Annabelle Nielsen
Senior Thesis | 2024
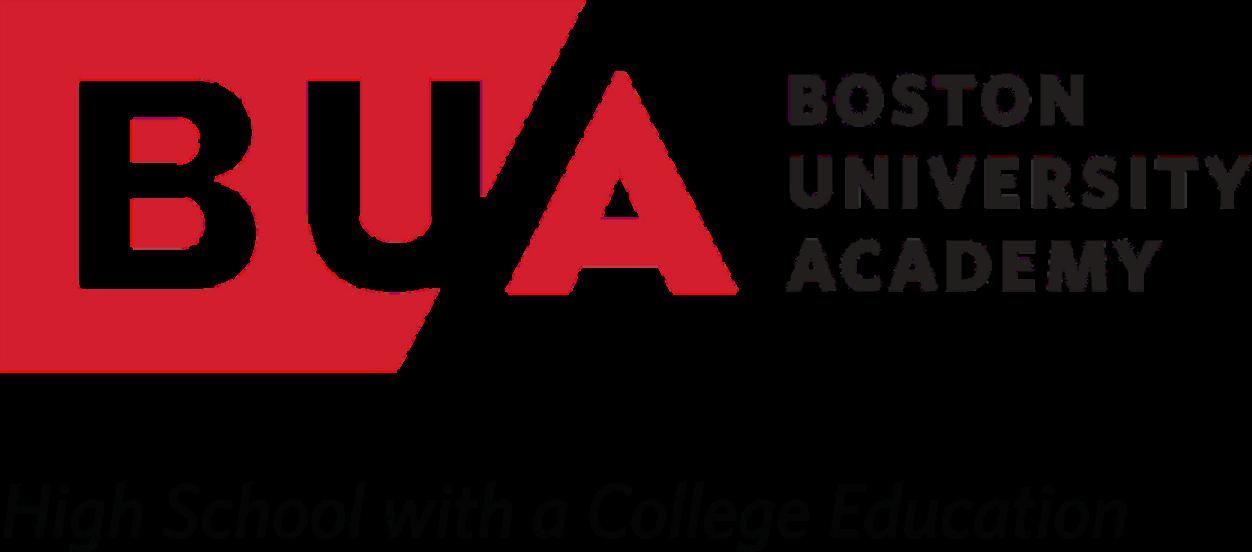
Cryopreservation Practices for Mesenchymal Stem Cells and Potential Effects During CpG Stimulation
Annabelle Nielsen
Abstract:
Mesenchymal stem cells (MSCs) are a key element for the future of regenerative medicine because they are multipotent stem cells that can differentiate into multiple cell types including bone, cartilage, and fat cells. MSCs have had a recent uptick in usage from immunology to traumatology because of their vast clinical applications. Thousands of trials have already been reported in the early phases for treatment of spinal cord injuries and traumas. MSC cryopreservation needs to be studied because fresh MSCs are not attainable due to economic and quality testing purposes. Additionally, since they must be harvested, preserved, and cultured in vitro prior to clinical trials, maximizing the efficacy of their freeze-thaw practices has been an area of research with high interest and need. Harvested human MSCs were cryopreserved and then thawed in different cryopreservation agents in order to explore the viability of different MSCs depending on their freezing agents. Three cryopreservation agents were tested: cryostore, 50:50 EZPZ to Rooster Media, and 25:75 EZCPZ to Rooster Media. 25:75 yielded the most live cells after one cycle of cryopreservation. In the second experiment, MSCs, now with a maximized yield, were combined with CpG stimulants to explore the possible expanded effects in stem cell therapy. CpG oligonucleotides were used along with a control of GpC. The stimulation was performed on both fresh and thawed MSCs with a cryopreservation agent of EZCPZ with a ratio of 25:75 EZPZ to Rooster Media, the culture media used for these MSCs. The cells were preconditioned with GpC and CpG and then examined through flow cytometry staining. Slight differences were detected between both CpG thawed and GpC thawed, and CpG fresh and GpC fresh. These preliminary experiments will form the basis for future research aimed at providing expanded populations of functional MSCs for clinical trials.
Introduction:
Mesenchymal stem cell clinical usage and prospects:
Discovered as early as 1974, mesenchymal stem cells (MSCs) have been a growing topic in cell therapy research.¹ MSCs have had an extensive but short history in clinical practice. As of July 2020, 1,138 registered clinical trials were using mesenchymal stem cells. The top fields represented in clinical trials were traumatology (234), pneumology (99), and neurology (77).² These numbers prove the rising use and positive response of MSC treatment. MSC therapy has additionally indicated it to be relatively safe along with its effectiveness. One metaanalysis paper analyzing 62 clinical trials with various disease targets concluded no serious adverse events. The most common adverse event recorded from MSC therapy was transient fever which was concluded to be caused by the immunomodulatory properties of MSCs.³ Ultimately, the popularity of this treatment is growing as its safety and efficacy are being confirmed.
