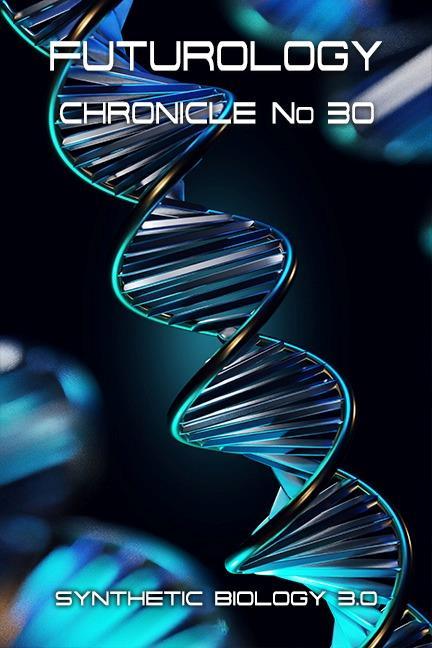
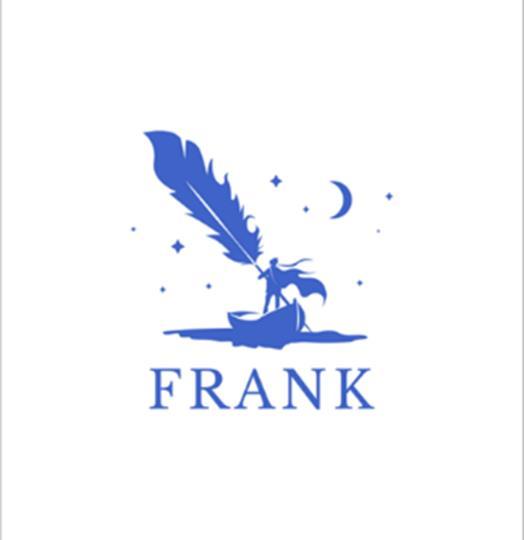
It is important to understand how it evolved to get at what we currently call "Synthetic Biology 3.0."
This term heralds a new era of complexity, technological innovation, and application diversity, encapsulating a considerable transition from the early phases of this discipline.
The field of synthetic biology has experienced a remarkable metamorphosis, characterized by several phases of development, each with notable breakthroughs and growing opportunities.
Synthetic Biology 1.0 (1990-2010)-:
The field's foundation was established during this first stage. It entailed laying the groundwork for creating artificial biological systems through design and construction.
To provide the groundwork for more sophisticated applications, researchers concentrated on creating standardised genetic components, circuits, and fundamental genetic engineering methods.
Synthetic Biology 2.0 (2000-2020):
This stage represented a step up, building on these underpinnings. It brought with it more sophisticated genetic engineering, enhanced technologies, and expanded applications.
High-throughput DNA assembly, genome editing, gene synthesis, and computer modellg were among the significant advancements.
This period witnessed the investigation of complex genetic circuits and metabolic engineering, expanding the applications of synthetic biology in the fields of medicine, energy, and agriculture.
Synthetic Biology 3.0:
Since 2021 and mRNA Covid vaccine discovery, the field is set to make tremendous scientific strides as we enter Synthetic Biology 3.0.
Deeper AI and machine learning integration, which further improves genetic manipulation precision and capacities, is probably what will characterize this new phase.
The list of possible uses is growing and now includes global food security solutions, more individualized medical care, and environmental sustainability.
Synthetic Biology 3.0 holds the potential to bring about revolutionary shifts in our understanding and resolution of intricate biological problems, rather than merely minor enhancements.
Combining concepts from biology, engineering, genetics, chemistry, and computer science, Synthetic Biology, known also as "Biogen Tech" is a cutting-edge, multidisciplinary topic.
This subject is developing quickly, providing fresh approaches and cutting-edge technologies that could completely transform a wide range of facets of our lives.
Biogen Tech is at the forefront of scientific advancement in fields including healthcare and environmental protection. Let's examine the eight essential categories that make up this fascinating field.
Genetic Engineering a technique by which scientists alter an organism's DNA to change its properties, is the cornerstone of Biogen Tech. This includes methods such as CRISPR-Cas9, which enable accurate gene editing.
Numerous medical uses of genetic engineering exist, including the creation of gene therapies to cure hereditary illnesses.
Within the field of Biological Design and Synthetic Genomics , researchers devise new biological components and systems or rework preexisting ones.
This covers the creation of novel creatures with desired features as well as the synthesis of synthetic genes. The development of novel materials, biofuels, and medications depends heavily on this strategy.
Metabolic Engineering is an intriguing field in which organisms' metabolic pathways are altered to create novel compounds or improve ones that already exist.
This has a big impact on how effectively biofuels, medications, and other useful molecules can be produced.
Protocell Biology is a field focuses on the engineering of artificial cells or structures that resemble cells. This innovative work contributes to the development of new biological machinery and helps understand the origins of life.
This field opens doors in biotechnology and medicine by blending the boundaries between the artificial and the living.
Bioinformatics and Computational Biology model and comprehend intricate biological processes, highly potent computer techniques are utilised in this fifth pillar.
The development of artificial biological systems and the analysis of enormous volumes of biological data, which result in novel findings and uses, depend heavily on this computational method.
The category Biomaterials and Tissue Engineering is a transformative one that concentrates on creating new materials using biological sources.
This involves creating new organs and tissues, which could transform wound healing and transplant therapy. It's about developing biocompatible materials that are also functional.
Environmental Applications of Biogen Tech are vital in the context of environmental sustainability. This covers the application of genetically modified organisms for bioremediation, the act of removing contaminants from the environment using live organisms.
This area of study combines biology and environmental science to address some of the most urgent ecological problems facing our world.
Finally, Agricultural Applications of Biogen Tech include genetically modifying crops to increase productivity, resistance to pests, and environmental adaptability.
This area also encompasses the application of synthetic biology to enhance agricultural methods, promoting sustainable agriculture and global food security.
To summarise, Biogen Tech is a multidisciplinary field that has great promise for tackling some of the most pressing issues of humanity.
Biogen Tech has a wide range of significant uses, from improving healthcare to promoting environmental sustainability.
Biogen Tech is well-positioned to significantly advance science and technology and improve our planet as we continue to investigate and expand these eight categories.
The history of synthetic biology, also referred to as "Biogen Tech," is a tapestry woven from the contributions of various scientific fields and the work of numerous scientists over centuries. Here's a brief overview:
Term Coinage: The term "synthetic biology" was first coined by the French biologist Stéphane Leduc in 1912 in his publication "La Biologie Synthétique."
However, the concept as we understand it today began to take shape much later, with the advancements in molecular biology and biotechnology.
Early Foundations: The roots of synthetic biology can be traced back to the fundamental work in genetics and molecular biology. Scientists like Gregor Mendel, who laid the groundwork for the understanding of genetic inheritance in the 19th century, and James Watson and Francis Crick, who discovered the double-helix structure of DNA in 1953, set the stage for future developments.
Modern Pioneers: In the late 20th and early 21st centuries, scientists like Herbert Boyer and Stanley Cohen, who performed the first successful recombinant DNA experiments in the 1970s, are considered pioneers. These experiments marked the beginning of genetic engineering, a cornerstone of synthetic biology.
Recent Developments: Jennifer Doudna, along with Emmanuelle Charpentier, was awarded the Nobel Prize in Chemistry in 2020. They received this prestigious honor for their development of the CRISPR-Cas9 gene-editing technology, a groundbreaking achievement in the field of molecular biology.
This technology allows scientists to edit DNA with high precision and has vast implications in genetic research, medicine, agriculture, and beyond. Their work represents a significant milestone in the history of synthetic biology and biotechnology.
More recently, the field has been shaped by the work of scientists like Craig Venter, who created the first synthetic bacterial genome.
Interdisciplinary Contributions: It's important to note that synthetic biology is inherently interdisciplinary. Contributions from computer science, engineering, and other fields have been crucial in modeling complex biological systems and in the design of new biological parts and devices.
Synthetic biology, therefore, is not the brainchild of a single individual or discovery; it's a field that has evolved through the collective efforts of scientists and thinkers across various disciplines over many years.
The groundbreaking genetic engineering technology CRISPR-Cas9 is revolutionising science and medicine. Imagine being able to precisely cut DNA with a pair of molecular scissors.
That's the CRISPR-Cas9 system, which is being used for genome editing after it was first identified as a component of bacterial immunological defence.
