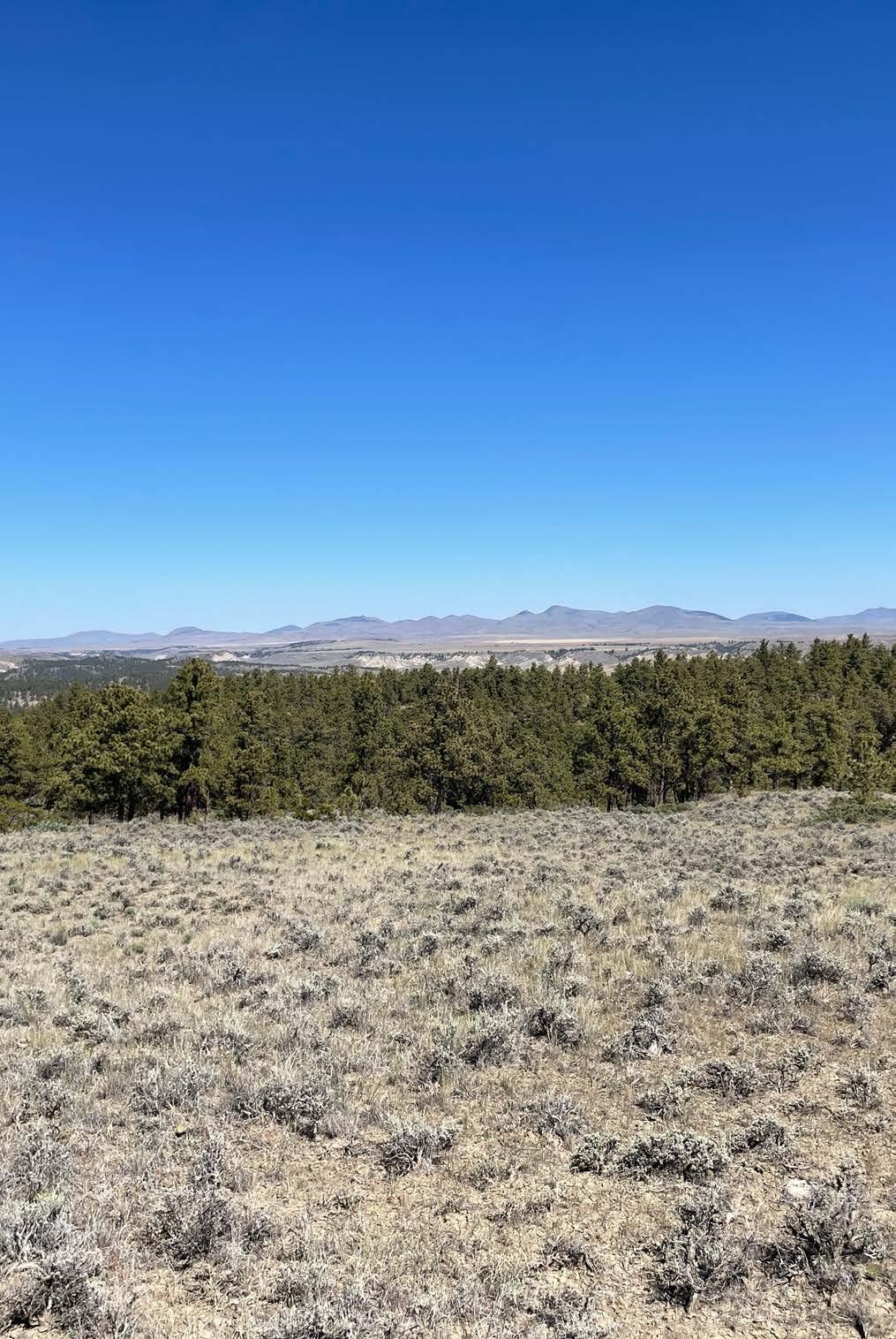
25 minute read
Understanding Circadian-Effective Lighting
from DEC 2022
By Randy Reid
Pursuing controversial topics is nothing new at designing lighting (dl).
In OCT 2020, we tackled whether lighting designers should be licensed or certified. In FEB 2021, we compared leading design software, all of which are popular with the specification community. Both articles were controversial but relatively well-received.
For this story, several industry experts either refused to comment or backed out last minute. More on that later.
designing lighting (dl) examines the three circadian methodologies in layman’s terms. Most designers do not specify circadian methodologies primarily for two reasons: it is extremely complicated, and the science is ongoing.
New data are still being generated by many labs throughout the world. First, we will share results of a survey regarding the industry's knowledge of circadian-effective lighting. Then, we will examine three methodologies used to measure circadian lighting. Lastly, we will discuss what can be done to come to a consensus on this issue.
SURVEY RESULTS AND COMMENTS
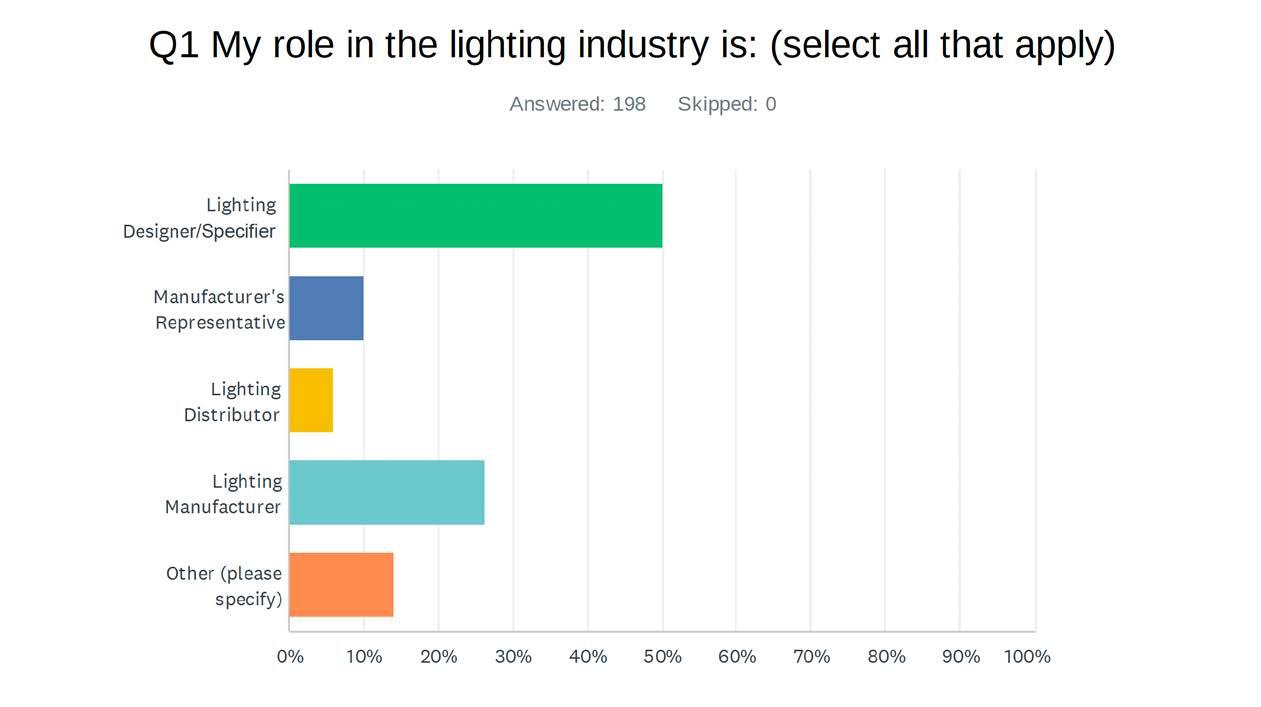
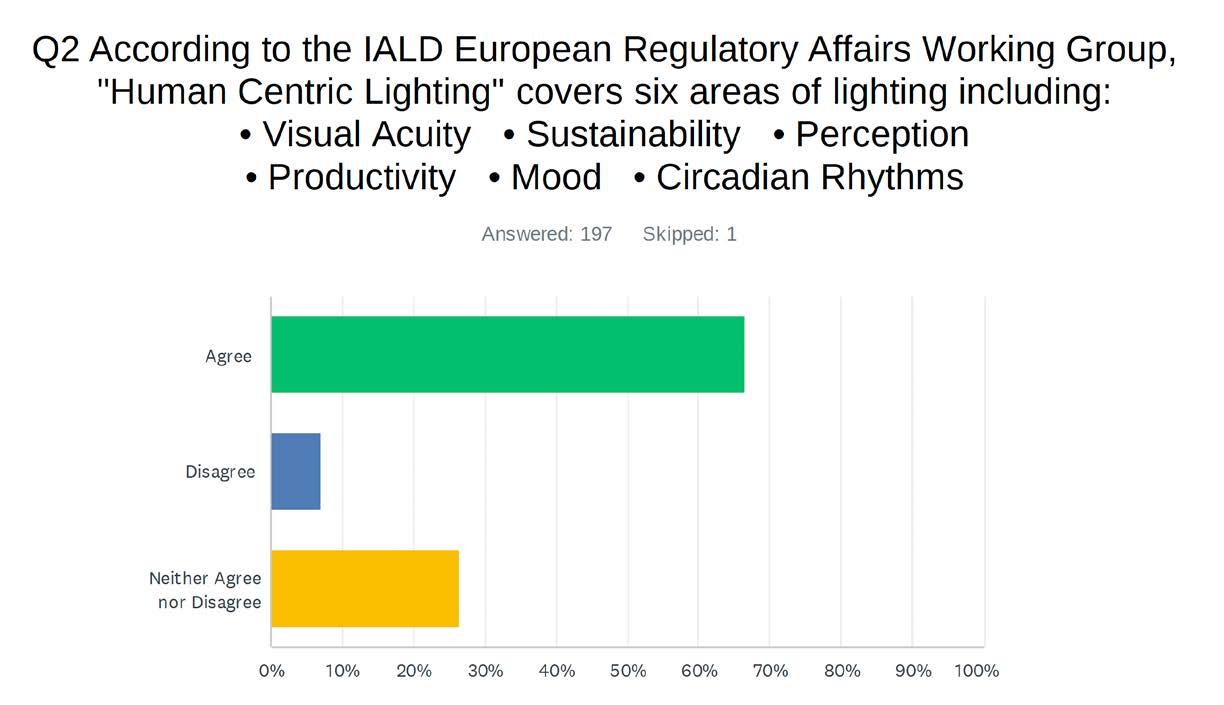
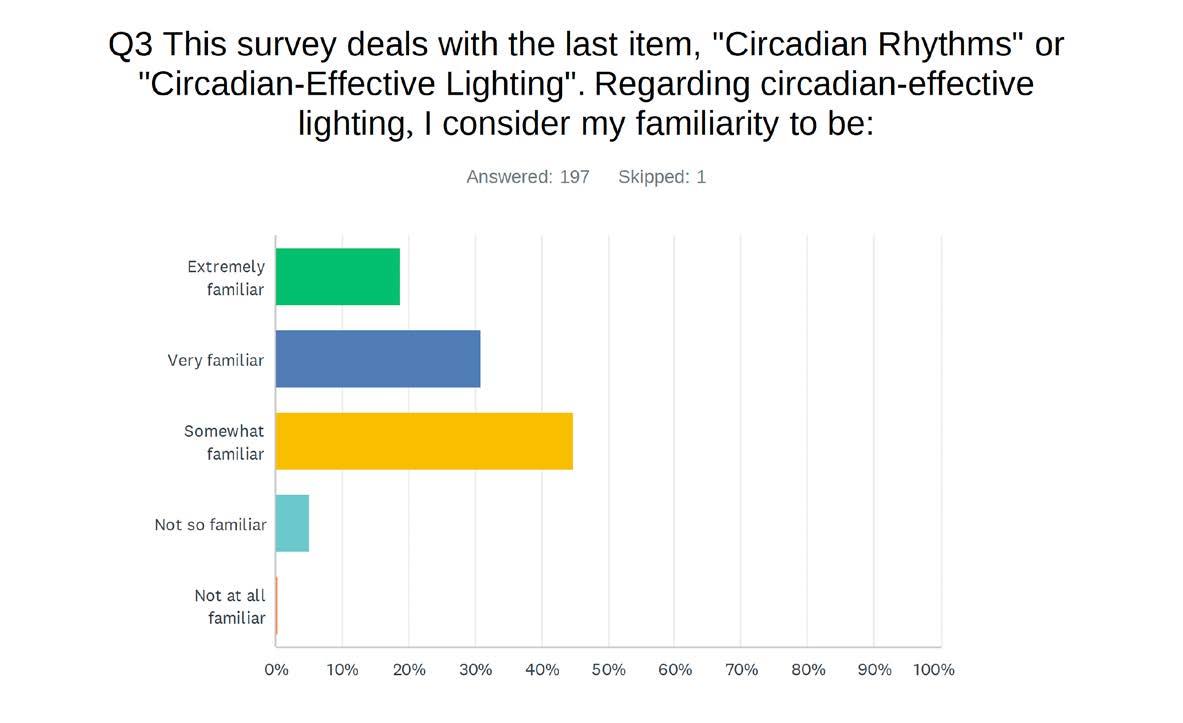

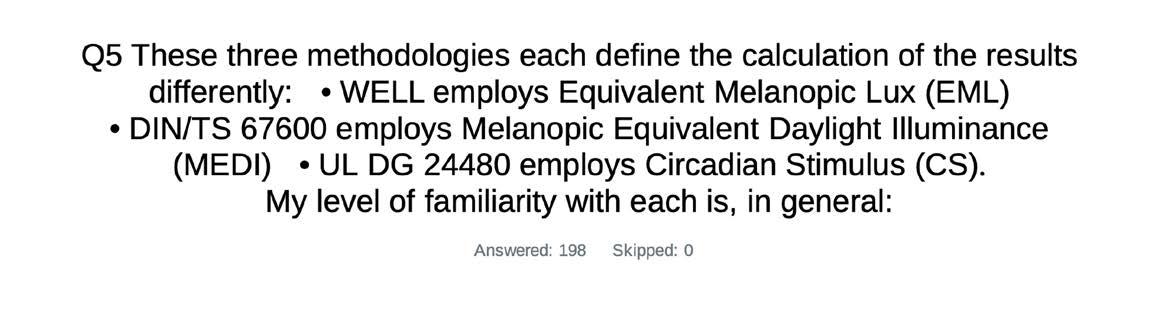
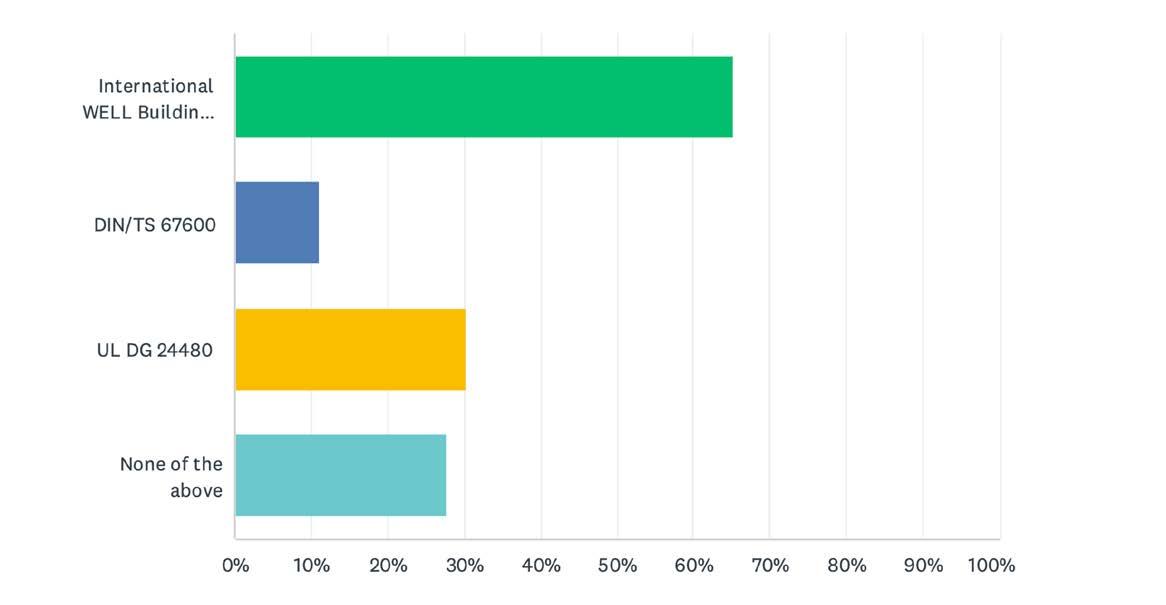
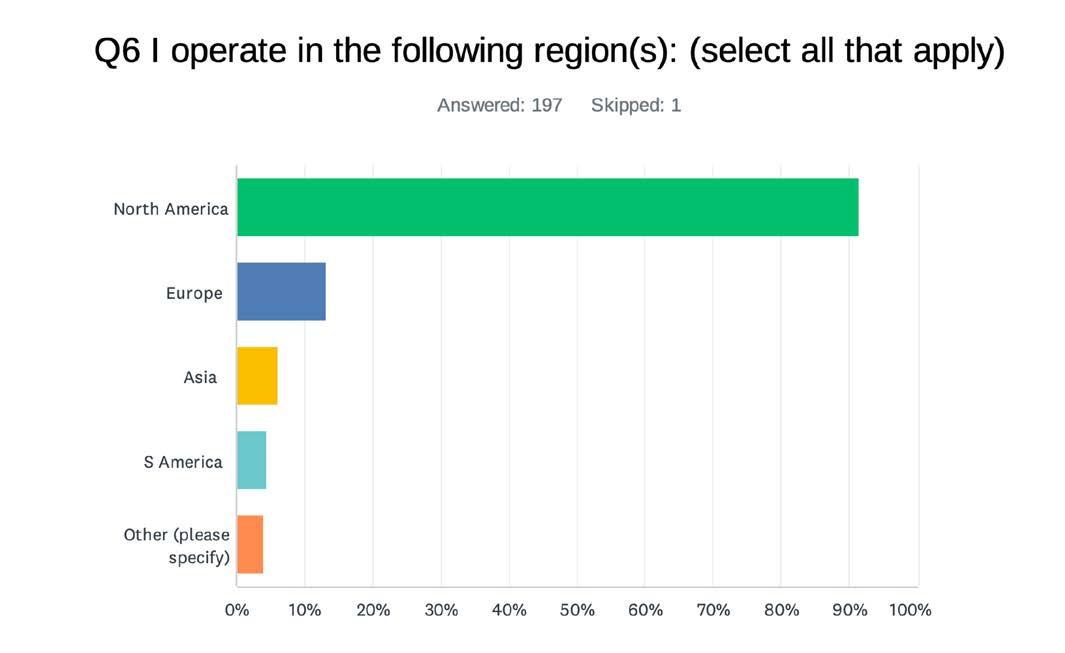
Melanopic Equivalent Daylight Illuminance (M-EDI) Explained
By Luc Schlangen, PhD, Human-Technology Interaction group and Intelligent Lighting Institute, Department of Industrial Engineering and Innovation Sciences, Eindhoven University of Technology, The Netherlands. Director, CIE Division 6 – Photobiology and Photochemistry
In 2018, the International Commission on Illumination (CIE) standardized spectral sensitivity functions and SI-compliant quantities that describe optical radiation for its ability to stimulate each of the five photoreceptor classes that can contribute to eye-mediated non-visual effects of light in humans. This new metrology is defined within international standard CIE S 026:2018 (1,2) and uses the shorthand α-opic to represent one of the five photoreceptor classes within the human retina: S-cone opic (α=sc), M-cone-opic (α=mc), L-cone opic (α=lc), rhodopic (α=rh) [rods] and melanopic (α=mel) [intrinsically-photosensitive retinal ganglion cells (ipRGCs)]. Under most practically relevant situations (i.e. long duration exposures to polychromatic light), the spectral sensitivity of circadian and neuroendocrine responses to ocular light exposure and, by extension, other related non-visual responses, can be well-described by the melanopsin-based spectral sensitivity of the ipRGCs (see Brown et al. (3)). The next section of this article will therefore only discuss the melanopic quantities of CIE S 026 in more detail. However, it’s worth noting that similar quantities can also be defined for the