MSCs are most notable and useful because of their self-renewal and differentiation abilities.⁴ MSCs pathway to inflammatory sites occurs after they bypass the immune system. From there, they can differentiate into a multitude of cell types: neurons, blood cells, and skeletal myocytes.⁵ This differentiation into other cell types occurs when the MSCs are in a state of pluripotency. These numerous cell types increase the variety of the pathologies they can help treat. Then, their self renewal properties lead to a greater number of cells and a more efficient treatment. The combination of self-renewal and differentiation leads to their effective use in cell therapy.
Mesenchymal stem cells have various sources: the umbilical cord, dental pulp, and bone marrow. In this research and many others, bone marrow-derived stem cells are used. Bone marrow mesenchymal stem cells (BMSCs) account for ~0.001 - 0.01% of bone marrow. The method for retrieving these cells from the donor is invasive, and results in a small but effective amount of these cells. BMSCs are considered the
gold standard for cell sources.⁶ They express several immune markers including CD105, CD73, and CD90. These immune markers are important because in the latter half of this research their expression will alter after stimulation with CpG oligonucleotides. Many different characteristics apart from immune markers identify BMSCs and their subclasses making it difficult to define them.⁷⁸ BMSCs have become one of the most commonly used sources for clinical trials, but it is important to decipher the differences and potential downfalls they might propose in comparison to other sources.
An application of MSCs and their differentiation ability is spinal cord injuries. MSCs have been used in at least 21 clinical trials to treat severed spinal injuries. These conditions traditionally lack a cure because of the central nervous system’s inability to regenerate cells after damage. Once the damage is done there is nothing your body can do to repair the severed cord. Because of MSCs differentiation qualities, they can be used in therapies to recreate damaged sections of the spine.⁹ They work to bridge the gap in the spine. Another application for MSCs is inflammatory diseases and chronic degenerative disorders; MSCs are effective because of their anti-inflammatory effects. Thus, they can be used to treat pathologies like chronic degenerative disorders and a number of inflammatory diseases.¹⁰ Mesenchymal stem cells adaptability in the environment they are placed in makes them an effective cell for a multitude of stem cell therapies.¹¹
Cryopreservation of MSCs:
Though mesenchymal stem cells have continued to grow in popularity for cell therapy, there are many issues with their freezing practices thus making it difficult to expand many of the successful clinical trials. Economic feasibility is the overarching complication with cell therapies; a close location and thorough regulation is key to having a sustainable product.¹² Once derived from the donor, quality testing needs to be performed to ensure MSCs will be effective, but pressing medical cases often do allow for this delay. Additionally, proximity wise, the donor needs to be near the recipient due to the timely manner the cells need to be injected. Frozen MSCs provide time to relocate for more accessibility and pre-prepared cells allow for optimal testing.
Cryopreservation refers to the process of freezing and thus preserving cells, in this research, MSCs. Reducing intracellular crystals during freezing, cryopreservation uses a cryoprotective agent (CPA) which creates stability and maintains cell health. DMSO is a common CPA and is an example of a cell membrane penetrator. DMSO is commonly applied to bone marrow, which was the source of MSCs for this research. It is also important to note that if DMSO was used without limit cells could be perfectly preserved but due to possible cell damage at high amounts it is limited.¹³
Freezing is the most effective option, but there may be many complications associated. It is important to note the varying results and opinions regarding the effects of cryopreservation. Due to the new nature of this work, additional research will continue to clarify. Three of the most notable potential challenges are stronger innate and adaptive responses, altered biodistribution, and cellular senescence. One of the innate responses that thawed MSCs can trigger is a blood-mediated inflammatory reaction, which consists of multiple response cascades. Apoptosis with interaction with allogeneic T cells is observed in cryopreserved MSCs when immediately thawed which is an additional negative effect. This leads to the issue of which model of induction into the patient. The rapid destruction of these cells by the immune response suggests that infusion may not be as effective. Altered biodistribution refers to thawed MSC’s decreased ability to bind to the extracellular matrix ultimately not allowing engraftment in the lung which is the first organ that MSCs interact with after infusion. Additionally, the overall use of biomaterials can affect MSC’s post-thaw. Research on cellular senescence, and cell division, in cryopreserved MSCs suggests that BMMSCs may be more susceptible than other sources to lower function than pre-freeze cells.¹⁴ Research performed in 2019 suggests that freezing of P2 BM MSCs is feasible and maintains most properties needed for clinical use.¹⁵ More research will continue to clarify the effects and the procedures that can be used to limit them. Thawed MSCs inherently pose differences to fresh, but they are not too severe for clinical use, and the benefits of cryobank-ing lead to this as the most effective way to implicate MSCs for clinical use. Overall, cryopreservation of MSCs is a
topic in need of greater research, and a vital step to maximizing efficacy of MSCs in stem cell therapies.
