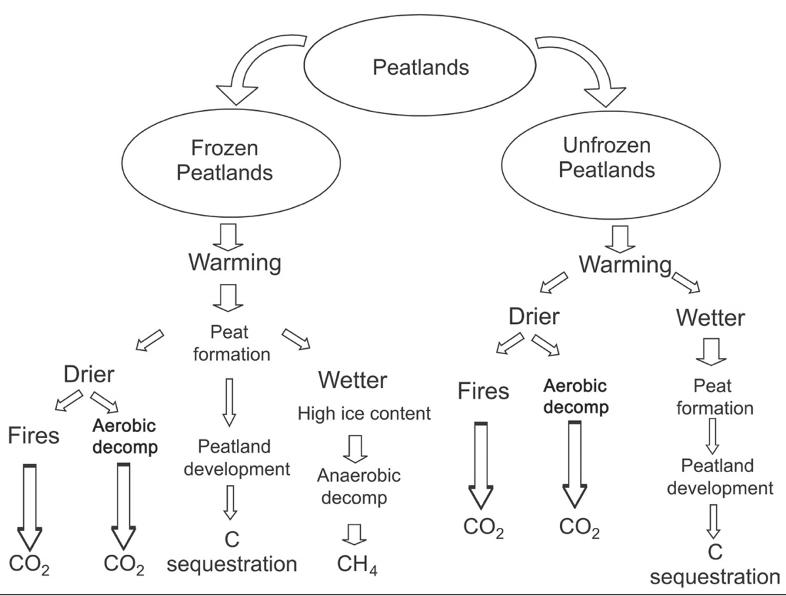
13 minute read
Rising Heat and Draining Peat: Anthropogenic Impacts on Northern Peatland Landscapes and Their Consequences for Global Climate Forcing
from Field Notes Volume X
by Field Notes
ABSTRACT
Despite comprising only 3% of Earth’s total surface area, peatlands store approximately half of the global atmospheric carbon dioxide stock1. This has significant consequences in regulating global carbon feedback mechanisms, and ultimately climate as a result. Given the global increase in greenhouse gas emissions in the atmosphere as a result of anthropogenic activities since the onset of the industrial revolution, the question of what role peatlands will play in the global carbon budget moving forward remains a relevant area for research. Anthropogenic warming of peatlands distributed among the vulnerable Boreal and Subarctic regions poses a great threat to their capacity as a global carbon sink and the possibility of long-term environmental change. In addition to carbon, arctic peatlands in particular are important stores of other potent greenhouse gases, notably methane and nitrous oxide, with the consequence of further emissions under rapidly warming conditions. Further, the biogeochemical control stabilized by the presence of sphagnum moss in northern peatland ecosystems may be undermined under a rapidly warming arctic and subarctic. How the degradation of peatlands as a whole will shift from a sink to a potential source of greenhouse gases in the coming century without the implementation of mitigation strategies implies a potential reversal in this once important climate-cooling negative feedback mechanism.
Advertisement
1 Nancy B. Dise, “Peatland Response to Global Change,” Science 326, no. 5954 (June 2009): pp. 810-811, https://doi.org/10.1126/ science.1174268, 810.
2 Jens Leifeld and Lorenzo Menichetti. “The Underappreciated Potential of Peatlands in Global Climate Change Mitigation Strategies.” Nature communications 9, no. 1 (2018): 1-7. INTRODUCTION
Peatlands are widely distributed across the planet, found primarily in boreal and temperate ecosystems and to a lesser extent in the tropics2. Their range largely reflects the Last Glacial Maximum, initiated by warmer growing seasons and enhanced primary productivity in deglaciated landscapes3 . These histosols, or organic soils, develop where local conditions favor an accumulation of organic material combined with large precipitation such that decayed organic matter exceeds the rate of decomposition4. The largest system is found in the Western Siberia Lowlands, with other important peatland locations spread across Northern Europe, North America and Pata-
3 Paul J Morris et al. “Global Peatland Initiation Driven by Regionally Asynchronous Warming.” Proceedings of the National Academy of Sciences 115, no. 19 (2018): 4851-56.
4 Dise, “Peatland Response,” 810.
5 Morris, “Global Peatland Initiation,” 4851.
6 Dise, “Peatland Response,” 810
7 Ibid.
8 Ibid.
9 Leifeld and Menichetti. “The Underappreciated Potential of Peatlands,” 2.
10 Tobi A. Oke and Heather A. Hager. “Plant Community Dynamics and Carbon Sequestration in Sphagnum-Dominated Peatlands in the Era of Global Change.” Global Ecology and Biogeography 29, no. 10 (2020): 1610-20
11 Vincent Jassey et al. “Above‐and Belowground Linkages in Sphagnum Peatland: Climate Warming Affects Plant‐Microbial Interactions.” Global Change Biology 19, no. 3 (2013): 811-23.