other four α-opic photoreceptors. As such, the α-opic metrology provides a suitable framework to develop multi-photoreceptor models of spectral sensitivity that eventually might yield a more accurate prediction of circadian, sleep, neuroendocrine, and/or cognitive responses to light as compared to the melanopic-only model as currently adopted in, for instance, the CIE Position Statement “Proper Light at the Proper Time” (4) and other recommendations for healthy indoor light exposures. (3,5)
Melanopic irradiance is a quantity that expresses the effective irradiance as detected by the melanopsin-based photosensitivity of ipRGCs. Melanopic irradiance is obtained by multiplying the spectral irradiance (E-e,λ (λ), i.e. the spectral power density per unit wavelength) as measured for a particular test light source (or test light condition) with the melanopic spectral sensitivity function (s-mel (λ)) according to:
melanopic irradiance = ∫ E e,λ (λ) · smel (λ) ·dλ [W/m2]
Recognizing the importance of daylight as a light source, the CIE chose CIE Standard Illuminant D65 as the basis for calculating further quantities for comparison. One lux of a light source with a spectrum that conforms to standard daylight with a color temperature of ~6500K (CIE Standard Illuminant D65) always corresponds to a melanopic irradiance of 1.3262 mW/m2, see Figure 1. This can be used to convert any melanopic irradiance (as measured for a particular test light/condition) into the corresponding melanopic Equivalent Daylight (D65) Illuminance (melanopic EDI) according to:
melanopic EDI = melanopic irradiance / 0.0013262 [lx]
Hence, the melanopic EDI represents the illuminance that provides an equal melanopic irradiance as the test light source/condition within an environment where all radiation conforms to CIE Standard Illuminant D65. The melanopic Daylight Efficacy Ratio (melanopic DER) is a dimensionless spectral characteristic of the light source that is defined by the ratio between the melanopic EDI and the (photopic) illuminance as measured for a particular test light/condition, according to:
melanopic DER = melanopic EDI / illuminance [-]
Stated differently, the melanopic DER of a source is equivalent to the melanopic Equivalent Daylight luminous output of the source (M) divided by its (photopic) luminous output (P). The melanopic DER hence represents a dimensionless “M/P” ratio, quite analogous to the S/P ratio (R-SP), an established lighting metric defined as the scotopic luminous output (S) of a source divided by its (photopic) luminous output. (6)
