Ancient Greek Cosmology
The seeds of Cosmology as it is understood today began in Ancient Greece. In fact, the word cosmology itself is Greek in origin from κοσμος meaning universe, adopted into English as cosmos, and of course λογος meaning discourse or discussion.1 Thus it is fitting that the Ancient Greek theories of the universe are the starting point for this evaluation of cosmology’s evolution.
The Ptolemaic Model
In roughly 150 CE, Greek astronomer and philosopher
Ptolemy crafted a highly accurate model of the universe on the largest scale imaginable at the time, the Solar System. The model itself was geocentric, as the Greeks could not justify a heliocentric universe for their inability to detect any parallax in the stars. Parallax is the slight shift in the position of all background objects observed from any body in orbit around another body. The Greeks
1 "Cosmology," Online Etymology Dictionary, accessed April 12, 2024.
knew that if the Earth were in orbit around the sun, then there should be detected parallax in all the stars that make up the night sky between the Earth’s perihelion (the point closest to the sun) and aphelion (the point furthest from the sun). What the Greeks had not yet understood is the inversely proportional relationship between parallax and distance, which makes the detected parallax smaller and smaller for increasing distances. This misunderstanding, coupled with the fact that the stars they were seeing were believed to be orders of magnitude closer than they actually were, lead them to the conclusion that the Earth could not be in orbit around the sun.
This led Ptolemy to craft a visual representation of the Earth in relation to the other known objects nearby. Meticulous observation and motion tracking allowed him to gather an understanding of where objects were in relation to each other and the Earth, and he mapped his interpretation of the heavens as concentric circular orbits around the Earth (Figure 1).
Figure 1. A visual representation of the Ptolemaic Model.2
Still, the geocentric model presented a challenge in explaining retrograde motion. The term that refers to the observed brief reversal of orbital direction for planets close to the Earth. The Copernican revolution, which solidified heliocentricity, provides a
2 "Medical Astrology," Yale University Library Online Exhibits, accessed April 12, 2024.
simple understanding that Earth’s orbit effectively laps the orbits of the other planets nearby, so from the observer’s point of view on Earth it looks like the other planet is traveling backwards, when in reality Earth has just surged past the other planet. However, this cannot be the logical conclusion if Earth is understood as the center of said orbits. And so, Ptolemy adapted his model to include a far more complicated explanation of retrograde motion. He set each planet on a deferent orbit with attached epicycles (Figure 2). Each planet would stick to its regular orbit (the deferent) and periodically move off into an adjoined smaller circle (the epicycle), then complete a full rotation around it before returning to the deferent orbit. Despite the intricate complexities and blatant inaccuracy that existed within the Ptolemaic model, it was able to accurately predict the motion of the bodies contained within it for upwards of three centuries after its creation.
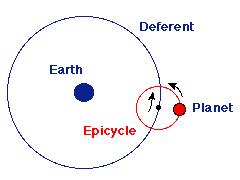
Figure 2. A graphical representation of the Epicycle and Deferent Model.3
The
Antikythera Mechanism
The most advanced implementation of these theoretical advancements at the time was the Antikythera mechanism. The Antikythera mechanism was a hand-powered orrery created in 3 "Ptolemy's Cosmology," University of Oregon, accessed April 12, 2024.
roughly the 2nd century BCE and can be understood as one of the first computers used to predict astronomical movement. The mechanism itself was a computer composed of bronze gears that utilized cutting edge astronomy of the age and adapted theories and cycles into mechanisms in order to make predictions of stellar and planetary movement (Figures 3 and 4).4 Similar to Ptolemy’s model of the cosmos, its primary function was to track and predict the future motions of what were then referred to as the “heavenly bodies.” Surviving remnants of the mechanism itself have led astronomers, historians, classicists, and archeologists to collaborate in an accurate reconstruction of its former state to better understand the depth of its precision.
4 Guo, Y., Lee, J., Kim, J., & Nam, Y. (2021). Deep reinforcement learning based elevator group control. Scientific Reports, 11(1), 1-12.
Figure 3. Diagram and output model for nodes and gears on the Antikythera mechanism.5
5 Freeth, T., et al. "Decoding the Ancient Greek Astronomical Calculator Known as the Antikythera Mechanism." Nature 444.7119 (2006): 587-91.
