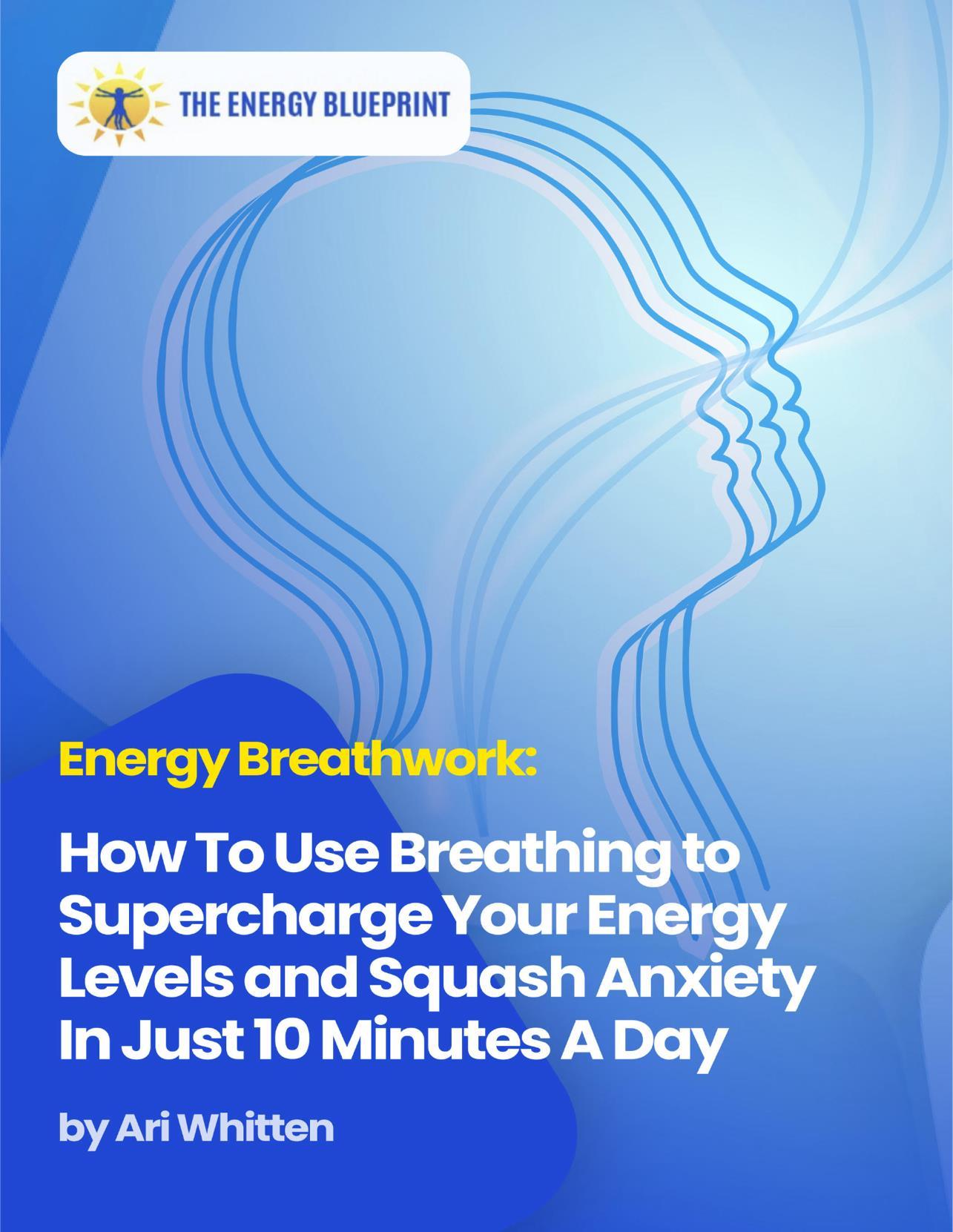
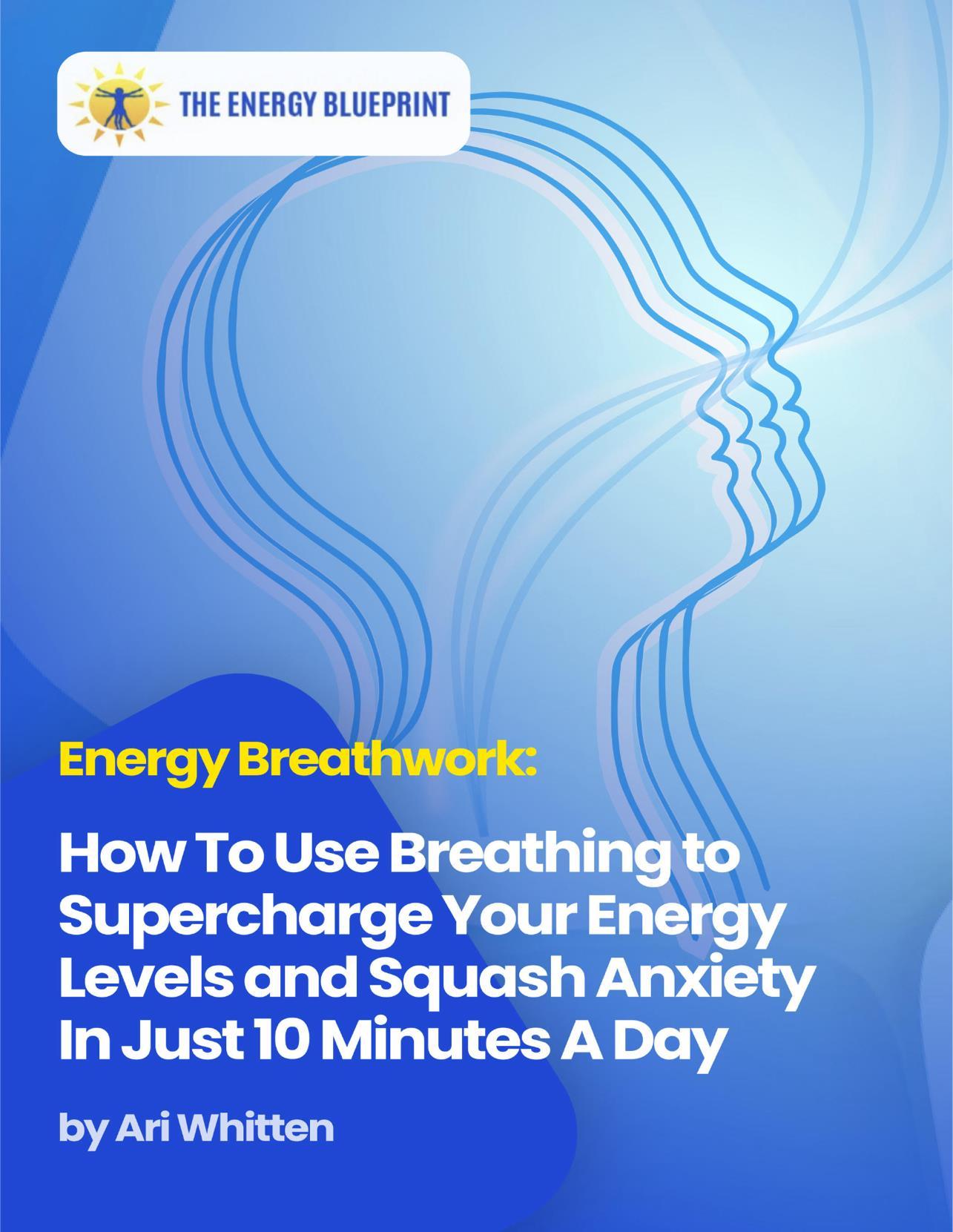
Energy Breathwork:
How To Use Breathing to Supercharge Your Energy Levels and Squash Anxiety In Just 10 Minutes A Day
In this e-Guide, you’ll learn:
● The 10-minute morning practice that is the single fastest way to increase your energy levels that I've ever found in over 25 years of studying health science
● The hidden epidemic of dysfunctional breathing causes fatigue and wires your brain into chronic stress/anxiety (and why more than 8 out of 10 people are not breathing optimally)
● Why supercharging oxygen delivery to your cells is the big key to high energy (and how to do it!)
● Powerful breathing hacks to decrease anxiety (including a method to dramatically lower anxiety within seconds)
● Why breathing is one of the biggest needle movers in improving health and energy levels, and is the single most underrated and poorly factor that few experts are even aware of
● How dysfunctional breathing wires your nervous system into stress/anxiety mode, and how to fix it
● The critical link between breathing and mitochondria (your cellular energy generators) and how a specific breathing routine can supercharge them
● Why resetting your CO2 threshold is the little-known hidden key to conquering anxiety and getting supercharging oxygen delivery to your brain (and the fastest way to do it)
About the author
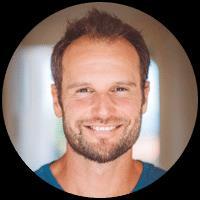
Ari Whitten, M.S. is the founder of The Energy Blueprint. He is the best-selling author of The Ultimate Guide To Red Light Therapy, and Eat For Energy: How To Beat Fatigue, and Supercharge Your Mitochondria For All-Day Energy. He has a Bachelor of Science in Kinesiology, certifications from the National Academy of Sports Medicine as a Corrective Exercise Specialist and Performance Enhancement Specialist, has completed extensive graduate training in Clinical Psychology, and holds a Master of Science degree in Human Nutrition and Functional Medicine. He was recently voted the top health influencer of the year by thousands of his peers in the Mindshare wellness/functional medicine community. Ari is a tireless researcher who has obsessively devoted nearly 3 decades of his life to the pursuit of being on the cutting edge of the science on health and human energy optimization. You can find his podcast, programs, and supplement formulas at www.theenergyblueprint.com.
Disclaimer: The content contained in this e-guide, is for general health information only and is not intended to be a substitute for professional medical advice, diagnosis, or treatment. Readers of this e-guide should not rely exclusively on the information provided in this e-guide for their own health needs. All specific medical questions should be presented to your own healthcare provider, and you should consult your physician prior to following any information from this e-guide.
Imagine you’re swimming in the ocean and a big wave is headed your way. You know you have to go under and hold your breath.
So right now, take a big breath of air. And hold it. (And continue reading).
You’ve now just initiated an ancient evolutionary program inside your body that, within mere seconds, will cause a series of remarkable physiological changes to unfold within the body. At first, As the seconds are passing, carbon dioxide is beginning to accumulate, which is sensed almost immediately by specialized receptors in the brain. The activation of these receptors then initiates a response from the sympathetic nervous system, which governs the body's stress response.
The sympathetic nervous system rapidly springs into action, releasing stress hormones such as cortisol and adrenaline. As cortisol and adrenaline course through your veins, your heart rate quickens, and your senses sharpen. The body senses the perceived threat of decreased oxygen and increased carbon dioxide levels, initiating a full-blown stress response. The pupils dilate, muscles tense, and your body prepares itself for action. Less immediately, more after at least one minute of breath holding, blood oxygen levels began to decline gradually.
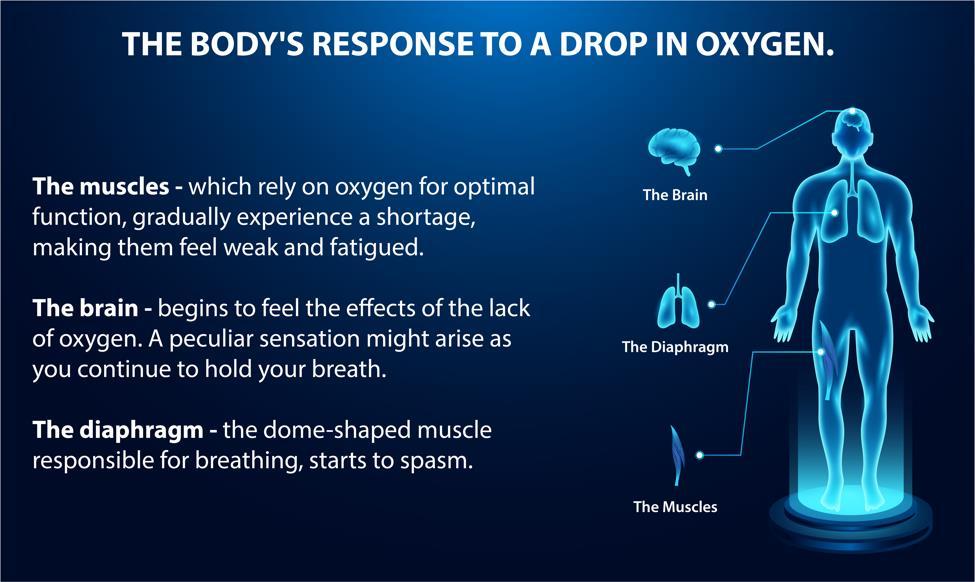
As oxygen is the life force that fuels our muscles and brain, this drop in oxygen triggers a cascade of events.
The muscles, which relied on oxygen for optimal function, gradually experienced a shortage, making them feel weak and fatigued.
The brain begins to feel the effects of the lack of oxygen. As you continue to hold your breath, a peculiar sensation might begin to arise.
The diaphragm, the dome-shaped muscle responsible for breathing, starts to spasm.
This involuntary spasm is the body's way of signaling to you that it needs air urgently. Finally, as the limits of breath-holding approach, the body's survival instincts grow too overpowering to ignore. By now, your brain is probably yelling at you "Release, and let the air fill your lungs once more." You finally make it to the surface, and your brain, sensing the urgency, intervenes and forces a gasp for air.
In that exhilarating moment, fresh oxygen flooded their lungs, providing relief and replenishment to the oxygen-starved muscles and brain. As you exhale and take a gasp of air, a sense of calm washes over you. The body gradually returns to a state of equilibrium, the sympathetic nervous system eases its grip, and the stress hormones begin to subside.
You rest for a little bit, and all of a sudden, another wave is headed your way. Here we go again…
Within minutes of breath-holding, you’re now experiencing a surge in the hormone erythropoietin (EPO) from your kidneys, a hormone normally associated with altitude, which is responsible for stimulating the production of red blood cells. Within just minutes of breath holding multiple times, these crimson carriers of life multiply, increasing your body’s oxygen-carrying capacity. This repeats again and again until you finally make it safely back to shore.
You might think that you just went through a very stressful ordeal that was hard on your body.
But in fact, this drop in oxygen now triggers a remarkable dance of biochemical mechanisms to occur that is profoundly beneficial to your health. Within the energy generators of your cells, the mitochondria, a buildup of reactive oxygen species (ROS) or “free radicals” occurred. These ROS, like tiny sparks, set in motion a profound cascade of adaptations.
The rise of ROS activated an ancient molecule that is a master regulator of the response to low oxygen levels, known as hypoxia-inducible factor 1 (HIF-1). HIF-1, sensing the reduced oxygen levels, creates a metabolic switch to shift cellular energy production away from mitochondrial energy production (which requires oxygen) towards anaerobic glycolysis – which, although much less efficient, allows
for continued cellular energy production even with the deficit in oxygen supply, without the excessive reactive oxygen species (“free radicals”) that would be produced if the cell was still trying to use mitochondrial energy production. Another of its actions is to upregulate the production of peroxisome proliferator-activated receptor gamma coactivator 1-alpha (PGC-1α), the maestro of mitochondrial biogenesis, which then begins to conduct an ensemble of cellular changes to birth new mitochondria within your cells – your body’s way of better preparing itself for the next time it might encounter hypoxia (low oxygen levels).
With each breath-holding session, your cellular powerhouses multiply, strengthening your body's capacity for energy production. Furthermore, this heightened state of ROS also triggered a strengthening of the antioxidant response element (ARE). Sensing the need for future protection against oxidative stress, the body bolsters its defenses by fortifying the ARE. This shields your cells from potential harm and paves the way for enhanced resilience in the face of future stressors.
As you continue holding your breath again and again, the effects ripple beyond the cellular realm to the macro level. Your body sets in motion adaptations to trigger your lung capacity to expand, allowing for greater oxygen intake and exchange. Alterations occur at the delicate lung-blood interface, optimizing the transfer of lifegiving oxygen into your bloodstream.
Your cardiovascular system also undergoes remarkable adaptations over time, improving its ability to extract oxygen from the blood and deliver it to the working muscles and organs. Astonishingly, stem cells – the guardians of regeneration and anti-aging – also respond to the call. Within your body, the production of these remarkable cells surges by a staggering 50% within minutes of repeated bouts of breath holding. These powerful agents of renewal held the promise of healing and rejuvenation, capable of repairing damaged tissues and countering the effects of time itself.
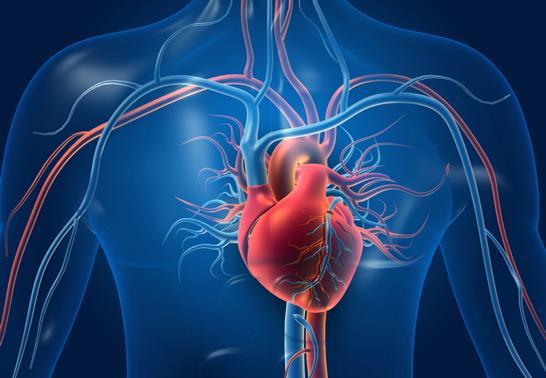
Together, all of these effects are now known to translate into: Increased energy levels, increased resilience to stress, reduced anxiety and depression, increased endurance and working capacity of the human brain, increased immunity and resistance to respiratory tract infections, generalized improvements in metabolic health and body composition (fat loss, improved insulin sensitivity, improved blood lipids, etc.), improved athletic performance, and widespread anti-aging effects.
This is the remarkable power of holding your breath.
As crazy as it might sound, that very stressful bout of holding your breath multiple times not only doesn’t harm you – but it actually translates into absolutely incredible benefits to your health, energy levels, resilience, and performance. Your body, as it turns out, is a remarkably intelligent, dynamic, adaptive system that is designed to transform stress into strength.
