Sharvil Bijumalla
Senior Thesis | 2024
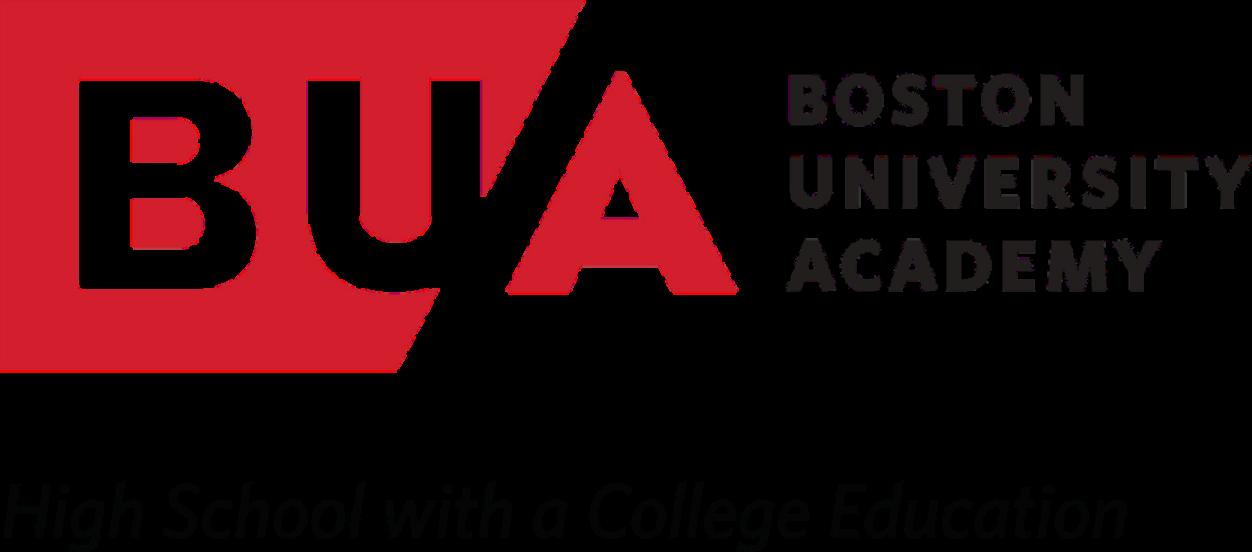
Neuroregenerative Applications of STEM Cell-Loaded Extracellular Vesicles in Biocompatible Hydrogels: Insights from Nonhuman Primate Models
Sharvil Bijumalla, Rosene Lab, BU Medical Center
Introduction:
Intracellular communication is vital to understand because it is the method through which the transfer of macromolecules such as proteins, lipids, and RNA strands transfer between cells is facilitated. In recent decades, EVs (Extracellular Vesicles) have gained popularity for their potential as minimally invasive biomarkers as well as their applications in clinical practice and various bioengineering fields. Biocompatible hydrogels (a mixture of porous, permeable solids and water) can be used as a medium to deliver EVs to damaged neural tissues because the hydrogel can act as a scaffold, providing structural support as well as a controlled environment for the release of EVs. Hydrogels are able to function in this manner because their structure is very similar to that of the extracellular matrix that surrounds the cells. This paper focuses on how EVs that contain STEM cells can repair the damage to lesions in the primary motor cortex.1 Nonhuman primates (NHPs) are crucial for testing the efficacy, safety, and mechanisms behind this therapeutic approach, because they offer a closer physiological and anatomical resemblance to humans compared to any other animal model. Because of this, nonhuman primates are essential to study and use in the lab-to-clinical pipeline. For this study, rhesus monkeys (Macaca mulatta) were used as the primary subject because their brain anatomy is more
1 Yamanaka, Shinya. “Pluripotent Stem Cell-Based Cell Therapy-Promise and Challenges.” Cell stem cell vol. 27,4 (2020): 523-531. doi:10.1016/j.stem.2020.09.014
similar to that found in humans than other nonhuman primates such as orangutans or chimpanzees. Monitoring the rhesus monkeys over an extended period of their time during their treatment provides insights when studying neural regeneration and recovery that would not be possible if other animal models were used.
STEM cells are cells that have not yet become fully developed. The least specialized type of STEM cells are pluripotent STEM cells, which are present in bone marrow, blood, and adipose tissue. Pluripotent STEM cells are capable of becoming any type of cell, and because of this versatility they are the most sought after in both lab and clinical settings. Pluripotent STEM cells eventually become multipotential STEM cells, which in the context of the nervous system, are only able to specialize into either neurons or glia (non-neuronal cells in the brain).