The acronym CRISPR refers to "Clustered Regularly Interspaced Short Palindromic Repeats." These are DNA sequences in bacteria that store portions of viruses' DNA in order to remember them.
When these viruses re-attack, the bacteria use the RNA molecules that were produced from these sequences to direct the Cas9 protein to the appropriate viral DNA, where it is cut, rendering the virus inoperable.
This approach has been modified by scientists to modify the genes of different creatures in the lab. They are able to precisely guide the Cas9 enzyme to a specific location in the DNA by creating a synthetic RNA that matches a target sequence in a gene. Cas9 then proceeds to cleave the DNA.
Then, the cell's built-in repair mechanisms take over, but they can make mistakes and alter the order. This is used by scientists to add new genetic material or delete genes.
The efficiency and ease of use of CRISPR surpass those of earlier gene-editing techniques. Because it doesn't require the elaborate and expensive equipment of previous procedures, more labs can use it. It has a wide range of uses, including the correction of genetic defects, enhanced crop resilience, the development of animals immune to disease, and even possible HIV and cancer treatments.
Notwithstanding its potential, CRISPR raises important ethical questions. Because of its capacity to modify DNA, there are concerns regarding its usage in human embryos, possible unforeseen repercussions in gene editing, and wider ramifications for ecosystems and biodiversity.
Though there is a lot of discussion and research being done in the sector, the future of CRISPR is quite bright. Discussions on CRISPR involve not only what we can do, but also what we ought to do. The scientific community continues to give this technology serious ethical thought as it develops and as a beacon of hope.
The study of genomes, or genomics, has completely changed how we think about life. It entails the in-depth investigation of genomes, or an organism's whole genetic makeup.
Understanding genome structure, function, evolution, mapping, and editing is the main goal of this field.
Understanding the functions of the genes, their interactions with one another, and how they affect an organism's traits and behaviour is just as important as knowing which genes are present.
Genome science relies heavily on the sequencing and study of genomes. Thanks to the development of high-throughput sequencing methods, scientists can now swiftly and cheaply sequence whole genomes.
Important discoveries have resulted from this in a variety of fields, including environmental science, agriculture, and human health.
Through genome analysis, scientists may pinpoint the genes causing illnesses, comprehend the evolutionary connections between different species, and create novel approaches to producing plants and animals with specific characteristics.
The idea of genome editing, a method that enables accurate, targeted modifications to a particular sequence in a genome, is inextricably related to the field of genomic science. Scientists can now modify genomes with previously unheard-of precision and efficiency, thanks to technologies like CRISPR-Cas9.
This ground-breaking method cuts DNA at a precise region by acting as molecular scissors. Researchers are able to add, remove, or replace genetic material because once the DNA is cut, the cell's natural healing mechanisms take over.
Genome editing has far-reaching consequences. It presents a chance for novel treatments for hereditary illnesses in medicine.
For example, researchers are looking at using genome editing to fix abnormalities that lead to illnesses like sickle cell anaemia and cystic fibrosis.
Genome editing has the potential to produce crops that are more nutrient-dense, disease- and pest-resistant, or climate change-adaptive.
However, there are significant societal and ethical issues that are brought up by genome editing and genomic science. Concerns over unexpected outcomes arise when considering the ability to modify genomes, particularly in relation to altering human embryos or the potential for "designer babies.
Concerns about equity and access also exist: who gains from new technology and who controls their application?
Despite these obstacles, the area of genomics is still expanding and changing. The study of functional genomics, which aims to comprehend the connection between the organism's phenotype—its observable traits and behaviors—and its DNA, is one of the most fascinating fields.
Scientists can better understand how genetic variants result in changes in health, behaviour, and susceptibility to diseases by connecting genes to functions.
An additional emerging field is environmental genomics. It entails examining the genomes of living things in their native habitats to shed light on biodiversity, ecosystem function, and the effects of changing environmental conditions on living things.
To sum up, genome editing and genome science are the frontiers of biological study.
They have the potential to significantly improve healthcare, agriculture, and other fields in addition to bringing about substantial improvements in our knowledge of life.
The ethical implications of this potent technology must be carefully considered as we go with our exploration of the intricacies of genomes.
In the end, these domains present an unrivalled perspective on the structure of existence, presenting countless opportunities for exploration and creativity.
At first, the black genome—which makes up 98% of our DNA—was regarded as "junk" DNA. Its roughly 20,000 protein-coding genes, however, have been discovered recently, prompting a reinterpretation of the gene notion that now more effectively incorporates surrounding expression regulatory regions in addition to coding sequences.
Non-translated genes, such as microRNAs and long non-coding RNAs, have a key function in the regulation of gene expression. Since these non-coding sequences affect how the central nervous system develops and functions, they play a significant role in regulating gene expression.
To sum up, every sequence in the human genome explains how its genes work and is therefore deemed "useful." Utility is a difficult issue in genetics since it is linked to a notion of finalism that is at odds with the processes of evolution.
Transposons and retrotransposons are two examples of the significant class of mobile elements that make up our genetic makeup.
Mobile elements comprise about 45% of our genetic history, while sequences generated from retroviruses make roughly 8%.
In response to environmental changes, mobile elements alter gene expression and aid in the development of species and specific adaptations. One of the genomes with the highest proportion of mobile elements (80%) is corns.
Since the publication of the first draft (almost complete sequencing) map of the human genome in 2001, the percentage of coding and non-coding DNA has been determined with increasing accuracy.
The existence of mobile components enhances our understanding of the human genome's structure and functions, helping to explain the emergence of specific disorders.
Through structural modifications to a clotting factor gene, mobile elements can play a role in the development of hereditary illnesses like haemophilia.
Predicting evolutionary situations is not greatly aided by knowledge about species evolution. DNA is composed of "only" 20,000 genes, and the quantity of translated genes is not the main factor in the complexity of all living things, including humans. Genetic diversity, epigenetic changes, and the dynamic nature of gene expression are only a few examples of the various levels of integration.
In contemporary biotechnology, metabolic engineering—a branch of synthetic biology represents a frontier. In this cutting-edge discipline, metabolic pathways within cells are manipulated to maximise the synthesis of specific compounds or to confer new, desirable qualities upon cells.
The main goal of metabolic engineering is to improve and reroute the intricate web of chemical reactions that exist inside living things.
The key to metabolic engineering is comprehending and altering the metabolic pathways, which are the biochemical processes that transform substrates into more sophisticated products inside of a cell. T
hese channels serve as the biochemical superhighways of life, allowing materials and energy to move freely and support cellular processes. Scientists can manipulate these pathways to encourage the production of beneficial molecules, the breakdown of contaminants, or even the prevention of disease.
The synthesis of medications is one of the main uses of metabolic engineering. Microorganisms can be genetically engineered to create vaccines, antibiotics, and other medicinal substances.
This not only reduces costs and improves manufacturing efficiency, but it also makes it possible to synthesise substances that would be difficult or impossible to manufacture using traditional chemical synthesis methods.
Metabolic engineering is utilised in agriculture to produce crops with enhanced resistance to pests and diseases or better nutritional characteristics. To counteract malnutrition, for example, 'biofortified' crops are produced by altering the metabolic pathways in plants to raise the quantities of vital nutrients.
Metabolic engineering has applications in environmental biotechnology as well. A sustainable method of bioremediation is provided by the use of engineered microbes to break down environmental contaminants. T
hese microbes can help clean up oil spills, industrial waste, and other toxins by changing metabolic pathways that break down toxic molecules into less damaging substances.
Genetic engineering and molecular biology technologies are widely used in the field of metabolic engineering. The accuracy and efficiency of modifying metabolic pathways have been significantly improved by methods such as CRISPR-Cas9 gene editing. Another essential tool for scientists is computational modelling, which enables them to simulate and forecast the effects of metabolic changes before they are tested in the lab.
In conclusion, the fast-developing subject of metabolic engineering holds the potential to completely transform the way we create food, medicine, and clean our surroundings. It offers creative answers to some of the most important problems facing the planet by bringing biology and engineering together. The applications of metabolic engineering are growing, leading to new discoveries in synthetic biology and other fields, as our knowledge and technology advances.
The development of synthetic gene circuits is an example of human inventiveness in the rapidly changing field of synthetic biology.
These circuits are more than just a group of genes; they are complicated networks with specialised roles that mimic and occasionally even outsmart the intricacy of naturally occurring genetic systems.
