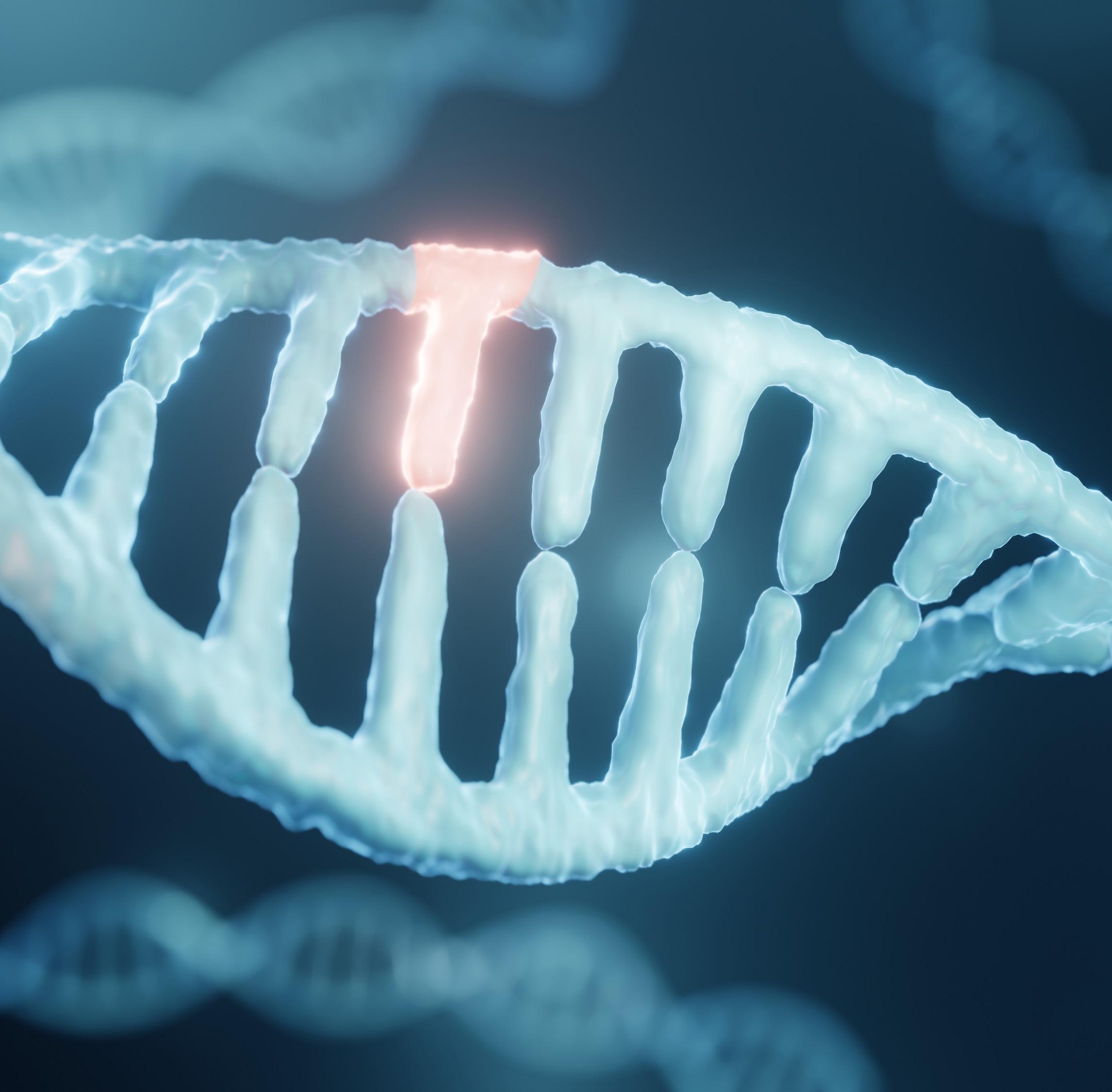
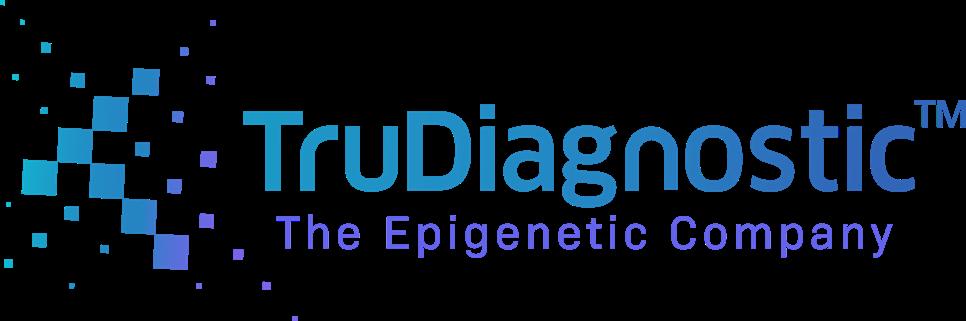
A beginner’s introduction to aging, epigenetics, and how they overlap
A beginner’s introduction to aging, epigenetics, and how they overlap
TruDiagnostic is a Health Data company. Our primary business is in research & development, human clinical trials, and other partnerships with academic and research institutions to expand the field of Epigenetics through a multi-omic approach.