Multipotential STEM cells are primarily found in bone marrow, fat tissue, dental pulp, and heart tissue. In this study, multipotential STEM cells were used to repair lesions on the motor cortex. These cells were administered through EVs in a hydrogel solution. Through the administration of a complex cocktail of growth and transcription factors, the multipotential STEM cells injected into the primary motor cortex specialized into upper motor neurons. These upper motor neurons then formed synaptic connections with each other as well as preexisting cells through utilizing the actin and myosin proteins on their growth cones.2 Chemoattractant gradients as well as preexisting axons caused the actin to polymerize the growth cone and chemo-repulsive gradients in combination with empty space caused the myosin to retract the growth cone. This balance of polymerization and retraction ensures that the proper synaptic connections are formed. Working with
2 Yamanaka, Shinya. “Pluripotent Stem Cell-Based Cell Therapy-Promise and Challenges.” Cell stem cell vol. 27,4 (2020): 523-531. doi:10.1016/j.stem.2020.09.014
multipotential STEM cells can be very challenging, as multiple challenges arise at each step and the failure rate is high.3 There is always a chance that the STEM cells do not specialize to the correct type of upper motor neuron or that the desired synaptic connections are not formed. Additionally, it is possible for the host to reject the cell transplant altogether. When working with pluripotent STEM cells, there is an additional challenge of ensuring that the STEM cell specializes into the correct type of cell at a particular developmental stage. Despite these challenges, the clinical benefits of STEM cell therapy are immeasurable. Not only could they repair damage to the primary motor cortex induced by stroke or injury, but they also have the potential to repair spinal cord damage that would otherwise be permanent.4
Hydrogels, composed of porous, permeable solids and water, mimic the extracellular matrix surrounding cells. This similarity makes them ideal for delivering EVs to damaged neural tissues, providing both structural support and a controlled environment for EV release. The primary focus here is on EVs containing stem cells, particularly their role in repairing motor cortex lesions.5
3 Hall, S.L., Chen, S.-T., Gysin, R., Gridley, D.S., Mohan, S. and Lau, K.-.H.W. (2009), Stem cell antigen-1+ cell-based bone morphogenetic protein-4 gene transfer strategy in mice failed to promote endosteal bone formation. J. Gene Med., 11: 877-888.
4 Zhang, Jing et al. “Exosomes derived from bone marrow mesenchymal stromal cells promote remyelination and reduce neuroinflammation in the demyelinating central nervous system.” Experimental neurology vol. 347 (2022): 113895. doi:10.1016/j.expneurol.2021.113895
5 Blanke, Nathan, et al. "Quantitative birefringence microscopy for imaging the structural integrity of CNS myelin following circumscribed cortical injury in the rhesus monkey." Neurophotonics 8.1 (2021): 015010-015010.
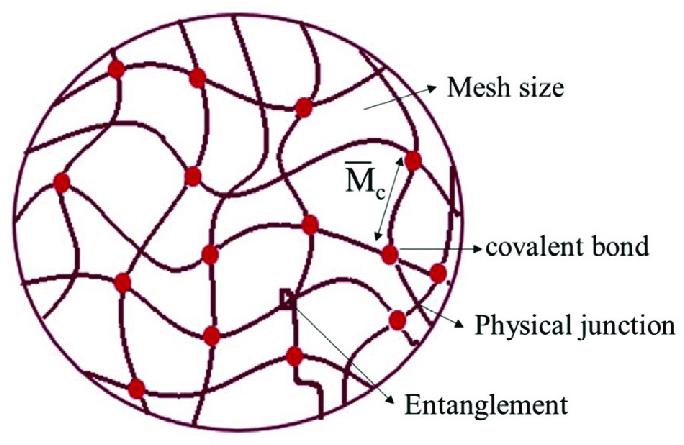
Figure 1. Structure of hydrogel at molecular level.
Nonhuman primates (NHPs), due to their close physiological and anatomical resemblance to humans, are crucial in translating laboratory findings to clinical applications. In this study, rhesus monkeys (Macaca mulatta) were chosen for their brain anatomy, which closely mirrors that of humans, more so than other NHPs like orangutans or chimpanzees. Monitoring these monkeys over extended periods provides invaluable insights into neural regeneration and recovery, insights unattainable with other animal models.