The combination of biology, engineering, and computer science in synthetic gene circuits opens up new possibilities in biotechnology and medicine.
Fundamentally, synthetic gene circuits consist of DNA sequences designed to operate similarly to electrical circuits. These circuits use RNA molecules, proteins, and enzymes to carry out operations in place of electrons.
The goal is to integrate these circuits into live cells, turning them into programmable machines that can carry out everything from basic calculations to intricate decision-making.
Therapeutics for medicine is one of the most amazing uses of synthetic gene circuits. Gene circuits that can recognise and react to illness situations within the human body are being designed by scientists.
A circuit might be designed, for instance, to recognise cancer cells and initiate a specific therapeutic reaction, providing a new degree of treatment precision.
This strategy has enormous promise for personalised medicine, in which a patient's therapy is based on the specific genetics of their illness.
Synthetic gene circuits are being employed in environmental applications to develop biosensors, or creatures that are able to identify and report on environmental toxins. These living sensors provide a dynamic means of keeping an eye on ecosystems and provide up-to-date information on any hazardous materials present.
Synthetic gene circuits are also advantageous in the field of agriculture. Researchers are creating plants with genetic circuits specifically designed to improve the plants' growth, nutritional content, or disease and pest resistance. When it comes to solving issues with food security, particularly in areas with tough environmental circumstances, this technique may be quite important.
Synthetic gene circuit creation is a challenging process that requires not just circuit design but also integration and control within a living cell. A thorough comprehension of cellular dynamics and genetic regulatory processes is necessary. The creation of synthetic gene circuits has been substantially expedited by developments in gene synthesis and editing technologies, such as CRISPR-Cas9. This has made it possible to construct and test complicated genetic networks more effectively than in the past.
Synthetic gene circuits, in summary, are a revolutionary development in the realm of synthetic biology. They provide unheard-of control over biological systems, opening the door to creative applications that were previously only possible in science fiction.
We are not only improving our understanding of life, but also reinventing its potential as we continue to investigate the possibility of these artificial genetic networks.
A ground-breaking development in genetic medicine, RNA editing is quickly becoming recognised as a key tool with enormous promise for creating safer and more adaptable genetic condition treatments.
This method differs from DNA editing in that it allows for transient and reversible changes, which offers a promising way to correct mutations that cause disease without modifying the genome permanently.
At the centre of this cutting-edge field is Wave, a pioneering business among a growing consortium of firms harnessing the potential of an endogenous enzyme called adenosine deaminase acting on RNA (ADAR).
This approach is critical because it utilises the body's natural RNA editing mechanism, which allows genetic abnormalities to be corrected at the RNA level before proteins are synthesised.
The significance of this innovative technology is emphasised by two major deals signed in the preceding year.
A notable collaboration between the pharmaceutical giant Eli Lilly and Leiden, Netherlands-based ProQR Therapeutics is a prime example of the growing interest in RNA editing.
Expanding on a previous agreement from 2021, this partnership highlights ProQR's capabilities in developing treatments for genetic ocular illnesses in addition to a broader focus that includes genetic neurological and metabolic problems.
Their cooperative effort with Eli Lilly intends to create up to five therapeutic targets, utilising ProQR’s specialized Axiomer RNA base editing technology. This technology makes it possible to precisely alter single nucleotides in RNA, which can reverse mutations or affect the expression of proteins.
GSK and Wave of London have formed a noteworthy cooperation that focuses on RNA editing, silencing, and splicing.
The only program in this partnership that has been made public is WVE-006, which treats alpha-1 antitrypsin deficiency (AATD), a disease that affects the liver and lungs. GSK's expertise in genetics and genomics, along with Wave's cutting-edge PRISM oligonucleotide technology, offer a viable approach to creating oligonucleotide medicines that target new genetic markers.
Furthermore, Roche, a Basel, Switzerland-based company, entered the ADAR-based RNA editing space in 2021 when it formed a full alliance with Shape Therapeutics. This partnership demonstrates how big pharma is becoming more interested in RNA editing technologies.
Besides these large-scale collaborations, numerous alternative RNA editing approaches are in development. For example, Ascidian Therapeutics is developing a novel exon-editing platform, and Rznomics from Yongin
, South Korea, has pushed its RNA-guided endonuclease (RGEN) technology into clinical trials. These varied methods highlight how RNA editing research is a dynamic and developing field, with the potential to transform treatment for a wide range of illnesses.
A new age in genetic medicine is dawning with the rapid advancement of RNA editing technology.
Researchers might potentially treat genetic problems more precisely and reversibly by focusing on RNA, which gives previously incurable conditions new hope.
By concentrating on RNA, diseases can be treated more precisely by addressing the underlying genetic mistakes instead of taking the chance of permanently altering DNA.
Furthermore, RNA editing is a very promising treatment for disorders with intricate genetic roots.
It provides a sophisticated method of regulating protein activity and gene expression, which may be essential for treating complex illnesses like cancer, neurological conditions, and cardiac conditions together with the capacity to adjust protein and gene expression.
In the fast developing environment of biological research, bioinformatics has emerged as a vital field. It functions as the computational foundation that supports the analysis and interpretation of complex biological data, standing at the intersection of biology, computer science, and mathematics.
Creating and using computer tools to make biological discoveries is the fundamental activity of bioinformatics. Finding valuable insights from massive amounts of data is more important than merely managing it. This field is now essential to comprehending the complex webs of genetic sequences, molecular structures, and cellular functions.
The abundance of data produced by contemporary technologies like highthroughput sequencing and proteomics is responsible for the emergence of bioinformatics. Conventional laboratory techniques are inadequate when genome sequencing, protein cataloguing, and cellular process analysis reach previously unheard-of levels of inquiry. The computing capacity to manage, examine, and comprehend these huge datasets is here supplied by bioinformatics.
Genomic science is one of the main fields in which bioinformatics is used. Bioinformaticians can investigate gene functions, track the evolutionary history of a gene, and find genetic variants associated with disease by analysing DNA sequences.
Bioinformatics is essential to proteomics, which is the study of proteomes, or the whole collection of proteins expressed by a genome, in addition to genomics. Comprehending the structures, activities, and interactions of proteins is beneficial for the development of new drugs and the identification of diseases.
Additionally, bioinformatics is not limited to fundamental research. It is applicable to agricultural biotechnology, environmental conservation, and pharmacogenomics, among other domains. For instance, bioinformatics helps in pharmacogenomics by assisting in the identification of therapeutic targets and the prediction of drug responses, which expedites the drug development process.
By examining plant genomes and finding genes linked to desired features like insect resistance or drought tolerance, bioinformatics helps the agricultural industry boost crop yields. In the same way, genome data helps in monitoring biodiversity and comprehending ecosystem processes in environmental conservation.
To sum up, bioinformatics is a topic that not only helps the biological sciences but also transforms them. It is spurring breakthroughs in a range of fields, from environmental sustainability to healthcare, by making it possible to analyse complicated biological data. The significance of bioinformatics in interpreting the increasing volume and intricacy of biological datasets cannot be emphasised; it is the computational foundation of contemporary biology.
Biosensors have become an indispensable instrument in the field of scientific innovation, transforming the methods by which we identify and evaluate molecular and environmental changes.
These advanced instruments combine biological elements with a physicochemical detector to offer quick, precise, and economical analysis.
Applications for biosensors are numerous and include food safety, medical diagnostics, environmental monitoring, and more. Their basic idea is based on a biological recognition element that can selectively interact with a target molecule, such as enzymes, antibodies, or nucleic acids.
The transducer then transforms this interaction into a measured signal, providing quantitative or semi-quantitative data.
Biosensors are essential for the detection of diseases, poisons, and pollutants in environmental applications. The need for sensitive, focused, and quick detection techniques has grown as environmental concerns and regulations have become more stringent.
By enabling on-site measurement of soil and water quality and identifying pollutants including pesticides, heavy metals, and organic compounds, biosensors provide a solution. Since real-time monitoring enables prompt reaction to pollution problems, it is essential for sustainability and environmental protection.
Biosensor technology has brought about a tremendous transformation in the medical diagnostics industry. One well-known use for biosensors is the easy and continuous surveillance of blood sugar levels in diabetic patients.
In addition to glucose, biosensors are being developed for a broad range of medical applications, such as the detection of cancer biomarkers, the diagnosis of infectious diseases, and the monitoring of drug levels in the body.
These innovations have the potential to revolutionize medicine by enabling customized care plans based on the unique biomarkers found in each patient's biological samples.