We specialize in DNA Methylation, with a private laboratory to offer a full suite of aging relating metrics, including telomere length measurements, immune cell subset deconvolution, intrinsic and extrinsic age calculations, and much more.
Our laboratory also offers epigenetic biological age testing services to the public.
Not a researcher or scientist? Didn’t quite follow that list of lab processes? That’s fine! Actually, that’s really good! We’re glad you’ve gotten your hands on this book!
This book was written to help educate the general public (not just folks with a Ph.D.) about Epigenetics, Biological Age, and why it’s important to everyone - regardless of age. education, or experiance.
If you still have questions after reading this, there is contact information and our website at the end of this book, which offers even more information.
We’ll start with aging , to explain the stakes.
Aging is the #1 risk factor for nearly all chronic diseases. As people get older, their risk of developing diseases increases almost exponentially.
So it makes sense that if they could reduce the impact of aging, the medical field could theoretically reduce the likelihood of patients developing those late-age diseases.
In the next section, we’ll introduce epigenetics - our specialty, and our passion.
Basically, epigenetics is the interface between the outside world and the human genome. It is what helps genes turn on and off in response to outside influencesreshaping our most essential building blocks throughout our entire life. Humans might change the most while young, but we never actually stop changing.
Finally, we’ll dive into Epigenetic Biological Aging.
With the first two sections you should already have a good handle on what it is and what it means for you, but now we can dive even deeper into the types of information we can get from Biological Age by looking at DNA Methylation, and the different kinds of reports
We’ll also touch on what you can do to deliberately influence your aging process, based on the latest research.
It is time to classify biological aging as a disease. And with that classification, it enables us to target and treat aging rather than aging becoming unavoidable.
Bulterijs et al.
Have you ever met someone in their 70’s who seems to defy the aging process? Some people can still run marathons at that age, while others are bedridden. What’s the difference?
It often comes down to the core aging processes of your body - your biological aging. Biological aging is the process of your cells & internal systems slowly losing their ability to function.
For a long time, aging was widely viewed as a natural process, where a person’s body slowly degraded over time. They simply developed diseases later in life, which made them frailer and eventually passed away.
However, this isn’t really accurate. With further research, scientists found that early-life adversity could cause and even predict later disease.
The latest aging and longevity research finally shows that connection. Early adversity, like malnourishment or early trauma influences a person’s entire body – down to their genes. These aging markers create significant changes to the brain, immune system, and the rest of your body at a deeply fundamental level.
These changes speeds up aging, which is closely tied to disease occurrence and frailty.
Accelerated aging is the largest risk factor for chronic disease.
Since the weakening integrity of cells, tissues, and organs is what defines aging, accelerated or advanced aging contributes to the onset of various conditions and diseases.
In fact, accelerated aging is the main risk factor for developing conditions like cancer, diabetes, cardiovascular disorders (heart and blood), and neurodegenerative diseases (brain and nerves).