Hypoxic (low oxygen) therapy has actually been known about and utilized by humans for thousands of years. Hippocates, the ancient Greek physician (430-370 BC) sometimes advised ill patients to move to altitude to help get well.
Healing retreats at high altitudes in Europe have a rich history that dates back several centuries. These retreats were based on the belief that the pristine mountain air, combined with the unique environmental conditions found at high altitudes, held therapeutic benefits for various physical and mental ailments. The practice gained popularity during the 18th and 19th centuries, particularly among the European aristocracy seeking refuge and rejuvenation.
One of the earliest instances of high-altitude healing retreats can be traced back to the Swiss Alps.
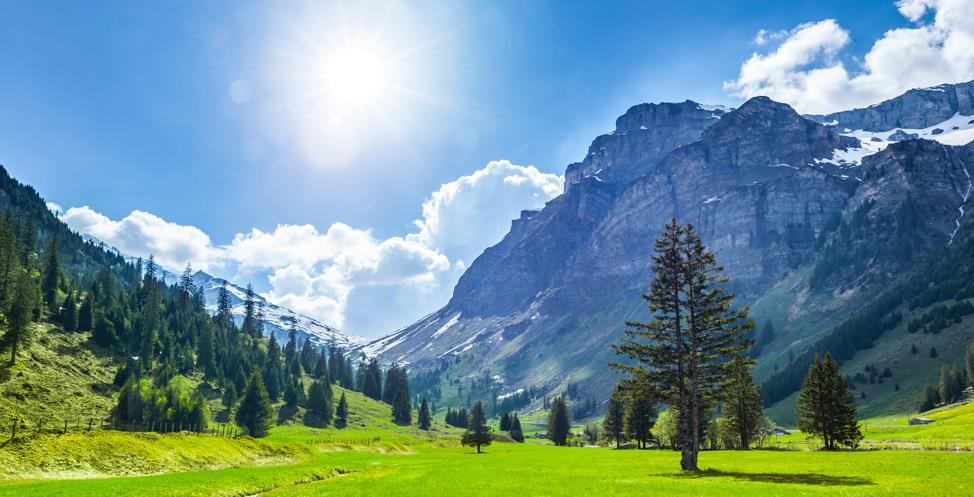
In the late 18th century, the Swiss physician Samuel-Auguste Tissot promoted the health benefits of the Alpine environment, advocating for its healing properties. Tissot's ideas sparked interest among the elite, who flocked to the Swiss Alps for rest, relaxation, and the purported healing effects of the mountain air. This led to
the establishment of numerous sanatoriums and health resorts in regions like Davos and St. Moritz, which became renowned destinations for the upper class seeking a therapeutic escape.
During the 19th century, the concept of "climatic therapy" gained momentum across Europe. Physicians and health practitioners recognized the potential of highaltitude environments in treating respiratory diseases, such as tuberculosis. The fresh, unpolluted air and increased oxygen levels found at higher elevations were believed to have a positive impact on lung function and overall well-being. As a result, sanatoriums and health retreats dedicated to treating respiratory ailments sprang up in the mountainous regions of France, Italy, Austria, and Germany. The early 20th century witnessed a surge in the popularity of high-altitude healing retreats.
During the 1980s, Russian scientists introduced the concept of simulating the effects of high-altitude by building chambers with low air pressure or alternatively, by simply inhaling gas mixtures with reduced oxygen content. This concept led to the development of intermittent hypoxic training (IHT). Over time, a substantial body of clinical and experimental evidence has been accumulated in scientific literature, demonstrating the effectiveness of IHT in various fields from training military pilots and pre-adapting soldiers to high-altitude operations, to combating anxiety and panic disorders, to enhancing sports performance in elite athletes, to the treatment of cardiovascular, metabolic, neurological disorders, allergies, bronchial asthma, diabetes, and other diseases.
Breathing is one of the most powerful, and the single most underappreciated and poorly understood factors in human health.
Most people never think twice about their breathing. Without any conscious awareness, our brain breathes for us, 24/7. Because of this, most people (including most health experts) never suspect that anything unusual is going on with one’s breathing, or that it could be related to one’s state of health (or disease).
It turns out that not putting any effort into training your breathing habits (and imagining your breathing will take care of itself), is a lot like expecting your muscles to be strong without ever exercising them. Or expecting your brain to be smart without ever spending time learning anything. Like every other system of the body, if it doesn’t receive regular challenges, it atrophies and begins to dysfunction or at the very least, become much weaker.
As it turns out, we actually have a large body of evidence – unbeknownst to most experts and the general public alike – that breathing dysfunction is rampant in modern humans.
I’ve found that optimizing one’s breathing habits is one of the absolute most powerful needle-movers in recovering our health. I’d go so far as to say that the practices I’ll tell you about in this chapter are the single fastest and most powerful method I’ve found to help people struggling with chronic fatigue rapidly improve their energy levels.
How do we do this?
The most powerful method to optimize your body’s respiratory function is through the hormetic stress practice of “air hunger.” That is, deliberately breathing less air (or breathing hypoxic/low oxygen air using machines) and especially, using breath holding practices.
The science of how all this happens has to do with something called “intermittent hypoxic training.” Basically, all that means is occasional training of your body in low oxygen states.
More broadly, we can term these hormetic practices as “air hunger” training. It actually entails not only hypoxic (low oxygen) training, but also, very importantly, hypercapnic (high carbon dioxide) training – an important point we’ll talk about extensively that especially relates to the brain’s regulation of stress, fear, and anxiety.
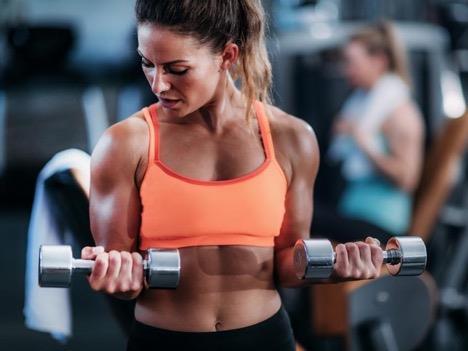
You can think of the respiratory system of our body much like the many other systems of our body in this regard. If you subject your muscles to challenges to lift heavy objects, they grow larger and become stronger. Challenge your body to run long distances, and your heart increases its capacity to pump blood, and your muscles shift their physiology to optimize endurance. Intellectual challenges make you smarter. Emotional and physical challenges develop mental toughness and grit. Subjecting your body to the heat and cold develops adaptations in the thermoregulatory systems of your body, to cool you in the heat and warm you in the cold.
Stress doesn’t make us weaker (as most people think) – it is the very basis of becoming stronger. Our body only becomes stronger when challenged.
The respiratory system is no different. Like the systems I just mentioned (and the larger macro system of the whole human body and brain!), the respiratory system is a dynamic, adaptive system that is constantly responding and adapting to the demands imposed on it (or atrophying, in response to the lack of demands imposed on it).
When you challenge it, it adapts by growing stronger.
But how does it adapt? And why would it be necessary to strengthen this aspect of human physiology? You might be wondering what benefits one might stand to gain by developing their respiratory system in this way.
Those are the questions we’ll answer in this chapter.
The short answer is that optimization of your respiratory system doesn’t only matter for breathing itself, but it turns out that our breathing systems are intimately connected with and related to our brain’s stress/fear-processing centers (which relate to anxiety and resilience), the way our muscles perform during activity, our overall metabolic health, and numerous anti-aging mechanisms built into our physiology. It also enhances universal stress-resistance mechanisms.
As stated by researcher Simon Basovich,
“Hypoxic training is not, however, a method of treatment a specific disorder. Rather, hypoxic training is a method to improve general resistance the organism…For example, the effects of hypoxic training (10% O2) on increasing the compensatory capabilities of organisms were researched [in] … asphyxia, acute hypoxia with hypercapnia, hemorrhagic shock, physical load, tick-borne encephalitis virus infection, and intoxication by 8 different poisons…All data show significant and reliable increase in compensatory capabilities after previous hypoxic training/preconditioning.”[1]
In other words, intermittent hypoxic training (training your body in low oxygen states) not only makes your body more resilient to low oxygen levels – it actually induces adaptations that make you more universally stress-proof to essentially all types of stress, from physical stress, to mental stress, to infections, to toxin exposures.
So optimizing your breathing isn’t just a matter of only breathing better – it’s something that stands to make you more immune to stress, increase your energy, improve your mood, improve your body composition, keep you metabolically healthy, increase your physical and mental performance, and ward off diseases associated with aging, helping you live to a ripe old age in good health.
The Incredible Benefits of Intermittent Hypoxic Training
In my 25 years of studying health science, I’ve found that Intermittent Hypoxic Training (breath holding or breathing air with low oxygen) is the single fastest way I've ever found to increase energy levels and build resilience in those with chronic fatigue.
What is IHT?
Basically, it’s systematic practices that induce low oxygen (and often high CO2) states in the body. There are various ways this can be applied. Some studies use physical chambers (where humans or animals are studied as they’re exposed to lower oxygen levels) or wearing masks where a person breathes a lower oxygen air mixture. But low oxygen states can also be induced through breath holding practices (sometimes referred to as either “apnea” or “voluntary hypoventilation”). And with breath holding practices (in contrast to chambers or masks), one also profoundly increases their CO2 levels in the blood, which also has profound benefits.
The Buteyko Breathing Method (BBT) also uses “air hunger” practices which also reduce one’s air intake, but these practices – in contrast to breath holding practices – are much more subtle in their ability to induce hypoxic (low oxygen) states, but are largely used to produce hypercapnia (higher CO2 levels). The practices of BBT and true hypoxic practices have overlapping and synergistic benefits. Both fall into the category of using “air hunger” to induce adaptations in the body.
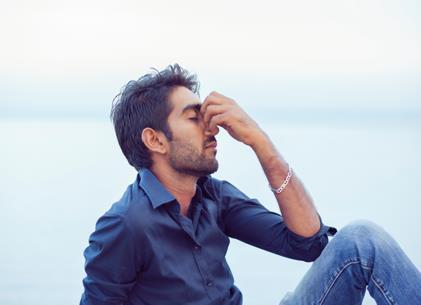
Fundamentally, IHT is using breathing techniques (or technology) to induce low oxygen levels and also high CO2 levels in the blood and low oxygen levels in the cells and the mitochondria to progressively train the body to extract and utilize oxygen more efficiently.
The benefits of intermittent hypoxic training (IHT) are vast. It:
1. Raises your CO2 tolerance in the brain (improving nervous system function and reducing anxiety).
2. Stimulates adaptations at the mitochondrial level that improve cellular energy production.
3. Improves gas exchange and utilization.
4. Increases oxygen-carrying capacity of the blood.
5. Enhances athletic performance.
6. Increases mental toughness and calmness under stress.
IHT gives us incredible benefits with regular practice – increased energy levels, lowered anxiety, resilience, improved physical performance, among many other benefits.
All of these effects on energy levels, mood, brain function, and athletic performance are the result of the adaptations the body makes in response to this transient “stressor” of raising CO2 levels and lowering O2 levels in the body.
Now let’s take a look at the specific adaptations the body makes to this kind of practice.
The Body’s Adaptations To IHT
IHT leads to numerous physiological adaptations that are first and foremost, designed to help the body better tolerate and survive low oxygen states without incurring damage. But interestingly, these adaptations also increase the overall universal stress-resistance of the body to a wide variety of stressors. This has to do with the phenomenon of cross-adaptation (common with hormetic stressors) where adaptation to one form of stress enhances resistance to a wide variety of other stressors different from the one that induced the adaptations. And it does this while also creating improvements in overall metabolic health and engages numerous antiaging mechanisms that combat age-related diseases and likely extend longevity.
So here, let's talk about some of the hormetic mechanisms behind how this works.
1. Improved oxygen
extraction, lung capacity, oxygen-carrying capacity, and oxygen utilization
One of the most remarkable things that IHT practices do is to mimic being at altitude.
Remarkably, within minutes of doing these practices, the body increases the secretion of erythropoietin (EPO), which is a hormone that we produce when we're at altitude that stimulates the body to produce more red blood cells. So within a few minutes of doing this practice, you can actually boost the amount of red blood cells in your blood and your hemoglobin levels so that your blood can now carry more oxygen. [46]
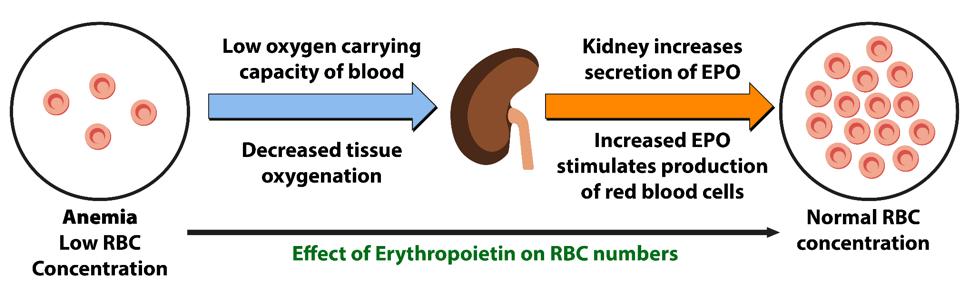
First, it trains your blood to extract oxygen more efficiently from the lungs.
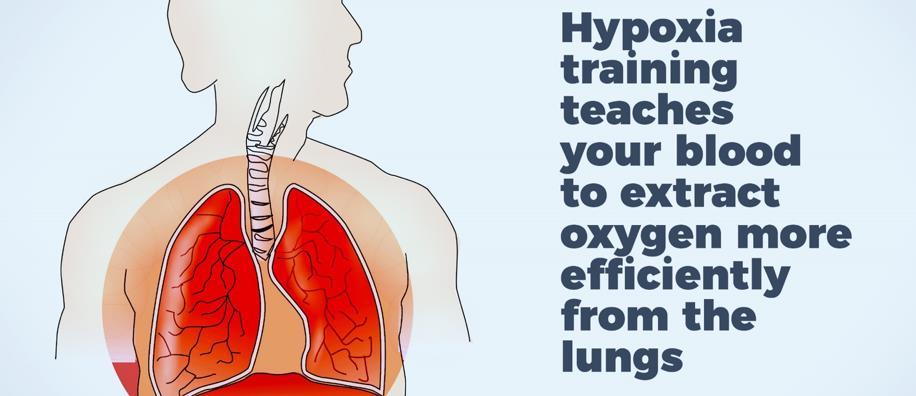
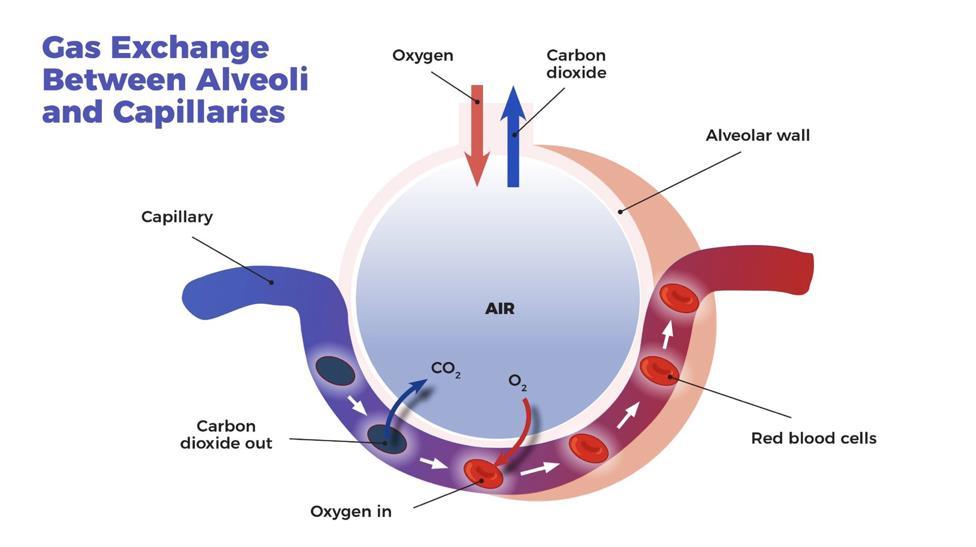
It actually physically alters the interface around these little alveoli, these little sacs of air in your lungs and the tiny capillaries that run around them. It structurally alters that interface to create more surface area, to allow for more efficient gas exchange, so you can get oxygen from the lungs into the blood more efficiently. Why would it do that? Well in the state of low oxygen during the breath holding the body is basically saying “we need more oxygen.” So that's one of the things that it's doing to get more oxygen into the system – actually modifying this interface between the lung and blood.
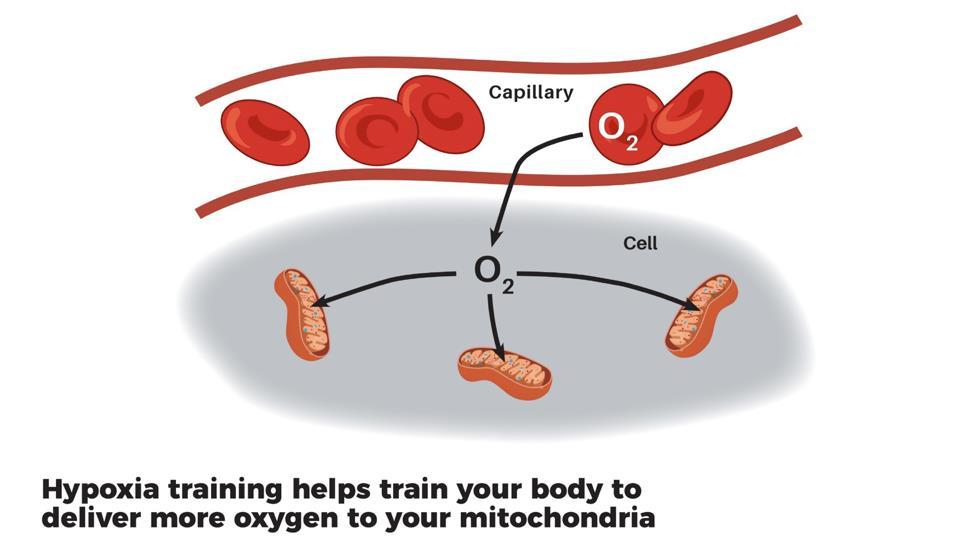
It's also modifying the interface between the blood and the cells where that oxygen needs to get delivered to.