By definition, a light source that emits radiation that conforms to D65 has a melanopic DER of 1. When a test light has a melanopic DER of 1.25 or 0.50, this means that the test light has a melanopic activation (per lumen or lux) that is 25% higher or 50% lower, respectively, as compared to standard daylight D65. In general, light sources with a higher correlated color temperature (CCT) tend to have a higher melanopic DER, compared to sources with a lower CCT, (7,8) but it’s quite possible to adopt dedicated spectral tuning strategies to design light sources that combine a high melanopic DER with a low CCT, or vice-versa. (9-11) Finally, it is worth noting that any illuminance as produced by a given light source can be converted into the corresponding melanopic EDI by multiplying it with the melanopic DER of the light source.
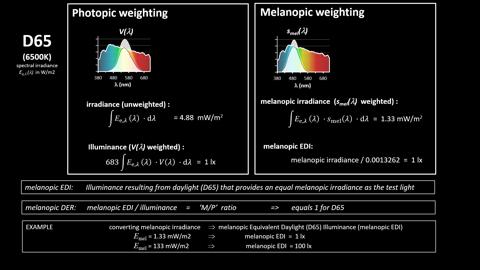
Figure 1. Schematic representation of the spectral irradiance of CIE standard illuminant D65 (rainbow area), the photopic and melanopic spectral sensitivity functions (V(λ) and s-mel (λ), plotted as dark grey areas) and the corresponding weighted spectral irradiances (white areas) and the melanopic quantities that result from this weighting.
Equivalent Melanopic Lux (EML)
Bright Light TO the Eyes - For Healthier and Productive Workplaces
By Nathan Stodola , WELL Building Institute and Shengliang (Daniel) Rong, Delos Living
Light Beyond the Visual Effect - Metrics To Quantify the Nonvisual Light Response
Historically, designers looked only at the light for visual purposes, largely concerned with how much light was reaching task surfaces. This was quantified using a series of “photopic” metrics, like illuminance, measured in lux or foot-candles. But, light also plays an important part in regulating our body’s sleep-wake cycle, impacting our biological and mental health. Integrating daylight and electric light to create lighting strategies focused on human health, along with traditional requirements for visual acuity and comfort, can lead to healthier and more productive workplaces.
Our body has a natural cycle of activity that lasts approximately one day (hence known as our circadian rhythm). Light is the primary, but not the only, signal our body uses to synchronize this cycle with actual day and night. This happens mainly through ‘novel photoreceptors’ discovered about 20 years ago, the ipRGCs. They are located in the eye, like our rods and cones, but are not used directly in vision.
Our visual system is most responsive to light in the green-yellow part of the spectrum. The ipRGCs, on the other hand, are most sensitive to blue-green light, with nearly no response in the red end of the spectrum at all. Given this drastic difference in light sensitivity between visual and non-visual systems, metrics quantifying visual responses like traditional visual lux are inappropriate for quantifying non-visual responses of the circadian systems.
Melanopic Metrics for Circadian Response Quantification
An initial approach for melanopic metrics was a unit dubbed “equivalent melanopic lux (EML)” in 2014 by Lucas et al. (1) In this approach, the traditional visual lux and equivalent melanopic lux are defined as equal for a light source that has the same output at all wavelengths.