Additionally, biosensor technology helps the food business maintain the quality and safety of its products. Biosensors are essential to the food supply chain from production to consumption because they can identify allergies, foodborne pathogens, and spoiling signs.
The development of biosensors is driven by developments in materials science, nanotechnology, and biotechnology. For example, the sensitivity and specificity of biosensors have been improved by nanomaterials, enabling the detection of incredibly tiny quantities of target molecules.
Furthermore, the amalgamation of biosensors with digital technology and the Internet of Things (IoT) facilitates the development of intelligent sensor networks, which have the ability to transmit data in real-time to smartphone users or central monitoring systems.
Biosensor technology has a very bright future ahead of it. These systems are getting more advanced with further research and development, providing increased stability, sensitivity, and the capacity to identify many targets at once.
Furthermore improving data analysis and enabling quicker and more accurate result interpretation is the incorporation of machine learning and artificial intelligence technologies.
In addition to improving current uses, this advancement in biosensor technology is creating new research opportunities in fields including smart agriculture, environmental preservation, and personalised healthcare.
It is evident that biosensors are more than just detecting instruments as we continue to push the envelope of what is conceivable with them. They serve as doorsways to a greater comprehension of the intricate chemical and biological systems that control our planet.
It is impossible to exaggerate their contribution to increasing scientific understanding and raising living standards.
Biosensors are a marvel of human creativity, the ideal fusion of technology and nature, and they have the potential to significantly influence the course of the future.
The rapid evolution of CRISPR technology from a laboratory curiosity to a potential revolution in gene therapy within a mere decade is nothing short of remarkable. Dr. Alexis Komor of the University of California, San Diego, who has been at the vanguard of developing these novel CRISPR tools, remarks on the swift progress: “The journey to the clinic has been unexpectedly quick.”
Originally, CRISPR technology functioned like molecular scissors, making precise cuts in DNA to disrupt harmful mutations. However, the advent of 'base editing,' sometimes referred to as "CRISPR 2.0," has refined the process. Instead of cutting the DNA, this method allows for the conversion of one DNA base into another, effectively acting like a pencil and eraser as described by geneticist Kiran Musunuru.
This new technique offers a potentially safer alternative to the initial CRISPR approach. Without severing the DNA strand, the risks of inadvertently removing essential genes or causing improper reattachments are minimized. Verve Therapeutics, among others, employs this base editing technique, advancing therapies for conditions such as high cholesterol and sickle-cell disease.
"CRISPR 3.0," or prime editing, is the next leap forward. This method not only alters existing DNA but also facilitates the insertion of entirely new genetic sequences. Still in its nascent stages and undergoing trials in lab animals, its potential is immense, promising to address a broader spectrum of genetic disorders beyond the reach of base editing.
Dr. Musunuru emphasizes the versatility of prime editing, explaining that it could be the only option for correcting mutations outside the scope of base editing. This groundbreaking technique could offer a universal remedy for numerous genetic conditions, irrespective of the mutations involved, by inserting functioning genes into the genome.
The ongoing development of CRISPR technologies could vastly expand the scope of gene-editing treatments, making them accessible for a wider range of conditions, even those not directly caused by genetic mutations. For instance, Verve's innovative cholesterol-lowering therapy utilizes base editing to manipulate the PCSK9 protein gene, potentially benefiting a vast adult population.
However, the implementation of these CRISPR-based therapies is not without ethical considerations, particularly when it comes to editing the genomes of healthy individuals. The benefits must be weighed against the risks, and new treatments must prove to be as safe if not safer than existing alternatives. As Tania Bubela
of Simon Fraser University points out, the irreversibility of gene therapies contrasts sharply with the modifiable nature of traditional medications.
As the CRISPR field progresses, it faces the dual challenges of ensuring safety and affordability. While gene therapies may carry inherent risks and a substantial price tag, the prospect of a one-time, enduring treatment holds considerable allure.
The earliest CRISPR trials have concentrated on patients with rare, untreatable conditions, reflecting a prioritization of urgent medical needs. This approach embodies a profound ethical commitment to addressing the most pressing health challenges before considering broader applications. As Dr. Komor wisely notes, expanding CRISPR's horizons is thrilling, yet we must first fulfill our moral duty to assist those with the direst needs.
Looking to the future, the promise of CRISPR extends far beyond current applications. The technology's potential to combat diseases like high blood pressure and diabetes, and perhaps even to prevent conditions such as Alzheimer's, hints at a new era in medical intervention. Nonetheless, caution is paramount, as genome editing in healthy individuals remains a contentious ethical frontier.
The path forward for CRISPR-based interventions is paved with both unprecedented opportunity and formidable challenges. As researchers like Dr. Komor and Dr. Musunuru navigate this landscape, their work will undoubtedly inform the contours of future medical ethics and treatment paradigms. With careful consideration and continued innovation, CRISPR could well redefine the boundaries of therapeutic possibility.
Ten years after its discovery, CRISPR technology has advanced medical therapies significantly. Most notably, exa-cel, a ground-breaking CRISPR-based therapy for sickle cell disease, was approved by the FDA.
The drug was created by Vertex Pharmaceuticals and CRISPR Therapeutics. To turn off the defective gene that gives blood cells their sickle shape, Exa-cel works by modifying a gene involved in the structure and function of red blood cells. This kind can obstruct blood vessels, leaving patients in excruciating agony and exhausted.
Exa-cel and another recently licensed gene treatment effectively prevented sickle cell disease symptoms for a full year in clinical trials. Long-term adverse consequences are still being assessed, though.
The price of exa-cel is a significant issue as many people who require it may not be able to afford it. Since 2017, the FDA has authorised about twenty cell or gene therapies; nonetheless, exa-cel is noteworthy due to its potential impact, particularly for the broader population afflicted by sickle cell disease, which is not regarded as rare and mostly affects the Black community in the United States.
With Exa-cel, a patient's own blood cell progenitors are taken, modified using the CRISPR/Cas9 gene-editing technology, and then reintroduced into the patient by a stem cell transplant following a high-dose chemotherapy treatment to remove any remaining defective cells.Red blood cell sickling is avoided by this process, which tries to replenish the blood with healthy hemoglobin-producing cells.
The majority of the patients tested in the ongoing Phase 2/3 CLIMB-121 clinical trial showed that a single infusion of Casgevy was sufficient to prevent any painful vasoocclusive crises (VOCs) for at least a year.
Exa-cel seems promising, however given that most gene treatments cost more than $1 million, concerns about accessibility and coverage are raised by the drug's exorbitant cost. Casgevy, a gene treatment for sickle cell disease, will cost $2.2 million from Vertex and CRISPR Therapeutics, while Lyfgenia (lovo-cel), another gene therapy from Bluebird Bio, will cost $3.1 million. In terms of patient accessibility and affordability, the high costs of these medicines are still up for discussion.
Leading gene editing business CRISPR Therapeutics is committed to creating genebased treatments for severe illnesses by utilising the exclusive CRISPR/Cas9 technology.
This ground-breaking technique has created a portfolio of treatment programmes spanning multiple disease categories, including regenerative medicine, hemoglobinopathies, cancer, and rare diseases, and it enables precise, directed modifications to genomic DNA.
The development of CRISPR/Cas9 technology and its uses in gene editing mark a major breakthrough in medical science and provide hope for the treatment of diseases that were thought to be incurable. Nonetheless, there are still important factors in the area to take into account, including the sophisticated therapies' accessibility and ethical consequences.
A significant advancement has been made in the fight against cancer thanks to a ground-breaking study led by investigators from the Wellcome Sanger Institute, Open Targets, and their partners.
By using a novel strategy, the team has identified 370 potential therapeutic targets for 27 different types of cancer, including common ones like ovarian, lung, and breast malignancies.
This achievement is particularly significant since it stems from a complete examination of both functional and genomic data, delivering an unbiased, panoramic perspective of the mechanisms fuelling cancer cell proliferation and survival.
The cornerstone of this research is the use of data from 930 distinct cancer cell lines. Researchers have pinpointed which patients could benefit most from these experimental treatments by using machine learning algorithms to identify prospective drug targets.
This method offers a more effective and individualised cancer treatment pathway, which is a change from standard approaches.
The innovative Cancer Dependency Map project, which aims to map out every vulnerability in every type of cancer, is at the centre of this endeavour. This map serves as a hope light for the development of targeted medicines in addition to being an invaluable tool for understanding disease.