And so your cells learn, they're being trained, how to extract oxygen from the blood more efficiently. Hypoxia, low oxygen levels also trains your mitochondria inside your cells to be protected from low oxygen states. That is, it makes them more stress-resistant. [47]
Together, these mechanisms – the lung blood interface, the lung cell interface, and increased oxygen-carrying capacity in the blood – we're training these different layers of our physiology to extract and utilize oxygen more efficiently.
In addition to the direct mechanisms involved in oxygen extraction, blood oxygencarrying capacity, and oxygen utilization by the mitochondria, there are several other mechanisms at play in how intermittent hypoxic and hypercapnic training increases our physiological resilience. One of the most important of those is how it rewires the stress/fear-regulation centers of our brain and the regulation of our autonomic nervous system (“fight or flight” vs “rest and digest”).
2. Autonomic nervous system balance
Hypoxic training has been shown in numerous studies to affect the way our autonomic nervous system (sympathetic and parasympathetic) functions[48]. For example, research has shown it can help prevent autonomic imbalance from exercise and other types of stressors – reducing the increases in heart rate, blood pressure, and perceived exertion[49,50]. Major depression and anxiety disorders are frequently linked with increased sympathetic nervous system tone, so reducing sympathetic tone is likely to impact on these mental conditions[51]. In addition, Low heart rate variability (HRV) scores are often linked with depression, anxiety, and numerous chronic diseases. HRV is also a biofeedback tool used by athletes to guide their training – on days with lower HRV scores, they do light days or rest days, whereas higher HRV scores indicate the body is ready to perform (and be trained) at a high level. IHT also positively modulates heart rate variability (HRV) after stress – another important indicator of increased resilience[51,52]. As further evidence of adjusting the autonomic nervous system setpoints, studies have also shown that breath retraining alters the non-conscious breathing regulation by the brain in a way that corrects hyperventilation and reduces the number of breaths taken per minute, helping to restore optimal CO2 levels.[53] By increasing HRV and tamping down sympathetic (fight or flight) nervous system tone, IHT profoundly
increases our universal stress-resistance potential.
3. HPA axis changes
Hypoxic training, like exercise and several other types of hormetic stress acts to enhance adaptation to stressors in part by modulating the sensitivity of the HPA axis to a broad range of stressors. Essentially, we “tune” our hormonal stress response through regular exposure to stress, and hypoxic training seems to do this exceptionally well.[54] Basically, our body puts adaptations in place that prepare it to better handle (and be less damaged by) future exposures to the same stress.
4. Reduced inflammation
Inflammation is also now strongly linked as a contributing factor in depression, other types of mental illness, and numerous chronic diseases. It is also linked with accelerating the process of aging itself, which has been termed “inflammaging.” It is known that hypoxic training strongly reduces systemic inflammation.
5. Builds your mitochondria to increase energy production (and oxygen utilization)
Research on intermittent hypoxic training (IHT) and its effects on mitochondria has gained significant attention in recent years. There are several key dimensions to improving mitochondrial health:
1. Mitochondrial Biogenesis: Several studies have shown that IHT can promote mitochondrial biogenesis, which is the process of generating new mitochondria within cells. Hypoxia helps build your mitochondria – literally to grow bigger and stronger. It's stimulating the creation of new mitochondria from scratch. This is the basis of building a stronger cellular engine, with greater energy-production capacity. Stress-buffering capacity at the physiological level, is largely synonymous with energy production capacity. The size of your cellular engine (mitochondria) is the core of this.
2. Mitochondrial Respiration: IHT has been reported to positively influence mitochondrial respiration, which is the process by which cells utilize oxygen to produce energy. Studies have demonstrated increased oxygen consumption and improved respiratory capacity in response to IHT.
3. Antioxidant Defense and Redox Balance: Reactive oxygen species (ROS) are natural byproducts of cellular metabolism that, when produced excessively, can lead to oxidative stress and cellular damage. IHT has been shown to induce adaptive responses that help maintain ROS balance within cells, preventing excessive oxidative stress. IHT has been linked to the upregulation of endogenous antioxidant defense mechanisms. This includes the activation of antioxidant enzymes and increased production of endogenous antioxidants, which can help protect mitochondria from oxidative damage.
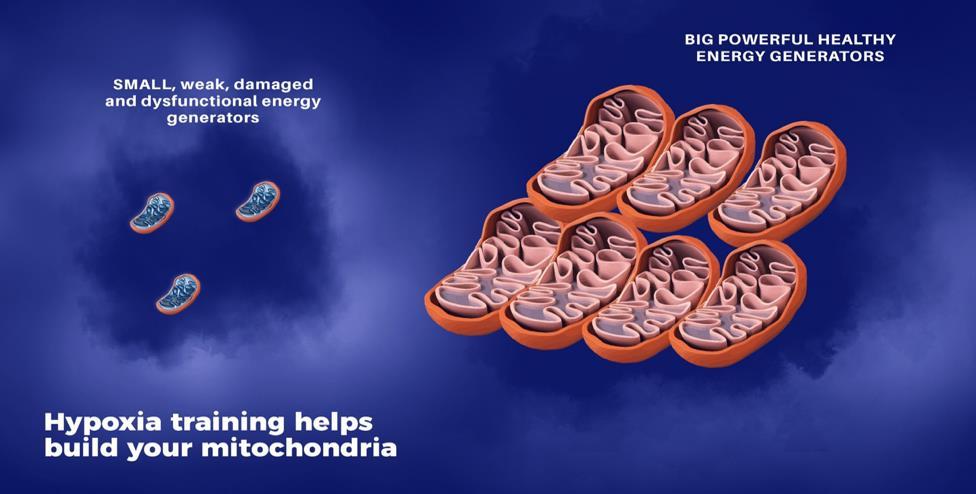
In one study where they looked at biopsies of mitochondrial tissues in rats that were subjected to intermittent hypoxic training, they showed profound changes in the actual structure of mitochondria. They called it “the phenomenon of micro mitochondria within mitochondria.”[55] They found smaller mitochondria almost layered into the bigger mitochondria – as an adaptation for those mitochondria to extract and utilize oxygen, to produce energy more efficiently.
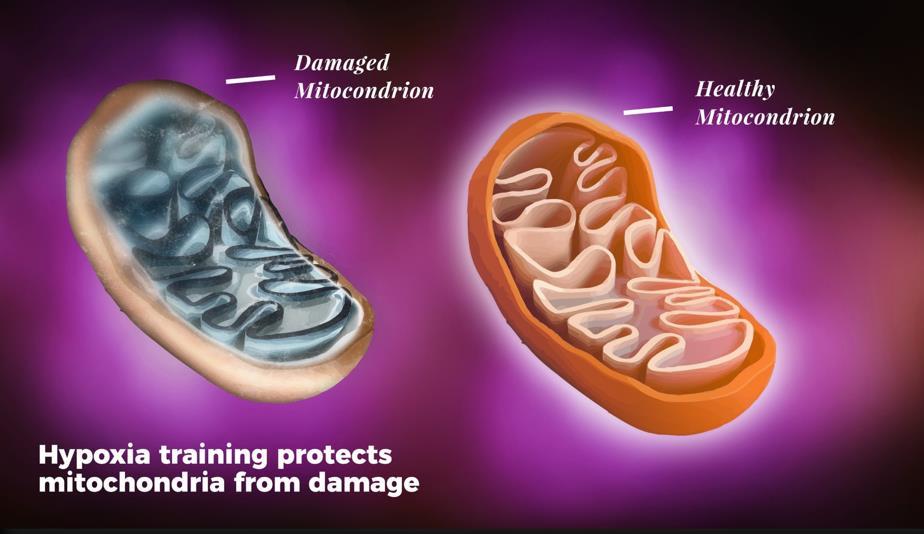
Some research has also shown that adding hypoxia to exercise training results in profound improvements in mitochondrial function. One study showed a remarkable 55.2% increase in mitochondrial density of skeletal muscle in the group that combined hypoxia with high-intensity interval training.[56]
Another study found a similar 59% increase in mitochondrial content in the group doing hypoxia with their exercise training, whereas the group that did the same training without hypoxia only increased mitochondrial content by 23.9%. [57]
Mitochondria are literally our cellular energy generators. So any strategy that can increase the amount of mitochondria in our cells by upwards of 50% is a massive effect. It’s the equivalent of going from a V6 engine in your car to a V8!
6. Increases CO2 tolerance in the brain (and rewires the stress response circuitry)
While all the aforementioned mechanisms are extremely important to the amazing benefits of IHT, where hypoxic and hypercapnic training really
shines is that it retrains our chemoreceptors in the brain and body (that sense O2 and CO2 levels in our blood), and thereby actually alter our CO2 tolerance.
We’ll discuss this in greater depth in the next section on mental stress, depression, anxiety, but here’s a quick rundown.
It’s a little-known fact that one’s “CO2 tolerance” (the degree to which the brain can tolerate higher levels of CO2 in the blood) is a major factor that ties directly into how stressed you feel and how much anxiety you have.
Our breathing ties directly into our nervous system. Specifically, our autonomic nervous system – the sympathetic (fight or flight) and parasympathetic (rest and digest) nervous system – and the stress/fear-processing circuitry of the brain.
The brain is also directly wired to feel anxiety when CO2 levels exceed the brain’s CO2 tolerance!
Here are some of the layers of evidence that allow us to see the connection between breathing and emotional/stress regulation in the brain.
1. Studies where individuals are asked to breathe air mixtures with higher CO2 levels, anxiety and panic increase rapidly in response to increased CO2 levels.
2. Numerous studies have found that depression and especially anxiety are associated with low CO2 tolerance. And that there is an extremely high prevalence of depression and anxiety in people with breathing disorders.
3. Just to complete this train of logic, we also know that breathing retraining practices that increase CO2 tolerance lead to reductions in anxiety. (Of course, it’s also important to note here that breathing retraining practices do indeed profoundly increase one’s CO2 tolerance, often with very minimal amounts of practice. So this is not some fixed trait that one has no control over – we can profoundly improve our CO2 tolerance with breathing practices).
Basically, when we have a low CO2 tolerance (as most people do), then it shifts our physiology such that our CO2 levels are frequently in excess of our personal CO2 threshold. When this happens, we’re wired to feel stress and anxiety. And when we can raise our CO2 tolerance (which we can do by implementing the breathing practices described in this book), we alter how our brain and central nervous system operate such that we lower our tendency towards anxiety.
Overbreathing → Low CO2 Tolerance → Increased Sympathetic Tone → Anxiety
Breath Retraining → Reduced Breathing Rate + Higher CO2 Tolerance → Decreased Sympathetic Tone → Reduced Anxiety and Improved Mood
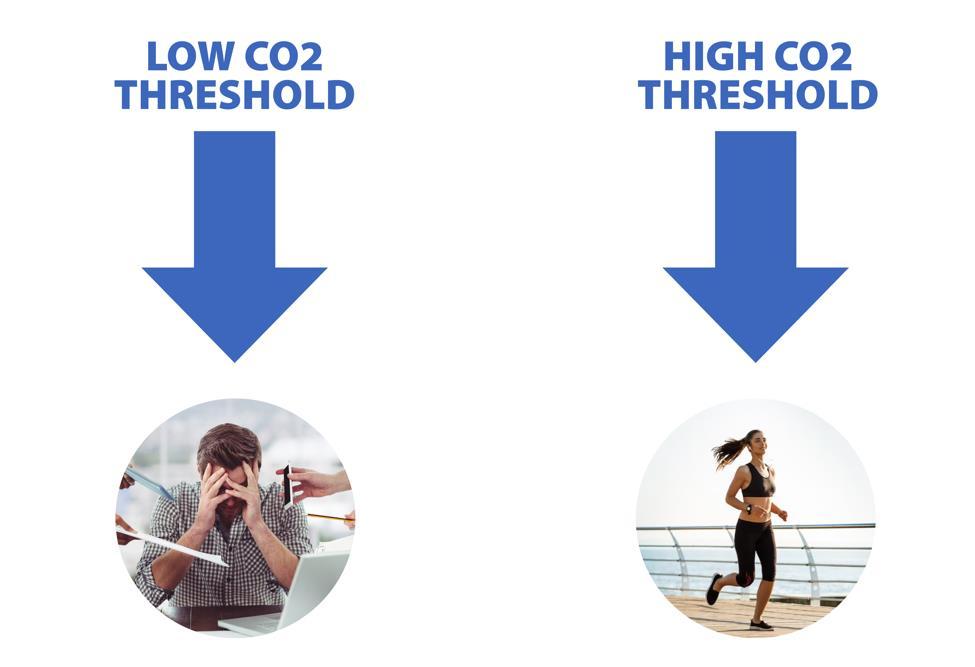
7. Makes it easier for oxygen to get into your cells
“All chronic pain, suffering, and diseases are caused by a lack of oxygen at the cell level.”
-Arthur Guyton, M.D. (The Textbook on Medical Physiology)
Perhaps the single most widespread myth and misconception about human physiology is the notion that carbon dioxide (CO2) is just a waste gas that we breathe out after our cells use oxygen. We all kind of have this idea that when we breathe, “we take in oxygen, which is the good stuff that our body needs,” and then “we produce this waste gas, carbon dioxide that we gotta breathe out and get rid of.”
Of course, there is partial truth to it. CO2 is indeed a byproduct of cellular energy production. And we do need to breathe out some carbon dioxide. However, it is simply not accurate at all to conceptualize CO2 as a “waste gas.”
CO2 is actually far more important in human physiology than most people realize. And it’s ultimately what controls how much you breathe, and hugely impacts how much oxygen actually gets to your cells…
Red blood cells have a compound on them called hemoglobin. Hemoglobin bonds to and carries oxygen. From the lungs, when we take a breath in, we bring in oxygen and then we have capillaries that wrap around little air sacs in our lungs, and that's where gas exchange occurs. Oxygen moves from the lungs into the blood by attaching to hemoglobin on our red blood cells.
So how does it detach? How does it disconnect from the red blood cell and actually get dropped off in the cells so it can be used to produce energy?
This is a critically important question, because if the oxygen is just floating around in our blood all the time, it doesn't do us any good. It doesn't help our cells, our organs, our muscles actually produce energy if it stays stuck to the red blood cells. To produce energy at the cellular level, we don’t just need abundant oxygen in the blood – we need that oxygen to efficiently and easily disconnect from hemoglobin on red blood cells and enter the cell.
So again, how does it know when to disconnect? What causes that bond between oxygen and hemoglobin on our red blood cells to weaken, so that oxygen can move from the blood to the cells?
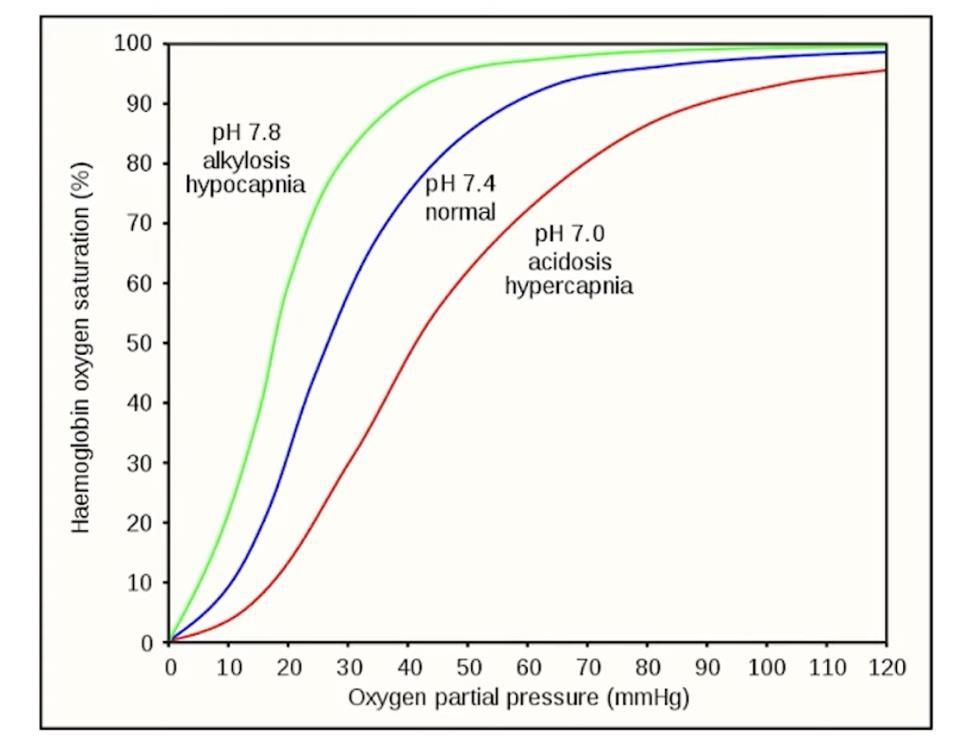
The answer: CO2!
Above you can see a chart of the degree to which oxygen will attach to hemoglobin. This shows that the detachment of oxygen from hemoglobin is a function of CO2 levels.
Let me take you through the above image so you can understand it. First of all, when there is no oxygen present (bottom left corner of the image), there will be no oxygen available to attach to hemoglobin, so hemoglobin will have no oxygen on it.
On the other extreme (the upper right hand corner), when there is extremely high levels of oxygen present (like in the lungs), hemoglobin will be fully saturated with all the oxygen that it can carry and that oxygen will be very tightly bound to the hemoglobin. That is, it is not releasing the oxygen.
But it’s really the middle part of this image that you want to pay close attention to – the range of about 30-40 mmHg partial pressure of oxygen. That’s the
physiological range where the magic is happening of needing to drop oxygen off at the cells.