Figure 4. Bronze gear models of individual controls on the Antikythera mechanism.6
6 Freeth, T., et al. "Decoding the Ancient Greek Astronomical Calculator Known as the Antikythera Mechanism." Nature 444.7119 (2006): 587-91.
At large, the Antikythera mechanism integrated the cycles and models of Babylonian astronomy as well as the complexity of Greek geometry7 in order to make its predictions. The mechanism relied on an in-depth understanding of the period relations between the objects that it tracked, including the epicyclic explanation of not only nearby planets, but also both the Sun and moon. Given that the orbits were modeled on extensive observational data points, they remained quite accurate even though the theories that the Antikythera mechanism brought to life were flawed in their epicyclic basis. This being the case for all objects that the mechanism tracked seeing that all variable motion in Ancient Greece was modeled through either epicyclic theory or equivalent eccentric variants, which applied to the Sun and Moon as well.8 So, in reconstructing the physical structure, a more complete
7 Guo, Y., Lee, J., Kim, J., & Nam, Y. (2021). Deep reinforcement learning based elevator group control. Scientific Reports, 11(1), 1-12.
8 Guo, Y., Lee, J., Kim, J., & Nam, Y. (2021).
understanding of the theory and mathematical relationships that powered planetary motion has resurfaced as a result.
The physical structure of the Antikythera mechanism has been almost entirely pieced together through the reconstructive process taken on in a 2006 study by Freeth et. al (Figure 5). The complex network of interconnected gears and dials each serve multiple functions for indicating and predicting the stellar and planetary movements in consideration. In this study specifically, some gear fragments have been incorporated that previously could not be connected to the entire model. Their model incorporates “the dual use of the large gear, e3, at the back of the mechanism" as well as a fixed-axis train that “turns the Saros and Exeligmos dials for eclipse prediction, and also doubles as the ‘epicyclic table’ for the gears k1, k2.”9 Piecing together the mechanism to the point where it very nearly resembles its original construction
9 Freeth, T., et al. "Decoding the Ancient Greek Astronomical Calculator Known as the Antikythera Mechanism." Nature 444.7119 (2006): 587-91.
displays much more than the impressive physical engineering that went into its creation in antiquity. By understanding the intention and practical implementation of gears and pieces that serve multiple functions within the mechanism, additional conclusions can be drawn about the correlation and overlap of the observational purposes that they represent within the mechanism. We can improve eclipse prediction by considering the gear mentioned above as well as maintaining the epicyclic motions for the tracked objects. Knowing that both of those roles are played by the same piece of equipment can spark conversations about the possibility of some overlap in the Greek understanding of eclipses and orbits.
Figure 5. Face-on model of the reconstructed Antikythera mechanism gear complex.10
Kepler’s Laws
As a somewhat intermediary point, Kepler’s laws of orbital motion provide a perfect transition from the classical cosmology into modern cosmology. Much like Ptolemy’s pursuit of modeling the cosmos, Kepler aimed to bring a more in depth understanding of orbital motion. This advancement completely redrew the 10 Freeth, T., et al.
framework of tracking objects in orbit, leading to questions such as, “How can we go from circular epicycles and deferents to the elliptical orbits of the planets or from the equant point to Kepler's second law?” which were vital to understand.11 Despite their lack of elegance in contrast with Ptolemy’s circular orbital construction of the solar system, Kepler’s laws introduce the accurate elliptical orbits for not only all of the planets within the solar system but also additional objects such as comets, dwarf planets, and more. Kepler’s first law states all planets in the solar system move in elliptical orbits with the Sun as a focus of the orbit. Kepler’s second law states that an equal amount of space is swept out for any same amount of time, no matter where in its orbit the planet is. The third law states that a planet’s orbital period, p, is directly
11 Carman, Christián C., and Gonzalo L. Recio. “Ptolemaic Planetary Models and Kepler’s Laws.” Archive for History of Exact Sciences 73, no. 1 (2019): 39–124.
proportional to how large its orbit is (the semi-major axis of the ellipse, a), which can be generalized into the equation:12
p2 = a3
Two of these three laws show direct improvements on the Ptolemaic understanding. For example, the first law maps out ellipses across the solar system with the Sun as a focus of each one, opposing Ptolemy’s concentric circles around the Earth. And the third law reveals that each Planet’s orbital period is proportional to the size of its orbit (the semi-major axis of the ellipse) rather than maintaining an orbit based on the given epicycles for that planet and its neighbors.
