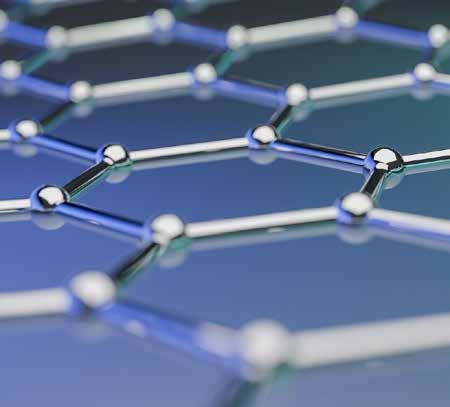
12 minute read
47 The structure and potential applications of borophene. Jacob Tutt
from Ink 2020/21
Jacob Tutt Lower Sixth
The synthesis of graphene, at the Univer- plications, its regular honeycomb structure sity of Manchester in 2004, initiated huge could not be greatly manipulated, especially excitement and financial investment in to the degree that was required on the mithe nanomaterial industry. The planar, croscopic scale of nanomaterial research. honeycomb lattice of carbon promised a remarkable and wide range of potential The material borophene has gained recent applications across the scientific spectrum. attention and it is suggested to rival graGraphene is a semi-metallic material with phene in many applications. Borophene is previously unparalleled a 2D allotropic matemechanical, electrical, rial, with a triangular chemical, and optical base structure. All properties. The material three phases (alis a zero-gap semicon- lotropes) of boroductor with extremely phene have recently high carrier mobility and been synthesised on is therefore a poten- single-crystal silver tially efficient transistor. substrates, as the Furthermore, by only absorbing 2.3% of regular arrangement of silver atoms within visible light, it offered the first major steps the crystal force boron atoms into a regular towards a transparent conductor. Material pattern of hexagons with bonding to a scientists imagined a new era of “graphene- maximum of 6 boron atoms each. However, based computer processing and a lucrative under certain conditions it is common for graphene chip industry”. boron atoms to form only 4 or 5 B-B bonds, creating vacancies in the structures. This This visionary graphene-based world is is one of the two properties that make yet to materialise on the scale intended by borophene so promising. Its ability to form material scientists 16 years ago. However, regular vacancy patterns within a structure, it accelerated the research into the fabrica- which create subtle changes to charactertion of other 2D materials. One of the major istics, allows it to be strategically tuned for limitations of graphene was, despite its the desired properties. incredibly wide range of conceivable ap-
Before discussing further characteristics, it is important to understand the terms used to describe the planar directions in which 2D molecules are described – the two directions which are perpendicular to each other are described as the armchair and the zigzag directions. In contrast to most 2D honeycomb materials, borophene has a highly anisotropic crystal structure in space, implying a structure in which the armchair and zigzag directions have varying arrangements of atoms and characteristics. Due to the strongly anisotropic structure, the molecule can be manipulated for ideal application by correctly orientating the molecule so there is the correct combination of zigzag and armchair characteristics. It is important, before looking at the uses of each structure, to show the 2D arrangement of atoms within the 3 major allotropes/ phases (Striped, B12, and X3). The ‘base structure’, known as the stripped phase, has the densest arrangements of atoms, in which bonds travelling in the armchair direction are 1.6 Angstrom (Å) and those travelling in the zigzag direction are 2.8 Å. It is shown to have a ‘planar’ structure with anisotropic corrugation, with ‘out of plane’ buckling along the zigzag direction, and an un-corrugated structure in the armchair direction. This buckling along the zigzag direction is caused by the arrangements of atomic orbitals in this direction, as they have a majority of SP3 hybridisation Borophene is a 2D allotropic rather than less stable SP2 hybridisation. This can be proven by the accumulation of electrons on the top of the upper plane and material, with a the bottom of the lower plane. Although we triangular base structure. can see the dissociation of electrons from the bond along the zigzag direction, the electrons along the armchair direction are localised near strongly covalent bonds. The two other phases of borophene (B-12 and X-3) are planar without vertical undulations. These structures can also be referred to by their boron vacancy concentration, which is defined as the ‘ratio between the number of hexagon boron vacancies and the number of atoms in the original triangular sheet’, with B-12 borophene having a vacancy concentration of 1/6. Finally, it is important to appreciate the 3 major allotropes discussed are only those which have been physically synthesised, and many more allotropes have been theoretically predicted.