So on the x-axis, find the number 30 (for 30 mmHg partial pressure of oxygen). Then imagine a vertical line straight up from there. Now look at the difference between the green line and the red line. At 30 mmHg O2, on the green line, the hemoglobin oxygen saturation is over 80%. But on the red line, it’s only about 30%!
The same exact partial pressure of oxygen is present. But when there is a difference in CO2 levels, there is a radically different force acting on the bond between hemoglobin and oxygen – either to keep them tightly bound, or for oxygen to detach from hemoglobin.
That’s the difference between most of the oxygen staying stuck on the red blood cells vs. most of it detaching from the red blood cells (where it can move into the cells and be used to produce energy).
The difference between the green line and the red line is CO2 levels. In the case of the red line, CO2 levels are much higher. This lowers blood pH, and the result of high CO2 (lower pH in the blood) is that the affinity of O2 to hemoglobin drops dramatically. The higher the CO2 levels, the more weak the bond between oxygen and hemoglobin gets.
In other words, the way that O2 detaches from hemoglobin on the blood cells (i.e. so oxygen can move from the blood into our cells) is in the presence of higher CO2 levels!
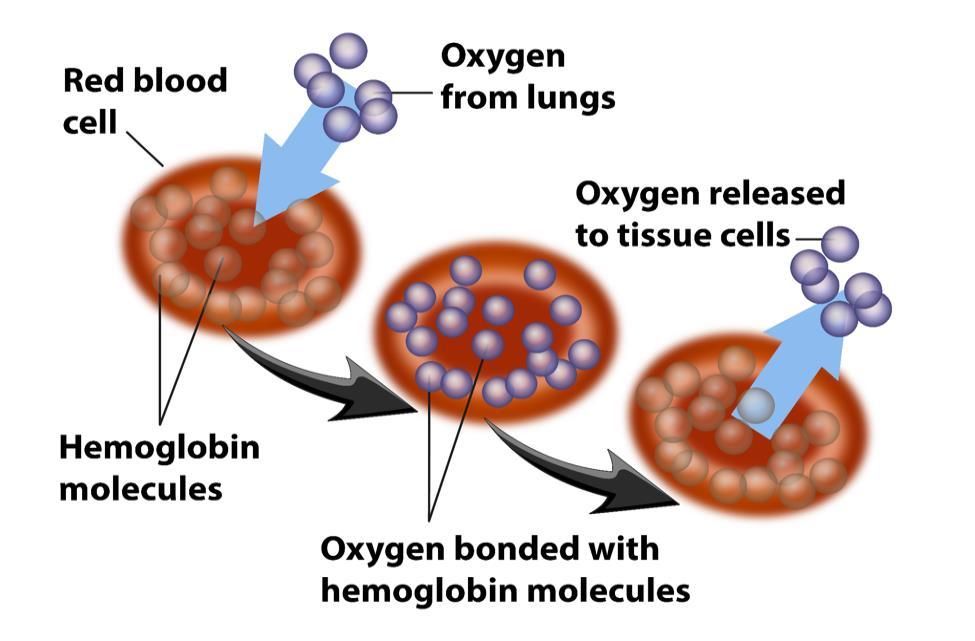
Carbon dioxide is essentially the doorway that lets oxygen reach our cells – our muscles, our organs, our brain. If the door is only partially open, then only some of the oxygen at our disposal (the oxygen that's there in the blood) will actually get delivered to the cells.
If the door is wide open and oxygen flows through the doorway, then we can deliver that oxygen to our cells efficiently. Think: energy! In order for your cells to produce energy efficiently, they need to be getting oxygen efficiently.
Just to make sure you understand how all this ties together, let’s go over how our body is designed to get oxygen, how it’s used, and the role that CO2 plays in all of this…
The very simple version is this: Oxygen is taken into our lungs, transferred to our arteries, and then delivered to the trillions of cells our body – from our brain, to our gut, to our heart, to our liver, our bones, our skin, our hormone-producing glands, our muscles, and so on – so that the mitochondria in those cells can produce energy, and so that those cells can do their job. When oxygen is not being efficiently or effectively delivered to those cells, those cells produce much less energy. When the trillions of cells in your muscles, your brain, your heart, your internal organs, your hormone-producing glands, etc. are not producing enough energy, they don’t do their jobs well. Brain cells not performing well leads to a poor performing brain, muscle cells with an energy deficit lead to poorly performing muscles. Same for heart cells, liver cells, thyroid gland cells, and so on.
So getting oxygen to your cells is critically important. And keeping higher levels of CO2 (rather than exhaling too much chronically) is critical for getting that O2 to your cells. CO2 helps O2 get into your cells.
The system must be tuned through training the breath (exercise and IHT are the two big keys) in order for CO2 tolerance to be optimal, and therefore for O2 delivery to the cells to be optimal. In short: Using IHT rewires your physiology in a way that allows more oxygen to flow into your cells 24/7.
What do all of these adaptations ultimately translate into? A whole host of amazing benefits…
Key Benefits of IHT
1. Improved overall metabolic health and reduced risk of dying from the major killers
First, it’s important to note that intermittent hypoxic training is a highly beneficial hormetic stressor. We know this definitively because it induces widespread systemic positive effects in virtually every physiological system of the body, while it also has profoundly beneficial effects on mitochondrial health, brain health, and physical performance. But most importantly, because it is clearly linked with the prevention and treatment of numerous diseases. Perhaps the easiest way to conceptualize this benefit to the whole system (i.e. all systems of your body) is to understand that IHT improves overall metabolic health. In terms of markers of overall metabolic health, research has already shown that IHT has profound benefits on markers such as: [58]
1. Insulin Sensitivity: Some studies have suggested that IHT can improve insulin sensitivity, which is an important marker of metabolic health. Insulin resistance (the opposite of insulin sensitivity) is linked with numerous diseases, from cancer, to neurological disease, to heart disease and countless others. Improved insulin sensitivity means that the body's cells become more responsive to insulin, facilitating better regulation of blood sugar levels. A randomized controlled trial published in the journal Diabetes Care examined the effects of IH on glycemic control in type 2 diabetes patients. The study found that IH exposure resulted in improved glycemic control, evidenced by reductions in fasting blood glucose levels and glycated hemoglobin (HbA1c) levels.
2. Glucose Metabolism: IHT has been shown to influence glucose metabolism. It can affect glucose uptake, utilization, and storage in various tissues. Research suggests that IH may enhance glucose metabolism in skeletal muscle and adipose tissue, potentially contributing to improved metabolic health.[59]
3. Lipid Metabolism: IHT may also have an impact on lipid metabolism. Studies have reported changes in lipid profiles, such as decreased triglyceride levels and increased high-density lipoprotein (HDL) cholesterol levels. These alterations can be indicative of improved lipid metabolism and a more favorable lipid profile. In subjects with type II diabetes, it’s been shown that
IH increases insulin sensitivity, reduces blood sugar and cholesterol levels. [60–62]
4. Body Composition: IHT has been investigated for its potential effects on body composition. Some studies have shown that IH interventions may lead to reductions in body weight, body mass index (BMI), and body fat percentage, while promoting an increase in lean body mass. These changes can be beneficial for overall metabolic health.
5. Blood pressure: IHT has also been shown to lower blood pressure levels in those with hypertension. [63]
6. Inflammation: Chronic low-grade inflammation is associated with metabolic disorders. IH has been found to modulate inflammatory markers, leading to reductions in pro-inflammatory cytokines and markers of systemic inflammation. These anti-inflammatory effects may contribute to improved metabolic health.
Let’s place particular importance here for a moment on cardiovascular disease – the leading cause of death in the U.S. since 1950. Decades of research has now shown that daily sessions of intermittent hypoxic training can dramatically reduce markers (blood pressure, blood lipids/cholesterol, and blood flow to the heart) that indicate risk of dying from cardiovascular disease. [64]
Given that over 80% of Americans[65] (and similar numbers in most of the developed world) are now suffering from poor metabolic health, it’s hard to understate the importance of strategies that dramatically improve almost every aspect of overall metabolic health. IHT is one of the most powerful methods in existence to create widespread systemic improvements in your metabolic health.
2. Anti-Aging and Potential Longevity
Benefits
Now if that’s not enough, IHT also acts on several key longevity pathways. While no human research exists that directly assesses the longevity effects of long-term IHT, based on the known mechanisms of how it acts on the body, it’s as close to a certainty as possible that it does in fact enhance longevity. An amazing study that came out of Russia, where they looked at over 100 people engaging in this practice, concluded that intermittent hypoxic training may be a “bonafide anti-aging treatment.”
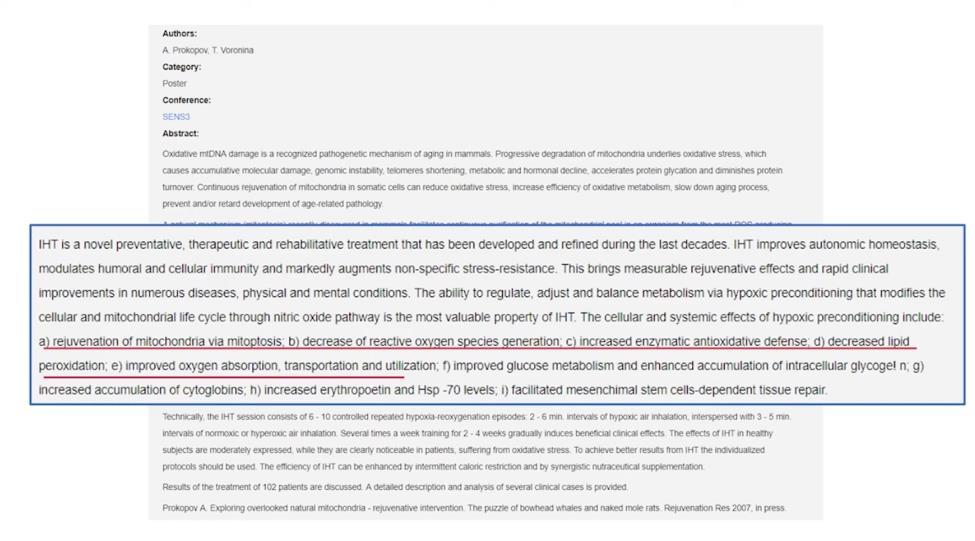
They found that IHT combats aging at the cellular level through the following mechanisms:
• Rejuvenation of mitochondria. IHT helps the body clean out damaged and dysfunctional mitochondria and replace them with healthy mitochondria
• Decreased reactive oxygen species generation (building up your internal antioxidant defense system).
• Decreased lipid peroxidation, which means decreased oxidation of the membranes inside cells and the mitochondria.
• Increased erythropoietin (the hormone that stimulates more blood cells to increase oxygen-carrying capacity in the blood), and therefore improved oxygen, absorption, transportation.
• Stimulating the production of stem cells to repair and regenerate damaged cells.
Other research[66] has similarly found an array of anti-aging mechanisms induced by intermittent hypoxic training – such as even acting to stabilize our telomeres (thought to help protect our DNA from damage/aging), help prevent cellular senescence (inflammatory “zombie” cells that drive cellular aging), and to increase cellular resistance to stressors (so they’re less likely to die when damaged).
The mechanism of stimulating our internal supply of stem cells is likely a hugely important aspect of combating aging and disease genesis. Stimulating endogenous stem cells has garnered significant interest in the fields of anti-aging, disease
prevention, minimizing cellular damage, and promoting longevity. Endogenous stem cells are present in various tissues throughout the body and have the remarkable ability to differentiate into different cell types, promoting tissue repair and regeneration. Here are the key reasons why stimulating endogenous stem cells holds immense importance in these areas:
1. Anti-aging: Aging is associated with a decline in the regenerative capacity of tissues and organs, leading to functional decline and increased vulnerability to diseases. By stimulating endogenous stem cells, we can potentially enhance tissue repair and regeneration, thereby slowing down the aging process. These stem cells have the ability to replace damaged or dysfunctional cells, maintaining tissue homeostasis and preserving organ function.
2. Disease Prevention: Endogenous stem cells play a crucial role in maintaining tissue integrity and preventing the accumulation of cellular damage. By activating these stem cells, we can enhance the body's natural defense mechanisms against diseases. These stem cells can replenish and repair damaged tissues, reducing the risk of age-related diseases such as neurodegenerative disorders, cardiovascular diseases, and metabolic disorders.
3. Minimizing Cellular Damage: Stimulating endogenous stem cells can help minimize the accumulation of cellular damage, which is a hallmark of aging and various diseases. Stem cells possess inherent mechanisms to repair DNA damage, counteract oxidative stress, and promote cellular rejuvenation. By enhancing the activity of these stem cells, we can potentially mitigate the detrimental effects of cellular damage and preserve cellular function.
4. Longevity: Stimulating endogenous stem cells can help minimize the accumulation of cellular damage, which is a hallmark of aging and various diseases. Stem cells possess inherent mechanisms to repair DNA damage, counteract oxidative stress, and promote cellular rejuvenation. The preservation and activation of endogenous stem cells have been linked to increased lifespan in preclinical studies. By stimulating these stem cells, we can promote tissue rejuvenation, delay age-related degeneration, and potentially extend healthy lifespan.
Efforts to stimulate endogenous stem cells involve various strategies, such as exercise, dietary interventions, pharmacological approaches, and regenerative medicine techniques. These approaches aim to enhance the proliferation, differentiation, and functionality of endogenous stem cells, harnessing their potential for anti-aging, disease prevention, and promoting overall longevity.
As luck would have it, it turned out that intermittent hypoxia training is a potent method to stimulate the proliferation and release of our internal supply of stem cells! You can literally turn out your cellular de-aging mechanisms in a few minutes with breath holding practices. For example, numerous studies in animal models have shown that intermittent hypoxia stimulates spikes in levels of circulating stem cells. [67]
Of course, rodent research doesn’t always translate into humans, so it’s important to note that at least one human study exists on the topic, which examined the effects of a 14-day IHT program consisting of breathing a 10% oxygen mixture for 5 minutes, four times per day. One week after completing the IHT regimen increased numerous measures of immune system activity, and lowered potent inflammatory cytokines TNF-α and IL-4 by >90% and ∼75%, respectively. When measured during the intermittent hypoxic training session, they found a transient 55% increase in circulating hematopoietic stem and progenitor cells.[68]
There is also evidence that hypoxia plays a central role in regulating the stem cell activity in the brain and central nervous system. Given that most neurons in the brain are non-regenerative, stem cell therapy is seen as a promising therapy for diseases of the brain and central nervous system. It has been shown that hypoxic training enhances the efficacy of stem cell therapy. [69]
As an added bonus, it’s worth mentioning that the stem cell mobilization (and tissue regeneration) benefits of intermittent hypoxic training may be further amplified by nitric oxide supporting supplements, such as arginine – as shown in some research where the intermittent hypoxic training and arginine combined group had the largest effect on upregulating growth factors and other mediators of tissue repair and regeneration that are associated with stem cell-mediated cellular repair.
While there is no human study on the lifespan extension effects of intermittent hypoxic training, the overall body of research on mechanisms activated from intermittent hypoxic training suggests that it likely has potent anti-aging, diseasepreventive, and longevity-enhancing effects.
3. Reduced Anxiety and Depression
(Increased Resilience to Mental
Stress)
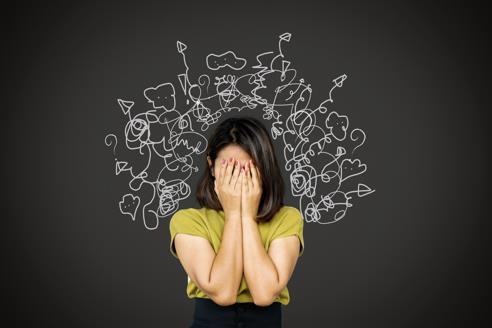
When most people are dealing with anxiety and mental stress, they tend to adopt strategies that engage mental tactics to try to lower or eliminate the anxiety. They try to combat what’s happening in the mind with their mind.
While this paradigm is heavily reinforced by current popular cultural paradigms and narratives (and approaches such as CBT and mindfulness), did you know that using your body – particularly by altering your breathing – may be an even more powerful way to combat these painful mental states?
By retraining the way your brain and body respond to changes in oxygen and carbon dioxide levels, you can profoundly alter the way you respond to mental stress, increasing your resilience and reducing the tendency towards anxiety and depression.
A fascinating but little-known fact is that many of the brain circuits that are activated with mental stress and anxiety/depressive disorders overlap with the brain circuits involved in sensing low oxygen levels in the blood and high CO2 levels.
Why might our body and brain be designed this way?
Consider that while the human body can go weeks without food, and days without water, it can’t survive for even 10 minutes without breathing air. So needless to say, just as the human body evolved sophisticated internal physiological mechanisms to make sure we get food when we’re running low on fuel, and that we get water when we’re starting to get dehydrated, we evolved even more sensitive mechanisms to attend to attend to getting adequate air and to prevent suffocation. The fear of suffocation is an ancient fear embedded into the human mind. So deep is this connection that it appears that the many of the same mechanisms that regulate blood oxygen and CO2 levels are the same mechanisms involved in regulating anxiety and mental stress.
In this light, it is interesting to note the profound overlap between the dimensions of breathing and mood (anxiety, panic, and depression). It is known that shortness of breath and feelings of suffocation are common in anxiety disorders. Similarly, anxiety disorders are extremely prevalent in those with respiratory diseases. In addition, mood changes in response to low oxygen supply – such as going to high elevation – are also common. We also know that breathing mixtures of air with high CO2 levels rapidly induces stress, anxiety, and panic.