Immune markers, and immunomodulatory factors:
The latter part of this research explored the expression of different immune cell surface and intracellular markers on mesenchymal stem cells in response to CpG stimulation. MSCs have great immunomodulatory effects in addition to their differentiation and self renewal properties. This means that their immune markers, or the proteins on their surfaces can change how a human immune system reacts to different diseases or infections. Many of these effects vary based on the source of the MSCs and this research will only consider potential effects of BMSCs. ¹⁶ CD73, an extracellular immune inhibitory protein, is one defining marker of MSCs. CD73 can be discussed in context with CD39, another antibody expression tested in this experiment due to their coupled nature in the cell's pathways. CD39 differs from CD73 because it is an integral membrane protein while CD73 is a dimer of two identical subunits. In this coupled process, CD39 yields ATP, ADP, and AMP. Glycosylation of CD39 then affects enzyme activity, protein folding, etc. CD73 follows CD39’s actions creating adenosine by degrading the ATP, ADP, and AMP created. Overall, these two markers are a prime example of how immune markers regulate the cell's functions in reaction to pathologies.¹⁷
CpG, an immunomodulatory factor, was used in this research to elicit a response from mesenchymal stem cell immune markers. CpG DNA are short oligonucleotide sequences that bind to CpG sites in nuclear DNA. CpG sites consist of 5’ cystine, a phosphate, and a 3’ guanine. Synthetic oligodeoxynucleotides contain immunostimulatory CpG motifs which will lead to a cascade that involves several immune cells including B and T cells. The oligonucleotides are taken up into the cell through endocytosis and interact with TLR9.¹⁸ CpG is effective only through TLR9 but this does not create a problem because it has been demonstrated that all undifferentiated human MSCs will express TLR9 and therefore CpG will be taken in through endocytosis. The cascade of cellular events following TLR9 activation ultimately leads to the
upregulation of cytokine/chemokine gene expression.¹⁹ The cytokines expressed are part of a superfamily of proteins that are a key to immune system cell signaling. Each cytokine has a specific receptor it interacts with; one example of a cytokine and its receptor presented in this research is cytokine CD178 whose receptor is CD95.²⁰ This is only one example, but as a whole, the use of CpG is an important insight into the potential effects of immunomodulators in stem cell therapies.
Data Analysis:
Both the yield experiment and stains were run using a flow cytometer. Flow cytometry offers a multitude of uses including cell counts and stains in just a short amount of time. Most stains receive results within one or two days and cell counts occur in a matter of minutes. The flow cytometry machine runs plates of samples and detects both cell size and complexity. The results are portrayed in a scatter plot. It runs cells through a series of lasers and based on how the cells reflect the beams from the laser, it can read the cellular size and complexity. The results can be interpreted by using knowledge about the size and complexity of different cell types, or cells in different states. Some examples are neutrophils vs. MSCs or live vs. dead cells. These cells are identified through their size and complexity.
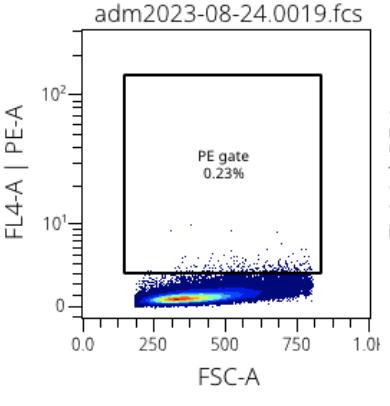
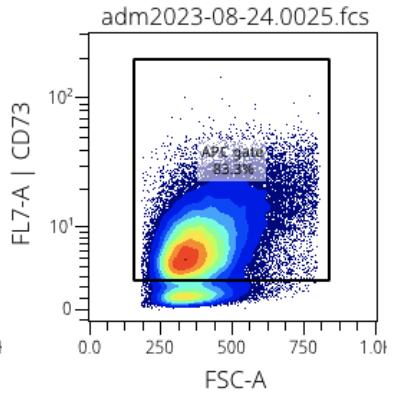
Figure 1: Example MSC PE-A stain Figure 2: Example MSC CD73 stain
Ultimately, different populations will be represented on the graph for each distinct group. The first experiment using the flow cytometer was the simpler of the two, simply separating live vs dead cells into distinct populations.