Until very recently, scientists primarily worked with only two varieties of STEM cells: embryonic and non-embryonic STEM cells. Scientists first discovered ways to derive embryonic STEM cells about thirty years ago by using mouse models. Less than a decade later, scientists were able to derive STEM cells from human embryos and culture the cells in a laboratory until they were ready to be used in clinical practice. These embryos were largely used for fertilization purposes, but in the modern-day applications of STEM cell therapy expand far beyond that. In 2006, there was another breakthrough that allowed some
specialized adult cells to be genetically reprogrammed and altered to assume a stem cell like state. These types of STEM cells are called induced pluripotent stem cells (iPSCs). The STEM cells used in this study (MSCs) are a specialized type of iPSC. STEM cells are important in living organisms for a multitude of reasons. In the embryo, STEM cells in the neural tube go on to make the entire body of the organism by specializing into cell types to be used in the heart, lungs, skis, and other organs. In some adult tissue such as bone marrow, STEM cells are present for the purpose of regenerating the body after injury. MSCs, for example, are derived from the bone marrow of adult male rhesus monkeys.6
Currently, STEM cell therapy can be used to combat all the following ailments: stroke, traumatic brain injury, learning deficits, Alzheimer’s disease, Parkinson’s disease, missing teeth, wound healing, bone marrow transplantation, spinal cord injury, osteoarthritis, rheumatoid arthritis, Crohn's disease, diabetes, muscular dystrophy, myocardial infarction, amyotrophic lateral sclerosis, deafness, blindness, and baldness. Our study is particularly interested in examining how STEM cells can repair the damage caused by strokes. Currently, there are three types of STEM cell therapies widely employed in clinical practice: adult STEM cells therapy, embryonic STEM cell therapy, and induced pluripotent STEM cell (IPSC) therapy. Adult STEM cell therapy is primarily used to treat blood disorders such as leukemia and lymphoma. Embryonic STEM cell (ESC) therapy is used to treat diabetes, spinal cord injury, muscular dystrophy, heart disease, and vision/hearing loss. Induced pluripotent STEM cell therapy is what we utilize in this study. IPSC therapy is used to treat many of the same conditions as ESC STEM cells, but IPSCs are preferable because they do not carry with them the ethical baggage that ESCs
6 Peters, A et al. “Neurobiological bases of age-related cognitive decline in the rhesus monkey.” Journal of neuropathology and experimental neurology vol. 55,8 (1996): 861-74. doi:10.1097/00005072-199608000-00001
carry, as ESCs usually have to be obtained from human Embryos. The following table from the University of Nebraska sums up the pros and cons of each type of STEM cell therapy effectively (Table 1).7
Adult Stem Cells Embryonic Stem Cells Induced Pluripotent Stem Cells
Benefits Trans differentiate and reprogramming of these cells is possible but is not well studied.
Thought to be less likely to be rejected if used in transplants.
Success has already been demonstrated in various clinical applications.
Can maintain and grow for 1 year or more in culture.
Established protocols for maintenance in culture.
ESCs are pluripotent cells that can generate most cell types.
By studying ESCs, more can be learned about the process of development.
Abundant somatic cells of donor can be used.
Issues of histocompatibility with donor/recipient transplants can be avoided.
Very useful for drug development and developmental studies.
Information learned from the “reprogramming” process may be transferable for in vivo therapies to reprogram damaged or diseased cells/tissues.
Drawbacks
Limitations on ASC ability to differentiate are still uncertain; currently thought to be multi or unipotent.
Process to generate ESC lines is inefficient.
Unsure whether they would be rejected if used in transplants.
Therapies using ESC avenues are largely
Methods for ensured reproducibility and maintenance, as differentiated tissues are not certain.
Viruses are currently used to introduce embryonic genes and has been shown to
7 University of Nebraska Medical Center. (n.d.). Stem Cells at UNMC. Retrieved [insert the date you accessed the website], from https://www.unmc.edu/stemcells/stemcells/unmc.html
Cannot be grown for long periods of time in culture.
Usually, a very small number in each tissue making them difficult to find and purify.
Currently there is no technology available to generate large quantities of stem cells in culture.
Ethical Concerns No major ethical concerns have been raised
new and much more research and testing is needed
If used directly from the ESC undifferentiated culture prep for tissue transplants, they can cause tumors (teratomas) or cancer development
cause cancers in mouse studies.
To acquire the inner cell mass the embryo is destroyed.
Risk to female donors being consented.
iPS cells have the potential to become embryos if exposed to the right conditions.
Table 1: As shown in this table, no type of STEM cell therapy is perfect; each therapeutic approach has benefits and drawbacks to consider. Therefore, the approach of using different therapies to treat different ailments is ideal.
The goal of this study was to see if MSCs could repair myelin damage and reduce inflammation in the brain in animal models. We used a chemical agent called MOG35-55 to induce consistent and long-lasting damage to the central nervous system of our animal model. The effects of this lesion are similar to the symptoms found in patients with multiple sclerosis (MS) or loss of motor function as result of a stroke. By using this method, we aimed to see if these exosomes/extracellular vesicles containing MSCs could accomplish the following three goals: improving the function of the nervous system, repairing the damaged myelin, and
controlling the inflammation of the brain and spinal cord. We will discuss the potential clinical implications of this technique in a future section.8
Materials
and Methods:
The type of STEM cells used in this study are called Mesenchymal Stromal Cells (MSCs), which are harvested from the bone marrow of a young adult male rhesus monkey to be cultured and grown. First, the cells were placed in a T75 flask along with a chemical agent. Next, to grow even more cells, the preexisting mix was inserted into a quantum incubator. We separated the exosomes from the cells by spinning the liquid in a centrifuge, where the exosomes became separated into a bottom layer. Spinning the fluid in the centrifuge also helped to filter out impurities. The size of these exosomes was studied using electron microscopy, and we used western blotting to identify specific proteins (CD63 and Alix) which served as markers for these exosomes. Before conducting the experiments with monkeys, we first wanted to ensure that the CD63 marker protein could cross the blood brain barrier and would thereby be detectable immunohistochemical staining. We injected these proteins into the mice bloodstream via viral transmission. After four hours, the mice were perfused, and their brain tissue was sliced and put on slides. Using electron microscopy, we confirmed that the exosomes had indeed crossed the blood brain barrier and that this technique would be viable.9
8 Peters, A et al. “Neurobiological bases of age-related cognitive decline in the rhesus monkey.” Journal of neuropathology and experimental neurology vol. 55,8 (1996): 861-74. doi:10.1097/00005072-199608000-00001
9 Moirano, Jeffrey M et al. “Rhesus Macaque Brain Atlas Regions Aligned to an MRI Template.” Neuroinformatics vol. 17,2 (2019): 295-306. doi:10.1007/s12021-018-9400-2
The first step we had to complete in beginning the treatment of these lesions was preparing for immunization. We created an emulsion primarily composed of H37Ra, a bacterial strain. Each monkey was immunized with this emulsion, which was injected into two separate sites on their lower backs. After this immunization, our monkeys were separated into three separate groups, one to receive MSCs, one to receive a control exosome (EXO-con), and the last to receive a saline solution. Each of the monkeys in these groups received these injections in timed and controlled doses. In addition to the solutions mentioned earlier, these monkeys were also injected with Bromodeoxyuridine (BrdU) to trace cell division. We monitored all of the monkeys for thirtyfive days following immunization. The severity of their conditions was rated on a scale 0 to 5, with 0 indicating no disease or symptoms and 5 indicating severe disease or death. We monitored tail tone, hind-limb weakness, hind-limb paralysis, and other symptoms.