Let’s look at how cellular aging is defined by many longevity researchers, to understand how aging and disease are linked: the Hallmarks of Aging.
To study aging, you have to know what aging is. Not just what aging looks like, but the biological processes that are occurring to make it happen.
There are currently 9 generally accepted molecular markers of aging - or, 9 reasons why we age. These are called the hallmarks of aging.
This is a rising frequency of mutations happening during cell division. You have cells dividing within you 24/7 - the new cells are what replace dead cells, heal wounds, and carry out jobs that need to be done in your body.
Normally a couple cells with mutated DNA isn’t a problem. Your body is pretty good at catching them. But scientists have found that a higher rate of genomic instability is connected to premature aging. The mutations are happening too often for your body to catch them all, and they build up.
Telomeres are protective caps that sit on the end of our chromosomes. They protect your DNA during cell division - basically signaling the end of the chromosome. Every time a cell divides, a little bit of that telomere is shaved off.
Telomere attrition (or telomere shortening) is the gradual loss of those caps. This mechanism limits the number of times our cells can divide - even in vital organs like the liver and heart. If the telomere is gone completely, then instead of shaving off a bit of a cap when dividing a cell, that division would instead shave off a bit of the DNA itself, changing its “recipe”
Unfortunately, only stem cells, egg cells, and sperm cells can repair their end caps. Regular cells do not.
When a cell’s telomeres are too short, the cell has the option to go into ‘Senescence’ or be dissolved and re-use for parts.
Cellular senescence is a condition where the cell no longer has the ability to divide. They don’t replicate, but they’re still using resources. The main reason we have cellular senescence is to prevent cells that have damaged DNA from replicating.
It’s a fantastic mechanism to prevent cancer (where a mutated or damaged cell replicates out of control) and heal wounds. However, senescent cells may also refuse to begin ‘apoptosis’ - the process where the cell recognizes that it cannot do its job anymore, and dies - letting the immune system break it apart. Senescence, at appropriate times, can be helpful. Excessive cell senescence can be very harmful, triggering chronic inflammation and even suppressing the immune system.
Cells have to be able to communicate with each other clearly, throughout the body, in order to coordinate their many complex jobs.
When the checkpoints and communication systems become exhausted, delayed, or skipped entirely, that can cause very damaging cascading effects in the body. One example is cancer: when a cell gains the ability to grow and divide, ignoring the cellular signals to stop.
Many disease treatments rely on cell communication. For instance, several drugs that treat asthma actually mimic natural signals that tell muscle cells in the lungs to relax, allowing breathing passages to open.
“The mitochondria are the powerhouse of the cell” - they create energy that cells can use. Mitochondria are also a key player with intercellular communication, calcium balance, and metabolism in general. Since mitochondria play a critical role in all organ systems, they are particularly impacted by disease.
When your mitochondria aren’t working well, your cells can’t get enough energy to replace what they use up. This is why a lot of chronic diseases come with persistent fatigue - even your cells don’t have enough energy to do everything that needs to be done.
When your proteostasis is off-balance or reduced, the protein-building machinery inside your cells aren’t working properly. They’re mis-folding, and proteins rely on their shape to do their job. With the wrong shape, they can’t do their jobs - and may even cause problems by doing the wrong job entirely.
Nutrient-sensing pathways are essential for the aging process. Your cells rely on getting the right nutrients to the right spots, so they can build things like proteins, give food to mitochondria, and perform basic functions like movement & sending signals to other cells. If your cells don’t know what nutrients they have, or what nutrients they’re missing, then they won’t be able to complete their normal functions.
Many of the genes that act as key regulators of lifespan also have functions in nutrient sensing.
Many of our cells can self-replicate - making copies of themselves to help replace damaged or old cells. However, there’s a limit to how many times a single cell can replicate. 50-60 replications seems like the current limit. Once a cell reaches the end of its lifespaneither through damage or because it can no longer replicate, then it needs to be replaced entirely.
This is where stem cells come in. Stem cells can change into any other type of cell. However, stem cells can decline in number, and lose their ability to renew themselves. Without stable populations of stem cells, tissues and organs lose their ability to recover from damage, and begin to fail. Healing takes longer, when there are fewer stem cells to supply fresh cells.
