui He served 3 years in prison following the experiment, prohibited from performing any further research, but the discussion it sparked among researchers was unrestrained.
Jiankui He claimed to use multiple methods to ensure and check the safety of the experiment, but there is still much left to question and doubt. He’s description of the process his lab
supposedly conducted to ensure the safety of the mothers and babies has aroused suspicion within the comments of the video he posted. The sensitivity of Whole Genome Sequencing is not perfect. Additionally, WGS is an extremely long process requiring copious amounts of information to properly evaluate mutations. It is difficult to believe that He used WGS to test for off target effects in any capacity, nevertheless full, which is concerning since WGS is still not perfect at full capacity. Many parts of this experiment were left to chance.
The pressing concern following CRISPR and, more broadly, gene alteration is the harm that it may result in, whether it be inadvertently or intentionally. The potential to treat currently incurable diseases is exciting, but how can effective regulations be placed on such powerful technology, and is there a way to impose such regulations without causing detriment to potential scientific discovery? With such a rapidly developing and growing topic in
science, it seems as though there are always more questions and problems to be accounted for.
One promising method to limit off target effects of CRISPR is the introduction of temporal control. This is achieved by inhibiting Cas enzymes themselves. Small molecule inhibitors are shown to have speedy reactions allowing for quick inhibition. Cas enzyme activity can be inhibited within minutes when protein based anti-CRISPR small molecule inhibitors are introduced; this provides the potential for precise temporal control.
CRISPR-Cas interaction sites are common on Acr proteins which function through various mechanisms like the inhibition of Cas binding with target DNA or prevention of enzymes cleaving target DNA sequences. These interaction sites allow Acr the ability to control Cas activity and result in improved targeting specificity. However, the introduction of Acr proteins is not without consequences as they have the potential to incite immune responses within the body (Liu et al., 2021).
In order to avoid the side effects that accompany Acr while maintaining the same capabilities, scientists have been researching alternative approaches to inhibit CRISPR-Cas systems, such as small nucleic acids. Nucleic acids are smaller than Acr proteins and have been proven easier to deliver in vivo; however, an inhibition technique without side effects is yet to be found. Small nucleic acids face the risk of degradation by cellular mechanisms, nucleases, renal clearance, and a myriad of other immune responses due to the body recognizing the acids as foreign (Liu et al., 2021).
Ethical Considerations
The possibility of genetic modification techniques being used to uphold eugenics being one of the greatest concerns. CRISPR must be regulated so that its use is limited to times of pure necessity. However, as time has proven, science is not often black and white. Not everything can be clearly categorized as
necessary or desirable. For example, one could argue that the experiment performed to grant HIV immunity through genetic modification of the DNA of babies was a necessary experiment.
On the other hand, it could be said that the experiment was simply feeding into the parents’ desire to have children biologically related to them, as there was an alternative option: not having biological children. Just as it is difficult to determine uses of CRISPR as necessary or not, it is hard to regulate the use of CRISPR. How can one stop doctors from simply breaking the law and continuing to experiment underground in attempts of further developing science or using the tool for their own gain? Chinese laws outlawing Jankui He’s experiment existed, the problem was a failure of the regulation of said laws.
What makes CRISPR the hopeful and exciting new tool it is is also what makes it so scary. The potential for change is not limited to positives; CRISPR can cause harm just as easily as it can good. If CRISPR is to work, what would stop people from
unnecessarily and preferentially altering genetics? But if CRISPR is to be heavily restricted, what can justify the potential for growth and good that is being restricted along with it?
Various attempts have been made to regulate illegal and unethical practices of gene editing. Shortly following the experiment which modified the genomes of babies to create HIV immunity, China’s highest policy making body, The Central Comprehensively Deepening Reforms Commission, led by the president, was authorized to establish a national committee led by an ethics advisory group to strengthen the implementation and coordination of a system of ethics governance for science and technology. The Association for Responsible Research and Innovation in Genome Editing (ARRIGE) is an organization established by a group of European scientists aiming to create policies enforcing the ethical use of gene editing tools (Liu et al., 2021).