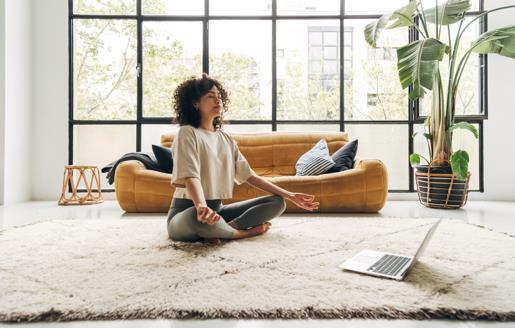
Putting this all together, we can see that the human body is very much designed to link mental states with breathing. In fact, we have a sophisticated internal system to detect drops in oxygen and increases in CO2 – both systematically in the blood and at the cellular level. Given how essential adequate oxygen supply to the cells is to stay alive, it’s not surprising that our body was designed in a way that alterations in O2 and CO2 rapidly trigger alterations in our mental state, particularly fear, anxiety, and panic.
Carbon dioxide (CO2) tolerance refers to an individual's ability to tolerate elevated levels of CO2 in the body. In individuals with anxiety disorders, particularly panic disorder, low CO2 tolerance has been found in numerous studies. In other words, the body’s CO2 levels – particularly in the blood – are linked with how the brain regulates stress and anxiety.
This has led some experts, such as Donald Klein, MD to postulate that many anxiety and panic disorders may be the result of a dysfunctionally hypersensitive “physiologic suffocation alarm system.” [70]
As it turns out, the amygdala – thought to be the central fear- and threatprocessing center of the brain that hugely impacts the body’s degree of sympathetic (fight or flight) activation and stress responses – has CO2 receptors built into it and is designed to increase stress/threat-activation signaling when CO2 levels rise. [71]
Of course, this system is “tuned” by our lifestyle and the events of our life from the time we’re a baby. It is highly dynamic, and can either be relatively easy or relatively difficult to activate. That is, some people can be hypersensitive to CO2 increases such that it easily triggers sympathetic arousal and a stress response.
This circuitry was designed into mammalian biology millions of years ago, again, as a “suffocation alarm” system.
But what’s important to understand is that the circuitry can be dysregulated –particularly due to factors in the modern human lifestyle – resulting in CO2 hypersensitivity and susceptibility to chronic anxiety.
Testing for CO2 hypersensitivity commonly involves administering an air mixture with higher CO2 levels to patients. Increased CO2 levels are known to trigger anxiety symptoms across various anxiety disorders, including panic disorder, posttraumatic stress disorder, and social anxiety disorder. Moreover, subjects often describe the sensations induced by breathing CO2-enriched air as remarkably similar to the anxiety and panic they experience in their daily lives.
Studies have shown that compared to demographically-matched healthy participants exposed to the same CO2 dose, anxious patients experience significantly higher levels of anxiety. This confirms that not only do elevations in CO2 trigger anxiety, but that chronic anxiety is linked with CO2 hypersensitivity. CO2 elevations are known to induce anxiety across most of the spectrum of anxiety disorders.
High levels of CO2 doses (breathing 35% CO2 mixtures) are known to induce panic, but lower doses (smaller elevations in blood CO2 levels) are known to strongly induce increases in anxiety. Studies utilizing lower CO2 doses ranging from 5.5% to 20% have consistently shown heightened anxiety levels in anxious patients, while the same CO2 exposures cause minimal anxiety in healthy (non-chronically anxious) persons. So we know there is a spectrum of CO2 sensitivity that maps onto the tendency towards anxiety. At one end of the continuum are healthy individuals who exhibit minimal anxiety in response to CO2. In the middle are patients with generalized anxiety disorder and social phobia, who typically report mild-to-moderate anxiety when exposed to CO2. At the extreme end of the continuum are patients with panic disorder and agoraphobia, who often experience the highest levels of anxiety in response to CO2.
When an individual has hypersensitivity to CO2, their body engages mechanisms to help keep CO2 levels low enough to avoid crossing the CO2 threshold to the point that triggers anxiety.[72] In particular, the brain may engage subtle strategies (that the person is unaware of or thinks are normal) such as increased sighing or yawning, and typically starts breathing more air per minute (increased minute ventilation).[73] These are basically forms of breathing dysregulation, and while they help combat anxiety in the short-term (by helping to blow off more CO2), the more these physiological changes are wired into your physiology, the more they
entrench CO2 hypersensitivity and thus actually contribute to the develop of chronic anxiety. [73]
It is known that individuals with numerous types of anxiety disorders and panic disorders also are prone to hyperventilation, and breathe more while at rest. This is thought to be the body’s compensatory way of dealing with a hypersensitive “suffocation alarm” setpoint.
Their CO2 threshold is very low, so even slight elevations in CO2 levels in the blood cause the body to react very strongly. This not only leads to increased breathing rate, but also often creates a subjective dyspnea (sensations of air hunger or breathlessness). Thus the compensatory chronic hyperventilation the body engages in serves to try to blow off as much CO2 as possible all the time, to keep CO2 levels sufficiently low to be setting off the suffocation alarm system.
Although this link between anxiety and breathing is not well-known in either the general public or among health practitioners, numerous lines of evidence support this general premise.[74–78]
The good news is that we can use this profound overlap between respiration and mental states to our advantage! By engaging in a practice of hypoxic (and hypercapnic, high CO2) breathing, we can rapidly modulate our brain’s CO2 threshold to make it less sensitive to elevations in CO2, change how our brain nonconsciously regulates our breathing habits, and change how our brain responds to stressful stimuli. In other words, we can use intermittent hypoxia to increase cellular and psychological resilience, thus making us much more resilient, and much less likely to be triggered into anxiety/panic states.
The adaptations induced by intermittent hypoxic and hypercapnic training directly interact with the central nervous system (and other physiological systems) that regulate our responses to mental stressors. (See the following image).
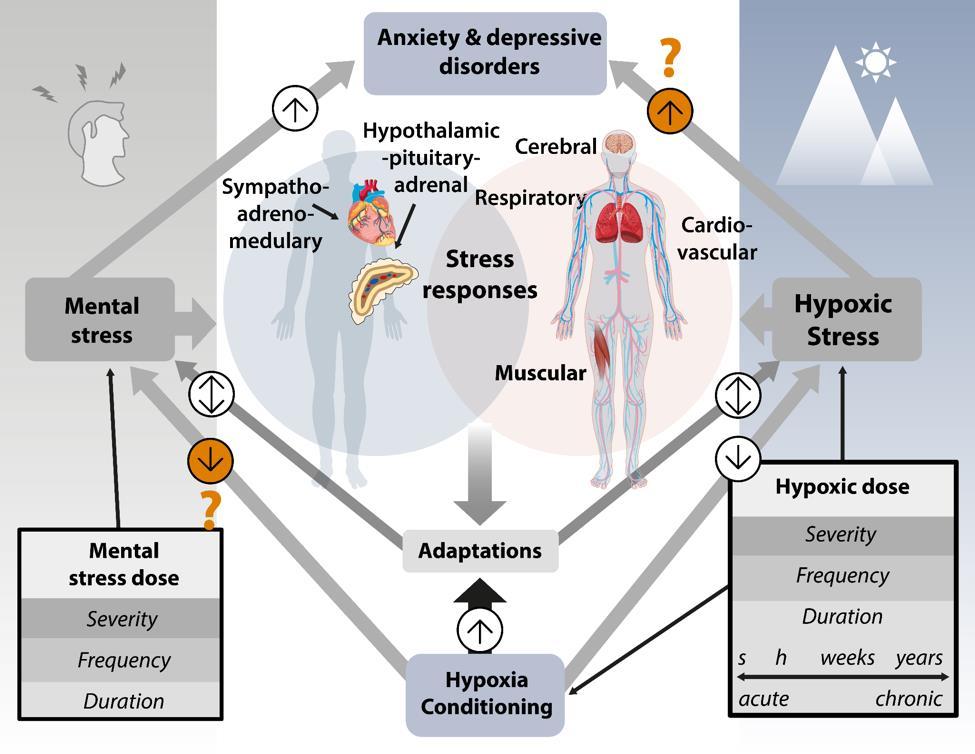
In other words, just as poor tolerance to decreased oxygen and higher CO2 levels contribute to anxiety, we can leverage this same system to increase our resilience in the face of mental stress, and increase resistance to anxiety and depression through intermittent hypoxic training.
In addition, IHT may also combat mental illness and improve brain health by virtue of its profound effects on mitochondrial health – specifically strengthening neuronal cellular energy production, and preventing the suppression of cellular energy production that can come from chronic mental stress. Numerous studies have now linked depressive and anxiety disorders (and many other types of mental illness) to poor mitochondrial health in the brain. Numerous mental illness and brain health experts, such as Chris Palmer, MD, now consider the brain’s mitochondrial health (and overall metabolic health) to be central causes of mental illness. Hypoxic training is known to induce profoundly beneficial effects on mitochondrial function (as discussed previously), and this is thought to be related to its ability to combat brain-related issues such as anxiety and depression[79]. Some evidence from animal models also suggests that chronic psychological stress also persistently elevates hypoxia inducible factor 1-alpha (HIF-1α ) which suppresses mitochondrial energy production.
Specifically, it does this by creating a metabolic switch to shift cellular energy production away from the much more optimal and efficient mitochondrial energy production towards anaerobic glycolysis (which is vastly less efficient). In a true low oxygen state, the metabolic switch induced by HIF-1α is profoundly beneficial – it allows for continued cellular energy production even with the deficit in oxygen supply, without the excessive reactive oxygen species (“free radicals”) that would be produced if the cell was still trying to use mitochondrial energy production.
So in the acute, transient oxygen deficit state, this is desirable. But when physiology is dysfunctional in such a way that drives chronic activation of HIF-1α even when adequate oxygen is actually present, that’s dysfunctional. This metabolic switch towards anaerobic glycolysis dominance is associated with numerous diseases, including cancer and chronic fatigue syndrome. Remember, suppressed mitochondrial energy production is linked with depression and anxiety! IHT prevents this effect of psychological stress on HIF-1α, thereby working to maintain healthy energy production in the brain and body even when under stress. This may be another mechanism through which IHT enhances resilience at the cellular level –preventing the stress-induced suppression of cellular energy production.
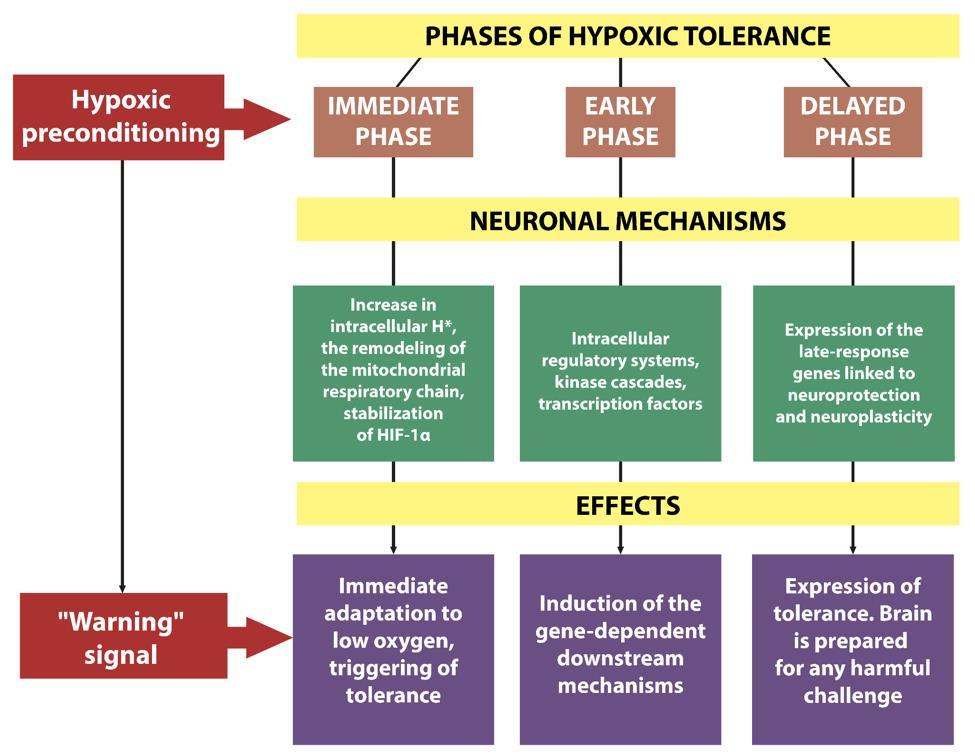
Let’s now talk about some specific aspects of depression, PTSD, and anxiety/panic disorder.
Depression
Accumulating evidence in both rodent and human studies has shown that intermittent hypoxic training combats depression at the level of the brain.[1] In animal studies, intermittent hypoxic training has clear preventive and antidepressant effects in models of learned helplessness and depression.[58,80] While there is minimal research in humans to date, the results thus far are impressive. One study found that a daily regimen of multiple 5-minute intervals of inhaling hypoxic air reduced depression symptoms in over 70% of people tested![1,58]
Mechanistically, we know that intermittent hypoxic training increases levels of neuronal growth factors and induces a state of increased neuroplasticity [58](particularly hippocampal neurogenesis) – both of which are known to combat depression and are a commonality among most effective treatments for depression. With intermittent hypoxic training, inducing this state can be done in minutes, doesn’t require any equipment or substances, and is free.
Post-Traumatic Stress Disorder (PTSD)
IHT has also been shown to have profound anti-anxiety effects in animal models of PTSD. [81]Also in animal models, IHT has been shown to alleviate the oxidative stress and physiological dysfunction in various organ systems associated with PTSD.[82] Other research in rodents has shown that IHT reverses structural and functional changes to the adrenal glands, and greatly reduces[83]
Anxiety
As we previously saw, chemosensitive (O2 and CO2 sensing) brain regions heavily overlap with brain regions that control physiological stressresponses and also the regulation of anxiety and depression. It is for this reason that IHT has profound effects on anxiety. This has led some researchers to make statements like
“We hypothesize that reversing homeostatic alterations with meditation and breathing techniques rather than targeting neurotransmitters with medication may be a superior method to address the whole body changes that occur in stress, anxiety, and depression…We propose that these breathing techniques could be used as first-line and supplemental treatments for stress, anxiety, depression, and some emotional disorders.”[84]
This link between respiration and anxiety also explains why various kinds of breathing practices can rapidly and powerfully modulate anxiety levels, often within just seconds or minutes. For example, a recent study from Stanford Medicine found remarkable reductions in subjective stress and improvements in positive affect (emotional state) from using certain breathing techniques – a larger improvement than with mindfulness meditation.[85]
But more importantly than the in-the-moment modulation of acute stress, I would argue that training the body into a physiologic state that is much more resilient and much less susceptible to anxiety/overwhelm from mental stress should be the real goal. This isn’t about doing a practice that creates an instantaneous effect anymore than one might expect to grow larger bicep muscles within moments of initiating a few bicep curls. The idea is to “train” the body to expose it to challenges that send it the signal that it needs to change to better survive those demands by making physiological adaptations that increase resilience to stressors.
A study in high school students found that even a tiny amount (5 minutes, just 3 times per week) of hypoventilation was enough to significantly improve CO2 tolerance and reduce anxiety levels. For just 5 minutes, 3 times per week, students were asked to do 5-second inhales, 5-second breath holds, 10-second exhales, and 5-second breath holds. This is a type of box breathing which, like breath holding, works to create a subtle hypoxic and hypercapnic state. Even with just 15 minutes of total practice per 7 days, they showed profound improvements in CO2 tolerance and a whopping 73% of students showed reduced trait (overall baseline) anxiety, with a mean of 12.3% improvement. Both short-term (immediately post-breathing practice) and long-term changes in adaptations occurred to increase CO2 tolerance and resilience.[86] Again, this was all with only a total of 15 minutes of breathwork per week – far from ideal.
While studies on intermittent hypoxic training are rare in anxiety disorders, the overall evidence linking hypersensitive “suffocation alarms” to anxiety is strong.[87] So mechanistically, we know that this type of training should be highly effective in reducing anxiety. Moreover, while breath-holding and hypoxia practices specifically haven’t been tested extensively in anxiety treatment, numerous breathwork modalities which challenge the setpoints of these breathing regulation systems have consistently shown that breathwork is a powerful way to modulate chronic anxiety. In particular, a 2023 systematic review of 12 studies titled “Effect of breathwork on stress and mental health: A meta-analysis of randomisedcontrolled trials” found strong evidence that breath training is effective in combating anxiety disorders.[88] These studies employed various approaches: Slow breathing exercises (to reduce air breathed per minute, and to retrain hyperventilation), hypercapnic breathing to induce higher levels of CO2 to increase
the body’s CO2 threshold, breath training with biofeedback to better control CO2 levels, and other methods.[87]
Based on the known respiratory dysregulation associated with anxiety – namely CO2 hypersensitivity, it is likely that hypoventilation (reduced breathing, or breath holding) and hypercapnic practices (also induced by reduced breathing and breath holding) promotes powerful anxiety-reducing effects by inducing high CO2 challenges (much like heavy weights challenge a muscle), and inducing dyspnea (the sensation of running out of air), which work to create adaptations that increase the body’s CO2 tolerance and desensitizing the body’s central fear/stressprocessing networks.[87,89]
Further proving the link between breathing dysfunction (specifically with hyperventilation creating low CO2 levels) is research on CART (CapnometryAssisted Respiratory Training) breathing therapy. CART is a technique used to help individuals regulate their breathing patterns and manage symptoms related to anxiety, panic disorders, and other stress-related conditions. It involves the use of capnometry, which is the measurement of carbon dioxide (CO2) levels in exhaled breath, to provide biofeedback and guide the breathing exercises. The basic principle of CART breathing therapy is to adjust and optimize the balance between oxygen and CO2 in the body by controlling the breathing rate and depth. Since people with anxiety or panic disorders often exhibit dysfunctional breathing patterns characterized by hyperventilation, where they breathe rapidly and shallowly, leading to lower CO2 levels in the body, which in turn creates a tendency towards chronic anxiety due to CO2 hypersensitivity (a low CO2 threshold), training the body to tolerate higher levels of CO2 helps to increase the body’s CO2 threshold and de-sensitize the system to CO2 increases, and therefore reduces anxiety. During CART breathing therapy, a capnometer is used to measure CO2 levels in the exhaled breath. The individual receives immediate feedback on their CO2 levels through visual or auditory cues. The goal is to maintain a target range of CO2 levels that promotes a balanced and relaxed state.