The second experiment used flow cytometry to identify the expression of different cell surface markers. To detect the presence of a marker a specific antibody that binds to the marker was added to the well with the mesenchymal stem cells. Each antibody was tagged with a fluorophore, a fluorescent molecule that is excited at certain wavelengths. As the flow cytometer separates cells based on size and complexity, the population of cells bound to the fluorophore-bound antibody will appear distinct and a percentage of expression can be calculated. Cells that were bound to the fluorophore-tagged antibody appeared larger and therefore in a separate population on the graph. Both Figure 1 and 2 are examples of final gated graphs. The x-axis represents forward scatter and the y-axis represents the specific antibody used to represent specific complexity (ex. PE-A and CD73). Figure 1 represents the cell surface marker PE-A and is an example of a cell surface marker
that bonded a very small quantity of fluorophore-tagged antibody. On the other hand, Figure 2 shows CD73, a commonly known cell surface marker for MSCs shows significant uptake of the fluorophore-tagged antibody. This uptake is understood because of the two distinct populations, one on top and one on the bottom. Figure 1 and 2 are both single stains, but through the program OMIQ, stains can be grouped together to create stronger conclusions from many data points.
Research Goals:
This research at the Lederer Lab was for exploratory purposes. Mesenchymal stem cells are a relatively new field of research and their freeze-thaw practices and stimulations are even newer. They have several relevant applications in trauma immunology and were therefore of high interest to the Lederer Lab Group. The goal of this paper is to identify where to direct further research.
Two experiments were performed in this research. The first was to test the cryopreservation agent used for the MSCs. DMSO was the active ingredient used in all of the cryopreservation media in this research; as a cryoprotective agent it ensures no crystallization in the cryopreservation process. Two cryopreservation media were tested: EZCPZ and CryoStore. EZCPZ was a cryopreservation not used by the Lederer Lab Group previously while CryoStore was used as standard practice. EZCPZ was frozen in two different ratios: 50:50 EZCPZ to Rooster Media and 75:25 EZCPZ to Rooster Media. The goal of this experiment was to determine the most effective media type and ratio to be used for the subsequent CpG and GpC stimulation and staining. Finding the most efficient cryopreservation agent would lead to the most effective results for subsequent research.
The second experiment continued with 75:25 EZCPZ to Rooster Media. Two factors were tested, resulting in four groups: CpG thaw, CpG fresh, GpC thaw, and GpC fresh. It is of note the circumstances, time in media, and direct methods, were not completely alike between fresh and thawed cells leading to a more effective comparison of GpC vs. CpG.
The next step in the research was to stimulate bone marrow human MSCs with CpG and GpC. CpG DNA was incubated with cells to
enact a response in cell surface markers for immune response to antibodies.
When staining, different immune marker levels were tested in the MSCs. Immune markers are present on both the cell surface and intracellular and bind a specific antibody. All immune markers in this research were extracellular except for CALR-APC. Two different staining protocols are used for extracellular and intracellular and for this research, only the intracellular markers were used to draw comparisons. Differences between CpG and GpC can be determined through different marker expressions because CpG will differ in this expression. The makers chosen for this research were based on previous RNA sequencing done at the Lederer Lab. Because this research was exploratory further research will be able to select the markers of most interest.
Methods:
The following two protocols, thawing and Trypl-E, are consistent throughout the research and whenever referenced in short, it can be assumed that the whole protocol outlined below was followed.
Thawing method for human P5 BM MSCs:
P5 BM MSC vials stored in liquid nitrogen were removed and placed in a water bath at 70 degrees Celsius to thaw for 3 minutes. Vials are then removed from the water bath and pipette mixed into 10 ml of pre-warmed thaw media in a 15 ml tube, incorporating 1 ⁄ 4 of the cells at a time into the media. One vial of cells per 10 ml of thaw media. Tubes are then spun down for 10 min at 100xg. Thaw media is aspirated. Thawed cells were then plated or analyzed through flow cytometry.
Trypl-E protocol to remove confluent cells from the flask: Rooster Media aspirated from flasks into 15ml conical tubes. Cells washed with 5 ml of HBSS, HBSS aspirated. 5 ml of Trypl-E pipetted into each flask to be placed in an incubator at 37 degrees celsius temp for 10 min. Trypl-E and cells aspirated into tubes already containing used
Rooster Media. Tubes spun down for 10 min at 100xg. Excess media and Trypl-E aspirated.
Cryopreservation agent yield testing post-thaw:
Three vials of P5 BM MSCs thawed from liquid nitrogen. After thaw media is aspirated cells are resuspended in 5 ml of Rooster Media. Resuspended cells are placed in 225 cm^2 with 25 ml of Rooster Media to total 30 ml of liquid per flask. Cells grow to confluence overnight. Once confluent, cells are removed from the flask using the TRYPL-E protocol. Each flask was split into 4 vials, for a total of 12 vials, 4 of each cryopreservation agent. Cells resuspended in 1 ml of respective cryopreservation agent: CryoStore, 50:50 EZCPZ to Rooster Media, or 25:75 EZCPZ to Rooster Media. Cells were placed in the cold room in a Mr. Frosty Freezing Container for 20 min then switched to -80 degrees Celsius freezer overnight.