The significance of this discovery cannot be overstated, particularly in light of the fact that cancer accounts for one in six fatalities worldwide, making it one of the major causes of mortality.On the other hand, there are several obstacles in the way of an efficient cancer treatment. Ninety percent of drug research projects fail, which makes it an expensive and frequently futile procedure. Therefore, the goal of this research is to completely transform the way that cancer is treated, not only to find new therapeutic targets.
To find out how to target cancer cells most efficiently, the researchers use state-ofthe-art genetics and computational biology.
It is possible to determine which tumours may be treated with current drug development techniques and which ones require new and creative ways by
employing this method, which has two advantages. Drug researchers can speed up the development of new medications and provide millions of cancer patients worldwide with hope and healing by concentrating on the most valuable targets. In essence, this finding marks a paradigm shift in cancer treatment. The scientific community can create more intelligent and efficient treatments by taking a more targeted and data-driven approach. This will bring us one step closer to a time when cancer is no longer a fatal illness but rather a treatable one
With the University of Washington, Microsoft Research is creating a technique to write megabytes of data per second on artificial DNA that can be read for millennia. When it comes to long-term storage, only a portion of the nine zettabytes of data storage that IDC projects will be required by 2024 will be used.
On the other hand, massive datasets from research and business, such pension data or data streams from the Large Hadron Collider, must be preserved.
The Vatican Apostolic Library manuscripts that were scanned using the PIQL digital preservation technique were placed in the Arctic Code Vault in 2020 together with 21TB of data from GitHub. T
his outlasts the tape archives by a significant margin, as they require rewriting approximately every 30 years. More than an exabyte of data might be stored by DNA in a single cubic inch for long-term storage.
Because parallelism fits more functionality into a given amount of space, it is the key to speed and cost reduction.
Microsoft Research has developed a method to construct and pack a thousand times more closely together the areas that DNA develops in: an array of electrodes in small glass wells, each surrounded by cathodes. In this technique, the size of the spots and the spacing between them are important variables.
In order to prepare the DNA chain for the next base to be attached, applying a voltage at the anode produces acid, and at the cathode, it releases the appropriate base. Acid that leaks from one glass well will not reach another well; instead, it will flow into the base that the cathode creates.
A DNA writer and molecular controller on a device with a PCIe interface have been created by Microsoft. Longer strands exhibit more mistakes, and the tiny DNA writing apparatus is currently producing strands up to 100 bases long.
The reagent fluid delivery system could be made more sophisticated in order to improve this as hardware advances. No more than current storage technologies, DNA data storage does not have to be entirely error-free.
Redundancy is incorporated at several levels, the first of which is called physical redundancy by Strauss. This is the process of making multiple copies of the DNA.
Logical redundancy is another built-in error correction technique that has a cost comparable to error-correcting memory.A 2KB/second write speed can be achieved using the proof-of-concept hardware. The 130nm process node that Microsoft utilised to construct this system will be used in the future to add circuitry to govern millions of electrode locations.
As a result of switching to more compact, contemporary techniques, arrays will be able to accommodate billions of electrodes and store megabytes of data per second, making them more economically and functionally comparable to tape storage.Faster writing is not as important as throughput. On the more significant front, Microsoft has been optimising writing DNA data bandwidth. The latency for reading is going to be improved.
In addition, Microsoft intends to improve the chemistry of the chemicals and solvents that are currently used with DNA which are based on fossils. Enzymes will expedite the chemical reactions that fundamentally construct the DNA chain and be more environmentally friendly.
Utilising ultrasonic technology, researchers at Concordia University in Montreal have made significant progress in the fields of tissue engineering and bioimplant repair by remotely printing three-dimensional structures. This amazing achievement was made possible by mechanical engineers Shervin Foroughi and Mohsen Habibi, and it has the potential to completely transform industrial maintenance as well as less invasive medical operations.
The discovery by the Concordia research team, dubbed "direct-sound printing," is a major advancement since it uses sound waves released from behind a barrier to create solid objects. The technology offers up a world of possibilities even if it is still in its early phases and faces obstacles on the way to being commercially viable. Using a nozzle to extrude materials, traditional 3-D printing techniques harden the materials layer by layer with heat or light. Direct and concentrated contact between the energy source and the substance is necessary for this hardening process. By utilising sound waves, the Concordia researchers aimed to get beyond these conventional approaches' drawbacks.
One special benefit of sound waves is that they may quickly focus and manipulate energy without requiring direct contact with the material. The group came to the conclusion that ultrasonic technology may be utilised to start chemical reactions in liquids at ambient temperature even in locations where access to heat and light is restricted.
Acoustic cavitation is a phenomena that holds the secret to this breakthrough. Tiny bubbles formed in a fluid by ultrasonic vibrations burst, producing extremely high pressures and temperatures at specific locations. The quick heating that follows creates the perfect environment for instantaneous 3-D printing.
In one set of trials, the scientists combined a common polymer with a curing agent and filled a chamber, then immersed it in water. They focused the ultrasound beam into the liquid polymer using a biomedical ultrasound transducer. Tiny bubbles formed along the transducer's path as they followed a predetermined pattern, hardening the substance. Using this technique, the team was able to effectively 3-D print a variety of objects, such as gears, honeycomb structures, and maple leaves, inside the liquid bath.
In 2022, their research was published in NatureCommunications. Tissue engineering and bioimplant repair have a great deal of potential thanks to this innovative 3-D printing method. Through the application of sound waves, scientists could be able to do minimally invasive surgeries inside the human body, trating diseases that were previously difficult to treat.
Moreover, the technology holds promise for industrial repairs by providing access to hard-to-reach places, like the fuselage of an aeroplane. Experts in the industry are enthusiastic about sound-based printing's ability to quickly produce complicated three-dimensional geometries, even though it may take some time for it to find its place.The application of ultrasound technology to tissue engineering and bioimplant repair may, as research develops, lead to a new age in medical care and industrial developments. The groundbreaking research conducted by the Concordia University team is evidence of the creative opportunities that arise from the combination of sound waves and three-dimensional printing.
A new injectable technique known as a bacterial "nano syringe" has the potential to enhance gene therapy by deftly introducing gene-editing enzymes into different kinds of cells.
A bioluminescent bacteria called “Photorhabdus asymbiotic”a has been repurposed by scientists into a contractile injection device that can cling to human cells and introduce big proteins into them. This may offer a means of introducing different therapeutic proteins—including ones with the ability to "edit" a cell's DNA—into any kind of cell.
For researchers that are interested in modifying genes, the nano injector may offer a vital tool. The largest unresolved issue with gene editing is likely delivery, but because the technology can be made to bind to specific cancer cell receptors, it has potential as a cancer treatment.
By transferring the injector's genetic blueprints into Escherichia coli bacteria, the researchers were able to generate the tiny injectors in large quantities.
After reading two publications on Photor habdus's injection method, Zhang and his graduate student Joseph Kreitz reasoned that since it could inject into an animal cell, it might also function on human cells.
By introducing the injectors' genetic blueprints into Escherichia coli bacteria, they were able to generate the tiny injectors in large quantities. The tiny needles, which the E. Coli dutifully secreted, bound to the insect cells and delivered their toxins as predicted.
They did not, however, function when tested on human cells. The researchers discovered that the modified tail fibres on the nanosyringes may adhere to the surface of some human cancer cells, where the epidermal growth factor receptor (EGFR) is located. Its selectivity was demonstrated by loading the injection system with a toxin, which killed almost all of the receptor-bearing cells while sparing healthy cells.
Additionally, the researchers discovered that by tagging the proteins with a marker that identifies them as munitions to be loaded onto a syringe's needle, the nanosyringes may load the system with a variety of protein payloads.
These proteins either destroyed or altered the genes of human cells when they were introduced. To enhance dose, each needle has the capacity to load several copies of a protein.
Though the technique is still in its infancy, Zhang intends to improve it as a delivery method and test out nonprotein payloads like DNA and RNA. It will be crucial to test the technology in larger mammals because the injectable systems contain bacterial proteins that may cause immunological reactions in people.
Future of drug discovery is being reshaped by a pioneering business located in the center of France's thriving biotech industry. Established by a group of forwardthinking scientists and businesspeople, AQEMIA has gained recognition fast for its distinct method of developing pharmaceuticals that integrates the state-of-the-art fields of quantum physics together with artificial intelligence (AI).