The therapy involves teaching individuals various methods of slowing down the breathing – specific breathing techniques, such as diaphragmatic breathing or paced breathing, which focus on slowing down the breathing rate, deepening the breaths, and prolonging the exhalation phase. By doing so, it helps increase CO2 levels in the body (it’s hypercapnic training) which works to increase the body’s CO2 tolerance.
Research has in fact shown that CO2 levels and CO2 sensitivity drive the changes in stress appraisal that mediate anxiety. As one works to decrease their CO2 sensitivity (increase their CO2 threshold), the body experiences fewer instances of
shortness of breath and lowers overall sensitivity to elevations of CO2 with stress or physical activity – both of which act to dampen sympathetic (fight or flight) nervous system activation and dampen activity in fear circuits in the brain – that is, it works to desensitize the hypersensitive suffocation alarms of those with anxiety, ultimately decreasing the tendency towards chronic anxiety.
One study on CART therapy compared the efficacy of CART breathing practices with traditional cognitive therapy in those with panic disorder. [90]The study found that both therapies were equally effective in reducing panic and anxiety symptoms. But importantly, only the breathing therapy changed the physiology – hyperventilation, low CO2 levels, and CO2 sensitivity – linked with the anxiety/panic symptoms. That is, cognitive therapy worked via a top-down process of using cognitive effort to monitor one’s thinking and control negative thoughts, while the breathing therapy worked via a bottom-up process of modifying one’s non-conscious physiological processes to change the brain’s tendency towards negative (fearful/stressful/negative/catastrophic) thinking. The breathing retraining corrected the low levels of CO2, while the cognitive therapy did not. So in other words, one is a cognitive skill that one must practice, while the other works to correct the dysfunctional physiology that drives the hypersensitive fear- and stressresponse circuitry of the brain and nervous system.
Research has found that CART therapy leads to clinically significant reductions in anxiety and panic symptoms in 68% of people with panic disorder upon completion of 4 weeks of training, and that this increased to 79% after 2 months of follow-up, and a whopping 93% at 12-month follow-up. [90]This suggests a lasting (relatively permanent) re-wiring of one’s breathing habits and the physiology (CO2 levels and CO2 threshold) that is involved with regulating fear/stress responses and anxiety.
Overall, this body of evidence shows that intermittent hypoxic and hypercapnic training is a powerful tool for increasing brain- and systemic resilience (reducing susceptibility to harm from mental stress), enhancing brain health, resetting the brain circuitry involved in fear/stress/anxiety regulation, and combating psychological issues such as depression and anxiety.
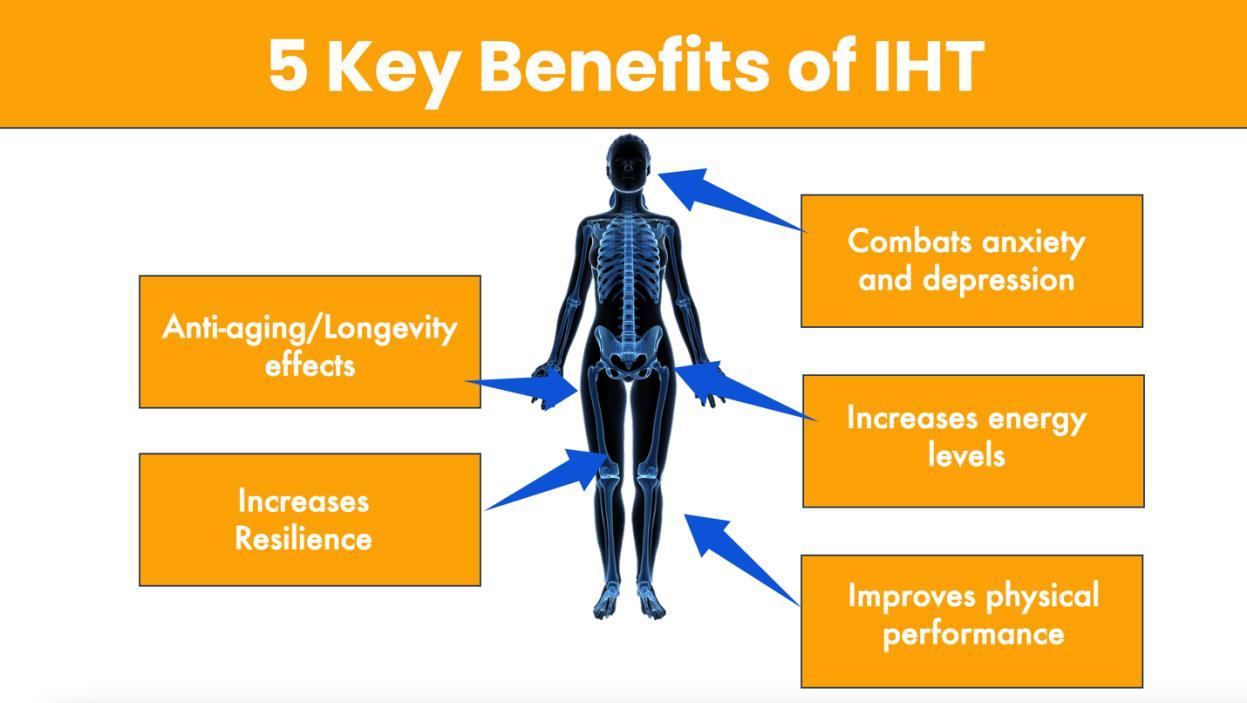
4. Improved athletic performance
One of the most well-researched areas within the field of intermittent hypoxic training is physical performance.
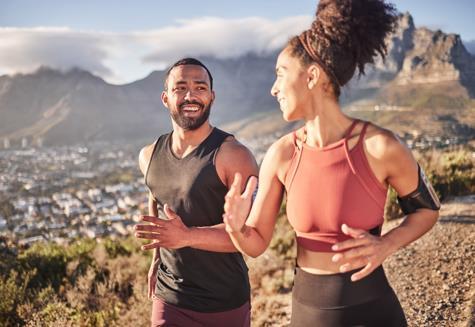
For many decades, athletes have been aware of the body’s special adaptations to low oxygen levels that occur at altitude. Exercise physiologists and coaches to elite athletes have spent countless hours devising and testing various methodologies for leveraging the body’s adaptations to low oxygen states into improved athletic performance. Methods to either go to altitude or mimic altitude such as [living high-training high (LHTH) or the living high-training low (LHTL)] improve athletic performance by enhancing oxygen delivery capacity do in fact work to improve athletic performance.
Tents and chambers were designed to make it possible for athletes to either exercise or sleep in low oxygen (and low air pressure) rooms to get these
adaptations. These methods work because they stimulate numerous beneficial adaptations such as the production of EPO (a hormone that increases red blood cells and increases oxygen-carrying capacity of the blood), and through other numerous adaptations that make the body more efficient at gas exchange at the level of the lungs, that enhance cardiac function and oxygen delivery to the cells, that enhance mitochondrial utilization of oxygen, and that enhance the energy production pathways that don’t utilize oxygen.
In addition, “hypoxicator” machines were invented that could be hooked up to an athlete’s face via a mask to allow the athlete to train (on a treadmill or stationary bike) while breathing in low oxygen air, thus stimulating many of the same physiological adaptations that occur at altitude. Voila. Just like that, the high elevations of the mountains could be accessed in a gym environment.
While these machines can be hooked up to athletes on a treadmill or stationary bike, it’s tough to do while swimming. Dr. James Counsilman, a highly regarded swimming coach, devised a training method to simulate the effects of training at high altitude. His approach involved restricting the frequency of breathing to create a hypoxic effect while training at sea level. Although this technique has become common in swimming and may offer respiratory adaptations that benefit athletes, it doesn't fully replicate the hypoxic environment experienced at high altitudes. That is, it’s not actually very effective at lowering blood oxygen levels.
Counsilman wasn't the only coach to promote hypoventilation training. Other coaches and athletes, including Emil Zatopek, have experimented with this idea to enhance performance. The general approach involves taking a breath, holding it for a specific duration or number of strokes, steps, or pedal rotations, and then exhaling before repeating the process.
A French physiologist Dr. Xavier Woorons then strategized a way to improve hypoventilation training. While traditional approaches primarily focused on training at relatively high lung volumes (i.e. holding the breath after a big inhalation), Woorons' hypothesized that one might create lower oxygen states by holding the breath after an exhalation.
To test his hypothesis, Dr. Woorons conducted a groundbreaking study. Ten triathletes cycled at 70% of their individual VO2max (moderately intense) for five minutes on two separate occasions. During one test, they maintained normal breathing, while during the other session, they incorporated a prolonged exhalation following each inhalation. This adjustment reduced ventilation frequency and led to more time spent in low lung volumes (the lungs mostly empty rather than mostly full of air).
The results? Cycling with a prolonged exhalation led to significant hypoxemia, resulting in lower blood oxygen levels – down to 87% instead of the 95% seen in those athletes using normal breathing. Just like that, hypoxic (altitude-mimicking states) states could be accessed at sea level, without even a hypoxic air machine –just with voluntarily managing one’s own breathing.
Numerous other studies have verified these findings. Blood oxygen levels drop to around 88% in young healthy adults holding their breath during moderate intensity cycling efforts.[91] When applied to repeated sprint exercises, voluntary hypoventilation at low lung volume (VHL) has also been reported to decrease blood oxygen to ~87% and to lead to greater muscle deoxygenation and blood lactate accumulation when compared to the same exercise protocol with normal breathing.[92]
More importantly, these studies have also conducted comparisons between the level of hypoxemia induced by this voluntary hypoventilation and doing exercise in a low oxygen chamber or breathing low oxygen air from a hypoxicator. Studies have found that both methods can reliably induce hypoxic (low oxygen) levels in the blood to similar degrees – indicating that modulating one’s breathing can in fact be used to induce hypoxic states as altitude does.
While all of that is nice is theory, the real question is: Does it actually work to enhance physical performance?
And the answer is yes. Numerous studies have found that inducing low oxygen states either through breathing hypoxic air (from a hypoxicator machine) or using voluntary hypoventilation/breath holding during exercise to induce low oxygen levels are both highly effective in inducing profound physiological adaptations and improving athletic performance.
Given that breath hold training (and other types of of intermittent hypoxic training) increases erythropoietin (EPO) levels (a well-known hormone injected by endurance athletes as a form of blood doping), lung capacity, and mitochondrial health, it’s logical to think that these changes might lead to improved athletic performance. While not all studies show profound benefits – because there is a huge variety of study designs and methods in which hypoxia is employed – numerous studies have also found that intermittent hypoxic training does in fact profoundly improve athletic performance, and can do so in some cases by huge amounts even in already highly trained athletes.
Here is just a small sampling of some of the relevant research:
● A group of 20 well-trained swimmers (10 men and 10 women) participated in a randomized controlled trial with both steady state and high-intensity interval training, either done in normoxic or hypoxic conditions. The results showed that the IHT group experienced significant improvements in various hemodynamic (heart and blood-related) parameters compared to the control group. The IHT group demonstrated increased cardiac output (blood output from the heart), stroke volume (amount of blood pushed out of the heart with each pump), and blood oxygen saturation both at rest and during exercise. The IHT group also had larger improvements in VO2max. These improvements indicated enhanced cardiovascular function and oxygen delivery to the working muscles. But of course, what really matters is not just the adaptations, so the study assessed exercise performance through a 400-meter freestyle swim test, and found that the IHT group exhibited a much larger improvement in their swimming performance compared to the control group.[92]
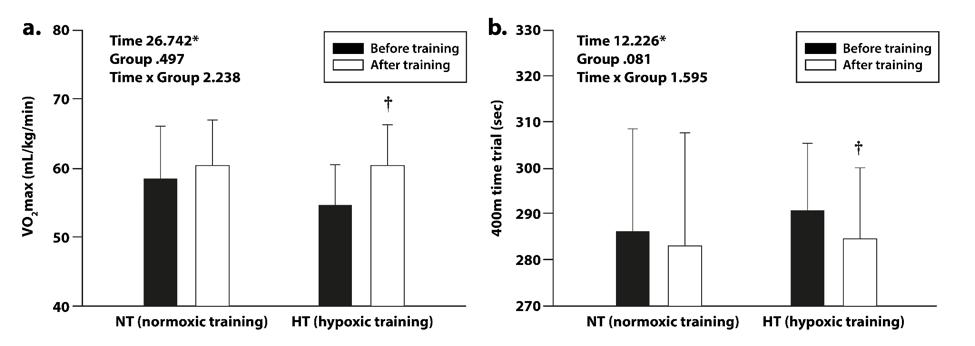
● A study examined how intermittent hypoxic exposure during training influenced anaerobic capacity and swimming performance. A group of 16 well-trained competitive swimmers participated in a randomized controlled trial. They were divided into two groups: an IHT group and a control group. After 4 weeks of training, the results showed that the IHT group demonstrated improvements in anaerobic performance and anaerobic power output, as measured by the Wingate Anaerobic Test, compared to the control group. [93]
● A group of 12 highly-trained male runners participated in a study where they were randomly assigned to either an IHT group or a control group. The IHT group underwent eight weeks of training that included running sessions in a simulated hypoxic environment, while the control group followed a similar training program in normoxic conditions. The results showed that the IHT group experienced significant improvements in running economy, a reduction in submaximal heart rate and oxygen cost during submaximal running. Furthermore, only the IHT increased their time-to-exhaustion while the group that did exercise alone (without hypoxia) did not improve.[94]
● Another study in competitive swimmers found that breath holding during swim training mimicked the same benefits seen from hypoxia induced by wearing a mask and receiving hypoxic air. They found that compared with the swimmers just engaged in the exercise training, those who incorporated breath holds into their training significantly increased their repeat sprint ability (how many sprints they were able to perform) by reducing accumulated fatigue, and increased the athletes’ lactate threshold (how much lactic acid their body can tolerate).[94] Research also indicates that the likely reason for the improved lactate threshold is to to increased acidbuffering capacity of the muscles.[95,96] It’s important to note that this “voluntary hypoventilation” (breath holding) was most effective when performed with low pulmonary volume (i.e. after an exhale rather than holding the air in the lungs after an inhale). [97]
● Similar studies on voluntary hypoventilation (breath holding) have also found similar improvements in repeat sprint ability in cyclists, hockey players, rugby players, basketball players, and triathletes. [96,98–100]
● A small study in 6 males in the military found that voluntary hypoventilation (breath holding) done 2-4 times per week for 7 weeks, improved their multistage 20-meter shuttle run test scores by a whopping 50.3%. [101]
● A study in 20 competitive runners who were divided into normoxic (normal oxygen levels) and hypoxic training groups performed the same exercise routines for 6 weeks found that the hypoxic training group had greater improvements in cardiac output, oxygen uptake, pulse, autonomic nervous system function, and VO2max. [102]
● Some research has also shown that adding hypoxia to exercise training results in profound improvements in mitochondrial function. One study showed a remarkable 55.2% increase in mitochondrial density of skeletal muscle in the group that combined hypoxia with high-intensity interval training.
● Another study found a similar 59% increase in mitochondrial content in the group doing hypoxia with their exercise training, whereas the group that did the same training without hypoxia only increased mitochondrial content by 23.9%.
● Another study that showed profound improvements in the mitochondrial function in competitive runners, and in a test of running time to exhaustion, those runners who did the exercise training combined with hypoxia increased their total time by a whopping 41.7% while those who only engaged in the exercise training without hypoxia had no significant increase.[103]
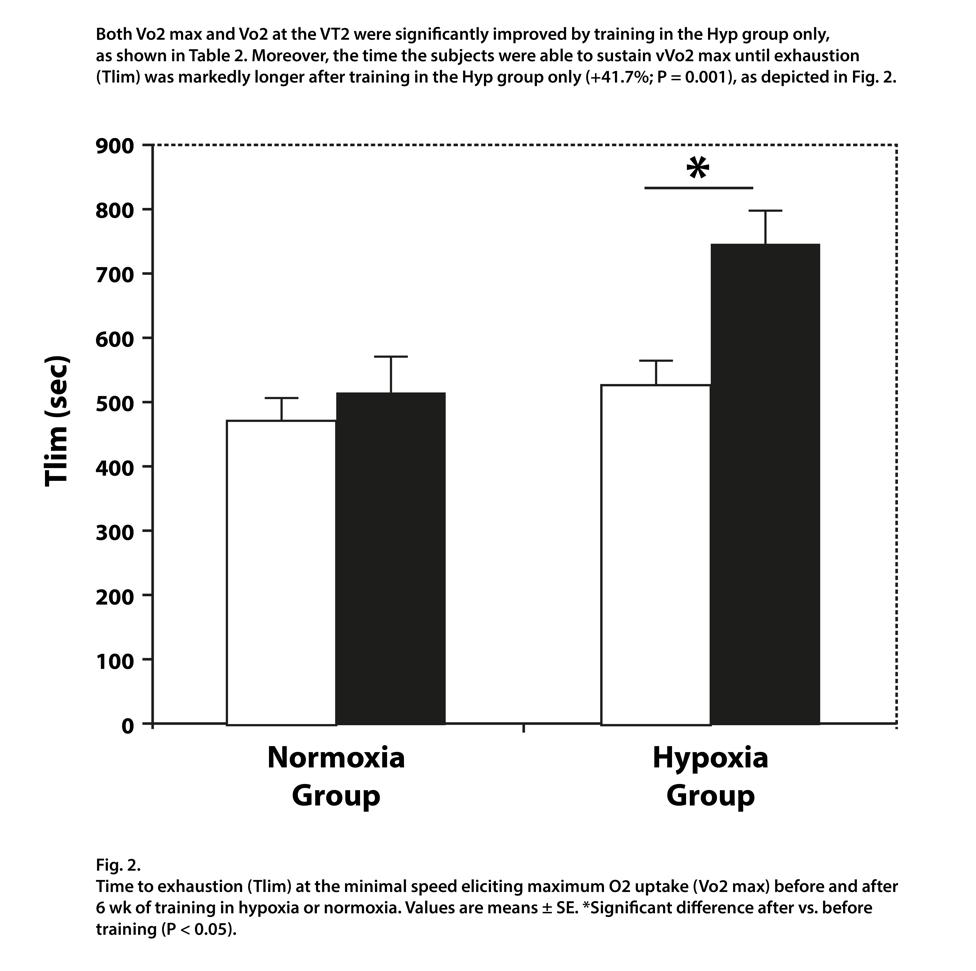
Overall, the body of evidence indicates that hypoxic training with exercise is remarkably effective at stimulating key adaptations – especially increasing the lactate threshold and mitochondrial adaptations – that ultimately lead to profound improvements in exercise performance.