The following day one tube of each respective cryopreservation agent was thawed. Each tube was resuspended each vial in 5ml of thaw buffer. Cells were filtered through a 70 dka filter. Cells were plated in a round bottom plate 20:180, cell solution to PBS. Cells run through the flow cytometer for the count. Count used to calculate dilution for Zombie Violet stains of live vs. dead cells. Zombie dye was diluted to 1:800 and then a conversion factor of 500,000 cells per 1 microliter of dye was used. This calculated the amount of dye solution that needed to be added to each sample. Three samples per cryopreservation agent were plated in a round bottom plate. Cells were incubated with Zombie Violet at room temperature for 10 minutes. Samples were washed with 100 microliters of CSB and spun at 750 Xgravity for three minutes, three times. Samples were then run through the flow cytometer to distinguish live vs. dead cells. Data was analyzed in OMIQ. Gating was added to remove doublets and debris.
CpG and GpC stimulation:
Eight 75cm2 flasks were placed in the incubator at ~20 degrees celsius temp to become confluent. Once confluent the BM MSCs were
designated into four groups of variables, two flasks were grouped: fresh CpG, fresh GpC, thaw CpG, and thaw GpC.
The fresh vs thawed protocols differ slightly because fresh MSCs were immediately stained after a 1 hour CpG and GpC stimulation, but the thawed MSC’s were frozen directly following stimulation, and thawed the following day. Therefore, though the direct stimulation time was the same, the thawed cells were tested further after stimulation. For the stimulation of all eight flasks, CpG and GpC DNA were diluted 60 microliters per 40 milliliters of Rooster Media. 45 ml of stock solution was made, and then 10 ml of solution was pipetted into each of the four flasks. After each was finished with stimulation the Trypl-E protocol was performed. 4 flasks were aspirated at a time, 2 CpG and 2 GpC of either fresh or thawed. All flask cells were aspirated into a 50 ml tube. Tubes were spun down at 100 xg for 10 min.
For fresh MSCs, the supernatant was aspirated, and the cells were resuspended in 2 ml of C5. 2.8 ml of stabilization added and incubated for 15 minutes. 20 ml of HBSS was added and then spun down for 10 min at 100 xg. They were resuspended in 4 ml CSB and now prepped to be stained.
For cryopreserved MSCs, the supernatant was aspirated and each of the 4 tubes of cells was resuspended in 1 ml of 25:75 EZPZ to Rooster Media and placed in cryopreservation tubes. Tubes were placed in the cold room for 20 minutes before moving to the -80 degrees Celsius freezer overnight. Cells were thawed using the above method and resuspended.
In summation of the timeline, the stains for fresh MSCs occurred on the same day as the stimulation, while the cryopreserved MSCs were stained the following day.
Antibody stains:
This procedure was obtained from the Lederer Lab Group, but was adjusted to the specific parameters of this experiment. This is used following the stimulation outlined above.
200 microliters of cells and CSB solution plated per well in a round bottom plate. 20 microliters of Fc-block were added to each well, 1:100 in CSB, and incubated for 10 min at room temperature. Antibody solutions mixed 2 microliters of antibody in 100 microliters of perm buffer. 20 microliters of antibody cocktail were added to each well. Plates were incubated for 40 min in a dark area.
The next set of procedures outlines the standard practice for an antibody stain, this may have to be adjusted depending on the antibodies used.
Antibody cocktail wells were washed with 150 μl CSB, and spun with 750 x g/3min. 16% PFA stock was thawed and 0.4% stock PFA from the 16% PFA stockwas made performing a 1:40 dilution of the 16% (100 μl into 3900 μl PBS). 100μl of the PFA was added directly to each sample for a final concentration of 0.4% PFA for 10 min RT, cells were mixed well with PFA using a vortexer. Cells were permeabilized with 100μl of eBioscience Fix/perm buffer (made from 4X stock 1:4 dilution mix two solutions) and left for 30 min at RT. 1X eBioscience perm buffer was made up with an end volume of 100 microliters per well. Made from a 10x solution by using the hand gun and pipettes were aspirated out the sterile, bottled water (not CSB). Wells were not aspirated. 100 μl of eBioscience perm buffer was added (made from 10X stock) directly to the cells and the F/P, then mixed. Cells were spun 750 x g/3min and the supernatant was discarded. Cells were washed with 150 μl perm buffer, spin with 750 x g/3min 20μl of diluted (in 1X eBioscience perm buffer) I.C. antibody was added per sample, incubated for 60 min at RT, meanwhile, setup the compensation (step 15) Cells were washed with 150μl eBioscience perm buffer, spin 750 x g / 3min, and then washed with 150μl CSB, spin 750 x g / 3min. No compensation was used for this
experiment. Cells were resuspended in PBS and run on cytometer immediately.
The data was analyzed in OMIQ using gating to remove doublets and debris.