There are areas on your DNA called ‘genes’ which code for RNA. RNA carries instructions about how to build proteins, how to stop protein synthesis, and other cell-controlling functions.
An epigenetic alteration is something that changes how genes are read, without changing the structure of the genes themselves.
Since many of the previous hallmarks of aging are managed by gene expression, and improper management of gene expression can cause problems throughout all the different hallmarks, keeping your epigenetics on-track is important.
Each of these hallmarks tie into the acceleration of aging, and slowing or reversing these hallmarks after they appear can show a positive effect on a person’s health and longevity.
All of the hallmarks of aging influence each other. Understanding how they are interconnected is paramount to how we treat aging and age-related diseases.
People have long been fascinated by the fact that some people seem to age rapidly in terms of health and appearance, while others age more slowly.
How old you are doesn’t necessarily correlate to how your body is aging at a cellular level. After all, there are 70-year-olds who run marathons, and 25-year-olds with greying hair and chronic mitochondrial dysfunctions that make it hard to get out of bed.
Being 30 years old isn’t a guarantee that your stem cells are vigorously healthychronological age and biological age (the Hallmarks of Aging) are only loosely tied together.
As time passes, there are more chances for errors to occur in things like epigenetic management of genes, or protein misfolds. After all, your body folds trillions of proteins throughout your life. You get millions of new cells, each with an incredibly complex system that needs to be perfectly replicated each time.
There’s a lot of chances to make a mistake, each year you’re alive. The more years you’ve been alive, the more it’s likely that your cells have goofed a couple hundred times, and that’s starting to build up.
However, there are also changes that are made to aging that happen in response to what you’ve lived through.
Things like extreme stress, excessive alcohol consumption or smoking, chronic dehydration, radiation can actually change how our genes are expressed. These changes are called epigenetic alterations, which have a cascading effect on many other hallmarks of aging. Epigenetic alterations can become outwardly visible, but they are often subtle changes throughout organs and tissues. They manifest in little tweaks to how well a cell can fight off the development of tumors, or how well it can regulate its own nutrient uptake.
Finding a reliable way to measure and track how your lifestyle affects aging has become the next great enterprise in integrative and preventive medicine.
A biomarker is a diagnostic tool that uses a change in the body to indicate something else, like general health or disease progression. Typically, a biomarker is found in blood or other tissue. A biomarker can indicate normal or abnormal processes. For example, your doctor may track blood pressure and cholesterol levels. These are two biomarkers which can indicate heart health.
To track biological aging, which happens in every cell across your body, you need some sort of marker that is present across the entire body, which can provide clinically useful information about the progression of the hallmarks of aging.
Since the 1980’s, it has been recognized that valid and reliable biomarkers of aging are needed to achieve the longstanding goal of understanding, slowing, and reversing the process of aging. After decades of research, a few notable biomarkers have been found, including telomere length, transcriptome profiles, protein and lipid metabolism, to name a few. All of these biomarkers have been used to determine biological age, yet none of them qualify as good biomarkers
A good biomarker needs to be:
• Able to provide clinically relevant information
• Objective - not swayed by a bias
• Quantifiable - translates to specific data, not vague ideas.
• Precise - When measuring the same item over and over, the measurements will be very similar.
• Accurate - Accuracy refers to how close a measurement is to the ‘true’ or accepted value.
A reliable biomarker, once extensively studied and replicated, leaves little room for error. If the biomarker you are using to assess a biological function leaves space for doubt, it is probably not a good diagnostic tool.
A good biomarker for aging would differentiate people who are of the same chronological age, yet have different rates of biological aging. They also would have been studied across a variety of demographics, over an extended period of time.
Quantitative biomarkers of aging should be able to define a group of measurements for ‘healthy aging’. It should be able to predict the outcomes of healthy or unhealthy aging. It should be able to narrow down a specific biological feature to explain the biological process behind aging and aging-related diseases.
And this is how researchers narrowed in on Epigenetic Biological Aging.
We mentioned epigenetic modifications briefly in ‘Hallmarks of Aging’ - let’s dive into it further.