As a result of rewiring our brain’s regulation of our breathing habits and our CO2 tolerance with IHT practices, we can enhance oxygen delivery our cells, stimulate
our mitochondria to grow stronger, and optimize the regulation of our nervous system (which hugely impacts on stress and anxiety levels).
IHT → Reduced Breathing Rate + Higher CO2 Tolerance → Increased CO2 levels → Increased oxygen delivery to the cells
IHT → Reduced Breathing Rate + Higher CO2 Tolerance → Decreased Sympathetic Tone → Reduced Anxiety and Improved Mood
IHT → Improved Oxygen Extraction + Oxygen-Carrying Capacity + Mitochondrial Biogenesis → Increased Cellular Energy Production
Putting this all together, it’s clear that IHT is one of the most powerful health-promoting practices you can do. Particularly if your goal is more energy, less anxiety, and increased longevity.
How To Do IHT
Hyperventilation and breath holds at rest
So how do you actually implement this method? Well, there's a few different ways. Wim Hof breathing has become popularized in the last few years. That's one way of doing this. There's also ancient techniques out of India, different kinds of pranayama techniques where they do fire breathing in tandem with breath hold practices, Nishesha rechaka Pranayama.[104] So for example, 30 to 50 fire breathing breaths, and this is where you pump your belly and breathe in and out of your nose.
Wim Hof breathing originates from the ancient Tibetan practice of g-Tummo breathing, also called Chandali yoga, which is claimed to “awaken the inner fire” and is used to help meditators increase their ability to focus, and to relax.
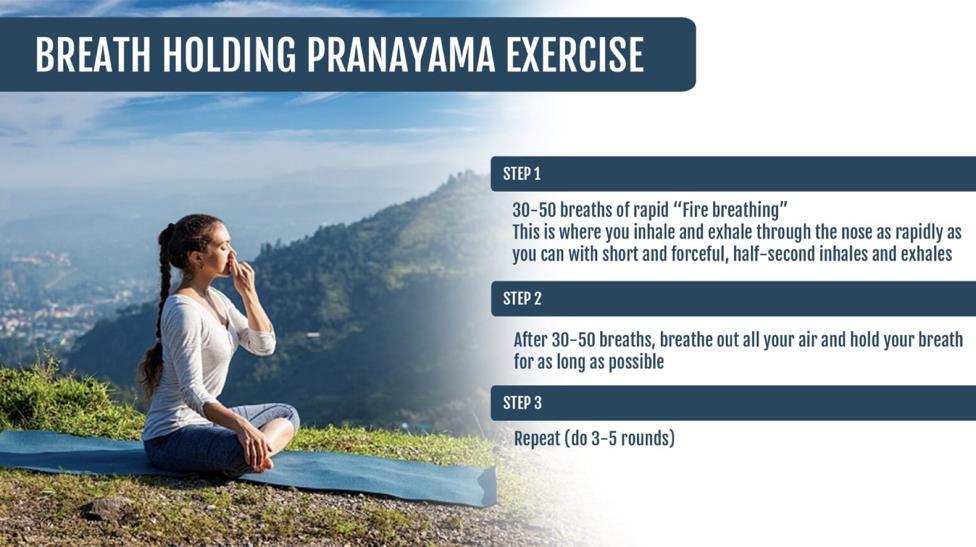
Wim Hof, the man who has set numerous world records for time immersed in crushed ice, under ice swims, his almost complete ascent of Mt. Everest wearing nothing but shorts and shoes, among other feats, has developed the practice into the following method:
● 30 full inhales followed by relatively relaxed partial exhales
● Fully exhaling the last breath and holding the breath
● Fully inhaling and holding breath again for about 15 seconds before starting the next round (and each round is consecutively longer)
The reason for the hyperventilation prior to the breath hold is to induce low CO2 levels, so that when the breath is held, one can hold longer in the hypoxic state before CO2 levels build up high enough that it causes one to need to take a breath.
Walking breath holds
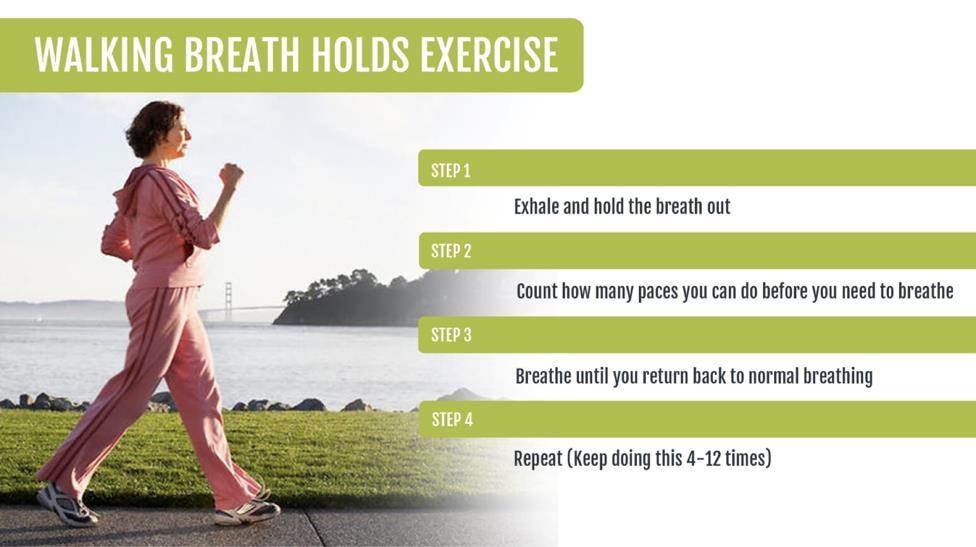
Hypoxia machine IHT while doing exercise
Another wonderful and thoroughly studied way to train hypoxic (but not hypercapnic) states is to use a machine that generates air with lower oxygen levels. This can be done for periods while at rest, or more commonly, is used with a mask while either on a stationary bike or using a treadmill.
During an intermittent hypoxic training session, individuals breathe in ambient oxygen at a lower pressure than normal. This deliberate reduction in oxygen pressure leads to a decrease in the body's blood oxygen saturation level. This saturation level plays a crucial role in both short-term and long-term adaptations during exercise.
When oxygen enters the body, it travels through the lungs and binds with hemoglobin in the bloodstream. However, a lower partial pressure of oxygen hinders the oxygenation process in the lungs. Consequently, the bloodstream carries reduced oxygen saturation, resulting in a diminished oxygen supply to the muscles that are actively engaged in the workout.
By training with a slightly lower oxygen saturation level (around 80-90%), it is possible to stimulate the hypoxia-inducible factor. This factor sends signals to the nuclei of the body's muscle cells, prompting them to adapt positively and achieve a higher level of homeostasis.
Voluntary hypoventilation during exercise
Finally, the method pioneered by exercise physiologist Xavier Woorons involves combining low to moderate intensity exercise with either breath holds or intervals There are far too numerous of nuances to get into a detailed practical guide of how to do this (and it’s generally a more advanced method of training appropriate for athletes) but the basic idea is using very short breath holds (or slowed breathed) for a few steps/strokes or for a short duration of time, alternated with normal breathing periods, during prolonged exercise.
CAUTIONS:
1. Do not do any type of breath holding underwater unless you are experienced and have someone there watching you. It’s rare, but it is possible to black out during a breath hold. If this happens while you’re on land, it’s not much of a problem because your body will keep breathing even while you’re non conscious. But if you’re underwater, unless there is someone there to save you within a few seconds, it’s likely going to be deadly. So please make decisions to practice this cautiously and intelligently.
2. If you're severely chronic fatigued with ME/CFS, you likely should not do maximal breath holding practices as they may be too intense for you. You have to do the more basic foundational breathing practices (gentle “air hunger” practices) to start to build up CO2 tolerance much more gently before you go into the more advanced practices of intermittent hypoxic training. Or you may wish to start with extremely gentle and short holds.
Want to Go Deeper and Practice Intermittent Hypoxic Training?
If you’re struggling with your energy levels or high levels of stress and anxiety, and you'd like to learn how to do all of this with step by world class guidance, I’d like to suggest my program done with world-renowned breathing expert Patrick McKeown, called Breathing For Energy.
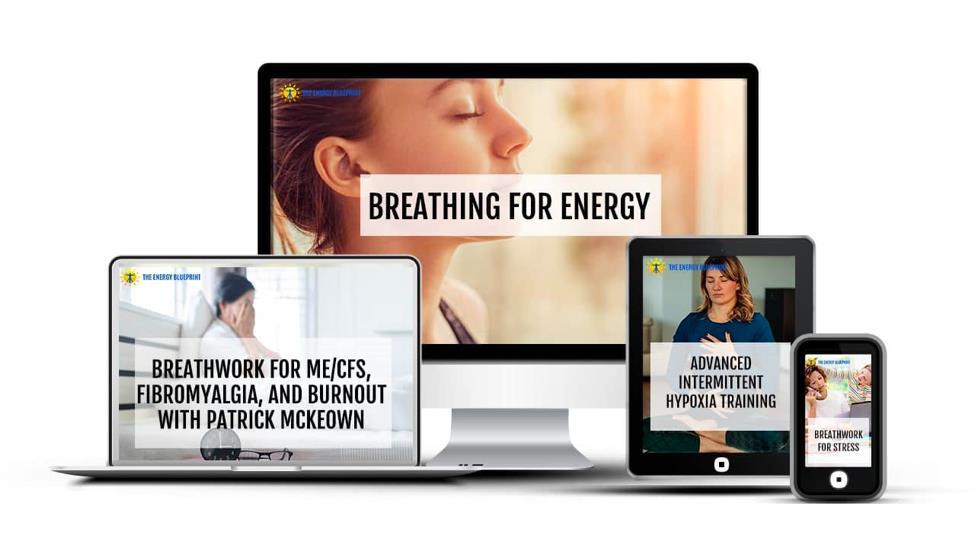
What you will get with the Breathing For Energy Program:
• 7 Levels of fully-guided IHT practices taking you from 15 second breath holds up to 4 minutes and beyond (Elite, Navy Seal level)
• Advanced breathing science masterclass with specific breathwork tools for increasing energy, reducing anxiety, and improving mood and brain function
• 3 separate multi-week courses in 1: Chronic fatigue fix, Sleep fix, and Anxiety fix breathwork
• Direct access to Ari's team of coaches in the Breathing For Energy Facebook members' community to answer your questions
Your Price $297
References
1. Basovich SN. The role of hypoxia in mental development and in the treatment of mental disorders: a review. Biosci Trends. 2010;4:288–96.
2. Tavel ME. Hyperventilation syndrome hiding behind pseudonyms? Chest. 1990;97:1285–8.
3. Folgering H. The pathophysiology of hyperventilation syndrome. Monaldi Arch Chest Dis. 1999;54:365–72.
4. Refer Medical and physiological textbooks with norms for breathing [Internet]. Normal Breathing: The Key to Vital Health. Normal Breathing; 2019 [cited 2023 May 31]. Available from: https://www.normalbreathing.com/refer-texts/
5. Minute Ventilation [Internet]. Normal Breathing: The Key to Vital Health. Normal Breathing; 2019 [cited 2023 May 31]. Available from: https://www.normalbreathing.com/minute-ventilation/
6. Dimopoulou I, Tsintzas OK, Alivizatos PA, Tzelepis GE. Pattern of breathing during progressive exercise in chronic heart failure. Int J Cardiol. 2001;81:117–21; discussion 121–2.
7. Johnson BD, Beck KC, Olson LJ, O’Malley KA, Allison TG, Squires RW, et al. Ventilatory constraints during exercise in patients with chronic heart failure. Chest. 2000;117:321–32.
8. Fanfulla F, Mortara A, Maestri R, Pinna GD, Bruschi C, Cobelli F, et al. The development of hyperventilation in patients with chronic heart failure and CheyneStrokes respiration: a possible role of chronic hypoxia. Chest. 1998;114:1083–90.
9. Clark AL, Volterrani M, Swan JW, Coats AJ. The increased ventilatory response to exercise in chronic heart failure: relation to pulmonary pathology. Heart. 1997;77:138–46.
10. Banning AP, Lewis NP, Northridge DB, Elborn JS, Hendersen AH. Perfusion/ventilation mismatch during exercise in chronic heart failure: an investigation of circulatory determinants. Br Heart J. 1995;74:27–33.
11. Clark AL, Chua TP, Coats AJ. Anatomical dead space, ventilatory pattern, and exercise capacity in chronic heart failure. Br Heart J. 1995;74:377–80.
12. Buller NP, Poole-Wilson PA. Mechanism of the increased ventilatory response to exercise in patients with chronic heart failure. Br Heart J. 1990;63:281–3.
13. Elborn JS, Riley M, Stanford CF, Nicholls DP. The effects of flosequinan on submaximal exercise in patients with chronic cardiac failure. Br J Clin Pharmacol. 1990;29:519–24.
14. D’Alonzo GE, Gianotti LA, Pohil RL, Reagle RR, DuRee SL, Fuentes F, et al. Comparison of progressive exercise performance of normal subjects and patients with primary pulmonary hypertension. Chest. 1987;92:57–62.
15. Travers J, Dudgeon DJ, Amjadi K, McBride I, Dillon K, Laveneziana P, et al. Mechanisms of exertional dyspnea in patients with cancer. J Appl Physiol. 2008;104:57–66.
16. Bottini P, Dottorini ML, Cristina Cordoni M, Casucci G, Tantucci C. Sleepdisordered breathing in nonobese diabetic subjects with autonomic neuropathy. Eur Respir J. 2003;22:654–60.
17. Tantucci C, Bottini P, Fiorani C, Dottorini ML, Santeusanio F, Provinciali L, et al. Cerebrovascular reactivity and hypercapnic respiratory drive in diabetic autonomic neuropathy. J Appl Physiol. 2001;90:889–96.
18. Mancini M, Filippelli M, Seghieri G, Iandelli I, Innocenti F, Duranti R, et al. Respiratory muscle function and hypoxic ventilatory control in patients with type I diabetes. Chest. 1999;115:1553–62.
19. Tantucci C, Scionti L, Bottini P, Dottorini ML, Puxeddu E, Casucci G, et al. Influence of autonomic neuropathy of different severities on the hypercapnic drive to breathing in diabetic patients. Chest. 1997;112:145–53.
20. Tantucci C, Bottini P, Dottorini ML, Puxeddu E, Casucci G, Scionti L, et al. Ventilatory response to exercise in diabetic subjects with autonomic neuropathy. J Appl Physiol. 1996;81:1978–86.
21. Chalupa DC, Morrow PE, Oberdörster G, Utell MJ, Frampton MW. Ultrafine particle deposition in subjects with asthma. Environ Health Perspect. 2004;112:879–82.
22. Johnson BD, Scanlon PD, Beck KC. Regulation of ventilatory capacity during exercise in asthmatics. J Appl Physiol. 1995;79:892–901.
23. Bowler SD, Green A, Mitchell CA. Buteyko breathing techniques in asthma: a blinded randomised controlled trial. Med J Aust. 1998;169:575–8.
24. Kassabian J, Miller KD, Lavietes MH. Respiratory center output and ventilatory timing in patients with acute airway (asthma) and alveolar (pneumonia) disease. Chest. 1982;81:536–43.
25. McFadden ER Jr, Lyons HA. Arterial-blood gas tension in asthma. N Engl J Med. 1968;278:1027–32.
26. Palange P, Valli G, Onorati P, Antonucci R, Paoletti P, Rosato A, et al. Effect of heliox on lung dynamic hyperinflation, dyspnea, and exercise endurance capacity in COPD patients. J Appl Physiol. 2004;97:1637–42.
27. Sinderby C, Spahija J, Beck J, Kaminski D, Yan S, Comtois N, et al. Diaphragm
activation during exercise in chronic obstructive pulmonary disease. Am J Respir Crit Care Med. 2001;163:1637–41.
28. Stulbarg MS, Winn WR, Kellett LE. Bilateral carotid body resection for the relief of dyspnea in severe chronic obstructive pulmonary disease. Physiologic and clinical observations in three patients. Chest. 1989;95:1123–8.
29. Radwan L, Maszczyk Z, Koziorowski A, Koziej M, Cieslicki J, Sliwinski P, et al. Control of breathing in obstructive sleep apnoea and in patients with the overlap syndrome. Eur Respir J. 1995;8:542–5.
30. Epstein SK, Zilberberg MD, Jacoby C, Ciubotaru RL, Kaplan LM. Response to symptom-limited exercise in patients with the hepatopulmonary syndrome. Chest. 1998;114:736–41.
31. Kahaly GJ, Nieswandt J, Wagner S, Schlegel J, Mohr-Kahaly S, Hommel G. Ineffective cardiorespiratory function in hyperthyroidism. J Clin Endocrinol Metab. 1998;83:4075–8.