12 Jassey. “Above‐and Belowground Linkages,” 812.
13 Yehui Zhong et al. "Effects of Water Level Alteration on Carbon Cycling in Peatlands." Ecosystem Health and Sustainability 6, no. 1 (2020): 1806113.
14 Dise, “Peatland Response,” 811
15 Ibid. gonia5. The accumulation of organic matter in peatlands is largely a function of their waterlogged conditions, with very slow decomposition rates allowing for decayed organic matter to accumulate6. This process of delayed anaerobic decomposition releases the potent greenhouse gas methane, though its role in long-term atmospheric carbon storage counteracts the short-lived methane emissions, creating a net cooling effect7 . While comprising only 3% of total land surface cover, peatlands are responsible for storing up to half as much carbon as found as carbon dioxide found in the atmosphere8. Despite this, peatlands are degrading their ability to retain the carbon they have accumulated for thousands of years. The processes of warming, changing precipitation patterns, and draining of these histosols all pose threats to shifting land cover towards grasslands or shrublands, thereby reducing their capability to act as a global carbon sink. Presently, anthropogenic activities are draining or mining roughly 10% of global peatlands, creating carbon loss pathways and releasing significant amounts of nitrous oxide in the process9. These, in turn, have the potential to see peatlands shift from a net sink to a net source of greenhouse gases in the near future, with further ramifications as a positive global warming feedback mechanism. Further, climate warming is projected to shift the dominant vegetative cover of northern peatlands, ultimately undermining their characteristic ability to sequester carbon10. Notably, peatlands characterised by the presence of sphagnum mosses store the most carbon out of any terrestrial ecosystem11. Under accelerated warming conditions, the biogeochemical cycling traditionally stabilized by the mosses will be undermined, with the potential for positively feedback towards global warming12.
RESULT
Undisturbed peatlands act as important net sinks for atmospheric carbon dioxide globally. Presently, peatlands store roughly 20-30% of the Earth’s total stock of soil organic carbon, with boreal peatlands in particular having sequestered approximately 270 to 547 Gt of carbon since the Last Glacial Maximum (LGM)13. This ability to sequester carbon has, however, declined over the course of the past 1,000 years owing to shifts in climate that have led to greater flooding14. This trend is likely to continue, given that peatland distribution is highly concentrated in the boreal and subarctic Northern Hemisphere, where the process of warming is accelerated15. Over the course of the next century, it is predicted that the boreal forest and tundra are likely to see upwards of a 5°C temperature increase16. Warming, combined with other anthropogenically-driven climatic effects such as changing precipitation
patterns and an increase in extreme weather events, is likely to amplify this reduction in carbon sequestration capacity. Peatlands are widely distributed across Subarctic and Boreal regions, creating a variation between perennially frozen and unfrozen soils. The influence of rising temperatures differs between these two landscapes, though in either case it acts as a catalyst for complex carbon and methane interactions between the surface and the atmosphere. For perennially frozen peatlands, the melting of the peat in combination with rising temperatures is likely to amplify the waterlogged nature of these landscapes, creating ripe conditions for anaerobic decomposition and subsequent accelerated methane emissions (Figure 1)17. In unfrozen peat, typically found towards the south of the Boreal region, the primary risk is increased wildfires brought on by periods of drought leading to increased aerobic decomposition, ultimately increasing carbon dioxide emissions (Figure 1)18. While it has been suggested that the higher temperatures may promote peat accumulation and associated carbon sequestration, this could be offset by a nutrient deficit in these regions that may undermine any attempt at negatively contributing to the greenhouse gas climate feedback19. In either case, the warming at northern latitudes is projected to, first, reduce the capacity of the peatland landscapes to sequester carbon, and ultimately become a source of net emissions of carbon dioxide and methane (Figure 1).
16 Philip Camill and James Clark. “Climate Change Disequilibrium of Boreal Permafrost Peatlands Caused by Local Processes.” The American Naturalist 151, no. 3 (1998): 207-22.
17 Charles Tarnocai. “The Impact of Climate Change on Canadian Peatlands.” Canadian Water Resources Journal 34, no. 4 (2009): 453-66.
18 Tarnocai. “The Impact of Climate Change,” 461.
19 Tarnocai. “The Impact of Climate Change,” 263.
20 Ibid, 462.
Figure 1 Potential emission and/or carbon sequestration pathways under warming temperatures in frozen and unfrozen peatlands20 .
21 Carolina Voigt et al. “Increased Nitrous Oxide Emissions from Arctic Peatlands after Permafrost Thaw.” Proceedings of the National Academy of Sciences 114, no. 24 (2017): 6238-43.
