BREATHABLE BUILDINGS
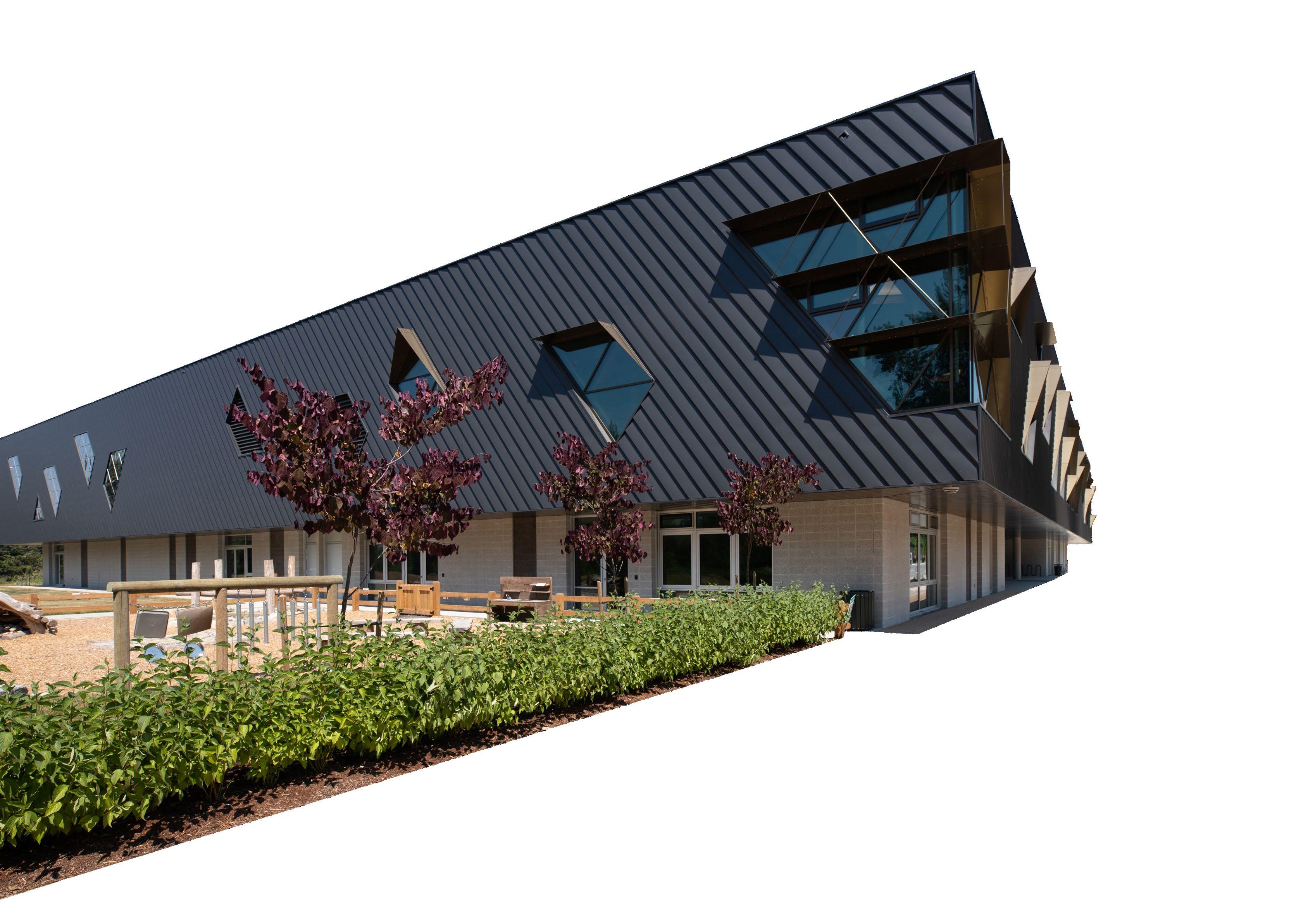

A contemporary examination of natural ventilation and its role in the future of building design
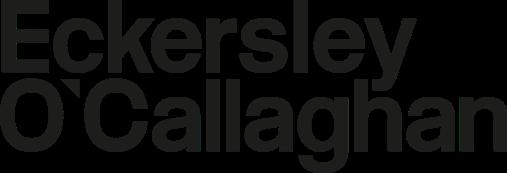
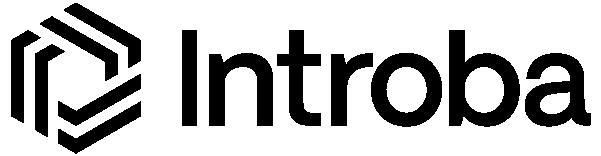
A contemporary examination of natural ventilation and its role in the future of building design
We would like to acknowledge Introba and Eckersley O’Callaghan’s sponsorship of this study, which has been made possible through the Impact Fund.
The Impact Fund is an internal program aimed at supporting thought leadership initiatives around sustainability and digital innovations for the built environment. It runs on an annual basis and funds projects, studies, essays and advisory pieces that provide new insights on how we percieve, design and build our human environment. It is an opportunity to reflect, question, think big and offer solutions to make our world more resilient, just, and regenerative.
We would like to thank the following people for their contribution to this study:
David Barker, Tom Donald,Thomas Bamber, Stephany Le Rhun, Damien Rogan, Louise Hamot, Keeley O’Reilly, Mat Loup, and Marissa Clark.
Cover image: Clayton Heights Community Centre ©IntrobaThis page:
1. Angel Square, London, UK
© Allford Hall Monaghan Morris
2 The Black and White Building, London, UK
© Ed Reeve
3. Artist’s Impression - Central Place Sydney, Australia
© Central Place Sydney
4. West Vancouver Passive House, Canada
© Battersby Howat
5. Indigenous House Toronto, Canada
© University of Toronto
6. American University of Beriut, Medical Centre Expansion
© Perkins & Will
7. Clayton Community Centre, Surrey, Canada
© Introba
8. Atlassian Central, Sydney, Australia
© SHoP / BVN
9. University of Victoria, HSD Building, Canada
© Perkins & Will
10. University of British Columbia, Arts and Science Centre
© University of British Columbia
In the wake of a global pandemic, we are witnessing concurrent stresses on our financial, social, environmental and health systems; and we are learning just how interconnected these systems are.
And yet, through these stresses we have an opportunity to reconcile, rather than compromise. In the words of Introba Principal, Mary Casey, we may harmonise these tensions into new solutions where “unseen and unrealised potential is revealed”1
As we grapple with this challenge, we are reminded that nature has provided us with a blueprint for success. Reconciling these tensions is something that the natural world — in all its complexity — excels at.
Breathable buildings are grounded in the belief that the built environment should function as an extension of the natural environment, supporting human health and wellbeing by drawing inspiration from the beneficial characteristics of natural ecosystems.
By integrating natural ventilation, daylighting and biophilic design principles, breathable buildings can enhance indoor air quality, increase operational resilience, and improve occupant satisfaction and productivity.
This study is a collaboration between two global engineering
1 Read more on The Law of Three in Mary Casey’s Resonances essay: https://www.introba.com/news/resonances-regenerative-practice
firms — Introba and Eckersley O’Callaghan — who share a commitment to advancing sustainable, resilient, and healthy building design. Drawing on our combined expertise and experience, we have developed a framework for designing and delivering breathable buildings to provide occupants with a greater connection to the outdoors.
This study explores breathable buildings through two lenses: why and how. In doing so, we discuss the value proposition and key challenges that commonly present themselves when delivering these buildings. It is intended to:
Support the business case for natural ventilation by articulating the social, environmental, and financial benefits of a breathable building
Provide guidance on procurement and integration to streamline the design and development process
Provide technical guidance for design teams to navigate challenges of natural ventilation system design
Remember that we inhabit a world of complex, interconnected systems. Breathable buildings are just one of a range of tools at our disposal.
We hope this study will spark meaningful discussions and inspire new approaches to building design that prioritise occupant health and wellbeing. We invite you to join us in this important conversation and to work together towards a future where all buildings contribute to better financial, social, environmental and health outcomes.
In this chapter, we explore why we should be building breathable buildings by drawing on experience working with clients on naturally ventilated projects around the world. We examine the trajectory of natural ventilation through time and the role it plays among alternative design approaches. We also highlight the key benefits that breathable buildings offer today for individuals and society.
Since the earliest human shelters and primitive buildings, the key objectives in building design have been to provide comfort and protection from the weather while allowing for a supply of fresh air.
Designers achieved this in various ways, but the challenge has always been providing fresh air without sacrificing that comfort and protection. Before air conditioning, passive systems were prevalent in vernacular architecture, from the hypocaust of Ancient Rome to the bagdir of Iran, relying on natural forces and materials to temper air and significantly improve comfort.
The turn of the 20th century saw a sharp change in the trajectory of ventilation design, relying increasingly on mechanical ventilation and air conditioning. These systems have been the driving force behind architectural efforts to seal buildings, avoid leakage and minimise the energy associated with heating and cooling. The aim has been to maintain tight control on thermal comfort while the fresh air component becomes diluted and difficult to adjust.
In recent years, however, the concept of the “breathable building” has experienced a resurgence. Accelerated by the challenges and stark impacts of COVID-19, there has been a rapid paradigm shift in how building designers consider the interface between indoor and outdoor spaces and the control of fresh air, comfort, and protection.
The following section examines the key drivers accelerating the move towards breathable buildings. These include the shifting requirements of the commercial workplace, industry advocacy, climate emergency, and occupant health and wellbeing.
Before discussing the drivers for natural ventilation, it is worth reflecting on another design philosophy building momentum, particularly in North America and Oceania.
Passive House (or Passivhaus) is an approach that uses five key principles to accomplish high performance. These are:
Continuous insulation
No thermal bridges
Airtight construction
High-performance windows and doors
Fresh air ventilation with heat recovery
The discourse regarding Passive House has often incorrectly assumed that a building designed to be airtight is incompatible with natural ventilation. Some have gone as far as to suggest that Passive House buildings cannot have operable windows at all.
Based on these misinterpretations, it seems like we are at a crossroads in our pursuit of high-performance, low-carbon buildings. It may appear as though we need to choose between opening the building up to create a breathable envelope or sealing the building up to create a Passive Housecompliant structure.
On the contrary, Passive House buildings worldwide have successfully implemented facade breathability, particularly in mild climates where operable windows can provide a significant proportion of summer ventilation.
Consider for a moment the objectives of a Passive House building, which is intended to be efficient, resilient, lowcarbon, healthy, and comfortable.
Sound familiar?
We are not at a crossroads, we’re simply tasked with taking a holistic view of our project context — risks, opportunities, constraints, and drivers — and developing a solution that meets the needs of its occupants, stakeholders, and community. That might not sound so simple, but it certainly shouldn’t preclude the pursuit of natural ventilation or a Passive House solution.
To support this strategic thinking, the following sections describe the drivers that put breathability on the agenda, from human and environmental benefits to financial and policy incentives.
This page: Artist’s Impression of Central Place Sydney and Atlassian Central ©Central Place Sydney
Much like biophilic design or a window view of nature, the provision of natural ventilation provides benefits to our wellbeing. Increasing outdoor air supply improves cognitive function and health by removing airborne pathogens and carbon dioxide (CO₂) released by occupants and volatile organic compounds (VOCs) released by building interiors1. It is surprising how often buildings, particularly schools, fall short of the minimum ventilation rates set out in standards such as ASHRAE 2 .
Even where code compliant ventilation is provided, these volumes are established as a minimum threshold rather than to support optimum health and wellbeing. The effects of underventilation carry a huge cost in terms of productivity and health and can lead to respiratory issues, allergies and absenteeism. Natural ventilation gives the option to boost outdoor air rates at a relatively low cost compared to the wholesale upsizing of mechanical systems.
Natural ventilation can also improve thermal comfort within buildings and the subsequent health impacts. There is mounting evidence that suggests uniform temperatures — as often found in mechanically ventilated buildings — can lead to reduced metabolism and glucose sensitivity. In contrast, natural ventilation coupled with an “adaptive comfort” design approach provides the variability in environmental conditions that is much more aligned with the needs of our body’s complex systems.
1 https://pubmed.ncbi.nlm.nih.gov/26502459/
2 https://onlinelibrary.wiley.com/doi/10.1111/ina.12403
For much of the 20th century, we have kept indoor environments as sterile as possible by sealing the building and killing any surface bacteria. There is now a growing consensus that we have been over-sterilising indoor environments, leading to an increased likelihood of asthma and allergies. Interior environments have a dynamic ecosystem of microorganisms. Some are healthy, and some are harmful3. A balanced culture of microorganisms in our built environment is necessary for human health. It is a challenging field to define because it must be balanced against preventing the spread of harmful pathogens, but in both cases, the provision of natural ventilation is beneficial.
Breathable buildings make for more resilient occupants, and the same is true for the buildings themselves. The inclusion of mixed-mode systems (i.e. the option to bring in natural ventilation to alternate with, or top up, mechanical systems) makes buildings inherently more adaptable and equipped to manage a host of possible events that may occur.
The past few years have been something of a portent in terms of climate change, consistently breaking records around the world, only to be rebroken a year or two later.
When we talk about designing a resilient building, the list of considerations is growing longer. In addition to heat waves and rising average temperatures, unusual weather has increased the likelihood of extreme storms and blackouts, wildfires are
3 Anthes, E. (2020) The Great Indoors. Scientific American / Farrar, Straus and Giroux
becoming commonplace across ever larger regions. Add to this the rising costs of energy, exacerbated by fuel shortages and geopolitical conflicts, and it is clear that building resilience is an urgent necessity.
When a building has multiple modes of operation, both passive and active, it provides flexibility to adapt over time and to weather short-term aberrant events. By balancing this with reducing energy consumption over the lifespan of a building and limiting the CO₂ released into the atmosphere, we can help slow the global warming causing these extreme events.
The commercial sector is evolving4. The shift to hybrid work following COVID-19 and its associated economic slowdown has caused companies, developers, and architects to rethink the modern workplace.
Employees are also demanding sustainable and healthy workplaces with better amenities , and there is increasing evidence that these features facilitate heightened productivity and improved staff retention rates. For most businesses, the financial expense associated with staff far exceeds outgoings for rent or energy bills, so the case for a people-first approach to workplace design is compelling.
For companies of all sizes, the challenge is to provide flexible and desirable workplaces to attract and retain the best
4 https://www.economist.com/finance-and-economics/2021/06/03/ what-a-work-from-home-revolution-means-for-commercial-property
workers and bring them back into the office. In Australian capital cities, the current high occupancy rates in Central Business District (CBD) office buildings are giving flexibility to companies who are out of lease to move to higher-grade, better-provisioned tenancies.
The pressure is on developers to provide these types of sustainable and healthy commercial products to retain and attract anchor tenants as well as smaller-scale leases . This includes flexible office space that can be used as co-working zones or informal meeting and event spaces.
Even within larger tenancies, flexibility and a range of spaces within a workplace fit-out have driven a requirement for an equal level of flexibility and adaptability of the building systems and fabric.
We are experiencing a swell of advocacy for healthy, resilient buildings among designers, engineers, industry bodies and institutions. Project teams, particularly in market segments where natural ventilation is less prevalent (e.g., new commercial buildings), are proposing breathable buildings more often than they were at the turn of the century.
One current focus area is reducing building material embodied carbon. The publication of the CIBSE Technical Memorandum 65 ( TM65)5 in 2021 has cast a light on the quantification of building services equipment embodied carbon, which drives 5 https://www.cibse.org/knowledge-research/knowledgeresources/engineering-guidance/technical-memoranda/tm65an-internationally-applicable-methodology-for-the-calculationof-embodied-carbon-in-building-services-engineering-alt
advocacy for the minimisation of HVAC equipment. This in turn drives appetite for mixed-mode or naturally ventilated buildings, creating incentive for all building design disciplines to ensure their knowledge of breathable buildings is in line with best practice.
Building certification schemes such as LEED, BREEAM, Green Star, Passive House/Passivhaus, and WELL align incentives to desired outcomes (such as improved ventilation) rather than rewarding specific approaches (such as natural ventilation). This technology-agnostic approach is a good thing; it reduces the risk of undesirable outcomes by avoiding prescriptive incentive schemes that promote a singular solution. Here are some examples:
France’s environmental standard ES RE2020, in force from 2022, mandated that 30% of the surface area of vision glazing must be operable. While well intentioned, the requirement has been typically side-stepped with loopholes. This often ends up adding cost without providing the benefits of natural ventilation
Vancouver and the province of British Columbia will introduce a 26 °C indoor air temperature limit in 2025 to avoid heat deaths. The policy has been criticised as it is expected to promote pushing costly and carbon-intensive active systems at the expense of passive and performancebased solutions
The London Plan, prior to the advocacy of the LETI and others, incentivised combined heat and power (CHP) technologies, even though they were higher carbonemitters than alternative solutions
Image: London cityscape © ShutterstockBy providing a roadmap for design and procurement, outlining the key steps involved and highlighting the challenges and opportunities along the way, this chapter will serve as a valuable resource for architects, engineers, developers, and building owners as they work to bring building breathability into the mainstream.
Global cities vary in their delivery of breathable buildings, and within most cities the prevalence of natural ventilation may vary significantly across building typologies. For example, London’s new commercial building stock features far less natural ventilation than its residential building stock.
In market segments where it is not considered business-asusual, design and procurement of breathable buildings can be challenging, from coordinating design elements across disciplines to finding the right ventilation products. The perceived risk associated with trying something new can quickly lead to breathability falling from the list of project ambitions.
We have developed an integrated process based on our experience delivering naturally ventilated buildings around the world. It provides a roadmap to mitigate the risks in the design and procurement process and protect ambitions for breathability that may otherwise become unviable.
And it’s certainly not all about computer simulations of airflow that are often showcased when discussing natural ventilation. Although these can be incredibly useful, there are many other elements to a successful breathable buildings project that will help to make delivery far more efficient and far less risky.
This
Determine the project goals and how they relate to a breathable building concept
Use building system data and post-occupancy evaluation to ensure objectives are met and inform future development projects
Allow for a program of testing and verification over time, to tune the ventilation system in various scenarios
7. Prototype and tune
Build mockups and create computational simulations of the design to verify performance expectations
Set up dedicated breathability workshops to collaboratively develop solutions that mitigate identified risks
Ensure the right specialist skills are available early in design and brief the team on breathable building objectives
Conduct a climate assessment and review how the site surrounds may influence breathability
Decide the most appropriate approach to breathability – flow, openings, control
Work with consultants to identify challenges to solve through design or in operation
As early as the business case/feasibility stage, it is important to establish a vision for the project and identify how a breathable buildings concept might contribute to — or become the centrepiece of — the achievement of project objectives. These goals may relate to climate resilience, prioritising health, and wellbeing, maximising the connection between indoor and outdoor spaces. The list goes on.
Once you have established project objectives, it is far easier to engage a team with the right skills and experience to deliver that vision. For breathable buildings projects, it is important to consider specialist expertise in facade design, building services, airflow (fluid dynamics), and the selection of architecture, quantity surveying and construction professionals with relevant delivery experience. Importantly, the client must ensure objectives relating to breathability aspirations are clearly briefed and specialist studies are scoped.
Based on the local context assessment, the team should develop a breathability strategy that harnesses the characteristics of the site and the proposed building. This is the time to set direction on key parameters such as flow regime (cross or stack ventilation), system integration (natural ventilation or mixed-mode), openings (operable windows or discrete louvres) and control philosophy (occupant or building management system) — among other key decisions.
Assuming the previous steps have gone to plan, the design development phase provides an opportunity to fine-tune the proposed breathability approach. Use physical and/or virtual mock-ups to validate the design and reduce uncertainty. This might include physical prototypes to test opening actuators or computational fluid dynamics (CFD) to assess indoor airflow characteristics and predicted comfort levels. Ensure performance requirements are embedded in project specifications for appropriate testing and commissioning.
Use the project consultants’ collective experience to identify risks associated with the breathability strategy, including condensation, airflow control, mechanism failures, occupant guidance, air leakage, noise, acoustic privacy, fire code compliance, etc. Create a risk register to track and navigate identified risks and develop integrated solutions.
During concept design, the consultant team should assess the local context to inform possible breathability approaches. The assessment should include a climate study (considering air, solar radiation, moisture, precipitation, and wind at a minimum) and a review of site surroundings to understand the potential influence of adjacent buildings and/or topography. This assessment is often captured in the sustainability consultant’s scope but can also be delivered by the architect, facade engineer or mechanical engineer if they possess the relevant expertise.
Set aside dedicated breathability workshops with project consultants to collaboratively develop integrated solutions that mitigate identified risks. Some risks can be designed out while others will need to be managed through operational policies or tenant lease agreements. During concept design, focus on solutions that resolve challenges using simple rather than complicated interventions. For example, if condensation risk is high, consider Low Temperature Variable Air Volume (LTVAV) systems rather than chilled beams with condensation sensors.
Make allowances for specific commissioning of the operable components of the building (e.g., facade actuators), HVAC equipment and building management system (BMS) controls in their capacity to control the natural ventilation performance of the building. Ensure performance requirements are met through targeted testing, which may occur over time as the building is operated in various occupancy and weather scenarios.
Use operational data to track building performance in relation to the breathable building systems. This may include the timing and duration of natural ventilation occurrences, the coincident indoor and outdoor environmental conditions, occupant behaviour, energy consumption, and frequency of maintenance calls. It is also recommended that postoccupancy evaluation surveys are conducted to assess how breathability is influencing the occupant experience. Finally, use this data and lessons learned in future development projects.
One surprising and powerful aspect of natural ventilation is that comfort can be achieved at higher temperatures than traditional systems. There are many examples of passive buildings where occupants report high satisfaction levels when it is 34°C outside and the windows are open. This is possible because our perception of comfort isn’t driven by air temperature alone, but is also influenced by humidity, sun exposure, surface temperatures and clothing.
While the opportunities and benefits are huge, designing buildings of this nature requires a fundamentally different way of thinking about comfort.
First, there is a fundamental prerequisite to block solar gain by reducing window-to-wall ratios and robust shading. This demands a departure from the heavily glazed buildings that many clients expect as the norm.
Second, the building must generate plenty of airflow and, ideally, exposed thermal mass. These are less strenuous demands but must be factored into the design at an early stage.
Finally, and arguably the greatest challenge, is that occupants must be convinced that temperature is misleading. Based on previous negative experiences in poorly designed buildings, many believe it is necessary to cool a space to 22°C on a hot, sunny day. We need more built examples, education, stakeholder willpower, and ultimately, a cultural shift to tip the scales back in favour of buildings that feel comfortable at higher temperatures.
Another key consideration in designing a breathable building is the occupant’s role in operating the natural ventilation system. It is worth developing a strategy in collaboration with the design team that addresses two primary decisions:
1. How conspicuous is the facade operability? Does the occupant experience operating windows, or are the openings concealed behind other features?
2. How much control does the occupant have? Are windows opened when they decide, or does the building management system automatically control operability?
Central Place Sydney is the centrepiece of a united vision to renew and transform the entire southern district of Sydney’s CBD into a burgeoning tech and innovation district “Tech Central”, attracting world-class tech and innovation talent to the city.
Introba facilitated collaborative design team workshops to develop the project’s natural ventilation approach. A key consideration was the occupant experience—how they will interact with the natural ventilation system and how they might better connect with the outdoor environment.
The goal from concept onwards is to generate robust airflow through all appropriate spaces. Given that most typologies can benefit from natural ventilation, this can include a significant portion of any building.
Six air change hours (ACH) is the typical target baseline ventilation rate on a warm day. The aim is to achieve comfort through the removal of heat, while 6ACH carries a further benefit in terms of dilution of air. Dilution is the rate at which airborne contaminants such as pathogens and exhaled carbon dioxide are removed from the space and replaced by outdoor air (OA). Traditional mechanical systems typically provide one to three ACH of OA, which corresponds with a 63% - 95% dilution rate. 6ACH provides 99.8%, which goes some way to explaining the improvement in cognitive function and reduction in colds and headaches associated with natural ventilation.
Guides such as the CIBSE Applications Manual 101 will walk a designer through the familiar options of single-sided and cross-ventilation. Stack-assisted ventilation is a reliable and economic way of generating plenty of airflow, provided a relatively centrally located central atria or chimney is available. Cross-ventilation is similarly efficient and effective, but a common challenge is that many spaces will be single-aspect. Single-sided natural ventilation is generally the least effective option. Openings must be significant in size, and the inclusion
1 https://www.cibse.org/knowledge-research/knowledgeportal/applications-manual-10-natural-ventilation-in-nondomestic-buildings-2005?id=a0q20000008I7m2AAC
of bug screens will blunt airflow for all but the smallest spaces.
What emerges is the opportunity to harmonise building form with function; to shape the building itself in a way which responds to the site and generates airflow, while mitigating ingress of noise or poor air quality. It is vital that wind — which is far more powerful than the stack effect — complements the intended direction of airflow and can generate additional buoyancy under all prevailing directions. If this sounds like a balancing act, it can also be the most thrilling part of the design evolution of a building.
The Oakridge Centre Redevelopment in Vancouver, Canada, quadruples the scale of an existing shopping mall to offer a true mixed-use community centre, including retail, residential and civic uses.
Introba provided multi-disciplinary services on the project, including computational studies to develop and validate the outdoor comfort and passive natural ventilation strategies.
To achieve natural ventilation during favourable external conditions while ensuring the building can be closed against extremes of external air temperature, pollution or wind, the facades of breathable buildings must have some operable elements that can be controlled.
The most common operable elements are horizontal louvres that pivot from the top to provide some resistance to rain while open. Using motors in the frame or by window actuators. Other forms and methods can be used but must balance the operational simplicity with the ability to protect from light weather and fully seal against extreme conditions.
For optimal performance, the facade operation needs to be controlled to respond to changes in air temperature, wind, heavy rain and even air quality. This requires an array of sensors linked to the building management system and coordinated with the mechanical systems.
From a system point of view, the operable systems typically incorporate multiple and additional smaller panels which require additional framing and associated gaskets. These are typically weak spots for both thermal performance and air permeability and consideration much be taken as to the impact of the elements on the overall facade performance. Other performance requirements such as safety, acoustics, and fire performance, can present challenges for specification and procurement if there is not a wide enough selection of products that have passed associated testing procedures.
From a system point of view, operable vents are typically incorporated as multiple smaller panels, requiring addition
framing and introducing more joints compared to a sealed facade. This creates weak points for both thermal performance and air permeability, and consideration much be taken as to the impact on the overall facade performance. Other performance requirements such as safety, acoustics and fire performance, must also be considered and will impact the choice of opening type and position of vents in the facade. Examples of opening types in a curtain wall system are illustrated below.
The process of designing, coordinating and commissioning breathable buildings can also have its challenges, although through partnerships such the one in this study between Eckersley O’Callaghan and Introba, the close integration of facades and building services is being streamlined.
Atlassian Central will be the world’s tallest hybrid timber building. Located in Sydney, Australia, the building aligns with Atlassian’s commitment to operate on 100% renewable energy and reach net zero emissions by 2050.
Eckersley O’Callaghan is providing structural and facade design, including the building exoskeleton that supports mega floors with a mix of naturally ventilated indoor and outdoor spaces.
Controls are the final hurdle that makes or breaks a natural ventilation strategy. Unfortunately, many natural ventilation systems do not operate as they were intended, and this contributes to the common perception that natural ventilation is too complicated or cannot achieve comfort.
Where natural ventilation systems are solely responsible for achieving summertime ventilation and cooling, there tends to be greater end-user understanding out of necessity.
In an era of wildfire smoke and heatwaves, natural ventilation is often designed as part of a mixed-mode strategy, where it supplements mechanical systems, to improve health and save energy. In mixed mode setups, it is common for the natural ventilation elements to operate below their potential in terms of number of occupied hours with natural ventilation operating.
Indeed, there are many high-profile projects featuring a carefully designed natural ventilation system that does not operate at all. While in some cases, the design may have been at fault, it is more likely that the controls were too complex and the operators reverted to mechanical-only mode.
The challenge for designers, particularly in larger, interconnected buildings, is that the controls must dynamically respond to multiple live conditions, including wind direction and velocity, internal and external temperature, room CO₂ and humidity, rain and even air quality. Ideally, the openings will modulate such that the user experience is seamless. Meanwhile, at the user end, the controls must be simple, intuitive, and empowering to the occupant.
From a landlord’s perspective, it is important to design controls to protect the base build from any inadvertent tenant behaviour that would see facades open in extreme weather events. These scenarios may put base building energy performance contracts or certification at risk potentially damaging base building materials and finishes.
At completion in 2021, Clayton Community Centre was Canada’s largest Passive House building. The community hub integrates visual and performing arts, a neighbourhood library, indoor sports facilities and outdoor recreation spaces.
Introba designed the building’s carefully calibrated mixed mode system and led a “lessons learned1” exercise following the first year of operation to maximise the building’s potential.
1 https://issuu.com/deepgreenengineering/docs/ impact_fund_clayton_community_centre
When approaching natural ventilation, another potential hurdle is the sheer array of systems, options and strategies available. Ultimately, there tends to be a common goal of providing outdoor air for energy savings and health. Here we present our “simple approach” to thinking about cost, carbon and payback of natural ventilation.
The hardware required to deliver natural ventilation is comparatively low-cost and low-embodied carbon:
• The hardware for an automated NV system typically includes window actuators, control panels, bracketry, framing for openings, weather stations and cabling
• An automated natural ventilation system typically costs around 20 to 50 USD / m² for the areas served1 This includes the hardware and the installation and commissioning of the system
• The whole life carbon of these hardware elements is fractional, typically around 0.1% of the project’s whole life carbon 1
The openings through which air enters and leaves carry much greater cost and embodied carbon. On this basis:
• It is optimal to consider how openings can be integrated with glazing that is functional for daylight and environmental quality. Where possible, integrate openings with glazing as it allows one feature to perform multiple functions (daylight, ventilation, environmental quality)
• Likewise, features such as atria and solar chimneys can be highly beneficial in expanding natural ventilation across large parts of the building but are best utilised and adapted where they would exist independently of the needs of the natural ventilation system
1 Based on Introba project experience
• Designers should think carefully about the potential payback of major architectural interventions such as double-skin walls and ideally carry out preliminary whole life carbon (WLC) calculations early on to guide these decisions. In some cases, the double skin may be required to control wind, but quite often these interventions provide limited payback in heating and cooling energy saving
Heat loss through leakage is another consideration, particularly in colder climates. While actuated systems can achieve an effective seal, many types of automated opening can increase infiltration and may cause drafts.
Natural ventilation systems should consider how to be as economical as possible. For example, use the stack effect and/or harness the wind energy to achieve the target airflows from fewer openings and architectural interventions.
The detailed design of NV openings in facades needs to take due consideration of other design criteria such as fire, acoustics, security and safety. The position, size and detailing of opening vents can have a significant impact on facade costs and must be considered with an appropriate specialist.
Finally, natural ventilation achieves more than just energy and potential carbon savings. It is hard to put a number to the health benefits and resilience of natural ventilation.
In buildings such as Vienna House2 , in Vancouver, Canada where mechanical systems can be reduced through the application of natural ventilation, there is a much more tangible payback regarding overhead costs, bills, and whole life carbon.
2 https://viennahouse.ca/
University of Waterloo Academic Building—Natural Ventilation Schematic
1. Outdoor air enters directly via automated windows
2. Acoustic ducts allow airflow without noise transfer
3. Used air rises in the solar chimney
4. Direct sun provides additional bouyancy
5. Additional pressure is generated by wind at chimney exhaust
6. Perimeter offices enjoy single-sided natural ventilation
This page: Natural ventilation schematic of University of Waterloo Academic Building, Ontario, Canada © IntrobaFigure 1: Whole Life Carbon (WLC) analysis of three alternate natural ventilation options for The University of Waterloo Academic Building.
1. WLC includes initial embodied carbon emissions of NV hardware and additional glazing components.
2. Reduction in WLC over time resulting from cooling energy savings (and heating energy savings for double-skin facades)
3. Spike in whole-life carbon at 25 years because glazing components are replaced. Note, the NV hardware component replacements at 12.5 year intervals have minimal impact. Note this calculation is representative of one building in one location - although insights may be transferable, we recommend conducting a WLC study on each project to verify outcomes.
An Introba project that exemplifies the “simple approach” is the University of Waterloo Academic Building in Ontario, Canada, designed by Moriyama Teshima Architects. With a total floor area of 12,500m2, roughly one quarter of the building will have natural ventilation as part of a mixed-mode system. The project is targeting completion in 2025. What makes the strategy simple is twofold. First, there are few moving parts; air enters via windows at the lower levels, and air exhausts via the solar chimney which illuminates the central, feature stair.
Second, only a ‘light touch’ was required to incorporate the system. Air movement is achieved through pressure generated by the architectural features - windows, corridors, atrium, and solar chimney - which were required in any case to provide views, daylight and circulation.
In other words, the strategy eschews costlier features such as double-skin facades or dedicated chimneys which are often specified as part of natural ventilation systems, but which can add significant cost and embodied carbon.
A key component of making the system economic is the utilisation of both wind and stack effect to generate airflow. Spaces which utilise natural ventilation are connected to the central atria and chimney, which collects warm, used air. Acoustic attenuators were included to allow air to transfer from the back of classrooms through to the chimney. A fire shutter is also used to maintain separation requirements while allowing free movement of air. The system will be automated, responding dynamically to internal and external conditions to ensure appropriate airflow for comfort, humidity and air quality.
Per Figure 1, Introba and Eckersley O’Callaghan carried out a whole life carbon (WLC) analysis of three alternate natural ventilation options over 50 years. The analysis compares natural ventilation strategies with different levels of architectural intervention and corresponding operational carbon savings, compared to a sealed baseline scenario.
At the simplest end, manually operated windows serve perimeter spaces only. The cooling savings are calculated to be 20% of annual cooling in the spaces served. This option represents the roposed strategy for the case study building.
The second option includes a central chimney for exhaust, and an automation system controlling the natural ventilation. The cooling savings are estimated to be 50% of annual cooling.
The most complex option includes a double-skin facade at naturally ventilated spaces only. The double-skin increases
the hours of the year when natural ventilation can be used, by allowing pre-heat of air in suitable conditions. For this option, cooling savings are estimated at 50% and heating savings are estimated at 20%.
The analysis demonstrates that the option with a central exhaust chimney and automated control provides the greatest payback in terms of kgCO2e. The key takeaway is that while greater interventions such as double skin facades can save more energy, the additional savings don’t outweigh the carbon premiums. By contrast, over time, a simple, automated natural ventilation system pays off.
The whole life carbon savings of natural ventilatio has the potential to be far greater when it is used to extend the life or reduce the size of costly mechanical systems. This whole life carbon assessment approach also does not consider the many other benefits of natural ventilation such as increased occupant productivity.
Assumptions used in Natural Ventilation
Whole Life Carbon Calculation:
Cooling COP = 2.0, heating COP = 3.0
Grid carbon intensity based on 2023 = 0.09 kgCO2/ kWh
Glazing and curtain wall replacement period = 25 years
Natural ventilation hardware replacement = 12.5 years
Mechanical systems carbon excluded, conservatively
Life cycle stages included: A1-A5, B2-B7, C1-C4 and a third of D
Whether you’re a developer or a designer, and whether you’re at feasibility or construction, there is opportunity to consider how breathability might influence the financial, social, environmental and health impacts of development. The intention of this study was never to provide all of the answers. Instead, we hope reading the report will inspire conversations that shape the next generation of breathable buildings.
On this page, you’ll find resources to seek further help. We regularly use these industry guides to support our design of naturally ventilated spaces and low carbon buildings. You’ll also find contact details for the three primary authors of this report.
CIBSE AM10 Natural Ventilation in Nondomestic Buildings
CTBUH Natural Ventilation in High-Rise
Office Buildings
DAVID BARKER
Introba
Managing Principal
Melbourne, Australia
david.barker@introba.com
TOM DONALD
Eckersley O’Callaghan Director
Sydney, Australia tom@eocengineers.com
CIBSE TM65 Embodied
Carbon in Building
Services: A Calculation
Methodology
THOMAS BAMBER
Introba
Associate Passive Design
Vancouver, Canada
thomas.bamber@introba.com
STÉPHANY LE RHUN
Eckersley O’Callaghan
Global Sustainability Lead
Paris, France