More recently, this approach has been formalized by CIE, with minor modifications, as a part of an SI-compliant system of metrics in the International Standard CIE S 026/E:2018. (2) This new melanopic metric is called the Melanopic Equivalent Daylight Illuminance, M-EDI (D65). For this metric, visual lux and M-EDI (D65) are defined as equivalent under the 6500 K daylight spectrum. A recent review study (3) has shown that M-EDI (D65) provides a reliable prediction of human circadian responses in different light environments (from bright to dim).
M-EDI and EML can be calculated by multiplying the visual light levels by a ratio: Melanopic Daylight Efficacy Ratio (M-DER) for M-EDI, and Melanopic Ratio (MR) for EML. Since both melanopic metrics are based on the same physiological response, they are easily converted between each other: MR = 1.103 × M-DER and 1 M-DEI(D65) Lux= 1.103 EML. Both ratios need to be calculated based on the spectrum of the light source. More detail on those calculations can be found in this recent study. (4)
For example, consider a light source that can produce 300 (visual) lux on the measurement plane where the spectrum of the light source has an M-DER ratio of 0.6. In this case, the M-EDI(D65) would be 300 × 0.6 = 180 M-EDI(65) Lux. This means 180 lux of daylight at 6500 K (D65) is required to produce the same melanopic activation as 300 lux for this light source. In other words, this light is 60% (M-DER = 0.6) as effective at stimulating ipRGCs as daylight.
Lights with lower color temperatures (“warmer” lights) tend to produce relatively lower melanopic illuminance for a given lux output, since lower color temperature typically has less blue content. However, two light sources of the same color temperature can have significantly different circadian effects, so it is always best to calculate the ratio directly from the spectrum.
Design Considerations with Melanopic Metrics
Typically, lighting designers work with a horizontal plane that is the task surface, such as the surface of a desk or a hallway floor, since light strikes that surface and is then reflected into the eye. However, for circadian lighting, what matters is how much light reaches the eye.
Thus, for healthier and more productive workplaces, designers should aim to provide sufficient light stimulus at the vertical plane at the occupant’s eye level when making calculations and measurements.
A potential risk when increasing light levels in a room –particularly on the vertical plane – is glare. An efficient way to provide light without causing discomfort glare is using reflected light from vertical surfaces such as walls and dividers. In addition, architects and the client must select the proper materials for interior finishings to minimize the absorption of active blue light. If the finishes are warm-colored (browns, tans, oranges), they will reflect less of the blue light, resulting in the circadian light levels being lower than expected.