The ambitious goal that drove AQEMIA's founding was to find novel medications by utilising AI and quantum-inspired algorithms. This strategy represented a substantial change from conventional techniques, which mostly depended on chemical experiments and trial and error.
The creators of AQEMIA saw a quicker and more precise approach to drug development by utilising quantum physics to comprehend molecular interactions at a level never before possible and AI to sort through large chemical landscapes.
At AQEMIA, technological innovation has reached truly revolutionary levels. The business created a special platform that combines generative AI and deep physics. With the use of this platform,
They are able to model and forecast the behavior of millions of chemical compounds in order to determine which have the best chance of becoming approved medications. Due to the method's great cost-effectiveness and speed compared to conventional drug discovery techniques.
Global pharmaceutical behemoth Sanofi was drawn to AQEMIA's abilities. The two businesses started working together on research for several years with the goal of finding small molecule medication candidates in different therapeutic areas. With this collaboration, AQEMIA achieved a noteworthy milestone and got the chance to demonstrate the strength of its technology on a worldwide scale.
The partnership is set up to cover every step of the drug research process, from finding early hits to choosing potential development prospects. AQEMIA's methodology is distinct from other AI-driven platforms since it can produce the required chemical experiment data internally.
This capacity eliminates the requirement for pre-existing chemical data by enabling the company to execute very efficient physics-based calculations from the outset of any project. This feature of AQEMIA's technology is revolutionary in the industry since it provides a degree of speed and accuracy never before possible.
The work of AQEMIA has a huge potential impact on healthcare and medicine.
AQEMIA is well-positioned to target key diseases that have long remained untreated with viable medicines by speeding up the drug development process and bringing novel treatments to market sooner.
They see a future in which medication discovery is as creative and flexible as the IT sector, enabling patients to get life-saving therapies at a rate never before possible.
The science of genomics is undergoing a revolution thanks to genome editing technology, which modifies gene expression without affecting the underlying DNA sequence and treats a variety of disorders.
The dynamic and complex layer of genetic regulation known as the epigenome is made up of chemicals and proteins. It can bind to DNA and govern many biological processes, such as regulating the creation of proteins in certain cells or turning on or off genes.
Utilising engineering and epigenetic profiling, businesses such as Moonwalk Biosciences are creating treatments that take advantage of the body's innate gene regulatory mechanisms. By controlling numerous genes simultaneously, this method seeks to permanently alter the basic DNA sequence without compromising its integrity.
Moonwalk's technology includes a'read' component that gathers methylation data over the entire genome, providing insights into both healthy and sick cell states. Moonwalk can determine which medicinal targets are most effective by comprehending these intricate methylation patterns.
Next follows the 'write' part, which precisely modifies these methylation statuses utilising in-house editing technologies.
Epigenetic treatments have substantial potential advantages. Unlike classical gene editing, which involves cutting and pasting DNA and comes with hazards including accidental insertions or structural changes, epigenetic editing focuses on methylating or demethylating DNA.
This lowers the risk profile without sacrificing the changes' permanence because epigenetic modifications are known to endure through cell divisions.
The epigenome is a window into the past of a cell, preserving physiological alterations and persistent environmental effects. It may be possible for scientists to correct abnormal gene expression profiles that cause disease by modifying the epigenome.
Moonwalk is in a unique position to advance in this rapidly developing industry because of its ability to precisely read and write the epigenome, as well as the experience of its staff and proprietary technology.
The revolutionary potential of epigenomic editing is demonstrated by Moonwalk's audacious strategy. Through exploring the depths of the genome's regulatory processes, the company hopes to open up new therapeutic avenues, providing hope in areas where conventional approaches have run their course.
Moonwalk’s path could alter genetic medicine and usher in an era where the onceelusive cure
Startups are actively seeking capital to advance the development of AI-enhanced protein design. This occurs concurrently with Generate Biomedicines and Amgen inking a $50 million drug development agreement that could bring the company's total value to over $1.9 billion.
In order to continue funding its protein design initiatives, Arzeda has obtained $33 million in series B funding. Other startups have also joined the space, including Monod Bio and Cradle.
Real-world protein structure prediction is already a strong suit for machine learning (ML) and other AI-based computational methods. Since its release in July 2021, AlphaFold 2, a DeepMind algorithm that can accurately predict protein structure based just on an amino acid sequence, has gained widespread recognition.
Made-to-order proteins, including ones with functionalities not seen in nature, might also be produced by this ML toolset.
According to Lucas Nivon, CEO and cofounder of Cyrus Biotechnology, 30 years from now, 30, 40, or 50% of medications will be computationally created proteins.
This means that the ultimate impact of in silico-designed proteins will be enormous. Instead of truly designing from scratch, businesses in the protein design area have up to now mostly concentrated on repurposing already-existing proteins to carry out new functions or improve particular qualities.
By utilising what is known about the SARS-CoV-2 spike protein and its interactions with the receptor protein ACE2, Generate Biomedicines has created a synthetic protein that can reliably prevent viral entrance across a variety of variations.
In order to get clinical testing approved, the company plans to submit Investigational New Drug paperwork in the second quarter of this year. Though it is unclear when de novo design will become the norm, more ambitious projects are in the works.
With the work of academic academics like David Baker and colleagues at the Institute for Protein Design at the University of Washington, the area of AI-assisted protein design has roots spanning more than 20 years.
Driven by the quick advancement of machine learning algorithms and deep learning techniques, Baker's team created Rosetta, a fundamental software suite for predicting and modifying protein structures, along with other potent tools for protein design.
Scientists are developing cutting-edge solutions to address the world's expanding need for animal feed, which is being driven by a growing population and greater health consciousness. Through 2030, the market for animal feed, estimated to be worth $570.72 billion globally in 2022, is anticipated to expand rapidly.
A group of Chinese scientists from the Tianjin Institute of Industrial Biotechnology, Chinese Academy of Sciences, have developed a novel method for producing microbial protein using coal—more precisely, coal-derived methanol.
Gasification of coal to create methanol, which is subsequently utilised as a fuel for microbial fermentation, is essential to converting coal into proteins. Methanol is metabolised by the yeast Pichia pastoris, which is designed to use it efficiently. This allows the plant to develop and create biomass that is high in protein.
This method offers a revolutionary approach to the manufacturing of sustainable animal feed through a sophisticated fusion of chemical engineering and biotechnology.
Going back to the main story, coal presents a viable substitute for conventional protein sources since it is abundant, with a global stockpile of roughly 1.07 trillion tonnes. Gasification is one way to turn it into methanol, which works well in fermentation operations.
The scientists attained an astounding protein conversion rate of 92% of the theoretical value by using the yeast strain Pichia pastoris, which is renowned for its capacity to thrive efficiently on methanol. This technique has the potential to revolutionise the manufacture of animal feed since it produces high dry cell weight and crude protein content.
The technique is not without difficulties despite its effectiveness. Because it is poisonous, methanol wastes 20% of its conversion process and produces carbon dioxide and water instead of protein. In order to improve the physiological properties and nutritional worth of the proteins generated, the research team is concentrated on streamlining the procedure.
This new method has a lot of potential for the worldwide animal feed industry. It is not impacted by seasonal fluctuations, does not require arable land, and is
environmentally beneficial. This approach yields microorganisms with a high protein content that is higher than that of natural plants, making it a very effective substitute. This method has the potential to transform the manufacturing of animal feed by producing microbial proteins with a full amino acid profile, vitamins, and other nutrients. It also offers an affordable and sustainable option.
A new age in biotechnology and the manufacturing of animal feed is already under way, with industrial-scale demonstrations currently under way. This technique has the potential to drastically alter how we generate protein for animal feed, lessening our dependency on conventional sources like beef and soybeans and opening the door to a more sustainable future.
Microbe Engineering as a Service is an innovative idea in the rapidly developing field of synthetic biology.
This cutting-edge service provides the rare capacity to create and alter microbes to satisfy particular requirements across a range of businesses.
The fundamental idea behind this service is to imagine having microbes specifically engineered to manufacture an enzyme for use in medications or biofuels.
Using cutting-edge genomic tools like CRISPR-Cas9 is essential to this process. With the use of these technologies, it is possible to precisely modify the DNA of microorganisms to add new activities or enhance desired characteristics.
These modified microorganisms have a wide range of possible uses. They might play a crucial role in the creation of vaccines or medication delivery systems in the medical field.
Applications such as bioremediation, in which bacteria assist in cleaning toxins, are beneficial to the environmental sector.