It is important to consider and evaluate the mechanical properties of borophene allotropes, as it provides evidence of the strength of the materials and leads to further understanding of their potential application. Upon initial consideration, it is thought that due to the strong nature of B-B bonds and the unique atomic structure, borophene materials will exhibit very high Young modulus, but with different numerical values for different perpendicular directions. It can be seen that the boron vacancy concentration can have a large effect on the mechanical properties, and therefore the allotropes must be evaluated separately. The densest structure, striped borophene, has the most regular atomic structure and therefore we can see a uniform extension throughout the material, whatever the loading direction. Striped borophene has been shown to have a Young modulus in the armchair direction of 398 N/m and 170 N/m in the zigzag direction, which are both higher than that of graphene. The much-reduced Young modulus in the zigzag direction in comparison to the armchair direction can be attributed to its ‘out of plane’ buckling, and its longer and less covalent B-B bonds discussed earlier. Furthermore, due to the corrugated lattice, the Poisson ratio is negative, describing it as a material that when stretching occurs along one direction causes stretching stresses along the perpendicular direction. hexagonal (B-12) or zigzag (X-3) lattices in comparison to bonds within these lattices. Interestingly, these phases of borophene exhibit a positive Poisson ratio. Therefore, when the materials experience stretching along one direction it causes contracting stresses along the perpendicular direction.
It has been proven that there is an almost perfect negative linear coloration between the Young modulus of the armchair direction and the boron vacancy concentration. For allotropes B-12 and X-3 the Young modulus in the armchair direction is 179 N/m and 199 N/m respectively, as stretching does not occur uniformly. There is a higher degree of deformation in bonds connecting conduction along the armchair direction yet a relatively prominent band gap along the zigzag direction. It is important to evaluate the term bandgap, which refers to the energy difference between the top of the valence band (minimum energy electron orbitals) and the bottom of the conduction band (maximum energy electron orbitals). Another point to consider is that these Metallic materials have an overlap between allotropes can be manipulated chemically these bands and therefore conduct at all and physically to give them the desired me- times. Semiconductors do not overlap; chanical properties. The power of chemical instead they contain an almost full valence modification can be seen by the dramatic band and almost empty conduction band decrease in the young modulus, in both of electrons. Therefore, they have varyperpendicular directions, when borophene ing resistances, because, as the material structures are completely hydrogenated or experiences work done to it, electrons can fluorinated. This effect can be attributed overcome the bandgap energy and transfer to bond elongation, of the B-B bond, from from the valence to conduction band. This 1.6 Å to 1.9 Å, which increases the number of decreases bond strength and, thus, the Young The electronic mobile electrons making it a more efficient conModulus. Interestingly, properties of ductor. Finally, insulators chemical modification increases the critical strain of the material, which borophene have created huge contain such a large band gap that an overwhelming majority are confined to increases the strain at which cracks form in the material. The structural excitement within the valence band making them very poor conductors. lattice of borophene with nanomaterial its ultra-high young modulus, high anisotropy, research. One of the major issues which face borophene as and negative Poisson a conductor is its high ratio (striped borophene), along with its energy state in comparison to bulk boron outstanding electric conductivity, may and, therefore, its thermodynamic instabilallow its application in flexible electronic ity and vulnerability to degradation under devices, biomedical sciences, and electro- high potential differences. Yet chemical chemistry. modification may be an effective approach to stabilising borophene structures and The electronic properties of borophene altering the bandgap. The fluorination have created huge excitement within of borophene creates two highly stable nanomaterial research. Like all other structures (B4F and B2F), which both characteristics of borophene, the electronic show remarkable thermodynamic stabilproperties are significantly anisotropic ity at temperatures as high as 1000K. Both with striped borophene showing metallic prove to be promising candidates for the production of semiconductors in electronic devices. Similarly, promising is the zerogap semiconductor that fully hydrogenated striped borophene creates. The material exhibits a Dirac cone electronic band structure, with the Dirac point located perfectly at the Fermi level (highest energy state at 0k). The term Dirac cone describes materials in which the valence band and conduction band take ‘the shape of the upper and lower halves of a conical surface, meeting at the Dirac point’. Zero-gap semiconductors are so promising in electrochemistry, due to their combination of metallic and semiconducting properties, which allow them to be low resistance transistors.
Many scientific studies have reported the superconductivity of borophene, the ability of the material to conduct electricity with zero resistance. The superconducting
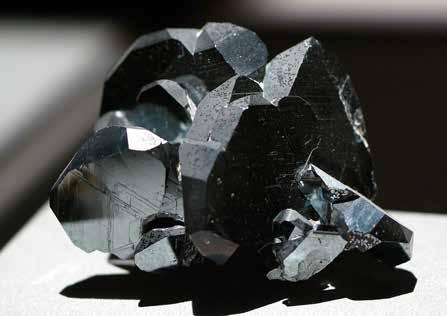
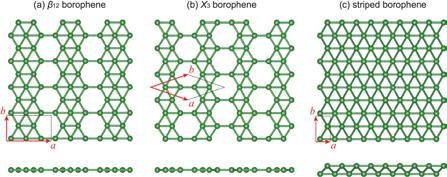
transition temperature, the temperature at which materials exhibit superconducting properties, is shown to be dependent on the boron vacancy concentration. As the concentration increases, to 1/9th (1 hexagonal boron vacancy per 9 atoms within the original triangular sheet), the transition temperature decreases gradually. Interestingly, once the boron vacancy concentration of 1/9th is surpassed the transition temperature rises again. This therefore suggests a minimum transition temperature at a concentration of 1/9th. The most stable structure, striped borophene (boron vacancy concentration of 0), has a transition temperature at 19K. The materials show a high ‘superconducting transition temperature and, therefore, a high application prospect in superconductivity’. property hints at its probable distinguishable performance during the charging and discharging process. Overall, the combination of borophene’s high alkali ion capacities, electronic conductivity, and ion transport speed suggests a very efficient anode material. These borophene electrodes may cause huge advancements in the field of medical devices, as the efficient energy storage and ultrafast charge time can greatly improve the functionality and practicality of biomedical implantable devices.