Each of your cells contains a copy of your 22,000 genes, in the form of tightly-coiled DNA. These genes contain the instructions for every cell shape, cell process, protein, and primal instinct hard-coded into your brain.
However, you aren’t made up of trillions of identical cells - each cell type has a unique appearance, and a unique function. Skin cells cannot act like liver cells. Nerve cells cannot twitch and contract to pull your limb like muscle cells.
How is a skin cell different from a liver cell, if they each have the same copy of the same DNA?
The answer is ‘epigenetic changes’
Epigenetic changes alter how portions of the DNA - your genes are read and accessed by the body. It can turn genes ‘off’, or only allow part of a gene to be read. Inside a skin cell, the genes that explain how to operate as a liver cell are turned off and tucked away so it doesn’t interfere with ‘being a skin cell.’
The DNA itself is not changed by epigenetics, just like you don’t change the shape or contents of a lightbulb by turning it ‘on’ or ‘off’.
Identical twins are a perfect example of epigenetics changes. Although identical twins share exactly the same DNA, their unique experiences in life will cause some genes (and not others) to express themselves. This is why, over time, identical twins come to look and behave differently.
There are 2 main types of epigenetic modifications.
First, is histone modification
Histones are little proteins that help your DNA get bundled away when it’s not in use.If your DNA was a thread, then the histones would be spools that the thread wraps around. However, these spools have the ability to change shape, changing how tightly the thread is wound around it. When they coil tighter, the DNA that’s wrapped around them becomes inaccessible. When a histone loosens up, the DNA becomes a bit more accessible.
With many many histones, they help customize which parts of the DNA are capable of being read.
Next is DNA Methylation.
A ‘methyl’ is a small molecule that is created through natural cell processes, and is present throughout your body. It can attach to certain spots on your DNA and act as a sort of genetic white-out. It changes gene expression by changing what areas on the DNA can be transcribed, one nucleosome at a time. When a methylated spot is encountered, the polymerase protein that reads DNA and turns it into RNA will just… skip over that spot.
This means that methylation changes to DNA will translate to every other function in the body, since all other functions rely on instructions from the DNA to begin.
If methylation occurs on the wrong spots:
• The recipe for a protein may be translated incorrectly - resulting in protien misfolding.
• The proteins that help cells detect nutrient levels may not be created
• The proteins that move energy from mitochondria to the rest of the body may be too slow, or too few in number.
• It may prompt cells to begin replicating out of control, because the genes that code for proteins to suppress tumors were accidentally turned off.
DNA Methylation influences all other forms of biological aging, so it’s only natural to examine methylation to learn more about aging - both measuring it, and predicting it. Discovering how integral DNA methylation was to systems of biological aging has completely revolutionized our understanding of chronic disease development.
DNA Methylation is subtly influenced by nearly everything you do in life. It’s actually quite impressive, the number of factors researchers have found to influence gene expression through methylation. Environment and lifestyle can directly interact with your genome, through methylation.
Known factors that can infulence methylation patterns:
• Sleep Habits
• Diet and nutrition
• Various medications
• Stress- both acute and chronic
• Exercise habits & activity
• Drinking alcohol
• Smoking
• Heavy metals
• Airborne pollution
• Altitude
• And much more...
Methylation is a crucial tool your body uses to adapt to the world around you, by changing gene expression throughout your life. Because those changes to gene expression can change any part of your body, it can also affect any of the hallmarks of aging. So, your methylation also changes how you age.
By examining methylation, we can get an up-close look at how your body is self-regulating - and how well it’s aging.
Methylation markers are on your DNA, and each mark is so small that it barely changes the profile of a nucleotide (the building blocks of DNA). If you stretched the DNA in one cell all the way out, it would be nearly 6 feet long! That’s a lot of methylation to sort through.
Since they’re so tiny and numerous, the process of extracting DNA and then analyzing it for patterns of methylation requires incredible precision.
The tiny amount of fluid in this vial contains DNA filtered out from thousands of cells.