Meanwhile, these modified organisms have the potential to revolutionize agriculture by enhancing soil health and agricultural production.
Microbe engineering's efficiency and scalability are major benefits. Engineered microorganisms provide a scalable and sustainable alternative to many conventional chemical processes, and they are also more efficient at what they do.
But there are obligations attached to this invention. This service prioritizes safety and ethical issues. It is crucial to guarantee that these modified microorganisms are safe and do not harm ecosystems or people's health.
The service also entails navigating a challenging regulatory landscape to guarantee biosafety criteria are met.
Another significant aspect of this service is its collaborative character. It frequently entails cooperative interaction between scientists and clients, creating an atmosphere that is conducive to invention.
This collaboration expands the possibilities for biotechnological applications by fusing goals and experience.
Microbe Engineering as a Service is not only an academic pursuit but also a driver for change across many industries. It represents a huge advancement in the way we approach problems and create answers in the modern world by offering microbial solutions that are efficient, adaptable, and sustainable.
In the face of escalating climate change, innovative solutions for carbon dioxide (CO2) capture are crucial. Traditional methods, while effective, have limitations in efficiency and scalability. Enter the THETA cycle: a pioneering approach in synthetic biology for CO2 fixation.
This cutting-edge method proposes a novel pathway, significantly enhancing CO2 capture and conversion efficiency compared to natural processes. It stands as a beacon of hope in the quest for sustainable environmental solutions.
CO2 fixation, the process of converting CO2 into organic compounds, is a cornerstone in combating global warming. Natural processes, such as photosynthesis, have long been the primary means for this conversion. However, these processes are limited by their slow rate and dependency on specific environmental conditions.
The advent of synthetic biology offers new avenues to optimize and accelerate CO2 fixation. The THETA cycle represents one such innovative approach, aiming to overcome the limitations of natural processes through a synthetic, more efficient pathway.
The THETA cycle is a synthetic pathway for CO2 fixation, distinct in its integration of 17 enzymes sourced from nine different organisms. This unique assembly allows for an unprecedented level of efficiency in converting CO2 into organic compounds.
The cycle is divided into three modules: the first focuses on CO2 fixation, the second on reducing power generation, and the third on product synthesis. Each module performs specific reactions, working in tandem to enhance the overall conversion process.
This ingenious configuration not only improves efficiency but also offers versatility in the type of compounds produced, ranging from fuels to feedstocks.
Successfully implemented both in vitro and in vivo within E. coli, the THETA cycle has demonstrated its practical viability. In laboratory settings, it has shown a remarkable ability to efficiently convert CO2 into a range of useful compounds.
This success indicates a significant step forward in synthetic biology, showcasing the potential of engineered pathways in environmental applications.
The implementation within E. coli also hints at the possibility of wider applications across different biological systems, expanding the reach of this innovative technology.
THETA cycle's success heralds a new era in synthetic CO2 capture, offering a promising avenue for sustainable environmental management. Its potential impact on reducing atmospheric CO2 levels and generating valuable compounds is immense. Ongoing R & D will refine and expand its applications, contributing significantly to global sustainability efforts. (Graph source- Nature bioscience)
IHC is a laboratory method that helps visualise the distribution and localization of certain proteins by identifying them inside tissue sections. The immunological system's antibodies, which attach to and recognise particular protein segments known as antigens, form the foundation of IHC.
To identify microorganisms that cause pneumonia, Dr. Albert Coons utilised a fluorescently labelled antibody in 1942, which is when the technique known as immunohistochemistry was originally developed in the 1930s.
To maintain their structure, tissue samples are first "fixed," or maintained, for IHC. The next step involves blocking any unintended, non-specific antibody binding.
Once the target protein has been bound to by the primary antibodies, secondary antibodies that aid in signal amplification are frequently added to the samples. Next, a colour shift (chromogenic detection) or fluorescent signal—which can be seen under a microscope is used to indicate the target protein's presence.
Multiple protein detection can be done at once using multiplexing, an enhanced version of IHC. The comprehension of the spatial linkages and interactions among various cell types, proteins, and other molecules is particularly helpful for investigating intricate environments seen in tissues, such tumors.
For predicting patient outcomes and being useful in the diagnosis of cancer, this is multiplexing “immunohistochemistry”.
The way the tissue is treated affects the IHC techniques. There are primarily two types of tissue sections: frozen (Fr) and formalin-fixed paraffin-embedded (FFPE).
For the long-term preservation of samples, frozen fixed paraffin embedding (FFPE) is recommended as it retains more of the tissue's structural characteristics.
Nonetheless, it's thought that frozen tissue slices preserve protein expression and enzyme activity more effectively. The protein being investigated, its position within the cell, and the preferred detection technique all influence which of these approaches is chosen.
For the layman, immunohistochemistry (IHC) is similar to an extremely focused staining method that enables researchers to identify and quantify the presence of specific proteins in various tissue sections by color-coding the proteins within the tissues.
This approach plays a critical role in helping to guide therapy decisions, especially with cancer, and in understanding diseases at the molecular level.
DNA writing methods are developing quickly as we explore more of the field of synthetic biology, which is moving us closer to the synthesis of entire genomes.
The ability to manufacture DNA fast, cheaply, and accurately is critical to the advancement of this discipline.
Conventional chemical synthesis techniques are effective in producing short DNA sequences called oligonucleotides, but they are not as effective in assembling these sequences into longer DNA sequences that span a chromosome.
Made-t-order DNA is the fundamental component of synthetic biology. DNA writing satisfies the three requirements of speed, economy, and accuracy is necessary to fully realise the potential of this emerging industry.
But the limitations of conventional chemical synthesis become evident as we set out on the audacious goal of creating whole synthetic genomes.
"It's affordable to buy a human genome in oligos, but you can't assemble it," points out George Church of the Harvard University-based Wyss Institute as a major obstacle.This problem stems from the high incidence of mistakes that occur while putting these small DNA sequences together to form an entire genome.
New technologies that have the potential to transform DNA synthesis have been spurred by the effort to overcome these challenges. With these developments, we want to make the process of building entire synthetic genomes more efficient by eliminating the need to start from scratch when producing huge DNA sequences.
One such method is the synthesis of DNA using biological systems and enzymes. Comparing this process to chemical synthesis, known as enzymatic synthesis, may result in increased precision and decreased error rates.
The automation of DNA synthesis is another innovative breakthrough.
The development of automated platforms aims to improve the overall correctness of the synthesized DNA by reducing human error and speeding up the process.
One of the most important steps towards assembling huge genomes is that these platforms can synthesize longer DNA sequences with fewer mistakes.
Furthermore, DNA writing technologies now function as an integral component of advances in mistake correcting approaches. To make the final output as error-free as feasible, complex algorithms and molecular techniques are being used to find and fix mistakes in the synthesized DNA.
A growing focus is focused on bringing down the cost of DNA synthesis, in addition to these technological advancements. Synthetic DNA is becoming more widely available and reasonably priced thanks to economies of scale and advancements in synthesis techniques.
In addition to helping academic research, the democratization of DNA writing technology has significant ramifications for a variety of businesses, including agriculture and medicine. In conclusion, quick development and innovation are anticipated in DNA writing technology. The problems of precision, velocity, and expense in the process of creating synthetic genomes are being tackled by combining enzymatic synthesis, automation, mistake correction, and affordability.
A group of scientists has developed small micro-robots that can examine individual cells to determine their health. These tiny particles which are barely ten microns in diameter have the capacity to move particular cells to a different site while simultaneously distinguishing between healthy and damaged cells.
These micro-robots have the potential to be a game-changing tool for targeted medicine administration and illness detection since they accomplish this through the application of electricity or a magnetic field.
The researchers created these micro-robots by taking inspiration from the natural roles that microorganisms in our bodies play, as described in a study that was published in the journal Advanced Science. "Biological micro-swimmers like bacteria and sperm cells inspired us to develop the micro-robot's ability to move autonomously," a lead researcher said. "
There are many applications for this cutting-edge research area in fields like environmental science, medicine, and as a research tool. It is evolving quickly."
These small robots have a wide range of possible uses. For biopsies and medical diagnosis, they could be utilised, for example, to separate cancer cells.
The study team was able to isolate cancerous and healthy cells within a single bacterium by using these robots to distinguish between them during testing.
"Our new development significantly advances the technology in two main areas: navigation by very different electric and magnetic mechanisms, and hybrid propulsion," the researcher continued.