The advancement of Keplarian cosmology, while rendering the Ptolemaic model untrue, certainly does not leave the continued studying of Ptolemaic cosmology obsolete. Rather than forgoing additional unpacking of Ptolemy’s contributions, cosmologists
12 "Orbits and Kepler's Laws." NASA Science, NASA. Accessed April 12, 2024.
expect their work to advance the understanding of “not only of the Ptolemaic theories for planetary longitudes through a clearer perception of the way in which Keplerian features are present - or absent - in Ptolemy's models, but also for a more balanced judgment of different aspects of the contribution of the first two laws of Kepler to the modern astronomical revolution.”13 At large, strengthening the transition from classical to contemporary cosmology such that there is less of a stark divide and more adaptation of certain views from the former into the latter.
The Accelerating Universe
To understand observation on a cosmological level, it is crucial to understand its clear correlation with perspective. Of course, observation is heavily influenced, if not entirely characterized, by the perspective(s) from which it partakes. In
13 Carman, Christián C., and Gonzalo L. Recio. “Ptolemaic Planetary Models and Kepler’s Laws.” Archive for History of Exact Sciences 73, no. 1 (2019): 39–124.
cosmology these perspectives are understood not only in the context of location but also time and the intertwining of space and time.
The current understanding of the universe and its trajectory comes from developing theories to make sense of observation. Prior to 1998, there were three possible theories to describe the expansion of the universe from the Big Bang to infinity (Figure 6). The first theory states that the initial velocity from the Big Bang was not strong enough to counteract the long-term gravitational effects of every object in the universe. Therefore, the Big Bang causes the expansion, that expansion peaks, and then everything compresses, resulting in a “Big Crunch.” In this theory, our place on the expansion curve lies before the peak, as the fact of the universe's current expansion is an indisputable fact. The second theory states that the velocity from the Big Bang is exactly equal to the escape velocity from the gravity of the universe, which would result in the universe settling to a neutral level in the long term and
is often referred to as the “critical universe.” In the third theory, the initial velocity from the Big Bang exceeds the necessary escape velocity, causing the universe to continue expanding in a linear fashion, which is often referred to as the “coasting universe” or the “Big Rip.”
Figure 6. The three dominant theories of the universe, as understood by cosmologists.14
But in 1998, all three of these theories were cast aside when two independent research teams gathered data from the redshift of Type 1a Supernovae that painted a new picture of the universe: the Riess team and the Perlmutter team. Redshift is the observed 14 Dillon Brout, “AS109 - Cosmology” (Boston University, Boston, Massachusetts, Fall 2023).
change in light spectra for objects at a large distance from the observer which can then be extrapolated into the speed at which it is moving away or toward the observer. Their data showed that the universe was initially slowing in expansion and is now accelerating in its expansion.15 This conclusion brought about a confirmation of a version of the “Big Chill,” since it showed that matter is moving at an accelerating rate to the point where, apart from galaxies that are gravitationally bound together, all objects will become so separated that they are no longer able to interact, leaving the universe to become static, cold, and isolated (Figure 7).
15 Riess, Adam G. “The Case for an Accelerating Universe from Supernovae.” Publications of the Astronomical Society of the Pacific 112, no. 776 (2000): 1284–1299.
Figure 7. The three dominant theories of the universe as understood by cosmologists, in addition to the fourth and nowaccepted long term expansion model.16
Riess et. al. (1998):
The Riess et. al 1998 study utilizes the standard candle method of determining distances using type 1a supernovae.17 A
16 Dillon Brout, “AS109 - Cosmology” (Boston University, Boston, Massachusetts, Fall 2023).
17 Riess, Adam G. “The Case for an Accelerating Universe from Supernovae.” Publications of the Astronomical Society of the Pacific 112, no. 776 (2000): 1284–1299.
standard candle in cosmology is any set of objects with consistent and/or known luminosity that can be used to determine distances based on the relationship between distance (D), apparent brightness, and luminosity (L):
Brightness = ! "#$!