At TruDiagnostic, since we focus on research into the epigenome, we use the most in-depth procedure possible - Bisulfite Conversion and the 850k BeadChip by Illumina. What this means is that we analyze over 850,000 points where DNA methylation occurs, each time we run a sample. This is the largest possible amount with the current level of technology.
It is non-targeted data collection, which means we’re collecting methylation points across the entire genome - not just those specifically related to aging.
We do this because epigenetic methylation is such a new field of study. While we have identified most of the places where DNA methylation can happen, there are a lot of areas where methylation happens but no one knows what it does, or how it influences the body.
By collecting as much data as we can, both on the patterns of methylation and on the information that our customers voluntarily provide about their own lifestyle, we are building a private database of correlations between methylation patterns and lifestyle habits. This allows our team to use powerful bioinformatics tools to sort through and find the patterns that can reveal how our lifestyles impact DNA methylation - and our aging.
When we notice a pattern in methylation that appears strongly correlated to some part of our lifestyles, we can partner up with an academic research institution or hospital to design a clinical trial to examine the methylation patterns before-and-after the treatment we suspect may influence aging.
So, while only 2,000 or so methylation points are widely regarded as the primary markers of aging in humans, we know that thousands of other points have cascading and interconnected effects on those ‘aging’ methylation patterns.
Since epigenetics is such a quickly-growing scientific field and area of interest for people in the fields of longevity, biohacking, health & wellness, a lot of new companies are popping up who offer to measure your biological age. They often test 2,000 markers or less, and only offer your ‘biological age’ - a metric that is, on its own, not actually very helpful to guide age-reducing behaviors.
Wait, I thought biological age was the definition of aging! Well, yes, but also: it’s complicated.
Remember; each cell type has a genetic expression that is unique to that type of cell, and so the methylation patterns that indicate ‘hallmarks of aging’ will also be specific to that cell. There are some universal markers, but there are also many differences.
This is for three main reasons:
First: DNA Methylation is one of the epigenetic mechanisms that your body uses to change gene expression & turn stem cells into different cell types. So, by their very nature, a skin cell will have a different methylation pattern than a lung cell or a brain cell.
Second: DNA Methylation is affected by the local environment and the experiences of that cell. Your skin, for instance, is exposed to UV radiation from the sun, and is constantly fighting off bacteria, viruses, and chemicals that you touch every day. It can also experience damage from scratches, sunburn, drying out, and so on.
Your liver, however, is only exposed to things that have already entered your body. What food you ate, what medicines you took, and so on.
Your brain, meanwhile, is protected by the blood-brain barrier and your skull. It’s never exposed to sunlight (hopefully) and there’s a strong defense between bloodborne contamination and its cells.
Brain cells will almost always measure ‘younger’ than other cell types, while liver and skin cells tend to be ‘older’, since they are our body’s primary way of defending against incoming damage and removing harmful substances from our body. They take on more damage, so they age faster.
Finally: We use algorithms to look through the many methylation points, analyze their patterns, and from that data create a ‘biological age’ metric. Almost all of these algorithms are trained on whole blood.
This means that, as the algorithm was being created, the methylation points all came from cells found in the blood. Primarily white blood cells, since red blood cells and platelets do not have a nucleus, and so do not have any DNA in them.
So, the main ‘cell type’ that is being analyzed to build these algorithms is actually from the immune system, plus some stem cells that haven’t yet differentiated into another cell type.
(It also means that tests which use a different cell type, like saliva, but use a bloodtrained biological age algorithm will have results that aren’t as accurate or precise as they could be, if they used blood instead. Saliva and blood both have white blood cells, but saliva will also have many epithelial cells, from your cheek and tongue. )
Deconvolution refers to a process of separating to heterogeneous mixture into its constituent components.
‘Candy deconvolution’ is what you’re doing when you sort your mixed color candies into piles of red, orange, yellow, green, etc. They go from an evenly blended mixture into individual groups. You can then count the number of red candies and compare it to the number of blue candies.
Cellular deconvolution is the process of identifying ratios of cell-types in the blood sample that is being used for epigenetic analysis.
When you’re having an immune system response like fighting off a bacteria or virus or allergen - or if you have an immune disorder like diabetes, lupus, or celiac disease, the ratio of different types of white blood cells in the body will change.