"In addition, the micro-robot exhibits an improved capacity to recognise and seize a solitary cell without the requirement for labelling, enabling on-site examination or extraction and conveyance to an external apparatus for comprehensive examination."
The study team wants to develop these micro-robots for use in internal body functions in the future. "Effective drug carriers that can be precisely guided to target areas" is one of the main objectives.
The way therapies are delivered could be completely changed by such developments, especially when it comes to treating diseases like cancer, where precise drug distribution can greatly enhance therapeutic results.
An important advancement in medical technology has been made with the creation of these micro-robots. This work opens the door to more individualized and efficient treatments by combining robotics and cellular biology.
These micro-robots hold the promise of a new era in medical diagnosis and treatment due to their capacity to carry out complex activities at the cellular level, providing hope for more effective and minimally intrusive therapies.
It is projected that as research develops, these micro-robots will have uses outside of medicine, such as environmental monitoring and as potent instruments in biological research. Their small size and precision control make it possible to explore and intervene at a scale that was previously thought to be impossible.
Forecasted to soar from $17.66 billion in 2021 to an astounding $44.19 billion by 2030, the bioprocessing market is undergoing a massive upswing.
The bioprocessing sector is changing quickly, as evidenced by this growth trajectory, which shows a compound annual growth rate (CAGR) of 11.0% from 2022 to 2030.
The current dynamism of the sector is a result of both an increased demand for biopharmaceuticals and revolutionary advancements in biotechnological processes.
As per the company's study, the upsurge in demand for customised medical treatments, the growing prevalence of chronic illnesses, and the expanding use of bioprocessing in other industries are the main drivers of this growth.
In order to produce vital bioproducts including therapeutic proteins, vaccines, and other bio-based materials, bioprocessing is becoming more and more important.
It is reshaping to meet the changing demands of environmental sustainability, healthcare, and more efficient, large-scale biomanufacturing processes. It is playing a crucial part in the continuing biotechnology revolution.
This market is expanding thanks to a number of important factors:
The demand for biopharmaceuticals is on the rise. According to Next Move Strategy Consulting, the growing demand for biopharmaceuticals around the world is one of the key factors propelling the bioprocessing industry.
These include recombinant proteins, monoclonal antibodies, and vaccinations, which are being employed more and more to treat a variety of illnesses.
To meet the production demands of these complex, high-value medicinal medicines, this trend calls for the development of sophisticated bioprocessing technologies.
Innovations in Single-Use Technologies with the adoption of single-use bioprocessing technologies, the industry is undergoing a revolution. Numerous benefits, including cost savings, increased flexibility, and decreased contamination risk, are provided by these systems.
Scalability and efficiency are being improved by the trend in bioprocessing workflows towards the use of disposable components.
The field of healthcare is witnessing a transition towards personalised medicine, with bioprocessing playing a vital role in this movement.
Advanced cell and gene therapies are among the personalised ways that bioprocessing technologies are evolving to handle as the industry adjusts to manufacturing patient-specific medications.
The practice of outsourcing biomanufacturing is rising. To expedite product releases, reduce costs, and streamline production, biopharmaceutical companies are working with contract development and manufacturing organisations, or CDMOs.
The competitive dynamics of the bioprocessing business are being drastically changed by this development.
Dedication to Environmental Sustainability In response to growing environmental awareness, the bioprocessing industry is implementing more environmentally friendly procedures.
The goal is to design technologies that minimise resource use, minimise the impact on the environment, and maximise energy efficiency.
This dedication to eco-friendly bioprocessing methods is consistent with international initiatives to promote a more sustainable and environmentally aware future.
To sum up, these developments emphasise the market for bioprocessing's critical role in adopting effective and sustainable manufacturing techniques while tackling present and upcoming healthcare issues.
The tremendous progress in gene and cell therapy is driving a revolution in the biopharmaceutical industry.Now well in 2024 this rapidly evolving discipline is not only changing the landscape of treatment approaches but also opening the door to possible treatments for illnesses that were previously thought to be incurable.
27 gene and cell therapy products have already received FDA approval in the United States, but this is only the beginning. Many more candidates are presently undergoing clinical trials and development.
Among the biomedical fields that are expanding the fastest are cell and gene treatments, which provide both new therapeutic options and promising business opportunities. To treat or cure diseases, these medicines modify particular genes or biological systems. For example, gene therapy includes introducing, modifying, or turning off genes in a person's cells in order to treat or prevent disease, whereas cell therapy replaces unhealthy or malfunctioning cells with functional, healthy ones.
There are multiple variables driving the acceleration in this field. Technological developments like CRISPR gene-editing have made it feasible to modify genes with previously unheard-of accuracy and effectiveness. Furthermore, increased funding and research have been spurred by the effectiveness of these treatments in curing a variety of ailments, from uncommon genetic illnesses to more prevalent diseases like cancer.
Through these therapies, we are also seeing a trend in 2024 towards personalised medicine. Customised therapies that focus on a patient's unique genetic composition or the genetic characteristics of their illness are becoming increasingly common; these therapies hold the potential to be more effective and cause fewer negative effects than conventional ones. The idea of patient care is being revolutionised in oncology and other fields by this individualised approach.
Furthermore, these medicines have important economic ramifications. Because of the difficulties involved in their research and production, they are frequently expensive, but the money spent is justified by the possibility of long-lasting or even curative treatments. These therapies should become more widely available as more are authorised and as production procedures grow more efficient and economical.
Nonetheless, there are drawbacks to this quick expansion. Laws and regulations are changing to keep up with these innovations in technology. Three crucial concerns that require ongoing attention include ensuring patient safety, ethical difficulties surrounding gene editing, and resolving the high prices of these medicines.
In summary, cell and gene treatments represent the vanguard of a medical revolution in 2024, not only a small segment of the biopharmaceutical industry. They have enormous potential to transform the way diseases are treated and cured. The future of healthcare will surely be greatly influenced by cell and gene therapy provided there is constant innovation, funding, and prudent regulation.
2023 has seen revolutionary shifts in the biotechnology field-BIO GEN TECH,ushering in a new chapter in the history of medical science. The FDA's groundbreaking approval of the first gene-editing therapy, a significant step towards the precise treatment of genetic illnesses, was essential to these advances.
Millions of people, particularly those of African heritage, who have long expected such a breakthrough are encouraged by this decision, which emphasises a major shift in medical research objectives by concentrating on medicines for severe sickle cell disease.
This FDA approval heralds a new era in genetic medicine and goes beyond merely being a significant milestone for sickle cell disease. Gene editing, which was long thought to be a future idea, is now recognised as a workable method that can provide accurate treatments by identifying and fixing the genetic mistakes that cause most diseases.
Compared to traditional therapies, which frequently merely treat symptoms, this strategy is very different.This discovery has far-reaching consequences that go well beyond a specific illness. It highlights the tremendous therapeutic potential of gene editing, providing new opportunities for the treatment of a variety of hereditary illnesses, such as Huntington's disease, Duchenne muscular dystrophy, and cystic fibrosis.
Thanks to gene-editing techniques, several long-challenged medical illnesses may soon have viable treatments.Beyond gene editing, the biotech industry has made incredible strides this year.
Novel approaches to replacing or repairing damaged tissues are promised by developments in regenerative medicine and personalised medicine, which is catered to each patient's unique genetic profile.
These developments have been accelerated by the application of AI and machine learning to disease diagnostics and drug research, which has increased effectiveness and efficiency.
Collaboration between academic institutions, biotech companies, and regulatory agencies has been essential to these advancements. Through this collaboration, innovative therapies that satisfy the highest criteria of safety and efficacy have been
developed and approved more quickly. Consequently, patients are now able to receive novel treatments more quickly than before.
In summary, the upcoming year will be a critical turning point for BIO GEN TECH, expanding the scope of medicinal potential and setting the stage for new developments.
With great promise for future developments in Bio Gen Tech, we are witnessing the dawn of a time when previously incurable diseases will be cured and personalised healthcare will no longer be a pipe dream but an achievable reality.
I appreciate your reading this month's issue of my independent futurology Chronicle. My mission is to provide you with a new, unbiased viewpoint on the most recent progress in science and technology, the advancement of space exploration, and the critical problems and solutions associated with climate change.
As a nonprofit publication, I work. with total editorial autonomy and flexibility, ensuring that my ideas stay impartial and objective.
In the months to come, I want to provide you with more interesting and educational information, and I thank you for your friendly support.
www.frank.blue