A common example of objects that can be studied with standard candles are cepheid variable stars. These are unstable pulsating stars that expand and contract periodically in accordance with their mass and density, making it easy to determine the luminosity based on what point in their expansion cycle they are observed in. While not extremely bright, they are incredibly common, so they function as reliable standard candles due to their multitudes in every galaxy. Type 1a supernovae are more preferable standard candles, as they are incredibly bright, and each explode at exactly the same mass (1.4 M☉). However, they are far less common, making them only useful to determine extremely large distances. Since the Riess study operates on the scale of the
entire universe, type 1a supernovae function as efficient standard candles. The study gathered evidence for 10 type 1a supernovae at high redshift (0.3 < z < 0.6, where z is the redshift factor defined between 0 and 1). The Reiss team identified potential supernovae and obtained their respective spectra in order to categorize the supernovae as SNe1a and calculate redshifts of each of their host galaxies.18 This in turn allowed them to calculate the velocities of said host galaxies. These calculations, coupled with additional information gathered from the data set, were used to fit the light curves of the supernovae as a means of comparing the calculated redshift velocity to the expected values for a predicted low-mass density universe. As the results are in excess of said expectations, “the data favor eternal expansion as the fate of the universe at the 99.7% (3.0p) to more than 99.9% (4.0p) confidence level from the
18 Riess, Adam G. “The Case for an Accelerating Universe from Supernovae.” Publications of the Astronomical Society of the Pacific 112, no. 776 (2000): 1284–1299.
spectroscopic SNIa sample.”
19 Essentially leaving very little room for the possibility for any trajectory other than the infinite accelerated expansion of the universe.
Perlmutter et. al. (1998): Saul Perlmutter led another research team during this same time frame, independent of Riess, with the same question to answer.20 Perlmutter et. al also employed the usage of high redshift type 1a supernovae as standard candles to date the universe, and they naturally reached the same conclusion as Riess et. al. They found that the apparent brightness of the supernovae indicates a missing piece in the understanding of the mass density of the cosmos through its expansion in the past and further expansion in the future. The close linkage between mass density and the progression of the universe's expansion allows for an application of
19 Ibid.
20 Perlmutter, S. “Supernovae, Dark Energy, and the Accelerating Universe.” Physics today 56, no. 4 (2003): 53–60.
the standard candle method that uses measurements of distant supernovae that could indicate the predicted progressive slowing of the expansion rate by gravity due to mass density. Since said cosmic mass density (ρm) would dictate the deceleration rate, any measurement of the supernovae prominence and distances would be effectively weighing the universe.21 But that is only a potential conclusion that could be drawn from the supernovae measurements. If instead the previous assumptions that mass density is the primary energy constituent across the universe the concluding measurements of the universe’s expansion rate would additionally indicate whether the cosmos is finite or infinite by determining the curvature of space. In turn, the end state of the universe depends entirely on the proportion of energy accounted for by mass density. They concluded that “A cosmic deceleration big enough to imply a ρm exceeding the “critical density” ρc
21 Perlmutter, S. “Supernovae, Dark Energy, and the Accelerating Universe.” Physics today 56, no. 4 (2003): 53–60.
(roughly 10-29 gm/cm3), that would indicate that the universe will someday stop expanding and collapse toward an apocalyptic “Big Crunch.”22
With those potential outcomes, amidst other variations, in mind, the team surmounted the challenge of using high redshift SN1a as the standard candle of choice despite their infrequent occurrence. The simplest cosmological models use mass density as a property through which to understand the expansion history of the universe; a higher mass density implies that more gravity slows down the expansion.23 However, the distances measured of the supernovae are drastically misaligned with this model. Since the supernovae are at high redshift, with an assumed high mass density universe, faint supernovae should be closer in the fabric of spacetime. Even in a low mass density universe, they should be further