Regarding the specific light stimulus targets for design, a recent study (Brown, et al. (5)) recommend 250 M-EDI(D65) Lux (275 EML) throughout the daytime at the eye, measured in the vertical plane at approximately 1.2 m height (i.e., vertical illuminance at eye level when seated). This also represents the higher tier of achievement in the Circadian Lighting feature in the IWBI's WELL Building Standard.
Summary
Beyond vision, light has a non-visual impact on our physiology and behavior, including circadian rhythms, sleep, and alertness (both via circadian system–dependent and circadian system–independent routes), mood, neuroendocrine, and cognitive functions. Metrics quantifying visual responses are not suitable for quantifying non-visual responses. Hence, melanopic metrics like EML and M-EDI(D65) were developed and formalized in the CIE International Standard. Today, melanopic metrics have been well-validated by studies for their reliable prediction of human non-visual responses and have been adopted by the WELL Building Standard to inform proper circadian lighting design.
The Circadian Stimulus Model: What Is It?
By Dr. Mariana Figueiro, Director of Mt. Sinai’s Light and Health Research Center
The Circadian Stimulus (CS) model is a mathematical representation of the circadian phototransduction mechanisms in the retina. It is not a model of nocturnal melatonin suppression; thus, even though it was developed using data from melatonin suppression studies, it is intended to be useful in characterizing circadian-effective light, both night and day. In other words, nocturnal melatonin suppression is a convenient outcome measure used to psychophysically infer the characteristics of the retinal mechanisms providing neural signals to the biological clock in the brain. The CS model is grounded in the neuroanatomy and neurophysiology of the human retina and was shown in laboratory studies to be predictive of nocturnal melatonin suppression. Perhaps more importantly, in the application studies, the CS metric was shown to be predictive of clinically relevant outcomes, such as alignment of melatonin rhythms with sleep opportunities, (1) reductions in depression and agitation among persons with AD, (2-4) and better sleep at night for people working in offices during the day. (3,5)
The Circadian Stimulus Model: What differentiates it from other metrics?
From a scientific perspective, the CS model has the following virtues that differentiate it from any other metric. CS is the only metric that:
• Accurately characterizes the spectral sensitivity of the phototransduction mechanisms of the retina to narrowband and broadband (“white”) light sources that can stimulate the master clock.
In an intact retina, no single photoreceptor, specifically the intrinsically photosensitive retinal ganglion cells (ipRGCs), can be responsible for circadian phototransduction. Indeed, results from several electrophysiological experiments with vertebrates (6-8) show that, while ipRGC efferent axons are the main conduit of light signals to the master clock, ipRGC afferent dendrites receive indirect input from the more distal rods and cones. Therefore, a completely successful model of human circadian phototransduction must take into account all retinal photoreceptors and their supporting neural mechanisms and must be constrained by the known anatomy and physiology of the retina and brain, (9) including neural interactions under a wide range of operating conditions. (10,11)
• Accurately characterizes the operating characteristics of the phototransduction mechanisms of the retina from threshold to saturation that stimulates the SCN. All other attempts have used post hoc, multi-free parameter fits to the data.
Practical metrics must also consider, just like CS does, the amount of light incident on the retina as it affects the response of the circadian system from threshold, the first measurable response to light, to saturation, the maximum output of the system. These metrics must also reflect, if required, changes to the spectral sensitivity of the system with the amount of light incident on the retina. For example, a brightness metric would have to reflect the fact that the human visual system becomes relatively more sensitive to short-wavelengths as light level increases. (12) For the circadian system, once the spectral sensitivity of the biological clock is defined, it is then possible to generate a stimulus-response function, such as CS. Again, like our many visual responses to light, a single photopigment model cannot possibly represent the dynamic operating characteristics of the neural mechanisms underlying circadian phototransduction.
• Is grounded in the neurophysiology and neuroanatomy of the retina. All other attempts have oversimplified reality by assuming a single photoreceptor is responsible for stimulating the SCN and, moreover, they only attempt to characterize the spectral sensitivity of the phototransduction mechanisms, not their operating characteristics (i.e., the amount of light incident in the retina needed to activate the master clock).
As a result, the CS model can account for two important phenomena: (a) the higher threshold for circadian system stimulation for diurnal humans than for nocturnal rodents, and (b) the non-linear, sub-additive response by the human circadian system to combined wavelengths. That is, it can account for subadditivity where adding certain wavelength energy reduces the system response. This accounting can only be achieved by understanding the neurophysiology and neuroanatomy of the retina. It should be noted that the CS model was developed as a metric that could be used by illuminating engineers to quantify a benefit (e.g., circadian entrainment) and reliably deliver that benefit to occupants of architectural spaces. It was not developed for commerce; thus, it is not imperative that it be SI compliant.
The Circadian Stimulus Model: Final Thoughts
As a matter of professional pride, it should be important to embrace the fact that CS is the most complete and validated model available for mathematically characterizing the phototransduction mechanisms stimulating the master clock. From a practical perspective, however, none of the metrics, including CS, are that important. In the simplest possible terms, lighting specifiers and regulators have a social obligation to provide building occupants with bright days and dim evenings. This provides occupants with, like diet and exercise, a recipe for better productivity, well-being, and overall health. This social obligation can be easily achieved by delivering “white” light to the occupants’ eyes of at least 500 lx during the day and less than 50 lx in the evening without having to worry about the science at all. Finally, it should be stressed that amount and spectrum are only two of many important lighting characteristics affecting the circadian system response. Getting more light at the eyes at the right time and for sufficient duration in a comfortable and energy efficient manner is healthier than arguing about what metric to use.
IES
The IES has spent several years drafting a recommended practice. I asked Dr. Bud Brainard, professor at Thomas Jefferson University and Chair of the IES committee, when we might expect to see the RP. His response? “I have no idea.” Due to confidentiality agreements with the IES, Dr. Figueiro would not comment on her work with the committee, but we do know that she abruptly resigned from that committee earlier this summer. There has to be a reason for one of the leading global experts to resign from the IES committee that is writing the recommended practice in her field of work, and we hope to uncover that at a future date.
UL’s Position
UL wrote the Design Guide UL24480, where they followed the ANSI balanced committee process with representatives from academia, science, industry and the general public. The committee addressed over 100 comments publicly. However, UL does not appear to be tied to any one methodology. Although their DG is based on CS, it also includes information on EML (M-EDI did not exist at the time). At LightFair 2022, Adam Lilien, UL’s Global Business Development Manager, Connected Technologies – Lighting, displayed a working robot that measures all three methodologies.
Recently, based upon discussions with the LHRC and a peer-reviewed article now in press, UL created the world’s first circadian test lab and launched a Circadian-Effective Luminaire Performance Verification system. UL now receives luminaires from manufacturers and issues reports on circadian-effective scores utilizing all three methodologies. Lilien explained, “If the luminaire is white tunable, the manufacturer will get a matrix at 2700K, 4000K, and 6500K. If the manufacture wants to go with 4000K, they will receive a badge with their EML, CS and M-sEDI score at 4000K.