As a society, we have become increasingly aware of our environmental impact. Consequently, the material science industry feels an ever-mounting pressure to come up with a solution. One option to reduce the worldwide carbon footprint is the transition from fossil fuel combustion to hydrogen fuel cells, a potentially powerful alternative. Massive funding has gone into increasing the feasibility of this mechanism over the last few decades. However, a major limitation has been finding a safe and efficient way to store hydrogen so that it can be readily accessed. Borophene might offer a massive step towards making this mechanism of energy production feasible. It has the potential to be a material with an ultra-high capacity for hydrogen storage, although pure borophene sheets exhibit too little of an ‘absorption energy for practical hydrogen storage’. The ‘decoration’ of borophene sheets with metal atoms strengthens the interaction with hydrogen. For example, the use of lithium decoration dramatically increases the absorption energy from 0.047 eV to 0.35 eV. It simultaneously creates a lattice capable of a Li:H2 ratio of 3:1, a figure high enough to make hydrogen storage effective and efficient. It has been proven that the use of alkali metals has the greatest effect and is, therefore, the most feasible. Finally, another potential application for the 2D boron lattice, is the production of microelectronic, ultrasensitive, toxic gas sensors. It has been observed that there is a sharp increase in the current through B36 (decrease in resistance) upon the absorption of ethanol and formaldehyde molecules, therefore generating an instant electrical signal. This property has the potential to revolutionise biometric sensors [9]. Formaldehyde, although highly toxic, has high thermal stability and reactivity, making it common in many industrial and medical settings. Borophene may provide the answer for a highly sensitive formaldehyde sensor and therefore could potentially save many lives. Furthermore, its high sensitivity to ethanol could lead to a higher degree of accuracy in breathalyser type devices, and once again save lives.
In the past two decades, two-dimensional nanostructures have received attention for their potential ability as effective electrode materials, due to their large surface area. Borophene has been investigated for its Many scientific studies have capacity for alkali metal ion storage, due to its low density and ability reported the superconductivity to influence the surface of borophene.tension of a liquid’s surface activity. Striped borophene has shown a strong surface interaction with aqueous lithium atoms, with a fully lithiated phase having a boron to lithium ratio of 4:3 respectively, corresponding to the capacity of 1860mAh/g. This suggests that, per gram of borophene, a charge is stored sufficient to generate a current of 1860mA for an hour, a much higher value than other 2D structures including graphene, silicene, and phosphorene. The promising potential of borophene as an electrode material was highlighted by the high electronic conductivity of borophene, as well as its ability to maintain this property through the lithiation process. Furthermore, the Liion migration barrier of 2.6meV along the armchair direction (the resistance to flow of ions across the barrier between cathode and anode) suggests an ultrafast ion migration, with a much lower resistance to migration than other conductive super materials. This However, it is as important to realise the key issues that lie ahead for the material, one of which is its susceptibility to oxidation due to its high surface activity, and thus lack of ‘air-stability’. Although covalent modification, such as hydrogenation, is effective, it is important to minimise the effect on the material’s properties. Finally, it is vital to find a way to produce the material on an industrial scale in order for us to see the wide range of effects possible in the real world. Currently, due to the process requiring chemical vapour deposition, the synthesis temperatures are too high for an economically viable production. Furthermore, the adhesion energy between the material and the substrate used to form the material, Ag or Al, is too great for practical application.
In conclusion, the recent discovery of borophene in 2015 has led to a wide range of speculation on the structure’s potential applications. This does not just include physically synthesised structures, but allotropes that have been theoretically investigated through first principle calculations. What makes borophene so remarkable is that its many properties, whether mechanical, thermal, electronic, optical or chemical, show huge potential for revolutionising nanomaterial research, and multiple other areas of science. Moreover, through its hugely anisotropic nature in almost all properties, as well as its high degree of change across boron vacancy concentrations, the material can be tuned to the highest degree through orientation and careful allotrope selection. The material can be further manipulated through the application of physical stress, chemical and covalent modification or even the dimensions of the material, i.e. the number of layers, but many issues must be overcome until we can see the widespread application of this material become possible.