22 Ibid.
23 Perlmutter, S. “Supernovae, Dark Energy, and the Accelerating Universe.” Physics today 56, no. 4 (2003): 53–60.
in space/time than the former but closer and more recent than the measured values. The faintness in the brightness data led to the conclusion that our universe has no mass density. Since that is clearly not the reality of our universe, the data suggest a flaw in the cosmological model. Mass density cannot be the driving factor in the universe’s expansion, so some other energy source must be increasing the expansion at an accelerating rate. Pinning down the exact driving factor, which is likely a concrete definition of dark energy, proves quite complicated as the universe is accelerating in its expansion now, but that has not always been expanding. Potential explanations of a cosmological constant (Λ) or shifting types of dark energy are not identifiable within a lab setting and remain elusive when searched for within the folds of the observable universe as well. It is defined to determine the expansion rate of the universe so, of course, varied dark-energy models have different implied expansion rates across eras of the universe’s history (Figure 8). So, as stated by
Perlmutter et. all; “we must hunt for the fingerprints of dark energy in the fine details of the history of cosmic expansion.”24 This conclusion is notable, as it marked not only the definitive existence of dark energy but also its dominating presence in the universe today; the authors hypothesize an eventual takeover that trends toward the Big Chill.
24 Ibid.
Figure 8. The cosmic expansion models measured from the redshift and brightness of SN1a.25
This conclusion is somewhat unnerving. It is hard to grapple with the understanding that the universe does not really 25 Saul Perlmutter; Supernovae, Dark Energy, and the Accelerating Universe. Physics Today 1 April 2003; 56 (4): 53–60.
end but rather fizzles away. But it is even harder to reconcile with the fact that this understanding seems to be the most convenient coincidence in history. If we were in a civilization living 100 billion years from now, this picture of the universe would be difficult to conclude by observation, as the expansion of the universe would have accelerated so quickly that the night sky would be virtually empty. In other words, each galaxy will be so far isolated from all others that the only conclusion that can be gathered from observation is that the universe has always and will continue to exist in that way (Figure 9). It is therefore imperative to wonder – why are we so lucky to live at this turning point in cosmological history in the 21st century? Answering that question in its entirety is far beyond the scope of current scientific capability, but the magnitude of this question should not prevent further analysis and theorization. There are a few options that can still be examined.
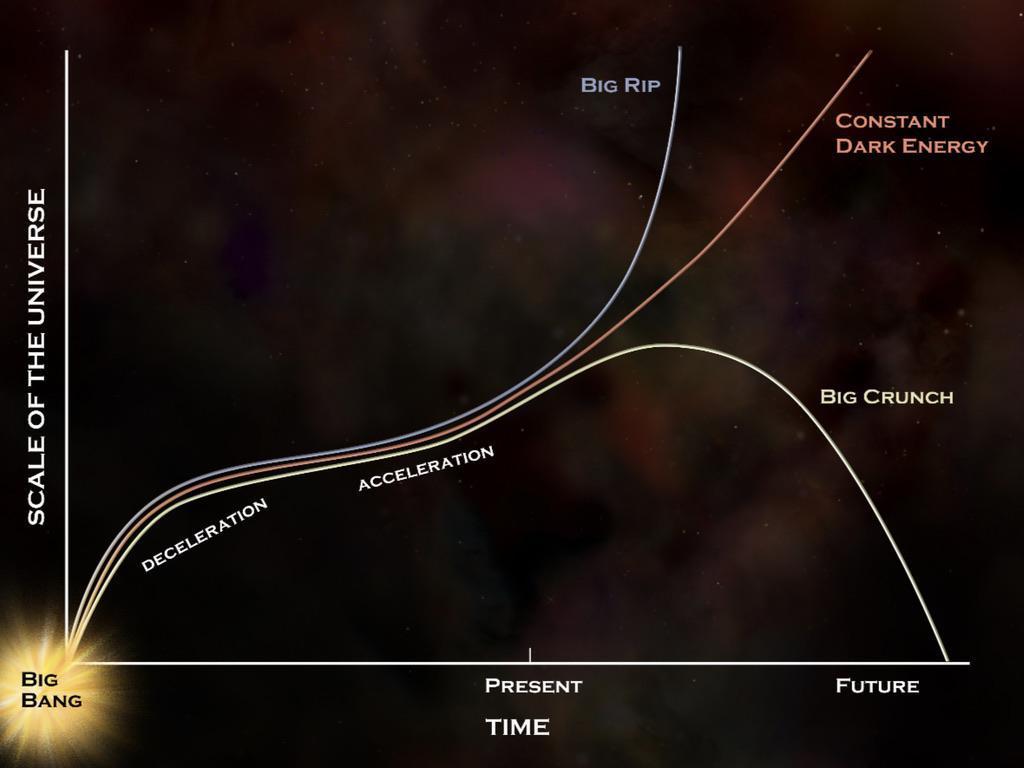
Figure 9. Possible long term behaviors of the expansion universe as a function of time.26
We live at a point in the universe’s history where these previous possibilities are all potential theories, capable of being at least thought through, even if they have been proven incorrect by
26 Riess, Adam G. "The Case for an Accelerating Universe from Supernovae," Publications of the Astronomical Society of the Pacific 112, no. 776 (2000): 1284–1299.
