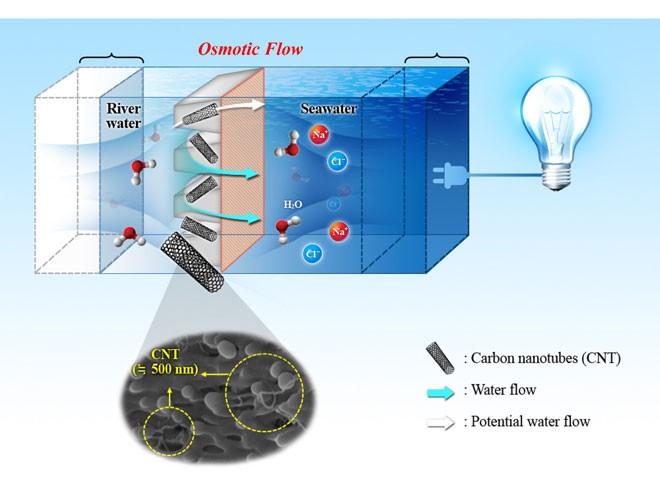
9 minute read
OSMOTIC ENERGY
Osmotic energy is the energy that is obtained from the salinity difference between fresh water and salt water, which are isolated from each other by a semi-permeable membrane. It involves applying a pressure caused by water molecules moving across the membrane or a height of water. The resultant water pressure guarantees a flow that is used to power a turbine.
Sodium and chloride, which are present in the water in the ionic forms Na+ and Cl-, make up most of the salts that are dissolved in saltwater.
Imagine two tanks that are separated by a semi-permeable membrane that blocks the large ions Na+ and Cl- and allows the smaller H2O water molecules to pass through.
One of the tanks is filled with freshwater and the other with a saltwater solution of the same volume.
Due to the two reservoirs' varying salt contents, their identical levels do not represent an equilibrium state.
As a result, water molecules move across the semi-permeable barrier from the freshwater solution to the saltwater solution. The resultant impact raises the level of the saltwater reservoir while simultaneously lowering the salt content of this solution, while the saline concentration of the freshwater solution increases in parallel.
When the osmotic balance, or the equilibrium between the couples of ‘pressures’ (the height of the water) and ‘concentration’ (in salts) of one and the other solutions is attained, the effect ceases to exist.
In other terms, the osmotic pressure is the pressure required to prevent the passage of a solvent (in this example, water) over a semi-permeable membrane from a less concentrated solution (here in salt) to a more concentrated solution.
By applying osmotic pressure to turn a turbine, the osmosis concept is used to generate energy. The water that is supplied to the turbine has the same amount of energy at a pressure of 12 bars as a volume of water falling 120 meters in a hydroelectric dam.
Osmotic energy harvesting by using thin film nanocomposite membrane via pressure retarded Osmosis-
An osmotic plant specifically tries to take advantage of the salinity differential at the natural confluence of fresh and salt water, or at river mouths.
Fresh water from the river is piped into the facility, while salt water from the sea is extracted, filtered, and then pressured in a pressure exchanger.
Almost 80% to 90% of the fresh water pulled into the plant goes through the osmotic plant's membrane, creating an overpressure that boosts the water flow in the saltwater tank.
The remaining two-thirds of this water are returned to the pressure exchanger to pressurize the entering saltwater, while almost a third is sent to the turbine to produce energy. Each nation with river mouths has the potential to use osmotic energy. It gives far better predictability of energy output than other renewable sources and is not reliant on the weather. An osmotic plant would likely run for up to 8,000 hours annually, which is close to three or four times longer than the typical working duration of a wind turbine.
The primary barrier now preventing the development of osmotic energy is semi-permeable membranes' high manufacturing and maintenance costs, although costs should decrease because of technological advancements like electro-osmosis and nanobiotechnology.
By 2030, CNR (Compagnie nationale du Rhone) and its partner Sweetch predict they will be able to generate more than four million MWh of energy at a "competitive price" as a result of this ground-breaking new generating membrane.
Long term production of twice the yearly consumption of the population of the city of Marseille (980,000 inhabitants) might therefore be achieved by only one plant in the Rhône delta.
Nice to hear some optimism after so many courageous but still half-baked energy solutions!
GLACIERS MATTERS.
Throughout Earth's history, glaciers slow-moving rivers of ice have molded mountains and carved out valleys.
Lakes, rivers, and seas get nutrients from glaciers’ melting. The foundation of aquatic and marine food systems, phytoplankton, flourishes due to those nutrients.