22 Voigt et al. “Increased Nitrous Oxide Emissions,” 6238.
23 Ibid.
24 Ibid, 6241.
25 Jassey. “Above‐and Belowground Linkages,” 812.
26 Lilli Zeh et al. “Vascular Plants Affect Properties and Decomposition of Moss-Dominated Peat, Particularly at Elevated Temperatures.” Biogeosciences 17, no. 19 (2020): 4797-813.
27 Oke and Hager. “Plant Cmmunity Dynamics,” 1611.
28 Ibid.
29 Ibid.
30 Ibid, 1613.
31 Zeh et al. “Vascular Plants,” 4798. Further, arctic peatland landscapes are underlain by significant stocks of nitrogen. Under warming conditions, more than 67 billion tons of nitrogen may become more readily available to be decomposed and emitted as the potent greenhouse gas, nitrous oxide21. As the permafrost melts, the organically fixed nitrogen undergoes mineralization, forming ammonium and nitrate, leading to the processes of nitrification and denitrification that produce the nitrous oxide emitted by these soils22. With a radiative forcing 300 times stronger than carbon dioxide, nitrous oxide poses a significant threat as a catalyst for further global-warming-related consequences23 . This greenhouse gas contribution is in addition to the already outstanding emissive potential of the global carbon and methane stocks present in permafrost. Though further investigation is required to determine how the role of soil moisture, vegetation, and local hydrology will either speed up or slow down mineralization and nitrification, it has been proposed that dried peat will release more nitrous oxide under aerobic conditions24 . This, combined with the long-term steady influx to the atmosphere brought about by a gradual thawing of the permafrost, has the potential to contribute positively to radiative forcing alongside carbon and methane emissions. Moreover, the effects of climate change are likely to lead to a shift in northern peatland vegetation structure, which will result in compromised carbon sequestration ability. Sphagnum mosses, a dominant vegetation of peatland landscapes, engineer strict conditions to regulate biogeochemical cycles and carbon feedbacks25. Under warming temperatures, landscapes are projected to see a decrease in sphagnum moss cover, shifting towards shrub-dominated vegetation26. This would be particularly disruptive to the global carbon budget, given that sphagnum accounts for roughly 50% of carbon in northern peatlands27. Traditionally, the rate of carbon sequestration in peatlands is regulated by sphagnum, whose ability to maintain waterlogged, acidic and anoxic conditions ensures the slow rates of decomposition28. However, the coupled effects of anthropogenic climate warming and N-deposition are projected to lower water table levels, compromising these conditions29. This lowered water table and greater peat aeration brought about by rising temperatures are expected to stimulate microbial activity and increase the rate of decomposition in northern peatlands, thus creating conditions for the emergence and dominance of vascular plants30. A systematic change in vegetation cover poses the risk of releasing the stored organic carbon as carbon dioxide, ultimately undermining the characteristic ability of peatlands to sequester carbon altogether31 . While anthropogenic emissions related to industry strongly contribute to the acceleration of climate change, the process of draining peatland landscapes for agricul-
ture, forestry, or extraction also poses a significant threat to carbon storage in peatlands. Carbon is lost through these processes via peat oxidation, leaching of carbon into the soil profile, or through combustion from peat fires32. Globally, draining peatlands has resulted in a loss of 80.8 GtC, lowering the water table of peatlands in the process33. Current estimates of greenhouse gas emissions from degraded peatlands are approximately 1.3 to 1.91 GtCO2 per year, corresponding to 2.6-3.8% of total anthropogenic emissions34. In Europe, more than half of peatlands have already been drained for agriculture or forestry35. Drained peatlands also release significant amounts of nitrous oxide, a greenhouse gas with a powerful radiative forcing capacity36. In order to mitigate these emissions, several strategies have been proposed, notably the preservation of peatlands and rewetting. While protecting intact peatlands poses an opportunity to slow down the emissive potential of draining, the process of rewetting peatlands presents the opportunity to reverse it37. Restoring these degraded peatlands has the potential to significantly reduce the carbon dioxide emitted annually from these ecosystems, all via a cost-efficient means38. Excluding temperate organic soils, the process of rewetting has been deemed successful in restoring peatlands as net carbon sinks39. Going forward, when combined with a significant reduction of N2O relative to drained histosols, the rewetting method is an optimistic and viable solution.