experiment. This is not something that can be taken for granted. The universe, on its largest scale, violates a fundamental scientific principle. When considering the universe as a whole, the conservation of mass cannot be assumed. This feels somewhat uncomfortable, yet it is vital to internalize in order to understand the long-term trajectory of the universe. As an aside - the infinite expansion of the universe implies that a violation of the conservation of mass is possible, given that we do not exist in a closed system. Now with this in mind, it is easier to see just how important the coincidence problem is. At point zero (i.e. the Big Bang) the universe was small and dense, dominated by mass. But as time moves along the universe grows and grows, more empty space exists so more dark energy can be “created”. There is a threshold at which the universe transitions to its “matter dominated” era, which isa point at which matter and energy (including dark energy) are in equilibrium. But then time continues, and the universe continues to expand, and another
threshold is crossed, pushing the universe into its “dark energy dominated” era. As humans in the 21st century, we exist just after this threshold. We live in this perfect moment wherein we can observe and understand the universe’s origin and predict its future. At what point is this considered a coincidence rather than a correlation, or maybe neither? There is the very real possibility that our observational understanding overshadows the potential alternative explanations for the behavior of the universe. After all, it is hard to confirm legitimate theories centered around realities that we have no capability of observing.
Common Issues
It is important to address the fine line between complexity and over-complexity. The universe is vast, chaotic, and complex, so there is nothing inherently wrong with complex models to explain the problems and behaviors within it. However, it is noteworthy that the addition of numerous caveats and conditional
clarifications within an already complicated theory may be a warning sign that there is a flaw closer to the core of the explanation. And so, Occam's razor plays a key role in both contemporary and ancient cosmological theories. Historical occurrences of this issue can clarify the red flags within modern theory; just because something is seemingly accurate does not necessarily mean its foundation is valid. Consider again the Ptolemaic model; even though its predictions held true and were able to be accurately applied to practical creations like the Antikythera Mechanism, the overcomplexity of the epicycles and deferents for every single tracked object led to 80 orbits for fewer objects in the final model, and as a whole was in clear violation of Occam’s razor. Of course, the model later proved to be false, as the fundamental principle of geocentricity that sits at the core of it has been dismissed. With the continued addition of modifying factors to the standard model of modern cosmology, there are calls for revaluation of the base structure of current theory. The confirmed
discovery of dark energy27 but lack of clarity on how to accurately represent it in modeling has prompted a plethora of potential theories that slightly alter our current understanding of the universe. These alterations bear resemblance to the caveats in the Ptolemaic model in that they seemingly smooth over obvious flaws in the attempt to keep the theory intact. The question then remains – will the modern understanding of the universe meet the same fate as Ptolemy's given the overly complex add-ons? Will a more elegant and simple understanding be uncovered? Or is the addition of a cosmological constant vital to bring a modern theory to its more complete and clear explanation?
27 Perlmutter, S. “Supernovae, Dark Energy, and the Accelerating Universe.” Physics today 56, no. 4 (2003): 53–60.
Riess, Adam G. "The Case for an Accelerating Universe from Supernovae," Publications of the Astronomical Society of the Pacific 112, no. 776 (2000): 1284–1299.
Concluding Connections
While ancient Greek theories may seem disparate from modern cosmology, their relationship may be vital to understanding the evolution of cosmological thinking. Uncovering their enmeshed, intricate overlap can strengthen the understanding of the past and in doing so, aid in understanding the future.