The first step of obtaining post-mortem slices of brain tissue is to put the monkey under deep anesthesia and using a small portable monitor to keep track of vitals such as heart rate, respiratory rate, and depth of anesthesia. Next, we ensured the perfusion tank was completely sterile, before preparing the perfusion pump and tubing. We also had to prepare a KREBS solution that would be used to clear out all the blood surrounding the brain as well as a Paraformaldehyde solution to fix the tissue, thereby halting any and all cellular processes. Right before the beginning of the surgery, we collected blood samples for analysis. An incision was made to expose the heart and insert a cannula into the ascending aorta. The right atrium was opened to allow blood to exit. Next, a brief flush of saline was used to clear blood from the vessels. Then we switched to the KREBS solution that helped to further remove blood while still preserving tissue integrity. Paraformaldehyde was used next to fix the tissue to a desired
amount and to halt cellular processes. Now that the monkey’s blood had been removed, brain extraction could begin. A cut was made in the back of the monkey’s brain, and then all layers of the meninges were removed (skin, bone, dura mater, arachnid mater, pia mater). Finally, the brain was extracted from the host and placed in a paraformaldehyde solution to fix and preserve the tissue. The brain was then moved to a refrigeration room, where it would be housed until it was time for tissue slicing.10
The first step in fixing the tissue was to soak it in paraformaldehyde for 24 to 48 hours. After fixation, the brains were cut into tissue slices that were merely 6 micrometers thick. We then used double immunofluorescence staining, which is a complex technique where two different antigens are used to tag two different antibodies with different fluorescent dyes. The antibodies we used included APC (an oligodendrocyte marker), IBA1 (a microglia marker), APP (an amyloid precursor protein marker) and BrdU (tracks cell proliferation). Using a color video microscope, we used a 40x zoom to count the number of cells reacting to antibodies and measure the area reacting to these cells. Finally, we used Transmission Electron Microscopy (TEM) to conduct a final examination of tissue sections. By using TEM, we were able to examine the ultrastructure (detailed cell structure) of myelin and axons in the tissue slices of these monkeys.11
10 Otsuji, Tsuyoshi et al. “Immunohistochemical staining and morphometric analysis of the monkey choroidal vasculature.” Experimental eye research vol. 75,2 (2002): 201-8. doi:10.1006/exer.2002.2020
11 Otsuji, Tsuyoshi et al. “Immunohistochemical staining and morphometric analysis of the monkey choroidal vasculature.” Experimental eye research vol. 75,2 (2002): 201-8. doi:10.1006/exer.2002.2020
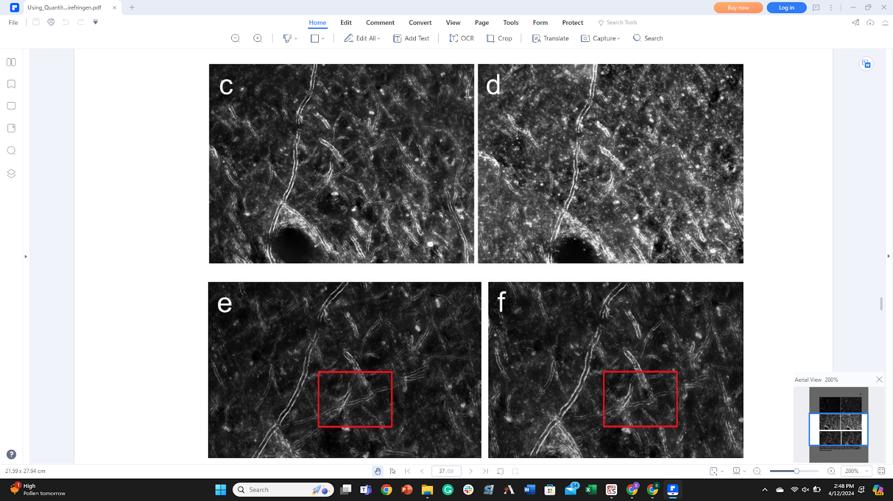
Figure 1. Day 1 (c) vs day 4 (b). Comparison of TDE coverslipping. Vesicles still appear and myelin has appreciably degraded. Additionally, tissue cover-slipped with glycerol (e) provides a better RI match and results in less myelin breakdown than TDE cover-slipped tissue (f). Red boxes indicate visible differences. Different brightness levels between tissues are the result of differing exposure time (user-dependent).
Results and Discussion:
We found in this study that the MSCs exhibited the ability to differentiate into upper motor neurons, a critical step in addressing motor cortex injuries or diseases. The formation of
12 Robinson, Rhiannon. "Using Quantitative Birefringence Microscopy to Identify Myelin in the Aging Primate Brain." Order No. 29065803 Boston University, 2022. United States Massachusetts: ProQuest. Web. 12 Apr. 2024
synaptic connections by these neurons is essential for restoring neural pathways and motor functions. This finding is not just a testament to the potential of MSCs in neural regeneration, but it also highlights the effectiveness of EVs and hydrogel as a delivery and support system. This approach could revolutionize cellular therapies by providing a more efficient and targeted delivery mechanism, ensuring that the cells reach the desired location in the brain and stay functional. Myelin sheaths, essential for the proper functioning of the nervous system, are often damaged in neurological disorders like multiple sclerosis (MS) and certain types of strokes. The MSCs facilitated myelin regeneration, suggesting a potential role in treating demyelinating conditions.