Results and discussion:
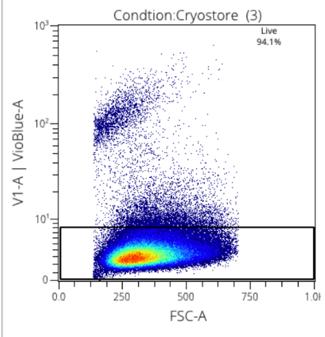
Figure 3: Viability stain with Cryostore
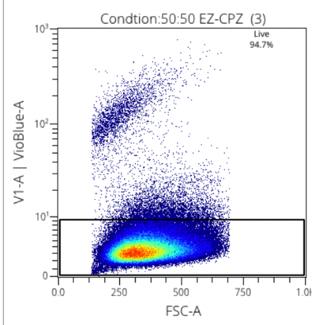
Figure 4: Viability stain with 50:50 Rooster:EZCPZ
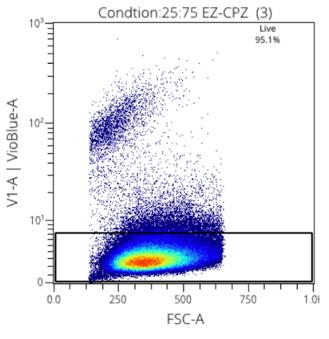
Figure 5: Viability stain with 25:72 EZCPZ
Figure 3: Cryopreservation agent and ratio yield results
Two media were used in this research, CryoStore, and EZCPZ. 50:50 and 25:75 ratios were used to find the maximized efficacy of the cryopreservation agent. EZCPZ was combined with Rooster Media in the final frozen solution. Three samples of each were tested using the same MSCs. The gating of stains was done manually for singlets and live cells. The two distinct populations demonstrate the live vs dead cells making it easy to distinguish the most effective cryopreservation agent. 25:75 EZCPZ had the highest yield after the removal of doublets and debris at 95%. Cryostore and 50:50 EZCPZ were close at 94.1% and 94.7% respectively. 25:75 EZCPZ to Rooster Media was used for subsequent research including the CpG stimulation on cryopreserved vs. fresh MSCs.
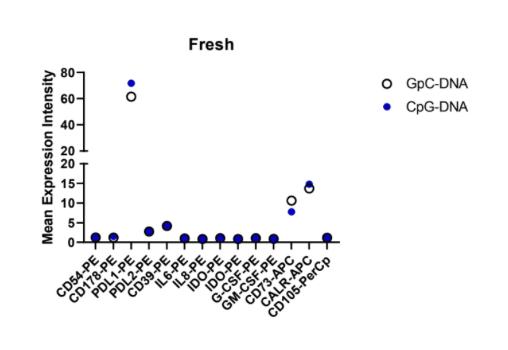
6: Fresh MSC
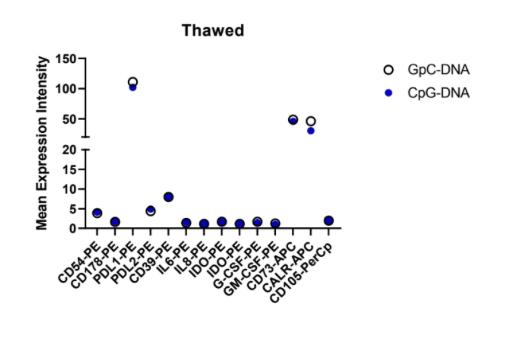
7:
This stain encompassed multiple variables: freeze vs. thaw and CpG vs. GpC. This inherently created a few weak spots in the data that need confirming results. As demonstrated by their methods, the fresh vs. thawed cells were not completely replicated in their methods. Conclusions can be drawn by comparing the pattern of expression among the antibodies, rather than the numerical values.
Slight changes can be observed in the mean expression between CpG and GpC DNA on CD73, and PDL1. CALR-APC was not interpreted when observing the pattern because as an intracellular antibody it had a separate staining method.
Overall, there was no significant change in the expression pattern between the CpG and GpC simulations. Specific values differ, for example CD73-APC which is in the range of 5-15 for fresh mean expression but as high as 50 for mean expression in thawed cells which is negligible due to the varying procedure. Repeat experiments with protocols more aligned between fresh and thawed will allow for more detailed conclusions to be drawn. From these results, the converging effects of cryopreservation and CpG on mesenchymal stem cells can be observed.
Future research:
Future research in freeze-thaw practices will continue to improve the effectiveness of MSCs in clinical trials. Additionally, it will hopefully increase the benefits derived from CpG oligonucleotide stimulation on MSC immune markers. Ultimately both of these experiments were used for exploratory purposes to discover the focus for subsequent research. Therefore, continuing with 25:75 EZCPZ subsequent research should first validate the results of CpG and GpC stimulation and antibody staining. Validation was not an option during this research period due to a week-long set back after an improperly mixed solution was used for culturing. With a longer timeline, this would have been the first priority. Once validated, specific antibodies can be identified as of interest and can be used for future research. Additionally, intracellular antibodies such as CALR-APC and others can be looked at more closely.