Meanwhile, slow glacier melting maintains animal and plant ecosystems in streams. Thus, glaciers often have a secondary effect on animals and fisheries. They also affect sea level. Any locations on Earth where water is frozen, such as snow, sea ice, ice sheets, and glaciers, are collectively referred to as the cryosphere. While making up just 0.5% of all terrestrial ice, glaciers and ice caps contributed more to sea level rise during the last century than ice sheets did.
Glaciers also provide risks to those who live downstream. When glacial lakes grow as a consequence of glacier reduction, hazards become worse in certain regions.
During a portion of the year, people who live in dry regions near mountains sometimes depend on glacier melt for their water supply. Many of the rivers that run across China, India, and other regions of Asia are primarily fueled by snowfall from the Himalayas, but in the late summer, glaciers melting contributes significantly to river flow.
Residents of La Paz, Bolivia, in South America, depend on glacial melting from a neighboring ice cap to provide water during the prolonged dry periods they sometimes suffer.
IceStupas
A Buddhist monument known as a stupa, which means "to stack" or "to pile up" in Sanskrit, often contains relics.
In 2013, the first ice stupa was built in Ladakh, Kashmir. The majority of the water used by the villages of Ladakh, a high mountain-desert area surrounded by the Himalayas, comes from glacier runoff.
The water flow has grown increasingly unpredictable as a result of the glaciers retreating due to climate change. Flash flooding occurs when there is too much precipitation or too little. Hence, the ice stupa is a kind of man-made glacier.
Throughout the winter, runoff or spring water that has been pumped underground and downhill is used to construct the stupas. When it is dark and below zero degrees Fahrenheit, the water is discharged. It flies through a sprinkler and freezes in the air.
Ice stupas may grow as tall as a ten-story structure. They begin to melt in March, and at higher altitudes—some Ladakh communities are more than 15,000 feet above sea level— the process may continue far into July.
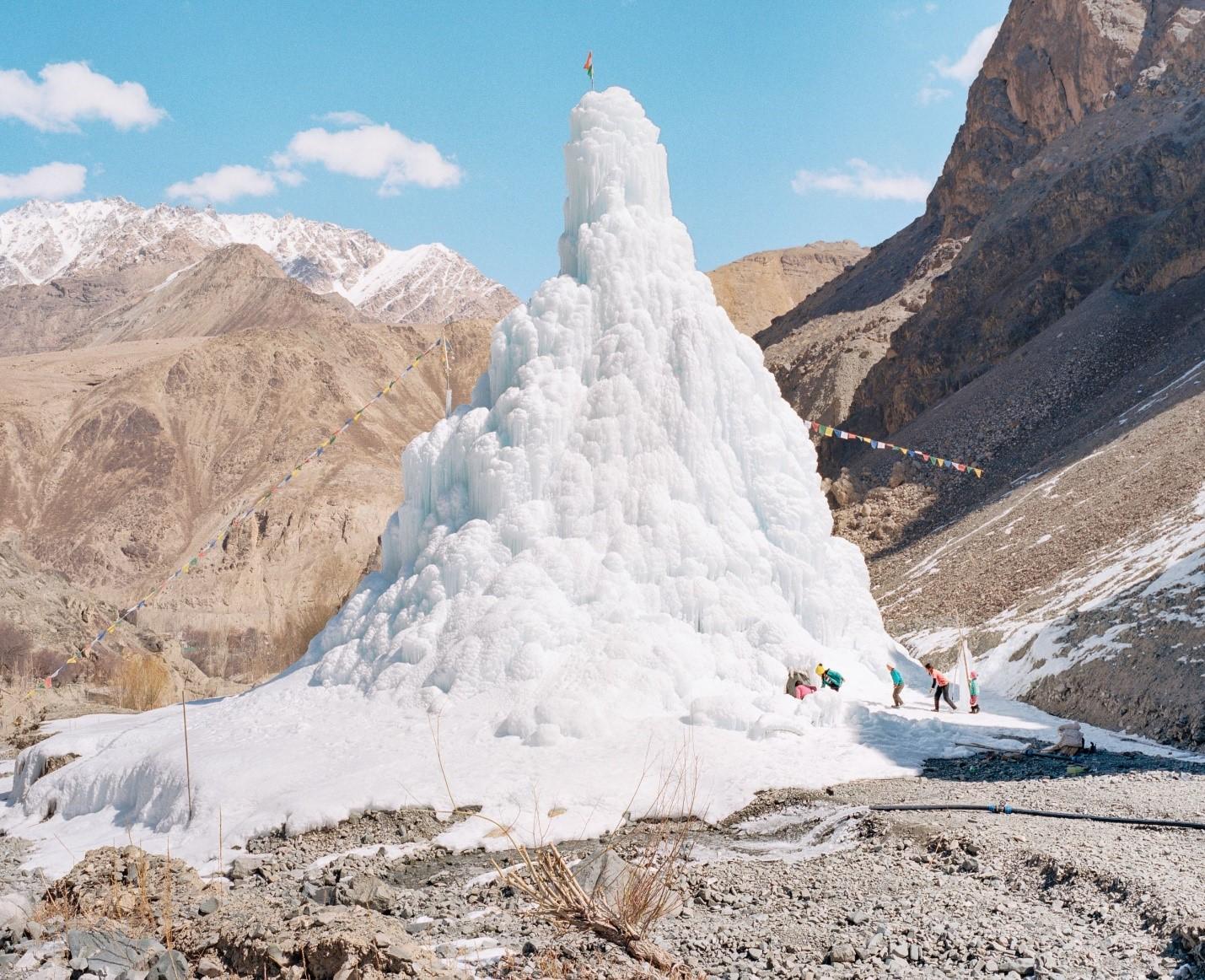
The vital spring planting season, when farmers sow vegetables, barley, and potatoes, is made easier by the meltwater. The number of artificial glaciers is increasing, in contrast to the world's natural glaciers, which are quickly thinning off.
This past winter saw the construction of stupas in at least eleven communities in Ladakh as part of the Ice Stupa Project, which started with a single prototype. Moreover, stupas have been constructed in the Alps, and Canada has shown interest in the project.