Additionally, we observed a reduction in inflammation markers in the MSC group compared to the control groups. This is crucial, as inflammation is a key factor in the progression of neurological disorders, often worsening symptoms and causing further damage. The MSC treatment led to improved motor functions and reduced severity of symptoms in the treated monkeys. Behavioral assessments, supported by lower scores on the disease severity scale, indicated a large improvement in the subjects' quality of life and functional abilities. The successful integration of MSCs into neural tissue, with minimal signs of immune rejection or adverse reactions, is a crucial finding for the potential clinical application of MSC therapy.
The implications of our study in the field of regenerative medicine, particularly for treating neurological disorders and spinal cord injuries, are profound. The ability of MSCs to differentiate into specific neural cell types and integrate into existing neural networks could revolutionize the treatment of conditions previously considered untreatable. This includes spinal cord injuries, which often result in paralysis or significant loss of function. The regenerative capacity of MSCs could transform the treatment landscape for a wide range of neurological conditions,
offering new hope to patients who currently have limited treatment options.
Our findings, while promising, present several challenges that need to be addressed in future research. Ensuring the precise differentiation and integration of MSCs into complex neural networks is a significant challenge. The risk of immune rejection, although minimal in this study, remains a concern in stem cell therapy. Additionally, the potential for uncontrolled cell growth leading to tumors is a critical safety concern. Advances in genetic engineering, immunomodulation, and cell culturing techniques are essential to address these challenges and improve the safety and efficacy of stem cell therapies. The successful application of MSC therapy in a clinical setting would require rigorous clinical trials to establish safety and efficacy in human subjects. Additionally, the development of standardized protocols for stem cell cultivation, differentiation, and delivery is crucial for the widespread adoption of this therapy.
The utilization of MSCs in the treatment of neurological disorders and spinal cord injuries represents a significant advancement in medical science. The study's findings highlight the potential of stem cells in regenerative medicine, offering hope for conditions previously deemed untreatable. However, the path from laboratory research to clinical application is complex and requires meticulous attention to detail and collaboration across multiple disciplines. The future of MSC therapy in neurological repair is promising, and this study lays a solid foundation for further advancements in this exciting field.13
The ethical considerations surrounding the use of MSCs, particularly those derived from primates, are an integral part of this research. The study adhered to strict ethical guidelines, ensuring
13 Peters, A et al. “Neurobiological bases of age-related cognitive decline in the rhesus monkey.” Journal of neuropathology and experimental neurology vol. 55,8 (1996): 861-74. doi:10.1097/00005072-199608000-00001
the welfare of the subjects, and minimizing discomfort. The use of non-human primates, while essential for the translational aspect of this research, raises important ethical questions that must be continually addressed as the field progresses. Looking forward, the potential of MSCs extends beyond the treatment of neurological disorders and spinal cord injuries. Their application could be explored in other areas of regenerative medicine, such as cardiac repair, diabetes, and even in the treatment of certain cancers. The versatility of MSCs, coupled with advancements in biotechnology, opens up a myriad of possibilities for future medical treatments. In summary, this study not only sheds light on the potential of MSCs in neural repair but also sets the stage for a broader exploration of stem cell therapy in various medical fields. The journey from laboratory research to clinical application is complex and challenging, but the potential rewards for humanity are immense. As we continue to unravel the mysteries of stem cells and their capabilities, we edge closer to a new era in medical science, one where regeneration and repair become a tangible reality for many debilitating conditions.
Lastly, it is worth discussing that STEM cells are not necessarily the only method of treating a stroke or many of the other pathologies previously mentioned. Traditional methods of rehabilitation following a stroke have shown promising results for some and have been a tried-and-true method for decades. Research has shown that people who participate in focused rehabilitation programs perform better than those who do not go through said program. Components of a rehabilitation program can include motor-skill exercises, mobility training, constraint-induced therapy, range of motion therapy, functional electrical stimulation, robotic technology, wireless technology, virtual reality, therapy for cognitive disorders, therapy for communication disorders, psychological evaluation and treatment, medication, noninvasive brain stimulation, biological therapies, and alternative medicine.
Motor-skill exercises can help improve muscle strength and coordination, which can help with balance, walking and swallowing. Mobility training with the use of mobility aids such as canes or a wheelchair can help patients relearn how to walk. Constraint-induced therapy works by restraining certain limbs to work on the movement of other limbs. Range of motion therapy helps to ease muscle tension and help regain range of motion. Functional electrical stimulation works via using electrical currents to restimulate muscles and get them moving again. Robotic technology provides extra strength and support to affected limbs. Therapy for cognitive disorders helps with speech restoration, memory improvements, language processing, problem-solving, social skills, judgment, and safety awareness. Therapy for communication disorders can help regain speaking, listening, writing, and comprehension abilities. Psychological treatment can help with emotional regulation. Medication can help with increasing alertness. Noninvasive brain stimulation can help with improving motor skills.