By knowing the ratio of cells that’s in the sample we’re working with, we can separate biological age into ‘Intrinsic Biological Age’ and ‘Extrinsic Biological Age.’
Learn more about those two measurements of aging in the next section!
Extrinsic Epigenetic Age shows the age of your entire body, including your Immune System. It is a more accurate version of Epigenetic Biological Age, analyzing cell-type ratios during calculation to ‘balance’ the biological age result into something that is more representative of your body’s average biological age. Extrinsic Epigenetic Age isn’t as weighted toward the immune system.
Intrinsic Biological Age shows the baseline age of your body, if the immune system’s aging wasn’t factored in at all - or if it was removed entirely during calculation. It only looks at the ‘universal’ changes in methylation that occurs in all cell types, thought to be our most basic mammalian aging.
This creates distance between the average aging of your other systems, and the aging your immune system has endured due to things like allergens, diseases, and immune disorders.
By comparing the original ‘Biological Age’, ‘Extrinsic Biological Age’ and ‘Intrinsic Biological Age’ together, you can get a picture of your actual immune system’s aging.
With all three metrics compared side-by-side, you can get a good idea if your immune system is in pretty good shape, or if you should focus on strengthening your immune system as much as you can in order to try to slow your rate of aging.
Remember how we mentioned ‘Telomere Length’ was a hallmark of aging? As it turns out, DNA methylation keeps very good track of how long your telomeres are.
Telomeres are repeating sequences of nucleotide base-pairs at the very end of all your chromosomes. They don’t carry your essential genetic data, but they do protect it. If we didn’t have telomeres, genetic information from the DNA on the ends of the chromosomes would be lost every time a cell divided.
Of course, your body generally doesn’t want to harm your genetic code. When the telomeres reach their shortest length, your cells take that as a sign to stop replicating. When a cell stops replicating, but sticks around to use up nutrients instead of being broken down by the body, that’s called cellular senescence.
Cell replication is necessary for healing and growing, so this process of telomere shortening and eventually stopping replication is widely accepted to be one of several factors that causes tissues to age.
What happens to your cells at the end of the cell’s lifespan – and how quickly they arrive at that end – is an important indicator of the aging process and a wide variety of diseases.
Shorter telomere length and low telomerase activity are associated with several chronic preventable diseases. These include hypertension, cardiovascular disease, insulin resistance, type 2 diabetes, depression, osteoporosis, and obesity. It’s also implicated in genomic instability and oncogenesis (the formation of cancer, where normal cells are transformed into cancer cells.)
Therefore, tracking the rate of telomere shortening is critical to tracking the overall progression of health and aging.
Telomere Length offers another metric to reveal your body’s aging process, a type of biological age marker focused on cellular replication and cellular fate.
So far, all the forms of biological age measurements we have looked at have been static. A static clock like our normal biological age or immune age measurements only show how much your body has aged, over your entire life. The ‘distance’ you’ve aged.
Let’s look at an example:
Smoking can result in accelerated epigenetic aging. At certain parts of the AHRR gene, smokers tend to have less DNA methylation than non-smokers.. The difference is even greater for heavy smokers and long-term smokers.
After quitting smoking, former smokers can begin to have increased DNA methylation at this gene. Eventually, they can reach levels similar to those of non-smokers. In some cases, this can happen in under a year, but the length of time depends on how long and how much someone smoked before quitting. The change takes time.
If you already smoked for 20 years, your rate of aging has likely been accelerated for a long time - your biological age is likely to be notably higher than your chronological age.
So let’s say, hypothetically, you’re 38 years old. You’ve been smoking since you were 18. The effect of heavy smoking over 20 years means your epigenetic biological age reads ‘49.2.’
You decide to quit smoking, and you manage to do so. Congratulations! 4 months later, you test again. Your epigenetic biological age now reads ‘49.4’.
But wait! You quit smoking! Why did it go up?
Well, there’s the baseline rate of aging to consider. Plus your diet, exercise and sleep habits, and many other factors that change aging.