DISCUSSION
Should peatland exploitation continue, we are on track to see a positive global soil greenhouse gas balance before the end of the century40. This, in combination with the projected 5°C warming of vulnerable subarctic regions as a result of anthropogenic emissions, may lead to irreversible limitations on carbon sequestration in peatlands41. Moving forward, environmental management and restoration of peatlands will be critical to counteract the effects of climate change. Further commercial exploitation and drainage has the potential to degrade valuable ecosystem services established by peatland ecosystems, including climate regulation and water purification42. That being said, the process of peatland restoration is a viable option to counteract the projected net greenhouse gas emissions. Given that it requires 3.4 times less nitrogen as compared to soil carbon sequestration, in addition to using less land, peatland restoration may prove to be an efficient long-term mitigation strategy43. While other strategies may be effective in reducing further emissions, it is only through integrating peatland
32 Leifeld and Menichetti. “The Underappreciated Potential of Peatlands,” 2.
33 Yehui Zhong et al. “Effects of Water Level Alteration on Carbon Cycling in Peatlands.” Ecosystem Health and Sustainability 6, no. 1 (2020): 1806113.
34 Florian Humpenöder et al. “Peatland Protection and Restoration Are Key for Climate Change Mitigation.” Environmental Research Letters 15, no. 10 (2020): 104093.
35 Zhong et al. “Effects of Water Level Alteration,” 2.
36 Leifeld and Menichetti. “The Underappreciated Potential of Peatlands,” 2.
37 Humpenöder et al. “Peatland Protection and Restoration,” 2.
38 Ibid, 7
39 David Wilson et al. “Greenhouse Gas Emission Factors Associated with Rewetting of Organic Soils.” Mires and Peat 17 (2016).
40 Leifeld and Menichetti. “The Underappreciated Potential of Peatlands,” 5.
41 Camill and Clark. “Climate Change Disequilibrium,” 207.
42 Kai Kimmel and Ülo Mander. “Ecosystem Services of Peatlands: Implications for Restoration.” Progress in Physical Geography 34, no. 4 (2010): 491-514.
43 Leifeld and Menichetti. “The Underappreciated Potential of Peatlands,” 1.
44 Ibid, 5
45 Humpenöder et al. “Peatland Protection and Restoration,” 8. restoration that organic soils may yield a net sink for greenhouse gases44 .
CONCLUSION
Climate change and anthropogenic disturbances to peatlands pose significant threats to their status as net greenhouse gas sinks. Anthropogenic emissions, coupled with the amplification of positive greenhouse gas feedbacks in peatland ecosystems, have the potential to permanently alter the global carbon budget, leaving lasting environmental impacts. Rapid warming has heightened consequences for Boreal and Subarctic landscapes, where increased melting and shifting vegetation may further intensify climatic forcing. Without implementing significant peatland restoration measures, the vulnerable histosols would become a net source of carbon dioxide for the remainder of the century45. The lasting impacts of a reversal to this once important negative climate feedback are inevitable and require further global consideration of peatland restoration as a leading climate change mitigation strategy.
BIBLIOGRAPHY
Camill, P. & Clark, J. (1998). Climate change disequilibrium of boreal permafrost peatlands caused by local processes. American Naturalist . 151(3), 207-222.
Dise, N.B. Peatland Response to Global Change. (2009). Science . 326(5954), 810-811.
Humpenöder, F. et al. (2020). Peatland protection and restoration are key for climate change mitigation. Environmental Research Letters . 15(10)
Jassey, V.E.J. et al. (2013). Above- and belowground linkages in Sphagnum peatland: climate warming affects plant-microbial interactions. Global Change Biology. 19(3), 811-823.
Kimmel, K. & Mander, U. (2010). Ecosystem services of peatlands: Implications for restoration. Progress in Physical Geography-Earth and Environment. 34(4), 491-514.
Leifeld, J. & Menichetti, L. (2018). The underappreciated potential of peatlands in global climate change mitigation strategies. Nature Communications. 9(1701).
Morris, P.J. et al. (2018). Global peatland initiation driven by regionally asynchronous warming. Proceedings of the National Academy of Sciences of the United
States of America. 115(19), 4851-4856
Oke, T.A. & Hager, H.A. (2020). Plant community dynamics and carbon sequestration in Sphagnum-dominated peatlands in the era of global change. Global
Ecology and Biogeography. 29(10), 1610-1620.
Tarnocai, C. (2009). The Impact of Climate Change on Canadian Peatlands. Canadian Water Resources Journal. 34(4), 453-466.
Voigt, C. et al. (2017). Increase nitrous oxide emissions from Arctic peatlands from permafrost thaw. Proceedings of the National Academy of Sciences of the
United States of America. 114(24),6238-6243.
Wilson, D. et al. (2016). Greenhouse gas emission factors associated with regretting of organic soils. Mires and Peat . 17(4), 1-28.
Zeh, L. et al. (2020). Vascular plants affect properties and decomposition of moss-dominated peat, particularly at elevated temperatures. Biogeosciences . 17(9), 4797-4813.
Zhong, Y.H., Jiang, M. & Middleton, B.A. (2020). Effects of water level alteration on carbon cycling in peatlands. Ecosystem Health and Sustainability . 6(1), 1-29.