STEM cells are a biological therapy that is currently used to treat strokes only as part of a clinical trial. As seen here, nearly all the negative effects of a stroke can be remedied or at least made better through means besides STEM cells, however, STEM cells are the most direct and effective treatment currently on the market. It is also worth mentioning that besides STEM cell therapy, the second-best way of treating a stroke is with Deep Brain Stimulation. Deep Brain Stimulation involves putting electrodes in different areas of the brain that then fire electrical impulses to affect and bolster brain activity. This technique affects the brain at the subcellular level. Deep brain stimulation is an established treatment for people with movement conditions. In one particular study, twelve individuals with severe upper extremity impairment following stroke were enrolled to receive DBS to an area within the cerebellum, (the area of the brain responsible for coordinating
movements) called the dentate nucleus. Each participant received physical therapy before, during, and after deep brain stimulation was administered, which was administered for between four and eight months depending on the patient. The goal of this study was to determine whether DBS in combination with physical therapy is a safe and effective treatment for stroke victims. No adverse events or failures were reported during the trail. Their encouraging results support the design of larger trials. Nine out of the total 12 patients had significant improvements in motor function following DBS plus physical therapy. These improvements were present even after DBS treatment concluded. The study’s secondary findings were also promising, with nine out of twelve participants showing improvements in motor function following deep brain stimulation in combination with physical therapy. One noteworthy thing is that not all patients in this study showed the same degree of improvement. Patients that retained a decent level of motor activity showed significant improvement. On the other hand, participants with a very low level of motor function showed relatively minimal benefit. All who had some measure of control showed improvement over the course of treatment.14
To briefly summarize the contents of this work, a type of STEM cell called an MSC was able to produce significant benefits in repairing myelin damage in Rhesus monkeys with lesions in the motor cortex of their brain. The findings of this study have clinical implications for patients with strokes who are suffering with motor function. While STEM cell therapy is not the end all be all, it is the most directly effective treatment of many pathologies. There are also ethical concerns to consider, but IPSCs reduce that element. STEM cells will undoubtedly be a massive aspect of regenerative medicine going forward, and the goal will become to optimize this
14 University of Nebraska Medical Center. (n.d.). Stem Cells at UNMC. Retrieved [insert the date you accessed the website], from https://www.unmc.edu/stemcells/stemcells/unmc.html
care to become as widely effective and efficient as it possibly can be. That is why clinical trials like the one discussed in this paper are so invaluable.
1. University of Nebraska Medical Center. (n.d.). Stem Cells at UNMC. Retrieved [insert the date you accessed the website], from https://www.unmc.edu/stemcells/stemcells/unmc.html
2. Blanke, Nathan, et al. "Quantitative birefringence microscopy for imaging the structural integrity of CNS myelin following circumscribed cortical injury in the rhesus monkey." Neurophotonics 8.1 (2021): 015010-015010.