Climate change and Glaciers
In addition to moving materials, glaciers also shape and erode the ground underneath them. Over hundreds or even thousands of years, a glacier's weight and slow movement may profoundly alter the terrain.
There are several fascinating glacial formations because of how the ice erodes the ground surface and transports soil debris and shattered rocks far from their original locations. Glacial erosion is widespread across the planet, and glaciated valleys are perhaps the most obvious glacial landform. They have a trough-like appearance, like fjords, and often have high, almost vertical cliffs where whole slopes were scraped by glacier activity.
At Yosemite National Park, glaciers physically scraped away rock, leaving deep valleys with sheer walls. This is one of the most stunning instances of glaciated valleys.
Glaciers are excellent markers of climate change. The International Commission of Snow and Ice was established in 1894 as a direct result of scientific research that began in the eighteenth and nineteenth centuries that focused on the connection between temperature and glacial changes.
With just a few notable exceptions, the great majority of glaciers are receding around the planet, one of the most obvious indicators of continuous climate change.
The 5,000-year-old iceman that was found in 1991 was preserved in a glacier in the European Alps. The find, however, indicated that the glacier had hit its 5,000-year minimum. During the last century, glaciers have receded at previously unheard-of speeds all over the planet. Some have completely vanished. Many more are vanishing so quickly that it might only take decades.
The quantity of carbon dioxide and methane (natural gas) in the atmosphere has grown as a result of human activities since the Industrial Revolution, which started about 1760. In fact, these gases are now more concentrated in the atmosphere than they have ever been in the previous 1,000,000 years. How do researchers know this?
Because glaciers capture samples of Earth's atmosphere like all forms of dense ice. The hanging air bubbles provide scientists with information on global warming.
How much of a warming does our atmosphere experience between ice ages? What impacts do humans have on the climate?
Direct glacier monitoring helps in addressing these questions since glaciers vary in response to climatic changes.
Glacier ice melt is accelerated by warming ocean and air temperatures. Scientists have established that the Industrial Revolution and current energy consumption had the unexpected consequence of causing glacier retreat.
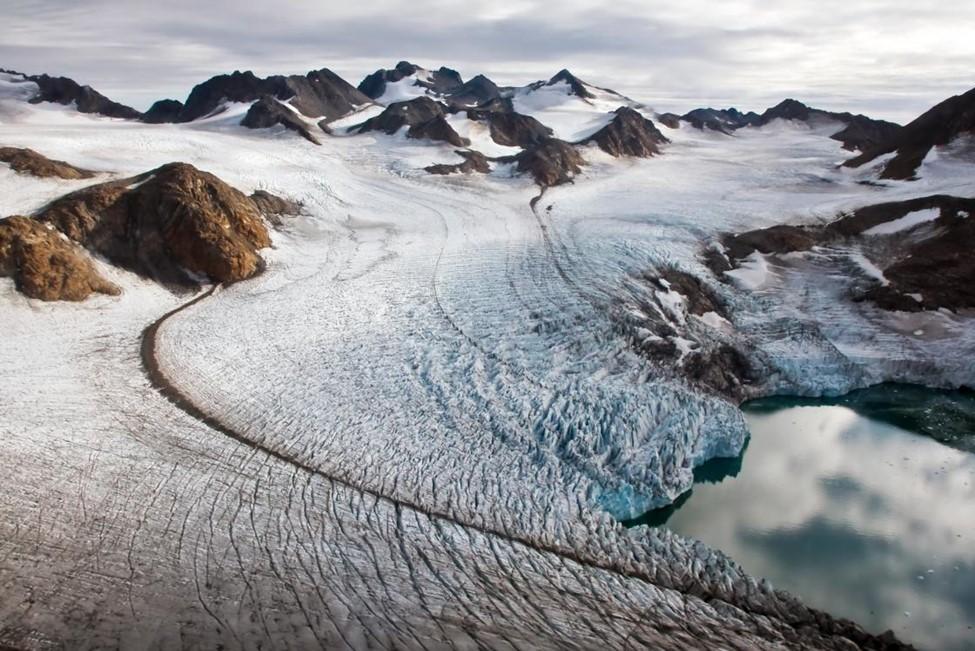
There are other factors at play as well, however. Glacier retreat is further accelerated by increased dust and soot from farming, grazing, burning of fossil fuels, and burning of forests. Glaciers develop a black covering from dust and soot that absorbs more solar energy and accelerates glacial melt. In fact, it's probable that the smoke from coal burning in the late 1800s was what started the most recent glacial retreats in Europe.
In reaction to a changing climate, glaciers expand and contract by altering their extent, thickness, or both. A glacier's volume changes as a direct result of climate. While tied to climate, changes in a glacier's extent might be compounded by modifications to its dynamics. Like a conveyor belt, glaciers move continuously while moving mass downward.
If the glacier is moving forward due to a mix of climate and ice dynamics, the glacier area as a whole will grow as the glacier terminal advances.
Yet, since glaciers move slowly, there may be a delay between the climatic changes and the ensuing glacial advance or retreat.
The intricate systems that regulate how quickly the glacier advances are what cause this temporal lag, which might endure for many decades.
Yet, scientists have proven that the broad glacial retreat we are now seeing is a consequence of the world's temperature rising. In addition, studies of glacier volume changes, which directly reflect climate changes, demonstrate that glaciers everywhere in the globe are losing mass at increasing rates.
DAM HYDROPOWER: STILL SOME POTENTIAL?
Because of the many well-known negative effects on the environment and society, the development of hydropower is debatable.
Analyzing energy potential in terms of a number of limitations that are applied to a theoretical potential is a typical strategy for investigating alternatives for future growth of renewable energy.
The potential energy of water with regard to a base level defines this theoretical potential in the case of hydropower, which is produced by gravity acting from an elevation.
The first limitation is to capture a portion of the potential energy with structures like smooth pipelines – which reduce friction – and dams, which increase head gradients.
A second limitation is financial in nature: not all dams are profitable. Costs may be influenced by land usage, accessibility, or the size of the dam needed to cross extensive valleys. And as river valleys often include people, flooding the lands upstream of a dam is not always an attractive or practical choice.
Impacts on society and the environment are the subject of a third limitation. Hydropower projects may force people to relocate, alter stream flows, nutrient and sediment sources, and have an impact on fish populations and aquatic ecosystems.
Dr. Gernaat's and his team’s research, which was published in Nature, transformed the examination of hydropower potential by creating a high-resolution evaluation approach that takes into account both physical and socio-economic limits.
The technique included utilizing a high-resolution digital terrain model to conduct a systematic scan of (nearly) all the rivers around the globe.
Every 25 km along these rivers, potential locations for hydropower stations were investigated by analyzing climatic and topographical data and taking into account hydropower capacity and the socioeconomic costs as a function of station parameters, such dam height.
The cost model considered expenditures for building the dam, which is based on local topography, as well as technical installations (such as turbines and power lines) and socioeconomic costs associated with land usage in the upstream catchment that would be submerged by the dam reservoir.