As the regulation of CRISPR becomes a greater challenge, the potential for harm posed to children only grows. The safety of kids came second to the progression of science and reproductive desires of the parents. Sperm Washing is the process of removing sperm cells from semen. It is a legal alternative to CRISPR gene manipulation that prevents the transmission of HIV found through IVT. The way that sperm washing does this is that it prevents the transmission of things that may impair fertility, such as bacteria and hormones, from the semen. Embryos were altered without scientific validation of the safety of the technique as well as the possible off-target effects which posed a great risk to the embryos being tested on.
While many may pose concerns about the ways in which CRISPR usage may go too far, there are also concerns on the other side of the argument. Regulations may be implemented to stop the harm that accompanies irresponsible use of gene editing techniques, but what if these regulations get in the way of progress
and potential developments from research that will never be allowed to happen. Even with many countries having laws in place limiting the usage of CRISPR, criminal punishments often feel unfitting as crimes violating scientific regulations tend to involve much nuance. The right to research, along with the freedom of science and right to access its benefits, is one protected in the Universal Declaration of Human Rights which was signed by 169 countries.
Philosopher and Bioethicist Françoise Baylis writes of four identical potential benefits and detriments that may result from gene editing (Baylis, 83-87). The first is the Benefit to Prospective Parents. She describes one of the most compelling reasons to pursue genetic modification as being the opportunity it provides couples who would otherwise be unable to have genetically related, and healthy, children. The couples would be able to have children without any added burden, whether it be financial or emotional. Baylis continues to describe Benefits to Children as one
of the four categories, where the benefit is that of life as the child otherwise may not have been born as the parents would be conscious of the health risks posed.
However, this argument is rather weak. There are many promising aspects to CRISPR technology, namely its use as a treatment for living beings. It is difficult to justify the necessity of the use of CRISPR prebirth. The distinction between necessary and desirable modifications can be difficult to find, but there is no necessity in modifying embryos as they are yet to live; it would be a modification performed in order to improve the quality of life for the baby, but the option to not have a biological child also exists. This route eliminates the potential for off-target effects and incidental cause of further harm that accompanies CRISPR experimentation, making it the less safe and reasonable option.
While those arguments may not be the strongest, they can still be posed as viable benefits to come from CRISPR development. Alternatively, the following two categories were
more concerning with no true moral justifications. They were, Benefits to Society and Benefits to the Gene Pool. The benefits of CRISPR shifted from the improved health of individuals to the way it can strengthen society which is where the conversation of gene modification becomes riskier. This benefit to society was reduction of costs for health and social programs currently in place to assist people with disabilities as they would simply be prevented at birth. Baylis describes the way modification tools could be used to provide equity as the technology would be used to correct genetic disadvantages and potentially offer increased skills, like increased memorization and strength, for the sake of improving society as a whole. The benefits to the gene pool described seem good as the category begins by listing corrections of bad versions of a gene and introduction of so called good ones, but it then shifted to describe a use of CRISPR which would introduce additional good genes rather than simply replacement problematic genes: “Over time, as the generations reproduce, the hope would
be to increase the chances of having more genetically healthy, gifted children in the population” (Baylis, 87)
The word that sticks out here is gifted. CRISPR has equal promise for good as it does harm. The benefit of CRISPR to increase chances of eliminating genetic disease is difficult to argue, however the use of the word gifted implies the use of gene modification technologies in a way that goes beyond pure necessity and roams into the realm of desirability.
Conclusion
There is the argument that limitations on CRISPR due to fear of misuse will end up slowing scientific progress, but it is worth considering whether all scientific progress is good progress. Is the full development of CRISPR and other gene editing techniques something that should be pursued? CRISPR could be thoroughly researched and developed to the point where it could be used safely and precisely to alter the genome exactly as intended, but is this level of control one people should be able to achieve?
This becomes concerning when facing the fact of the matter: regardless of how many regulations are put in place and how strong the efforts to control ethical CRISPR usage are, there is no way to completely monitor the proper usage of CRISPR. The same Universal Declaration of Human Rights rights protecting people’s right to research would be the one allowing people access to an infinitely powerful tool. If people are able to modify genes to impact the capability of performing any skill, any trait, any action, where will it end and how can it?
Work Cited
Alanis-Lobato, G., Zohren, J., McCarthy, A., Fogarty, N. M., Kubikova, N., Hardman, E., Greco, M., Wells, D., Turner, J. M., & Niakan, K. K. (2021). Frequent loss of heterozygosity in CRISPRCas9–edited early human embryos. Proceedings of the National Academy of Sciences, 118(22).
https://doi.org/10.1073/pnas.2004832117
Baylis, F. (2019). Altered inheritance: CRISPR and the ethics of human genome editing. Harvard University Press.