3. Zhang, Jing et al. “Exosomes derived from bone marrow mesenchymal stromal cells promote remyelination and reduce neuroinflammation in the demyelinating central nervous system.” Experimental neurology vol. 347 (2022): 113895. doi:10.1016/j.expneurol.2021.113895
4. Moirano, Jeffrey M et al. “Rhesus Macaque Brain Atlas Regions Aligned to an MRI Template.” Neuroinformatics vol. 17,2 (2019): 295-306. doi:10.1007/s12021-018-9400-2
5. Peters, A et al. “Neurobiological bases of age-related cognitive decline in the rhesus monkey.” Journal of neuropathology and experimental neurology vol. 55,8 (1996): 861-74. doi:10.1097/00005072-199608000-00001
6. Dong, Jin-Run et al. Dong wu xue yan jiu Zoological research vol. 33,1 (2012): 43-8. doi:10.3724/SP.J.1141.2012.01043
7. Otsuji, Tsuyoshi et al. “Immunohistochemical staining and morphometric analysis of the monkey choroidal vasculature.” Experimental eye research vol. 75,2 (2002): 201-8. doi:10.1006/exer.2002.2020
8. Jainkittivong, A, and R P Langlais. “Herpes B virus infection.” Oral surgery, oral medicine, oral pathology, oral radiology, and endodontics vol. 85,4 (1998): 399-403. doi:10.1016/s10792104(98)90064-6
9. Strausz, János. “Tuberkulózis 2006” [Tuberculosis 2006]. Orvosi hetilap vol. 148,18 (2007): 829-31. doi:10.1556/OH.2007.28057
10. Yamanaka, Shinya. “Pluripotent Stem Cell-Based Cell Therapy-Promise and Challenges.” Cell stem cell vol. 27,4 (2020): 523-531. doi:10.1016/j.stem.2020.09.014
11. Eckert, Matthew J, and Matthew J Martin. “Trauma: Spinal Cord Injury.” The Surgical clinics of North America vol. 97,5 (2017): 1031-1045. doi:10.1016/j.suc.2017.06.008
12. Morecraft, R J, and G W Van Hoesen. “Convergence of limbic input to the cingulate motor cortex in the rhesus monkey.” Brain research bulletin vol. 45,2 (1998): 209-32. doi:10.1016/s0361-9230(97)00344-4
13. Fiave, Prosper A, and Koen Nelissen. “Motor resonance in monkey parietal and premotor cortex during action observation: Influence of viewing perspective and effector identity.” NeuroImage vol. 224 (2021): 117398. doi:10.1016/j.neuroimage.2020.117398
14. Hall, S.L., Chen, S.-T., Gysin, R., Gridley, D.S., Mohan, S. and Lau, K.-.-H.W. (2009), Stem cell antigen-1+ cell-based bone morphogenetic protein-4 gene transfer strategy in mice failed to promote endosteal bone formation. J. Gene Med., 11: 877888. https://doi-org.ezproxy.bu.edu/10.1002/jgm.1369
15. Commercial hydrogels for biomedical applications - Scientific Figure on ResearchGate. Available from: https://www.researchgate.net/figure/Structure-of-hydrogel-atmolecular-level_fig1_340479680 [accessed 4 Apr, 2024]
16. Robinson, Rhiannon. "Using Quantitative Birefringence Microscopy to Identify Myelin in the Aging Primate Brain." Order No. 29065803 Boston University, 2022. United States -Massachusetts: ProQuest. Web. 12 Apr. 2024.a
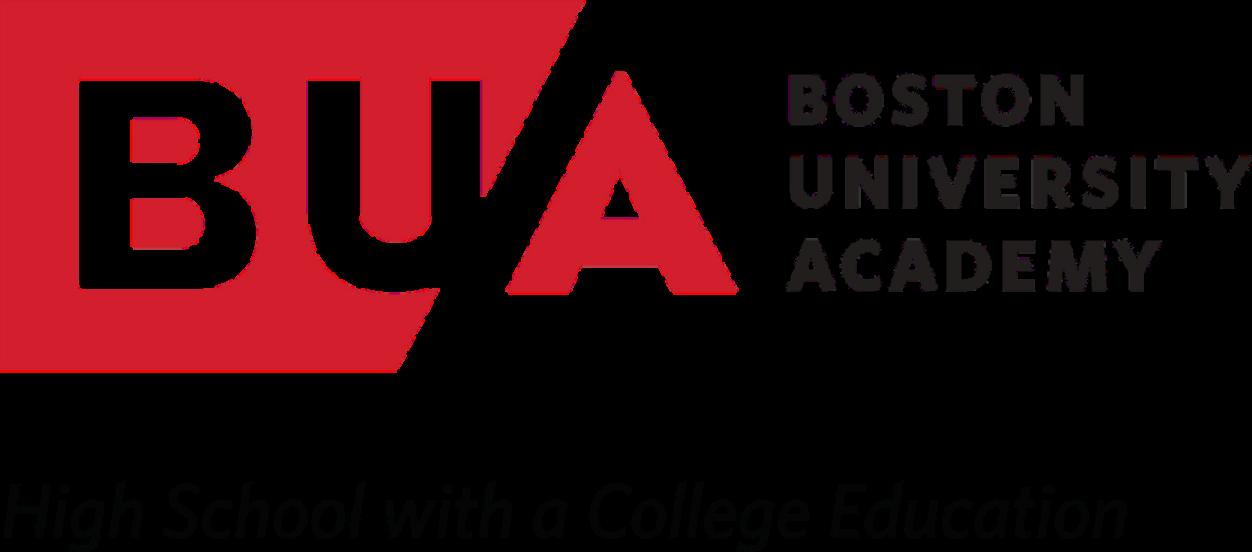