32. Fauroux B, Nicot F, Boelle P-Y, Boulé M, Clément A, Lofaso F, et al. Mechanical limitation during CO2 rebreathing in young patients with cystic fibrosis. Respir Physiol Neurobiol. 2006;153:217–25.
33. Browning IB, D’Alonzo GE, Tobin MJ. Importance of respiratory rate as an indicator of respiratory dysfunction in patients with cystic fibrosis. Chest. 1990;97:1317–21.
34. Ward SA, Tomezsko JL, Holsclaw DS, Paolone AM. Energy expenditure and substrate utilization in adults with cystic fibrosis and diabetes mellitus. Am J Clin Nutr. 1999;69:913–9.
35. Dodd JD, Barry SC, Barry RBM, Gallagher CG, Skehan SJ, Masterson JB. Thinsection CT in patients with cystic fibrosis: correlation with peak exercise capacity and body mass index. Radiology. 2006;240:236–45.
36. McKone EF, Barry SC, Fitzgerald MX, Gallagher CG. Role of arterial hypoxemia and pulmonary mechanics in exercise limitation in adults with cystic fibrosis. J Appl Physiol. 2005;99:1012–8.
37. Bell SC, Saunders MJ, Elborn JS, Shale DJ. Resting energy expenditure and oxygen cost of breathing in patients with cystic fibrosis. Thorax. 1996;51:126–31.
38. Tepper RS, Skatrud JB, Dempsey JA. Ventilation and oxygenation changes during sleep in cystic fibrosis. Chest. 1983;84:388–93.
39. Esquivel E, Chaussain M, Plouin P, Ponsot G, Arthuis M. Physical exercise and voluntary hyperventilation in childhood absence epilepsy. Electroencephalogr Clin Neurophysiol. 1991;79:127–32.
40. Han JN, Stegen K, Simkens K, Cauberghs M, Schepers R, Van den Bergh O, et
al. Unsteadiness of breathing in patients with hyperventilation syndrome and anxiety disorders. Eur Respir J. 1997;10:167–76.
41. Pain MC, Biddle N, Tiller JW. Panic disorder, the ventilatory response to carbon dioxide and respiratory variables. Psychosom Med. 1988;50:541–8.
42. MacKinnon DF, Craighead B, Hoehn-Saric R. Carbon dioxide provocation of anxiety and respiratory response in bipolar disorder. J Affect Disord. 2007;99:45–9.
43. Clague JE, Carter J, Coakley J, Edwards RH, Calverley PM. Respiratory effort perception at rest and during carbon dioxide rebreathing in patients with dystrophia myotonica. Thorax. 1994;49:240–4.
44. Martin BJ, Sparks KE, Zwillich CW, Weil JV. Low exercise ventilation in endurance athletes. Med Sci Sports. 1979;11:181–5.
45. Woorons X, Mollard P, Pichon A, Lamberto C, Duvallet A, Richalet J-P. Moderate exercise in hypoxia induces a greater arterial desaturation in trained than untrained men. Scand J Med Sci Sports. 2007;17:431–6.
46. Teległów A, Mardyła M, Myszka M, Pałka T, Maciejczyk M, Bujas P, et al. Effect of Intermittent Hypoxic Training on Selected Biochemical Indicators, Blood Rheological Properties, and Metabolic Activity of Erythrocytes in Rowers. Biology [Internet]. 2022;11. Available from: http://dx.doi.org/10.3390/biology11101513
47. Kurhaluk N, Tkachenko H, Nosar V. The effects of intermittent hypoxia training on mitochondrial oxygen consumption in rats exposed to skeletal unloading. Ann Clin Lab Sci. 2013;43:54–63.
48. Kim S-W, Jung W-S, Kim J-W, Nam S-S, Park H-Y. Aerobic Continuous and Interval Training under Hypoxia Enhances Endurance Exercise Performance with Hemodynamic and Autonomic Nervous System Function in Amateur Male Swimmers. Int J Environ Res Public Health [Internet]. 2021;18. Available from: http://dx.doi.org/10.3390/ijerph18083944
49. Burtscher M, Pachinger O, Ehrenbourg I, Mitterbauer G, Faulhaber M, Pühringer R, et al. Intermittent hypoxia increases exercise tolerance in elderly men with and without coronary artery disease. Int J Cardiol. 2004;96:247–54.
50. Glazachev OS, Kryzhanovskaya SY, Zapara MA, Dudnik EN, Samartseva VG, Susta D. Safety and Efficacy of Intermittent Hypoxia Conditioning as a New Rehabilitation/ Secondary Prevention Strategy for Patients with Cardiovascular Diseases: A Systematic Review and Meta-analysis. Curr Cardiol Rev. 2021;17:e051121193317.
51. Sanchez-Gonzalez MA, May RW, Koutnik AP, Kabbaj M, Fincham FD. Sympathetic vasomotor tone is associated with depressive symptoms in young females: a potential link between depression and cardiovascular disease. Am J Hypertens. 2013;26:1389–97.
52. Taralov ZZ, Terziyski KV, Dimov PK, Marinov BI, Kostianev SS. Assessment of the impact of 10-day intermittent hypoxia on the autonomic control measured by heart rate variability. Physiol Int. 2018;105:386–96.
53. Han JN, Stegen K, De Valck C, Clément J, Van de Woestijne KP. Influence of breathing therapy on complaints, anxiety and breathing pattern in patients with hyperventilation syndrome and anxiety disorders. J Psychosom Res. 1996;41:481–93.
54. Rybnikova E, Samoilov M. Current insights into the molecular mechanisms of hypoxic pre- and postconditioning using hypobaric hypoxia. Front Neurosci. 2015;9:388.
55. Rozova K, Mankovska I. The effect of intermittent hypoxic training on lung and heart tissues of healthy rats. Pneumonol Alergol Pol. 2012;80:296–300.
56. Vogt M, Puntschart A, Geiser J, Zuleger C, Billeter R, Hoppeler H. Molecular adaptations in human skeletal muscle to endurance training under simulated hypoxic conditions. J Appl Physiol. 2001;91:173–82.
57. Geiser J, Vogt M, Billeter R, Zuleger C, Belforti F, Hoppeler H. Training high living low: changes of aerobic performance and muscle structure with training at simulated altitude. Int J Sports Med. 2001;22:579–85.
58. Navarrete-Opazo A, Mitchell GS. Therapeutic potential of intermittent hypoxia: a matter of dose. Am J Physiol Regul Integr Comp Physiol. 2014;307:R1181–97.
59. Serebrovska TV, Portnychenko AG, Drevytska TI, Portnichenko VI, Xi L, Egorov E, et al. Intermittent hypoxia training in prediabetes patients: Beneficial effects on glucose homeostasis, hypoxia tolerance and gene expression. Exp Biol Med . 2017;242:1542–52.
60. Mackenzie R, Maxwell N, Castle P, Brickley G, Watt P. Acute hypoxia and exercise improve insulin sensitivity (S(I) (2*)) in individuals with type 2 diabetes. Diabetes Metab Res Rev. 2011;27:94–101.
61. Ling Q, Sailan W, Ran J, Zhi S, Cen L, Yang X, et al. The effect of intermittent hypoxia on bodyweight, serum glucose and cholesterol in obesity mice. Pak J Biol Sci. 2008;11:869–75.
62. Chiu L-L, Chou S-W, Cho Y-M, Ho H-Y, Ivy JL, Hunt D, et al. Effect of prolonged intermittent hypoxia and exercise training on glucose tolerance and muscle GLUT4 protein expression in rats. J Biomed Sci. 2004;11:838–46.
63. Muangritdech N, Hamlin MJ, Sawanyawisuth K, Prajumwongs P, Saengjan W, Wonnabussapawich P, et al. Hypoxic training improves blood pressure, nitric oxide and hypoxia-inducible factor-1 alpha in hypertensive patients. Eur J Appl Physiol. 2020;120:1815–26.
64. Serebrovskaya TV, Xi L. Intermittent hypoxia training as non-pharmacologic
therapy for cardiovascular diseases: Practical analysis on methods and equipment. Exp Biol Med . 2016;241:1708–23.
65. University of North Carolina at Chapel Hill. Only 12 percent of American adults are metabolically healthy, study finds: Trends help sound alarm for efforts to lower associated risk of types 2 diabetes, heart disease and other complications. Science Daily [Internet]. 2018 Nov 28 [cited 2023 May 31]; Available from: https://www.sciencedaily.com/releases/2018/11/181128115045.htm
66. Tessema B, Sack U, König B, Serebrovska Z, Egorov E. Effects of Intermittent Hypoxia in Training Regimes and in Obstructive Sleep Apnea on Aging Biomarkers and Age-Related Diseases: A Systematic Review. Front Aging Neurosci. 2022;14:878278.
67. Song T, Chen M, Wang X, Zhu E, Xue Y, Wang J, et al. Intermittent hypoxia: Friend or foe on endothelial repair in mouse model. Exp Lung Res. 2021;47:211–25.
68. Serebrovskaya TV, Nikolsky IS, Nikolska VV, Mallet RT, Ishchuk VA. Intermittent hypoxia mobilizes hematopoietic progenitors and augments cellular and humoral elements of innate immunity in adult men. High Alt Med Biol. 2011;12:243–52.
69. Li G, Liu J, Guan Y, Ji X. The role of hypoxia in stem cell regulation of the central nervous system: From embryonic development to adult proliferation. CNS Neurosci Ther. 2021;27:1446–57.
70. Klein DF. False suffocation alarms, spontaneous panics, and related conditions. An integrative hypothesis. Arch Gen Psychiatry. 1993;50:306–17.
71. Ziemann AE, Allen JE, Dahdaleh NS, Drebot II, Coryell MW, Wunsch AM, et al. The amygdala is a chemosensor that detects carbon dioxide and acidosis to elicit fear behavior. Cell. 2009;139:1012–21.
72. Feinstein JS, Gould D, Khalsa SS. Amygdala-driven apnea and the chemoreceptive origin of anxiety. Biol Psychol. 2022;170:108305.
73. Wilhelm FH, Gevirtz R, Roth WT. Respiratory dysregulation in anxiety, functional cardiac, and pain disorders. Assessment, phenomenology, and treatment. Behav Modif. 2001;25:513–45.
74. Meuret AE, Wolitzky-Taylor KB, Twohig MP, Craske MG. Coping skills and exposure therapy in panic disorder and agoraphobia: latest advances and future directions. Behav Ther. 2012;43:271–84.
75. Blechert J, Wilhelm FH, Meuret AE, Wilhelm EM, Roth WT. Respiratory, autonomic, and experiential responses to repeated inhalations of 20% CO₂ enriched air in panic disorder, social phobia, and healthy controls. Biol Psychol. 2010;84:104–11.
76. Meuret AE, Rosenfield D, Wilhelm FH, Zhou E, Conrad A, Ritz T, et al. Do unexpected panic attacks occur spontaneously? Biol Psychiatry. 2011;70:985–91.
77. Gorman JM, Martinez J, Coplan JD, Kent J, Kleber M. The effect of successful treatment on the emotional and physiological response to carbon dioxide inhalation in patients with panic disorder. Biol Psychiatry. 2004;56:862–7.
78. Gorman JM, Fyer MR, Goetz R, Askanazi J, Liebowitz MR, Fyer AJ, et al. Ventilatory physiology of patients with panic disorder. Arch Gen Psychiatry. 1988;45:31–9.
79. Burtscher J, Mallet RT, Burtscher M, Millet GP. Hypoxia and brain aging: Neurodegeneration or neuroprotection? Ageing Res Rev. 2021;68:101343.
80. Zhu X-H, Yan H-C, Zhang J, Qu H-D, Qiu X-S, Chen L, et al. Intermittent hypoxia promotes hippocampal neurogenesis and produces antidepressant-like effects in adult rats. J Neurosci. 2010;30:12653–63.
81. Ding F-S, Cheng X, Zhao T, Zhao Y-Q, Zhang G-B, Wu H-T, et al. [Intermittent hypoxic preconditioning relieves fear and anxiety behavior in post-traumatic stress model mice]. Sheng Li Xue Bao. 2019;71:537–46.
82. Manukhina EB, Tseilikman VE, Karpenko MN, Pestereva NS, Tseilikman OB, Komelkova MV, et al. Intermittent Hypoxic Conditioning Alleviates Post-Traumatic Stress Disorder-Induced Damage and Dysfunction of Rat Visceral Organs and Brain. Int J Mol Sci [Internet]. 2020;21. Available from: http://dx.doi.org/10.3390/ijms21010345
83. Manukhina EB, Tseilikman VE, Tseilikman OB, Komelkova MV, Kondashevskaya MV, Goryacheva AV, et al. Intermittent hypoxia improves behavioral and adrenal gland dysfunction induced by posttraumatic stress disorder in rats. J Appl Physiol. 2018;125:931–7.
84. Jerath R, Crawford MW, Barnes VA, Harden K. Self-regulation of breathing as a primary treatment for anxiety. Appl Psychophysiol Biofeedback. 2015;40:107–15.
85. Balban MY, Neri E, Kogon MM, Weed L, Nouriani B, Jo B, et al. Brief structured respiration practices enhance mood and reduce physiological arousal. Cell Rep Med. 2023;4:100895.
86. Bentley TGK, Wilson R, MacKenzie B, Russell S. Anxiety, CO2 tolerance, and breath practice in high school students: feasibility and observations of a 6-week slow breathing program. ICIMH 2020 …. Poster presented at: International. 2020;
87. Banushi B, Brendle M, Ragnhildstveit A, Murphy T, Moore C, Egberts J, et al. Breathwork Interventions for Adults with Clinically Diagnosed Anxiety Disorders: A Scoping Review. Brain Sci [Internet]. 2023;13. Available from: http://dx.doi.org/10.3390/brainsci13020256
88. Fincham GW, Strauss C, Montero-Marin J, Cavanagh K. Effect of breathwork on
stress and mental health: A meta-analysis of randomised-controlled trials. Sci Rep. 2023;13:432.
89. Meuret AE, Ritz T, Wilhelm FH, Roth WT, Rosenfield D. Hypoventilation Therapy Alleviates Panic by Repeated Induction of Dyspnea. Biol Psychiatry Cogn Neurosci Neuroimaging. 2018;3:539–45.
90. Meuret AE, Rosenfield D, Seidel A, Bhaskara L, Hofmann SG. Respiratory and cognitive mediators of treatment change in panic disorder: evidence for intervention specificity. J Consult Clin Psychol. 2010;78:691–704.
91. Yamamoto Y, Mutoh Y, Kobayashi H, Miyashita M. Effects of reduced frequency breathing on arterial hypoxemia during exercise. Eur J Appl Physiol Occup Physiol. 1987;56:522–7.
92. Woorons X, Mucci P, Aucouturier J, Anthierens A, Millet GP. Acute effects of repeated cycling sprints in hypoxia induced by voluntary hypoventilation. Eur J Appl Physiol. 2017;117:2433–43.
93. Czuba M, Wilk R, Karpiński J, Chalimoniuk M, Zajac A, Langfort J. Intermittent hypoxic training improves anaerobic performance in competitive swimmers when implemented into a direct competition mesocycle. PLoS One. 2017;12:e0180380.
94. Trincat L, Woorons X, Millet GP. Repeated-Sprint Training in Hypoxia Induced by Voluntary Hypoventilation in Swimming. Int J Sports Physiol Perform. 2017;12:329–35.
95. Woorons X, Mollard P, Pichon A, Duvallet A, Richalet J-P, Lamberto C. Effects of a 4-week training with voluntary hypoventilation carried out at low pulmonary volumes. Respir Physiol Neurobiol. 2008;160:123–30.
96. Lapointe J, Paradis-Deschênes P, Woorons X, Lemaître F, Billaut F. Impact of Hypoventilation Training on Muscle Oxygenation, Myoelectrical Changes, Systemic [K+], and Repeated-Sprint Ability in Basketball Players. Front Sports Act Living. 2020;2:29.
97. Woorons X, Gamelin F-X, Lamberto C, Pichon A, Richalet JP. Swimmers can train in hypoxia at sea level through voluntary hypoventilation. Respir Physiol Neurobiol. 2014;190:33–9.
98. Czuba M, Waskiewicz Z, Zajac A, Poprzecki S, Cholewa J, Roczniok R. The effects of intermittent hypoxic training on aerobic capacity and endurance performance in cyclists. J Sports Sci Med. 2011;10:175–83.
99. Ramos-Campo DJ, Martínez F, Esteban P, Rubio-Arias JA, Jiménez JF. Entrenamiento en hipoxia intermitente y rendimiento ciclista en triatletas / Intermittent hypoxic training and cycling performance in triathletes. RIMCAFD [Internet]. Servicio de Publicaciones de la Universidad Autonoma de Madrid; 2016;61. Available from: https://revistas.uam.es/rimcafd/article/view/3781
100. de Asís-Fernández F, Sereno D, Turner AP, González-Mohíno F, González-Ravé JM. Effects of apnoea training on aerobic and anaerobic performance: A systematic review and meta-analysis. Front Physiol. 2022;13:964144.
101. Hundley ZT. Voluntary Hypoventilation at Low Lung Volume (VHL) During Strength and Conditioning Training Increases Anaerobic Performance: A Pilot Study. 2020 [cited 2023 May 31]; Available from: https://digitalcommons.liberty.edu/masters/702
102. Jung W-S, Kim S-W, Park H-Y. Interval Hypoxic Training Enhances Athletic Performance and Does Not Adversely Affect Immune Function in Middle- and LongDistance Runners. Int J Environ Res Public Health [Internet]. 2020;17. Available from: http://dx.doi.org/10.3390/ijerph17061934
103. Ponsot E, Dufour SP, Zoll J, Doutrelau S, N’Guessan B, Geny B, et al. Exercise training in normobaric hypoxia in endurance runners. II. Improvement of mitochondrial properties in skeletal muscle. J Appl Physiol. 2006;100:1249–57.
104. Malshe PC. Nisshesha rechaka pranayama offers benefits through brief intermittent hypoxia. Ayu. 2011;32:451–7.