What further purpose do the intricacies of the Ptolemaic model and the Antikythera mechanism serve when understood in context with the accelerating universe and the discovery of dark energy (and vice versa)? All four of these snapshots of cosmological history share the common thread of attempting to map out and comprehend the entirety of the conceivable universe, by primarily if not entirely using the power of observation to accomplish this seemingly insurmountable task. Clearly observation alone cannot lead to accurate conclusions, as appearances from our skewed Earthly perspective are deceptive
and have led to errors and misunderstandings across centuries of attempts to understand the cosmos. As stated by Saul Perlmutter,
“We live in an unusual time, perhaps the first golden age of empirical cosmology […] Not only is the universe accelerating, but it apparently consists primarily of mysterious substances. We’ve already had to revise our simplest cosmological models. Dark energy has now been added to the already perplexing question of dark matter. One is tempted to speculate that these ingredients are add-ons, like the Ptolemaic epicycles, to preserve an incomplete theory. With the next decade’s new experiments, exploiting not only distant supernovae, but also the cosmic microwave background, gravitational lensing of galaxies, and other cosmological observations, we have the prospect of taking the next step toward that “Aha!” moment when a new theory makes sense of the current puzzles.”28
The rich history of cosmological thought is one fraught with mistakes and misunderstandings but also moments of timeless insight. It is those enduring observations, accompanied by the misinterpretations, that will propel the new wave of cosmology and 28 Perlmutter, Saul; Supernovae, Dark Energy, and the Accelerating Universe. Physics Today 1 April 2003; 56 (4): 53–60. https://doi-org.ezproxy.bu.edu/10.1063/1.1580050
those that will inevitably follow so that as time goes on, a more complete picture of the universe will be derived beyond simply looking up.
Works Cited
Carman, Christián C., and Gonzalo L. Recio. “Ptolemaic Planetary Models and Kepler’s Laws.” Archive for history of exact sciences 73, no. 1 (2019): 39–124.
Edmunds, M. G, T Freeth, Y Bitsakis, X Moussas, J. H Seiradakis, A Tselikas, H Mangou, et al. “Decoding the Ancient Greek Astronomical Calculator Known as the Antikythera Mechanism.” Nature 444, no. 7119 (2006): 587–591.
Goswami, G K, Anirudh Pradhan, and A Beesham. “Friedmann–Robertson–Walker Accelerating Universe with Interactive Dark Energy.” Pramāṇa 93, no. 6 (2019): 1–9.
Gott III, J. Richard, Michael S Vogeley, Silviu Podariu, and Bharat Ratra. “Median Statistics, H0, and the Accelerating Universe.” The Astrophysical journal 549 (2001): 1-.
Freeth, T., Higgon, D., Dacanalis, A., MacDonald, L., Georgakopoulou, M., & Wojcik, A. (2021). A Model of the Cosmos in the ancient Greek Antikythera Mechanism. Scientific Reports, 11(1), 5821–5821.
Kragh, Helgle S., 'From Myths To The Copernican Universe', Conceptions of Cosmos: From Myths to the Accelerating Universe: A History of Cosmology (Oxford, 2006; online edn, Oxford Academic, 1 Jan. 2010.
Lu, Meishu, Jun Su, Weiguo Wang, and Jianlong Lu. “Visualization of Kepler’s Laws of Planetary Motion.” Physics education 52, no. 2 (2017): 25006-.
“Orbits and Kepler’s Laws - NASA Science.” Accessed March 6, 2024. https://science.nasa.gov/resource/orbits-and-keplers-laws/.
Perlmutter, S. “Supernovae, Dark Energy, and the Accelerating Universe.” Physics today 56, no. 4 (2003): 53–60.
Riess, Adam G. “The Case for an Accelerating Universe from Supernovae.” Publications of the Astronomical Society of the Pacific 112, no. 776 (2000): 1284–1299.
Riess, Adam G, Alexei V Filippenko, Peter Challis, Alejandro Clocchiatti, Alan Diercks, Peter M Garnavich, Ron L Gilliland, et al. “Observational Evidence from Supernovae for an Accelerating Universe and a Cosmological Constant.” The Astronomical journal 116, no. 3 (1998): 1009–1038.
Timberlake, Todd K. “Modeling the History of Astronomy: Ptolemy, Copernicus, and Tycho.” Astronomy education review 12, no. 1 (2013): 010201-.