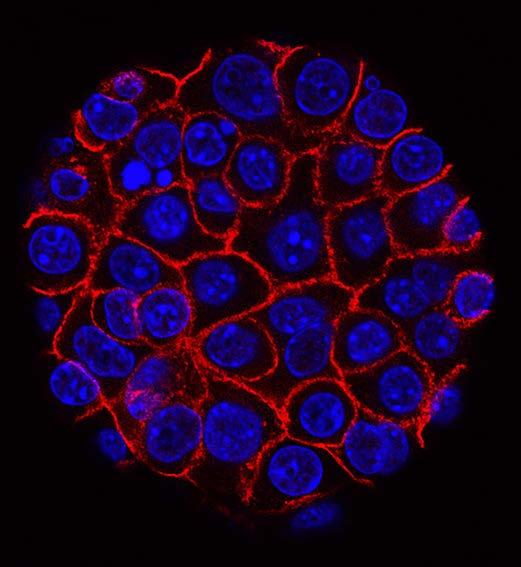
4 minute read
A Step Closer to Cancer-Killing Cells
A BU-led team has forged a set of powerful tools for building synthetic gene circuits that enable precise and customizable control of human cells, such as immune cells, for cancerkilling and other therapeutic functions. This tool kit might help accelerate the clinical adoption of synthetic circuits and realize the potential of emerging gene- and cell-based therapies.
“Gene- and cell-based therapies—in which cells are genetically modified to treat a disease or perform a therapeutic task—are poised to revolutionize medicine, but they also have significant limitations and need to be advanced,” says Associate Professor Ahmad “Mo” Khalil (BME), one of the study’s authors.
Advertisement
For example, in CAR T-cell therapy, a remarkable immunotherapy in which immune cells are genetically engineered with synthetic receptors that enable them to recognize and attack tumors, these immune cells can become overactive, often leading to toxicity and even death. That’s just one issue.
“Our argument is that we need platforms that allow us to develop clinically viable synthetic circuits to better control therapeutic cellular functions,” says Khalil. “This work is an attempt to fill that gap.”
Specifically, the team has developed a tool kit of programmable gene regulators they call synZiFTRs, short for synthetic zinc finger transcription regulators. Zinc fingers, which occur naturally, are proteins that bind to DNA. Using them as the basis for synZiFTRs carries distinct advantages: they’re human-derived, so they’re less likely to be rejected by the human immune system; and they’re compact, so they can be delivered easily into cells.
Next, the researchers used the synZiFTRs to engineer a set of drug-inducible gene circuits. In other words, if a certain drug were administered, that would be a signal to activate the gene circuit and instruct engineered cells to carry out defined tasks, such as expanding their population or killing a kind of tumor cell.
To further the platform’s chances of future success in the clinic, the researchers picked drugs that had already been approved by the FDA, such as tamoxifen, which is used to treat breast cancer. And, they chose to focus on CAR T-cell therapy to establish a proof of principle for their new system.
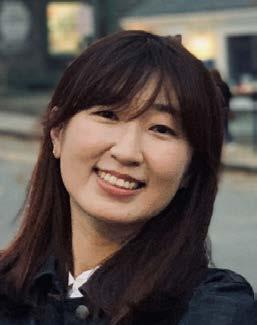
It worked. In both in vitro and in vivo experiments, synZiFTR-regulated T-cells could be activated by the drugs to trigger desired cellular responses. “We can remotely activate the T-cells in an on-demand manner to trigger their antitumor activity,” says Khalil. “This was effective enough to eradicate a blood tumor in animal models.”
According to Khalil, the team—which includes Associate Professor Wilson Wong (BME), postdoctoral researcher Hui-Shan Li and Divya Israni (ENG’21)—is excited to provide this tool kit as a resource to the research community. “Even though we used CAR T-cells for our demonstration,” he notes, “these synZiFTRs are broadly applicable genetic parts, which can be used to develop genetic circuits for other scientific and clinical needs.”
Capra especially wanted to work with Khalil because he’s developed a small-scale bioreactor system called eVOLVER, now in use in more than 50 universities. A customizable, automated platform that can remotely monitor and manage hundreds of cell cultures in real time for a variety of applications, this DIY open-source system is infinitely adaptable, allowing researchers to create custom automation tools for their own microbial experiments.
One of Capra’s objectives is to develop new methods for working with complex waste-based feedstocks—in other words, the material left over after bacteria break down manure and food scraps is what gets fed into a bioreactor—but a challenge with that kind of material is variability in its makeup. By running a slew of experiments with eVOLVER, Khalil will rapidly optimize the process, allowing different types of waste-based feedstocks to turn out a consistent level of product—in this case, retinol (vitamin A) to start with.
Khalil is adapting his invention to this work with a custom diffuser, precisely controlling fluid flow and culture conditions.
“By the end of 18 months, we want to have a fully automated biofilm reactor pilot plant that can continuously produce more than one kilogram of vitamin A per day,” says Yazicigil, but that will entail two more key stages.
Safe And Sustainable Sensors
Yazicigil’s expertise is in electronics, but she is more than a dabbler in biology. Some of her recent work includes designing an ingestible capsule that monitors gut health with the aid of a tiny sensor that runs on ultra-low power.