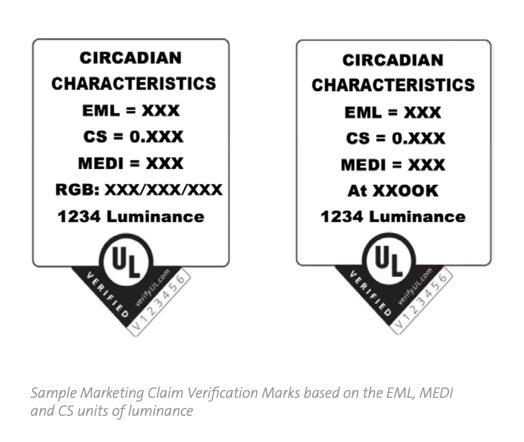
Lilien also conveyed that UL publishes a luminance score as a detriment to prevent companies from gaming the system. An illuminance meter will be aimed at the brightest part of the fixture and that number will appear on the badge. This will encourage designers to look for high circadian-effective scores with the lowest possible luminance score. UL will publish these results in an internal database, a wonderful tool for lighting designers.
Other Perspectives
Tony Esposito and Kevin Houser published a report, “Correlated Color Temperature is Not a Suitable Proxy for the Biological Potency of Light” in "Scientific Reports", which was published by "Nature Portfolio", last month.
It is well-written, but like much circadian content, it is highly technical. The authors lay out pros and cons of each methodology, but they did not use the most up-to-date Circadian Stimulus model. We applaud Kevin and Tony for furthering the conversation.
Questions:
M-EDI and EML are often lumped together, since EML can be easily converted to M-EDI with a simple multiplier. One leading and outspoken proponent of M-EDI refused my offer to write about it, saying, “I do not wish to participate in an exercise that creates a false balance between different types of entities.” He went on to say that “EML is an outdated, nonstandard concept that is not SI-conformant.” So, if M-EDI can be calculated from EML, what does that say about M-EDI?
Dr. Schlangen's discussion of M-EDI references that melanopic DER has a relationship to CCT and contrasts with the study published by Esposito and Houser that concludes CCT is not a suitable proxy for the biological potency of light.
One entrepreneur who regularly sells lighting to the senior living sector emailed me a case study which stated, “We installed lighting that is blue-enriched in the morning and changes to a warm color in the evening. This lighting protocol is designed to mimic the rising and setting sun with the intent of entraining the human circadian clock.” This same individual agreed to write an article but backed out at the last minute. In the case study, he doesn’t reference a specific CCT, but does say a “warm color,” which I assume to be 3000K or lower. Yet, the sun rarely gives us that level of warm color temperature, and never in the full sky. At sunset, I do see warmth, but only for a quick time frame, and it is only a sliver in the horizon. Assuming it is not cloudy, the remaining 90% of the sky is blue or white, making dusk typically about 5000K. In essence, nature never directs 2700K or 3000K into our pupils at sunset.
- Dr. Mariana Figueiro
What does blue-enriched actually mean? On a sunny day with a blue sky, the outdoor CCT is about 12,000K. Is the “blue-enriched” circadian lighting in the nursing home set for 12,000K in the mornings?
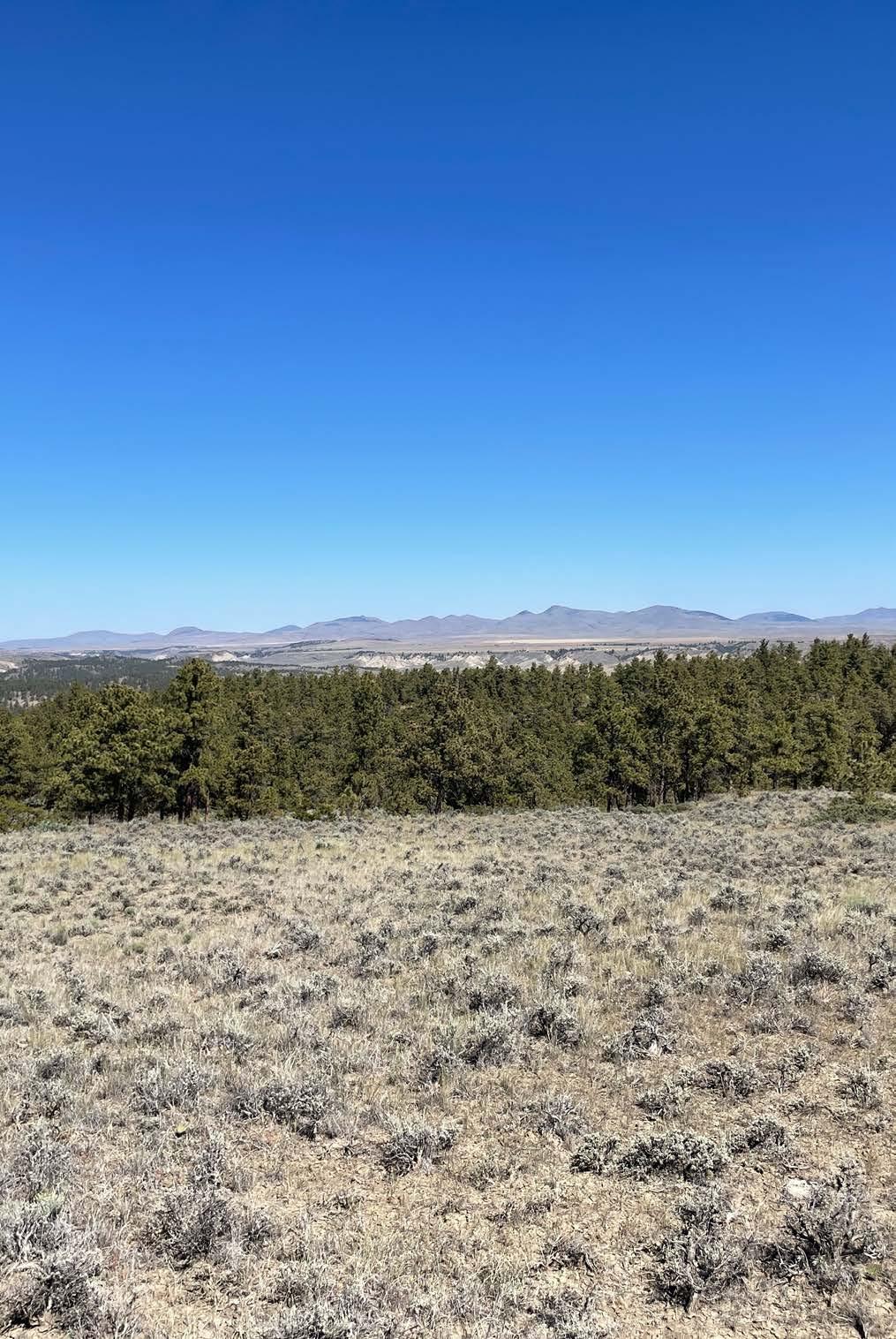
Montana skyline, 8 AUG 2022, approx 12,000K
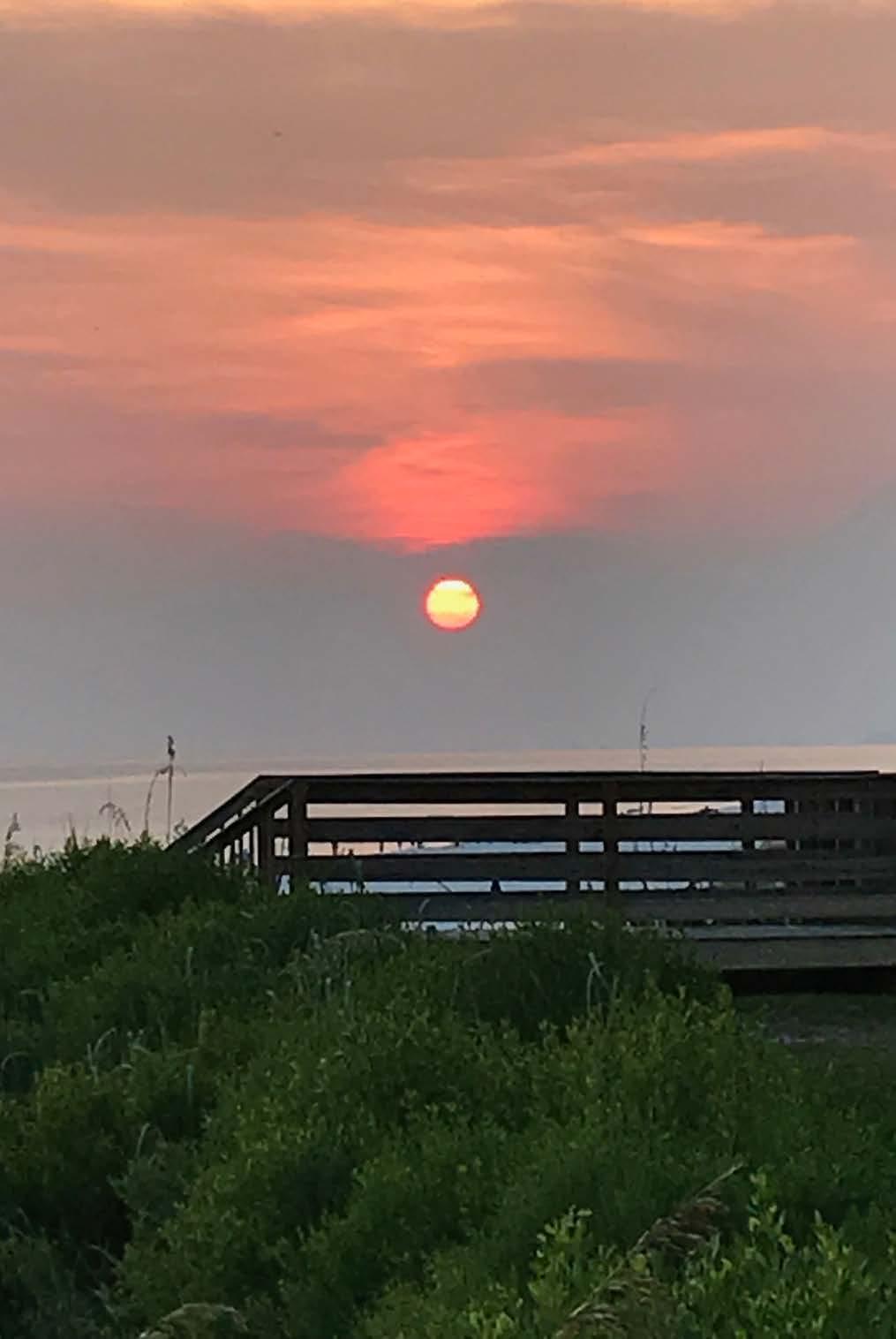
Santa Rosa Beach skyline, 15 DEC 2022, approx 5000K