The trade-off between energy output and cost was assessed using a cost optimization model, giving priority to the dams with the lowest cost per kWh. Lastly, ecological limits like protected areas and ecological flow restrictions were considered.
With better geographic coverage (60 S to 90 N instead of 56 S to 60 N, effectively including, for example, Scandinavia), higher resolution topography over a denser search grid (4.5 km instead of 25 km), and more consideration of social and environmental constraints, the recent research improves previous work.
For instance, this research expressly prohibits the construction of hydropower plants in historical sites, biodiversity hotspots, forests, peatlands, earthquake-prone regions, heavily inhabited areas, and sites where dams or reservoirs already exist.
According to their findings, the majority of the theoretically available hydropower (58 PWh/ year) is either already installed, impractical, or unprofitable.
Indeed, when both economic and environmental limits are taken into consideration, Europe and the Americas have already overused their hydropower potential.
The additional advantage of the analysis is that it enables a thorough assessment of the social and environmental effects of possible hydropower sites, which is important given the many debates surrounding hydropower.
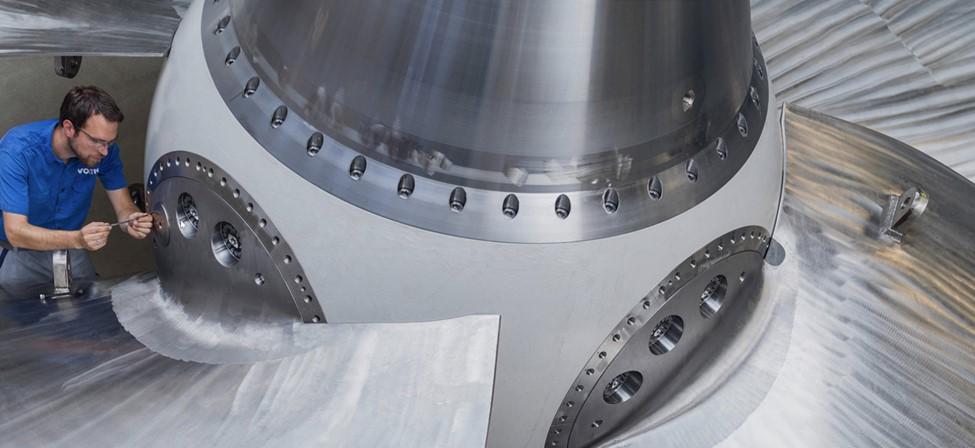
The only remaining giant project left is referred to as ‘Grand Inga’, located in the Democratic Republic of Congo.
Upon completion it will be the biggest hydroelectric plant in the world, in theory producing more than twice as much energy as China's Three Gorges Dam: a total of 40,000 megawatts (MW) of energy may be generated by six separate hydroelectric power plants, sufficient to power five southern African nations (70 million people), as well as DRC’s own 101 million citizens.
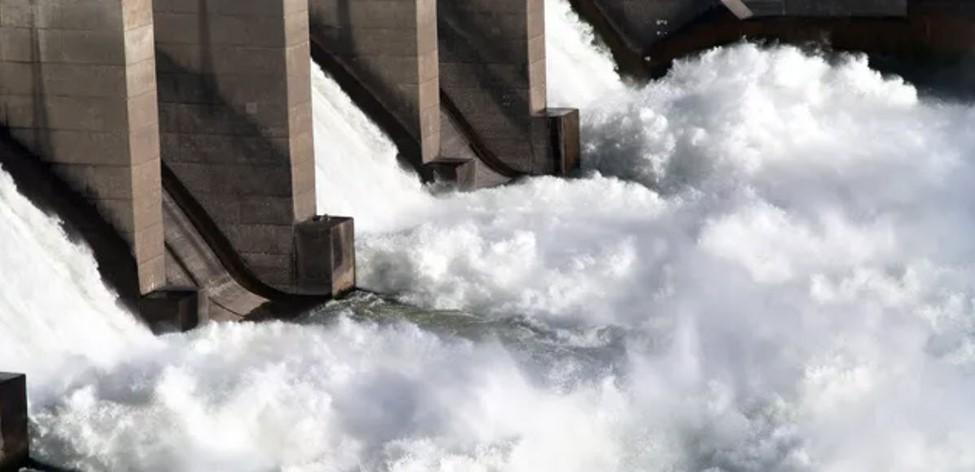
Please don't ask me why it is still in the air and has been suspended for dozens of years. I won't be very courteous...