Additionally, looking into clinical research once the effects of CpG oligodeoxynucleotides on MSC immune effects is clearly identified and cryopreservation practices are maximized animal testing will be the next step. Several clinical trials with mesenchymal stem cells have proven their safety and additional CpG use has proved non-lethal.²¹ Analysis on mice after trauma is induced will provide more insight into the functional effects of these treatments.
This research works to improve the efficacy of mesenchymal stem cell therapy and offers insight into the potential benefits of CpG stimulation in stem cell therapies.
Bibliography:
1. Friedenstein, A. J., Chailakhyan, R. K., Latsinik, N. V., Panasyuk, A. F., & Keiliss-Borok, I. V. (1974). STROMAL CELLS RESPONSIBLE FOR TRANSFERRING THE MICROENVIRONMENT OF THE HEMOPOIETIC TISSUES. Transplantation, 17(4), 331–340. https://doi.org/10.1097/00007890197404000-00001
2. Rodríguez-Fuentes, D. E., Fernández-Garza, L. E., Samia-Meza, J. A., Barrera-Barrera, S. A., Caplan, A. I., & Barrera-Saldaña, H. A. (2021). Mesenchymal Stem Cells Current Clinical Applications: A Systematic Review. Archives of medical research, 52(1), 93–101. https://doi.org/10.1016/j.arcmed.2020.08.006
3. Wang, Y., Yi, H., & Song, Y. (2021). The safety of MSC therapy over the past 15 years: a meta-analysis. Stem Cell Research & Therapy, 12(1). https://doi.org/10.1186/s13287-021-02609-x
4. Galipeau, J., & Sensébé, L. (2018). Mesenchymal Stromal Cells: Clinical Challenges and Therapeutic Opportunities. Cell Stem Cell, 22(6), 824–833. https://doi.org/10.1016/j.stem.2018.05.004
5. Ullah, I., Subbarao, R., & Rho, G. (2015). Human mesenchymal stem cells - current trends and future prospective. Bioscience Reports, 35(2), 1–18. https://doi.org/10.1042/bsr20150025
6. Attia, N., & Mashal, M. (2020). Mesenchymal Stem Cells: The Past Present and Future. Advances in Experimental Medicine and Biology, 107–129. https://doi.org/10.1007/5584_2020_595
7. Herrmann M, Jakob F. Bone Marrow Niches for Skeletal Progenitor Cells and their Inhabitants in Health and Disease. Curr Stem Cell Res Ther. 2019;14(4):305-319. doi: 10.2174/1574888X14666190123161447. PMID: 30674266.
8. Bhat, S., Viswanathan, P., Chandanala, S., Prasanna, S. J., & Seetharam, R. N. (2021). Expansion and characterization of bone marrow derived human mesenchymal stromal cells in serum-free conditions. Scientific Reports, 11(1), 3403. https://doi.org/10.1038/s41598-021-83088-1
9. Montoto-Meijide, R., Meijide-Faílde, R., Díaz-Prado, S., & MontotoMarqués, A. (2023). Mesenchymal Stem Cell Therapy in Traumatic
Spinal Cord Injury: A Systematic Review. International Journal of Molecular Sciences, 24(14), 11719–11719. https://doi.org/10.3390/ijms241411719
10. Gao, Q., Wang, L., Wang, S., Huang, B., Jing, Y., & Su, J. (2021). Bone Marrow Mesenchymal Stromal Cells: Identification, Classification, and Differentiation. Frontiers in Cell and Developmental Biology, 9, 787118. https://doi.org/10.3389/fcell.2021.787118
11. Li, H., Ghazanfari, R., Zacharaki, D., Lim, H. C., & Scheding, S. (2016). Isolation and characterization of primary bone marrow mesenchymal stromal cells. Annals of the New York Academy of Sciences, 1370(1), 109–118. https://doi.org/10.1111/nyas.13102
12. Cottle, C., Porter, A. P., Lipat, A., Turner-Lyles, C., Nguyen, J., Moll, G., & Chinnadurai, R. (2022). Impact of Cryopreservation and Freeze-Thawing on Therapeutic Properties of Mesenchymal Stromal/Stem Cells and Other Common Cellular Therapeutics. Current Stem Cell Reports, 8(2), 72–92. https://doi.org/10.1007/s40778-022-00212-1
13. Jang, T. H., Park, S. C., Yang, J. H., Kim, J. Y., Seok, J. H., Park, U. S., Choi, C. W., Lee, S. R., & Han, J. (2017). Cryopreservation and its clinical applications. Integrative Medicine Research, 6(1), 12–18. https://doi.org/10.1016/j.imr.2016.12.001
14. Cottle, C., Porter, A. P., Lipat, A., Turner-Lyles, C., Nguyen, J., Moll, G., & Chinnadurai, R. (2022). Impact of Cryopreservation and Freeze-Thawing on Therapeutic Properties of Mesenchymal Stromal/Stem Cells and Other Common Cellular Therapeutics. Current Stem Cell Reports, 8(2), 72–92. https://doi.org/10.1007/s40778-022-00212-1
15. Oja, S., Kaartinen, T., Ahti, M., Korhonen, M., Laitinen, A., & Nystedt, J. (2019). The Utilization of Freezing Steps in Mesenchymal Stromal Cell (MSC) Manufacturing: Potential Impact on Quality and Cell Functionality Attributes. Frontiers in Immunology, 10. https://doi.org/10.3389/fimmu.2019.01627
16. Song, N., Scholtemeijer, M., & Shah, K. (2020). Mesenchymal Stem Cell Immunomodulation: Mechanisms and Therapeutic Potential.