Berkeley, UCSF. (2024, January 19). What is CRISPR?. Innovative Genomics Institute (IGI).
https://innovativegenomics.org/what-is-crispr/ Cohen, J. (2022, March 21). As creator of ‘CRISPR babies’ nears release from prison, where does embryo editing stand? Science. https://www.science.org/content/article/creator-crispr-babies-
nears-release-prison-where-does-embryo-editingstand#:~:text=He%27s%20largely%20secret%20use%20of,researc h%20on%20human%20embryo%20editing
He, J. [The He Lab]. (2018, November 25). About Lulu and Nana: Twin Girls Born Healthy After Gene Surgery As Single-Cell Embryos. YouTube.
https://www.youtube.com/watch?v=th0vnOmFltc
Ishino, Y., Krupovic, M., & Forterre, P. (2018). History of CRISPR-Cas from encounter with a mysterious repeated sequence to genome editing technology. Journal of Bacteriology, 200(7).
https://doi.org/10.1128/jb.00580-17
Liu, W., Li, L., Jiang, J., Wu, M., & Lin, P. (2021). Applications and challenges of CRISPR-cas gene-editing to disease treatment in clinics. Precision Clinical Medicine, 4(3), 179–191. https://doi.org/10.1093/pcmedi/pbab014
Oxford Languages. (n.d.). languages.oup.com dictionary. Retrieved April 11, 2024.
Zou, R. S., Liu, Y., Gaido, O. E. R., Konig, M. F., Mog, B. J., Shen, L. L., Aviles-Vazquez, F., Marin-Gonzalez, A., & Ha, T. (2023, April 6). Improving the sensitivity of in vivo CRISPR offtarget detection with discover-seq+. Nature News. https://www.nature.com/articles/s41592-023-01840-z
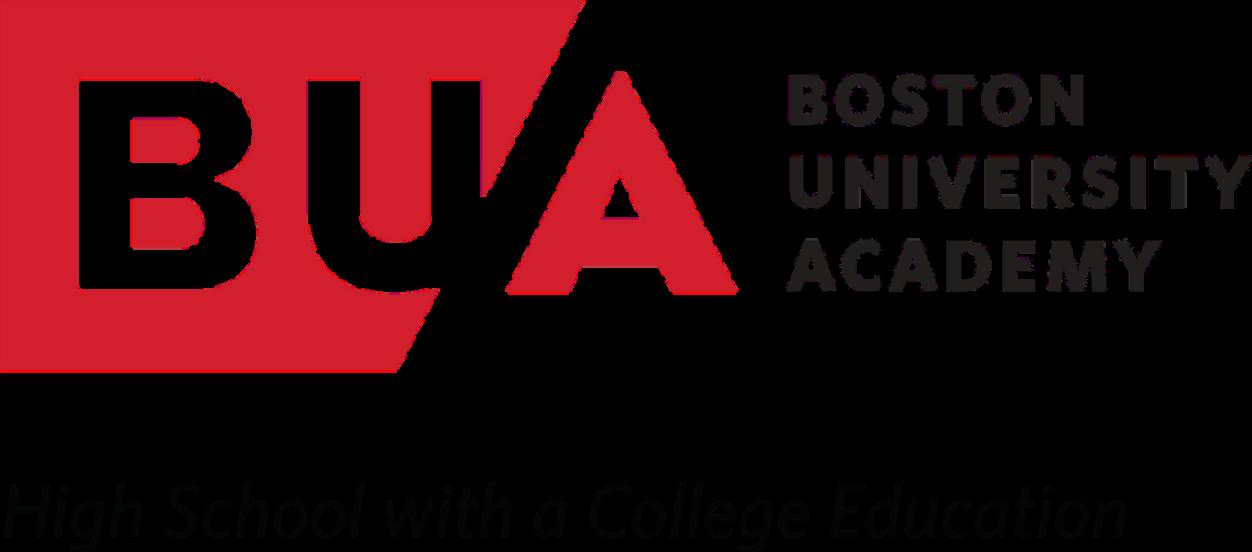