For the BioMADE project, Yazicigil is adapting that technology to produce sensors that will float inside the bioreactor and measure the levels of PH, oxygen, glycerol, lactate and various organic acids. The sensors will even evaluate the electric potential of the biofilm.
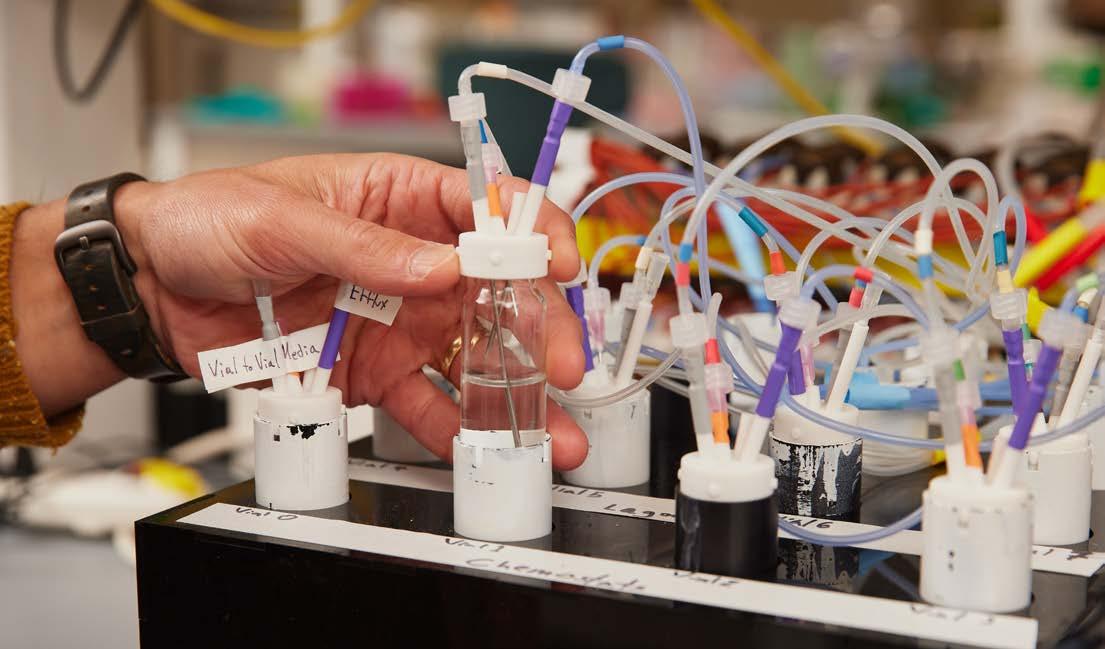
“Traditionally, bioreactors are monitored with bulky instruments or complex probes that have to be inserted in holes in the lid or side,” Yazicigil says. By contrast, her tiny sensors, running on mere nanowatt-level power, will wirelessly transmit the measurements in real time, allowing technicians to adjust the flow of nutrients as needed.
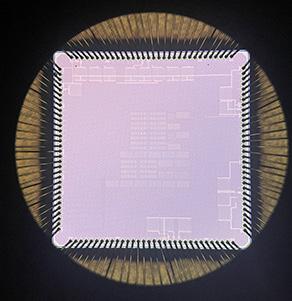
A crucial part of her task is making the communications secure, Yazicigil says, and her team is working with Capra to make security integral to the sensors. “We need to protect against communication attacks, like eavesdropping, or jamming attacks, which would impact communication between the sensors and the hub.”
“I have to tailor the sensor chips to fit the system needs of this bioreactor technology,” Yazicigil adds. “It’s an exciting project. It involves startups, defense, academia, biomedical and electrical and computer engineering. It’s powerful to bring these all together.”
Democratizing Synthetic Biology
To truly scale up the modified eVOLVER, the operation will have to be moved to Densmore’s Design, Automation, Manufacturing and Processes (DAMP) Lab. An electrical engineer by training, Densmore has been working in synthetic biology research since
2007 and is a natural fit for the BioMADE project due to his expertise developing electrodes for microfluidics and robotics for high-throughput testing.
The DAMP Lab is an advanced biofoundry that did double duty for a couple of years as a major part of BU’s award-winning Clinical Testing Laboratory, which processed up to 6,000 COVID tests of faculty, staff and students per day during the pandemic. Now, the heavily automated lab is getting ready to run several biofilmfocused eVOLVER systems at once.
For Densmore, the product is not (or at least not only) retinol; it’s the process itself. By sharing the results, technical reports and methods of the BU team with the other 140 members of the BioMADE consortium, Densmore and company will be propagating a replicable system for converting all sorts of bio-based feedstocks into all manner of useful products.
Replicability is key, Densmore explains. “No one wants to say, ‘I cured cancer once.’” To tackle global challenges from cancer to climate change, he says, “We need more eyes on these problems. To do that, we need to lower the barrier to entry—but safely. So we centralize the manufacturing infrastructure,” within a certain number of certified labs like BU’s, “and distribute the computational infrastructure.”
First, BioMADE researchers will remotely order up experiments, and Densmore and team will carry them out. In the longer