Static ‘lifetime’ measurements of aging don’t show if your lifestyle changes are affecting aging, short-term. If you waited 2 or 3 years and measured each year, you’d be able to track a shift in the difference between each number. As aging slows, your biological age would increase by less each year.
However, 3 years is a huge time commitment. What if you actually increased your aging acceleration? You’d want to know right away, so you could do something about it, right?
That’s where DunedinPACE comes in.
A team of researchers from Duke and Columbia worked together to create a powerful algorithm called DunedinPACE. DunedinPACE shows how fast you’re currently aging. It’s a ‘speedometer’ for aging. How quickly are you aging, right now?
This algorithm evaluates your current patterns of methylation and compares it to nearly 50 years of longitudinal data.
It offers a ‘speed’ metric, where being above 1 is accelerated aging, and being below 1 is decelerating, or actively slowing down aging. The further away from 1 you are, the stronger the effect of acceleration or deceleration.
Instead of years, changes in methylation can be detected by DunedinPACE in months. This means you can try a new lifestyle change, use DunedinPACE to check if it’s working to slow your aging, and then decide how to proceed from there!
DunedinPACE doubles as an incredibly powerful predictive tool. Dr. Eileen Crimmons in USC evaluated DunedinPACE, and found it was the most predicative age-related biomarker for health consequences.
Since age acceleration is so closely tied to disease development, this algorithm can identify when someone is aging faster - and therefore have a greater long-term risk of poor health, developing chronic diseases, or dying prematurely. Similarly, people who the algorithm identified as aging slowly later performed significantly better on tests of balance, strength, and mental ability.
Someone who had a slower pace of aging generally did better on memory and intelligence tests, and had better measures of brain health. Chronically accelerated aging created both a notable loss of cortical thickness and reduced surface area of the brain.
Being even slightly above 1 on this metric can increase your risk of death in the next 7 years by 56%, and can increase your risk of chronic disease diagnosis by 54%
Age-related diseases studied in the Dunedin trials didn’t appear suddenly. Many could be predicted decades ahead of time, by tracking markers of accelerated aging. This means folks could begin acting to prevent those diseases, decades ahead of time.
Even at 3 years old, participants in the Dunedin trials found that pace of aging predicted health outcomes they’d face at age 45. Changes to pace of aging were especially impactful when young.
This emphasizes that people of all ages should pursue a slower pace of aging, to have a longer life with fewer age-related diseases.
This incredible tool is available to researchers investigating longevity and health outcome prediction related to aging. It is also available for YOU. TruDiagnostic offers the DunedinPACE algorithm in publicly available biological age testing kits.
TruDiagnostic is on the cutting edge of epigenetics research. We are constantly updating our test to report out as much information as possible on your health and aging states.
Some of the health reports we currently offer include looking at your weight loss response to caloric restriction, your risk of certain age-related diseases, and how much alcohol consumption has impacted your aging.
Aging is not a unidirectional, unstoppable process. Epigenetic aging can be slowed. It can be reversed.
Slowing epigenetic aging has been shown to delay or prevent the onset of diseases like diabetes, cardiovascular disorders, and neurodegenerative diseases. Tracking epigenetic aging allows individuals to monitor a summary of their overall health, as it relates to the risk of chronic disease formation. It allows them to track how their own lifestyle changes affect their aging and associated risks.
Some studies have even found that epigenetic reprogramming in blinded mice could force damaged ‘old’ cells to revert to a younger state and begin repairing damage to optic nerve cells that we previously thought was irreparable.
Research has shown that stressors like intermittent fasting can prompt your body to activate genes that help repair broken DNA and protect chromosomes. Hundreds of studies have shown that dietary restriction activates multiple molecular pathways that promote proteostasis, genome stability, stress resistance, and stem cell function.
Vitamin B has been shown to offer protection against the epigenetic effects of pollution, and Vitamin D helps regulate the expression of over 1,000 genes across most human tissues and cell types.
Epigenetics is on the cusp of revolutionizing preventive and integrative medicine. It’s not just about looking younger or feeling better, though those are wonderful benefits.
Health isn’t just the absence of disease. Optimizing epigenetic health and longevity means helping your entire body - down to its smallest molecular components - work its absolute best.