Trends in Pharmacological Sciences, 41(9), 653–664. https://doi.org/10.1016/j.tips.2020.06.009
17. Antonioli, L., Pacher, P., Vizi, E. S., & Haskó, G. (2013). CD39 and CD73 in immunity and inflammation. Trends in Molecular Medicine, 19(6), 355–367. https://doi.org/10.1016/j.molmed.2013.03.005
18. Klinman, D. M. (2004). Immunotherapeutic uses of CpG oligodeoxynucleotides. Nature Reviews Immunology, 4(4), 249–259. https://doi.org/10.1038/nri1329
19. S. Nurmenniemi, P. Kuvaja, Lehtonen, S., S. Tiuraniemi, I. Alahuhta, Mattila, R. K., J. Risteli, Salo, T., Selander, K. S., Nyberg, P., & P. Lehenkari. (2010). Toll-like receptor 9 ligands enhance mesenchymal stem cell invasion and expression of matrix metalloprotease-13. Experimental Cell Research, 316(16), 2676–2682. https://doi.org/10.1016/j.yexcr.2010.05.024
20. Cameron, M. J., & Kelvin, D. J. (2000-2013). Cytokines, Chemokines and Their Receptors. In Madame Curie Bioscience Database. Landes Bioscience. Retrieved from https://www.ncbi.nlm.nih.gov/books/NBK6294/
21. Klinman, D. M., Currie, D., Gursel, I., & Verthelyi, D. (2004). Use of CpG oligodeoxynucleotides as immune adjuvants. Immunological reviews, 199, 201–216. https://doi.org/10.1111/j.01052896.2004.00148.x
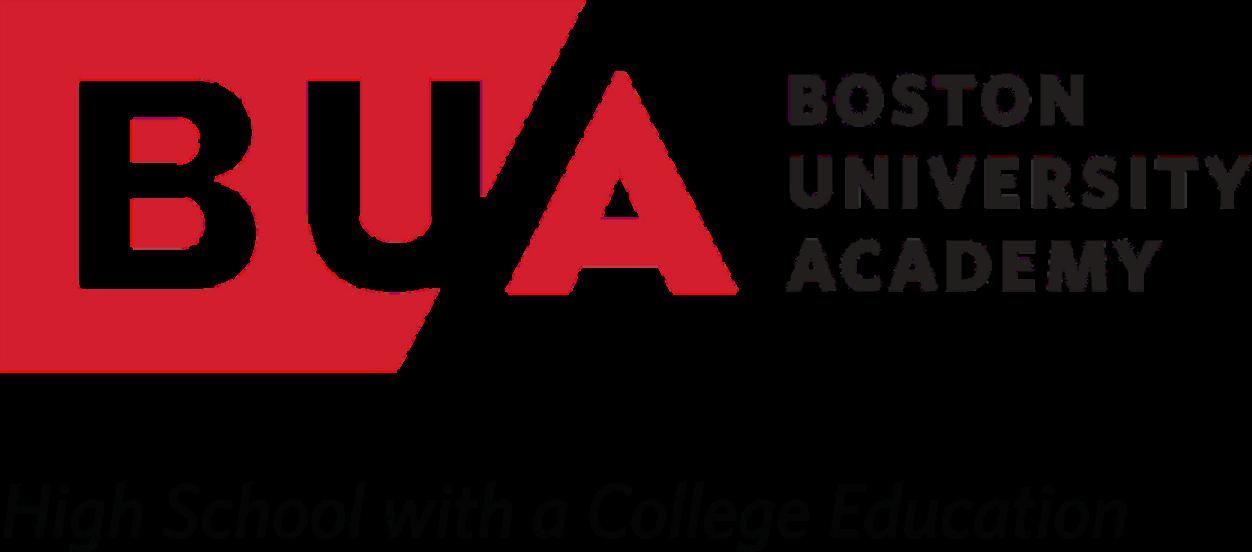