EML and M-EDI refer to the light reaching the eye (or the light stimulus based on how it stimulates one’s photoreceptor, melanopsin-containing retinal ganglion cells). CS does that, too, but it goes one step further and considers how much light the circadian system needs (using melatonin suppression as an example of the response by the circadian system). As emphasized in the article by Figueiro, CS is not a model to predict melatonin suppression. EML and M-EDI seem to be component models; CS is more comprehensive. Think of them as a luminaire. You can look at the light coming from the luminaire or you can look at the effect the light has on the application. Which is more important? What effect does the light coming from the luminaire have on the floor, the ceiling, the desk and the people? It reminds me of the flawed UGR model for individual fixtures. How can you have a UGR number on an individual fixture without knowing the application. You can’t. Yet, organizations use these UGR numbers to approve rebates, and manufacturers have figured out how to game the numbers.
Since passions are so strong on this subject, let’s put the experts in a room for a day, debate all three methodologies and try to hammer out a true consensus. Let’s include all the experts and not sideline anyone, as appears to have happened to Dr. Figueiro.
But, the outcome of such a meeting should be a consensus on a formal hypothesis to determine which metric is better at predicting results. That’s the scientific method. Without such a “horse race,” it’s unlikely that well-entrenched opinions would change regarding which horse is better.
Who is in? ■
Editor’s Note: Mt. Sinai’s Light & Health Research Center is a regular non-paid contributor to designing lighting (dl) magazine. Randy Reid is the editor of dl, as well as the Executive Director of the National Lighting Bureau. The NLB and LHRC co-host a video series, “Let’s Talk about Light and Health.”
Melanopic Equivalent Daylight Illuminance (M-EDI) References
1. CIE, CIE S 026:2018 CIE System for Metrology of Optical Radiation for ipRGC-Influenced Responses to Light. doi: 10.25039/S026.2018. International Standard, available online via http://www.cie.co.at/publications/cie-systemmetrology-optical-radiation-iprgc-influencedresponses-light-0 . 2018.
2. CIE, CIE S 026 a-opic Toolbox. doi: 10.25039/ S026.2018.TB. Available online at: http://cie. co.at/news/launch-cie-s-026-toolbox-anduser-guide . 2020.
3. Brown, T.M., G.C. Brainard, C. Cajochen, C.A. Czeisler, J.P. Hanifin, S.W. Lockley, R.J. Lucas, M. Münch, J.B. O’Hagan, S.N. Peirson, L.L.A. Price, T. Roenneberg, L.J.M. Schlangen, D.J. Skene, M. Spitschan, C. Vetter, P.C. Zee, and K.P. Wright, Jr., Recommendations for daytime, evening, and nighttime indoor light exposure to best support physiology, sleep, and wakefulness in healthy adults. PLOS Biology, 2022. 20(3): p. e3001571, https://doi. org/10.1371/journal.pbio.3001571
4. CIE, CIE Position Statement on Non-Visual Effects of Light: Reccommending Proper Light at the Proper Time; 2nd Edition; October 3, 2019. Available online at: http://www.cie. co.at/publications/position-statement-nonvisual-effects-light-recommending-properlight-proper-time-2nd. 2019.
5. Vetter, C., P.M. Pattison, K. Houser, M. Herf, A.J.K. Phillips, K.P. Wright, D.J. Skene, G.C. Brainard, D.B. Boivin, and G. Glickman, A Review of Human Physiological Responses to Light: Implications for the Development of Integrative Lighting Solutions. LEUKOS, 2021: p. 1-28, https://doi.org/10.1080/15502724.20 21.1872383
6. CIE, International standard CIE S 017:2020 ILV: International Lighting Vocabulary, 2nd edition, electronic version (E-ILV) accessible online at https://cie.co.at/e-ilv. 2020.
7. Schlangen, L.J.M. and L.L.A. Price, The Lighting Environment, Its Metrology, and Non-visual Responses. Frontiers in Neurology, 2021. 12(235): p. https://doi.org/10.3389/ fneur.2021.624861
8. 8.Schlangen, L., S. Belgers, R. Cuijpers, B. Zandi, and I. Heynderickx, Correspondence: Designing and specifying light for melatonin suppression, non-visual responses and integrative lighting solutions – establishing a proper bright day, dim night metrology. Lighting Research & Technology, 2022. 54(8): p. 761-777, https://doi. org/10.1177/14771535221120350
9. Souman, J.L., T. Borra, I. de Goijer, L.J.M. Schlangen, B.N.S. Vlaskamp, and M.P. Lucassen, Spectral Tuning of White Light Allows for Strong Reduction in Melatonin Suppression without Changing Illumination Level or Color Temperature. Journal of Biological Rhythms, 2018. 33(4): p. 420-431, https://doi.org/10.1177/0748730418784041
10. Zandi, B., O. Stefani, A. Herzog, L.J.M. Schlangen, Q.V. Trinh, and T.Q. Khanh, Optimising metameric spectra for integrative lighting to modulate the circadian system without affecting visual appearance. Scientific Reports, 2021. 11(1): p. 23188, https://doi.org/10.1038/s41598-021-02136-y.
11. Esposito, T. and K. Houser, Correlated color temperature is not a suitable proxy for the biological potency of light. Scientific Reports, 2022. 12(1): p. 20223 https://doi.org/10.1038/ s41598-022-21755-7
Equivalent Melanopic Lux (EML) References
1. Lucas RJ, Peirson SN, Berson DM, Brown TM, Cooper HM, Czeisler CA, Figueiro MG, Gamlin PD, Lockley SW, O'Hagan JB, Price LL, Provencio I, Skene DJ, Brainard GC. Measuring and using light in the melanopsin age. Trends Neurosci. 2014 Jan;37(1):1-9. doi: 10.1016/j.tins.2013.10.004. Epub 2013 Nov 25. PMID: 24287308; PMCID: PMC4699304.
2. CIE, CIE S 026:2018 CIE System for Metrology of Optical Radiation for ipRGC-Influenced Responses to Light. doi: 10.25039/S026.2018. International Standard, available online via http://www.cie.co.at/publications/cie-systemmetrology-optical-radiation-iprgc-influencedresponses-light-0 . 2018.
3. Brown TM. Melanopic illuminance defines the magnitude of human circadian light responses under a wide range of conditions. J Pineal Res. 2020 Aug;69(1):e12655. doi: 10.1111/jpi.12655. Epub 2020 Apr 19. PMID: 32248548.
4. Esposito T, Houser K. Correlated color temperature is not a suitable proxy for the biological potency of light. Sci Rep. 2022 Nov 23;12(1):20223. doi: 10.1038/s41598-022-21755-7. PMID: 36418869; PMCID: PMC9684473.
5. Brown TM, Brainard GC, Cajochen C, Czeisler CA, Hanifin JP, Lockley SW, Lucas RJ, Münch M, O'Hagan JB, Peirson SN, Price LLA, Roenneberg T, Schlangen LJM, Skene DJ, Spitschan M, Vetter C, Zee PC, Wright KP Jr. Recommendations for daytime, evening, and nighttime indoor light exposure to best support physiology, sleep, and wakefulness in healthy adults. PLoS Biol. 2022 Mar 17;20(3):e3001571. doi: 10.1371/journal.pbio.3001571. PMID: 35298459; PMCID: PMC8929548.
The Circadian Stimulus (CS) Model References
1. Young CR, Jones GE, Figueiro MG, Soutiere SE, Keller MW, Richardson AM, et al. At-sea trial of 24-h-based submarine watchstanding schedules with high and low correlated color temperature light sources. J Biol Rhythms. 2015;30(2):144-54. doi: 10.1177/0748730415575432
2. Figueiro MG, Plitnick BA, Lok A, Jones GE, Higgins P, Hornick TR, et al. Tailored lighting intervention improves measures of sleep, depression, and agitation in persons with Alzheimer's disease and related dementia living in long-term care facilities. Clin Interv Aging. 2014;9:1527-37. doi: 10.2147/CIA.S68557
3. Figueiro M, Plitnick B, Rea M. Research Note: A self-luminous light table for persons with Alzheimer’s disease. Lighting Res Technol. 2016;48(2):253-9. doi: 10.1177/1477153515603881
4. Figueiro MG, Hunter CM, Higgins PA, Hornick TR, Jones GE, Plitnick B, et al. Tailored lighting intervention for persons with dementia and caregivers living at home. Sleep Health. 2015;1(4):322-30. doi: 10.1016/j.sleh.2015.09.003
5. Figueiro MG, Steverson B, Heerwagen J, Rea MS, editors. Daylight in office buildings: impact of building design on personal light exposures, sleep and mood. 28th CIE Session; 2015 June 28 – July 4; Manchester, UK: Commission Internationale de l'Éclairage.
6. Belenky MA, Smeraski CA, Provencio I, Sollars PJ, Pickard GE. Melanopsin ganglion cells receive bipolar and amacrine cell synapse. J Comp Neurol. 2003;460:38093. doi: 10.1002/cne.10652
7. Hattar S, Lucas RJ, Mrosovsky N, Thompson SH, Douglas RH, Hankins MW, et al. Melanopsin and rod-cone photoreceptive systems account for all major accessory visual functions in mice. Nature. 2003;424:75-81. doi: 10.1038/nature01761
8. Panda S, Provencio I, Tu DC, Pires SS, Rollag MD, Castrucci AM, et al. Melanopsin is required for non-image-forming photic responses in blind mice. Science. 2003;301(5632):525-7. doi: 10.1126/science.1086179
9. Kolb H, Fernandez E, Nelson RC, Jones B. Webvision: The Organization of the Retina and Visual System. Salt Lake City, UT: University of Utah Health Sciences Center; 2004. Available from: http://webvision.med.utah.edu/
10. Figueiro MG, Bierman A, Rea MS. Retinal mechanisms determine the subadditive response to polychromatic light by the human circadian system. Neurosci Lett. 2008;438(2):242-5. doi: 10.1016/j.neulet.2008.04.055
11. Rea MS, Figueiro MG, Bullough JD, Bierman A. A model of phototransduction by the human circadian system. Brain Res Rev. 2005;50(2):213-28. doi: 10.1016/j. brainresrev.2005.07.002
12. Rea MS, Radetsky LC, Bullough JD. Toward a model of outdoor lighting scene brightness. Lighting Res Technol. 2011;43(1):7-30. doi: