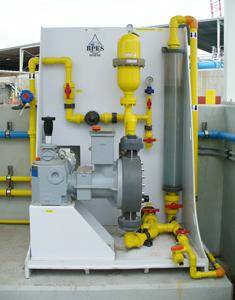
21 minute read
C Factor—Kenneth Enlow
C FACTOR Disinfection: Part One
Kenneth Enlow
Advertisement
President, FWPCOA
Greetings everyone. Here we are at midyear already. July brings hot weather, resulting in warmer water. This is the time of year when we face some of our most difficult disinfection challenges.
For July’s C Factor I am starting a two-part series on disinfection. This month’s column will take a look at the history and methods of disinfection.
The History of Disinfection
The Origin of Disinfection
The disinfection of water probably has been practiced for millennia, but obviously with little or no understanding at first of the principals involved in its improvement of water quality. Records show that the boiling of water was practiced as early as 500 B.C.
Until the germ theory of disease was established in the mid-1880s, odors were believed to be the transmitter of diseases. It was commonly believed that the control of odor would limit the spread of infection. From this erroneous belief, the disinfection of both water and sanitary wastes evolved.
Rational of Disinfection
Water disinfection involves specialized treatment for the destruction of harmful and otherwise objectionable organisms, primarily disease-producing (pathogenic) organisms and particularly bacteria of intestinal origin.
Efficiency of Disinfection
The major factors influencing the efficiency of disinfection are as follows: S Kind and concentration of organisms to be destroyed S Kind and concentration of disinfectant S Contact time provided S Chemical character and temperature of the water to be treated
The resistiveness of the same and different kinds of organisms to a specific disinfectant varies considerably. Nonspore-forming bacteria are less resistant than spore-forming types. Cysts and viruses are sometimes quite resistant and may require treatment in degree or kind different than that for less-resistive organisms.
Organisms within the same species can have varying degrees of resistance. The concentration of organisms in high numbers can cause clumping that forms a barrier against disinfection processes.
It’s very important to note that the kill of a species of organisms by a particular disinfectant, other factors being constant (pH, temperature, etc.), is proportional to the concentration of the disinfectant and the reaction time.
Means of Disinfection
There are several means of accomplishing disinfection, excluding the water treatment process, that result in the partial removal of potentially infective and objectionable organisms, such as sedimentation, coagulation, filtration, etc. They are: Physical treatment - such as the application of heat or other physical agents. Irradiation - such as by ultraviolet light. Metal ions - such as copper and silver. Alkalis and acids - such as contact with alkaline or acid waters related with pH adjustment and corrosion control, lime softening, etc. Surface-active chemicals - such as quaternary ammonium compounds (any of a group of compounds in which organic radicals replace hydrogen atoms of the ammonia radical used as solvents, antiseptics, and emulsifying agents). Oxidants - such as halogens, ozone, and other inorganic or organic material.
The criteria for determining the suitability of various potential water disinfectants are: S The ability of the disinfectant to destroy
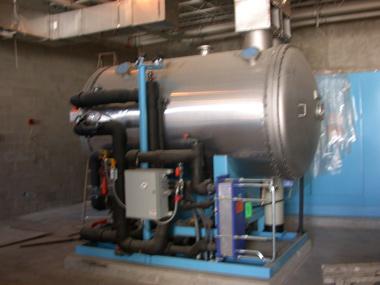
the kind and number of organisms present within the contact time available and given the physical characteristics of the water. S The availability of the disinfectant at a reasonable cost and in a form conveniently, safely, and accurately applied. S Ability of the disinfectant, in the concentrations employed, to accomplish the results desired without rendering the water toxic or aesthetically objectionable. S Ability of the disinfectant to persist in residual concentration to prevent recontamination. S Adaptability of a practical, duplicatable, quick, and accurate assay technique for determining disinfectant concentration for operating control of the process and for measuring the efficiency of the disinfectant.
Methods of Disinfection
Heat and Other Physical Agents
The important waterborne diseases are not known to be caused by spore-forming bacteria or other heat-resistant organisms; therefore, water can be disinfected by subjecting it to heat. Subjecting water to boiling temperatures for about 15 to 20 minutes will render it potable. This is why health departments will issue an emergency “boil water” order when a water system has been compromised due to a lowpressure condition or loss of chlorine residual. Boiling water as a disinfection method is impractical on a routine or large-scale basis.
The physical and biological effects of ultrasonic frequency waves have been a subject of laboratory investigations since 1926. The United States demonstrated in 1951 the lethal effects of ultrasonic energy at 400kc/sec on aqueous suspensions of E. coli at 98°F, resulting in complete sterility after an exposure of 60 minutes. Estimation of the operating costs of this method is approximately $15,000 to sterilize one million gallons, making it uneconomical and impractical for application to domestic water treatment.
Irradiation
The ultraviolet (UV) method of disinfection involves exposure of a film of water to one or several quartz mercury-vapor arc lamps emitting germicidal UV radiation at a wavelength in the range of 200 to 295 nm. Contemporary UV units can operate on standard 110 volts alternating current power.
The earliest known positive mention of the successful application of UV for water disinfection was 1877, and again in 1909 to 1910 in Marseilles, France. The first use in the U.S. of UV for water treatment was in Henderson, Ky., in 1916.
Between 1916 and 1928 three other U.S. municipal installations were made, but by 1939 they all had been abandoned primarily due to the greater cost of operation and maintenance compared with other disinfection processes. The UV treatment is still used with success, but as in the past, where it was limited to small systems, larger-system applications are being developed. Its use is more common in Europe, especially in France where it was first developed.
Metal Ions
It has been known for many centuries that copper is slightly bactericidal and strongly algacidal. Other metals, such as mercury and silver, are also effective in minute concentrations. The name “oligodynamic” has been given to the sterilization action of minute quantities of metals.
As early as 1869 the properties of silver were studied for their ability to kill organisms. The common use of silver for surgical instruments and for the impregnation of bandages and ointments, and the use of silver nitrate, argyrol, and other forms of colloidal silver suspensions for the treatment of various body infections, are well established.
In England in 1932, a paper was published on the use of silver for water disinfection. Later papers were published between 1934 and 1936 in the U.S.
The English paper dealt with laboratory experiments with silver-coated sand. The U.S. experiments dealt with filters containing metallic silver, with solutions of silver salt, and with electrocatynization, whereby finely divided silver was produced by an electrolytic system. Concentrations of silver are of a low magnitude, but contact times are long. There is a maximum contaminant level (MCL) of 0.1 mg/l for silver, so doses are limited.
It was observed by the U.S. Public Health Service in 1962 that, because of its relative expense, adsorption and bacteriostasis characteristics, long reaction time, and other factors, silver is not a very useful disinfectant in large applications. Silver is used very little in others countries; in Austria and Belgium, silver has been used in isolated incidents at private water supply installations. In France it’s said to be limited to the food and carbonated beverage industry.
Alkalis and Acids
Pathogenic bacteria normally do not survive long in strongly alkaline or acidic waters. Though of very limited direct interest as disinfectants, the application of certain material for pH and corrosion control, lime softening, etc., can affect a partial reduction of some microorganisms in water, especially where long contact periods are available.
Surface-Active Chemical
Cationic detergents are highly germicidal, but anionic detergents are a weak germicide. Neutral detergents occupy an intermediate position as a germicide. Quaternary ammonia compounds (QAC), known since 1916, include a wide range of cationic detergents that readily ionize in water. They work best in waters with a pH of 7 to 9.
Their mode of action as disinfectants is considered different from that of gaseous chlorine. They have a limited use in the lack of quantitative chemical tests of residual that can be interpreted in terms of germicidal effect. The treatment cost is about 70 times greater than chlorine at dosage concentrations of 1 mg/l. The QAC has not been seriously considered for use in potable water disinfection due to the reasons mentioned previously, possible toxic effects, and the objectionable tastes they impart. The attitude of current public health services in the U.S. is that it’s unlikely that they will be acceptable in drinking water.
Chemical Oxidants
Of these materials, the halogens, such as bromine, iodine, ozone, and chlorine, are the most widely used.
Bromine
Bromine is a dark, reddish-brown halogen that exists as a liquid at atmospheric pressure. It’s a good germicidal agent, and effective tests exist to determine residual concentrations. As in the case with chlorine, bromine combines with ammonia to form amino compounds and a similar breakpoint phenomenon has been demonstrated. Bromine and monobromamine have been reported nearly equal in bactericidal properties and essentially equal to free chlorine on a parts-per-million basis at comparable pH. There are no known applications of bromine in potable water systems. Application appears to be limited to swimming pools and industrial waters.
Iodine Iodine was introduced in water treatment in the early 1920s when it was suggested that small amounts added to water at infrequent intervals would prevent goiter. In 1923 the cities of Rochester, N.Y., and Sault Saint Marie, Mich., began adding iodine to the public water system. This practice was followed only briefly in Sault Saint Marie, but Rochester added iodine for 10 years. Iodization was also practiced in England from 1924 to 1925. With the practice of adding iodine to table salt, iodization of water died out. The use of iodine tincture for rapid sterilization of drinking Continued on page 44 Florida Water Resources Journal • July 2021 43
Continued from page 43 water was suggested by Vernoux in 1915. Further experiments of the application of iodine from 1917 and 1918 proved it destroyed diseaseproducing bacteria in water.
In 1922 the U.S. Public Health Service recommended the use of tincture of iodine for emergency disinfection of drinking water supplies. In 1941 research was conducted in the development of a method of disinfecting canteen water supplies, which would destroy amoebic cysts, as well as enteric pathogenic bacteria. By 1945 a stable tablet preparation, Globaline, had been developed and was demonstrated effective for destruction of enteric bacteria and amoebic cysts using one or two tablets per quart of water with a contact period of 10 minutes. Iodine has been found to be useful as a swimming pool disinfectant, but has not been used for large potable water systems.
Ozone
Ozone is generated by passing air through an electrical charge, creating the ozone molecule (O3). Because of its instability and other characteristics, it’s generated onsite. Discovered in 1783 by the Dutch scientist Van Marum, it was dubbed “ozone” in 1840 by Schonbein (from the Greek word meaning smell), who is said to have experimented with it for water treatment in Metz, Germany. A full-scale plant was built at Wiesbaden and Paderborn in 1896 for the purpose of disinfecting water.
Other investigations took place in Holland and France. The results of treatment at Oudshoorn, Holland, indicated the effectiveness of ozone against all spores, and pathogenic and saprophytic bacteria. This led to the adoption of ozone treatment at plants in Paris, Lille, and Nice, France, in 1898 to 1904.
By 1933 the Paris installation was treating about 90 million gallons per day (mgd) of water by ozone. In the U.S. the development of ozone, beginning in 1909, was primarily for the control of taste, odor, and color. Ozone is still employed in the U.S. as a treatment for these three, as well as a primary disinfectant. Most states require the addition of postchlorination in order to maintain a residual in the distribution system.
Chlorination
Chlorination is the most-common method of water disinfection. Chlorine is a chemical oxidant. It was discovered in 1774 by a Swedish chemist, Karl Wilhelm Scheele, as a product of the reaction of hydrochloric acid (HCI) and manganese dioxide. The gas was first liquefied in 1805 to 1806 by Thomas Northmore and was first identified as an element by Sir Humphry Davy, who named if from the Greek word cloros (meaning green) because of its characteristic color. History records its use as a disinfectant around 1800 by De Morveau in France and by Cruikshank in England.
When chlorine was first used specifically to improve water quality is not clear, but the earliest applications were for its deodorizing capacity rather that its disinfecting capacity. Bleach solutions (probably sodium hypochlorite) were being used by 1850 to treat well water, but again, apparently without definite knowledge of its capacity to destroy microorganisms. In 1854, chlorinated lime was being used to deodorize London’s sewers and was used in most of England to treat body wastes from typhoid patients before disposal to the sewers in 1879.
The earliest recorded use of chlorine directly for water disinfection in the U.S. was in January 1896 when W.M. Jewell utilized electrolytically produced chlorine gas for a week or two in conjunction with a filter study by G.W. Fuller in Louisville, Ky. The first continuous treatment process utilizing chlorination for water disinfection was in Middlekereke, Belgium, in 1902.
In 1903 studies of the destruction of pathogens by chlorine compounds were described by Lt. Nesfield of the British Indian Army Medical Service, who also suggested their application to prevent waterborne-disease transmission among military personnel. This study suggested compressing chlorine gas in lead-lined iron cylinders. The second continuous treatment process utilizing chlorination was in England at the filter plant in Lincoln. A sodium hypochlorite solution was added in an effort to combat an epidemic of typhoid.
In North America the first continuous application of a chlorinated compound for water disinfection was in 1908 where sodium hypochlorite was utilized to disinfect the 40mgd Boonton reservoir supply for the Jersey City (N.J.) Water Works.
During the infancy of water chlorination, the only commercial sources of chlorine were chlorinated lime and sodium hypochlorite bleach solutions, but in 1909, liquid chlorine became commercially available in steel containers. In 1910, Maj. C.R. Darnell of the U.S. Army Medical Corps experimentally employed chlorine gas for water disinfection in Fort Myer, Va., using a dry gas chlorine feeder of his own design, which was later patented.
The first full-scale use of liquid chlorine for water disinfection was employed at Niagara Falls, N.Y., to put down a typhoid outbreak in 1912. In 1913 improved equipment invented by C.F. Wallace and M.F. Tiernan to measure chlorine gas was used in Boonton, N.J., replacing the use of sodium hypochlorite.
The evolution of water chlorination processes began with simple chlorination of filter effluent, or in some cases, it was the only treatment provided. In 1914, prechlorination was introduced prior to filtration as an aid to coagulation.
Chlorine and Ammonia
This combined treatment was first recognized during the chlorination of sewage. It was noted that the bactericidal action continued even after all the free chlorine or hypochlorite had disappeared. Sir Alexander Houston also recognized the superior advantages of chloramine in some instances and suggested the application of ammonia prior to chlorine to avoid tastes resulting from reactions of chlorine with organic material.
In the U.S. the use of chloramines had been successfully adopted in Denver in 1916. Chlorine-ammonia treatment later lost its popularity, largely due to the advent and development of “free residual chlorination” processes and the realization of the superior bactericidal efficiency of hypochlorous acid (HOCl).
Today chloramination is employed as a postammonization process to provide a longlasting chloramine residual in potable water distribution systems. This also helps aid in the reduction of trihalomethane formation and other disinfectant byproducts in the distribution system.
The practice of superchlorination and dechlorination for the elimination of odors through oxidation was reported as early as 1912, and again in 1916. Sir Alexander Houston was the one who proposed the terms “superchlorination” and “dechlorination” in 1925, demonstrating that, the more chlorine that’s added, the more certain is the absence of taste after dechlorination.
The “breakpoint” chlorination concept began to emerge in 1939 when it was reported that some waters exhibit a break in the chlorine residual curve if a sufficient amount of chlorine is applied to the water and the contact time is adequate.
Chlorine Dioxide
Chlorine dioxide was experimented with for water disinfection as early as 1900. At about the time that breakpoint chlorination was introduced, sodium chlorite was commercially developed as a bleaching agent. This compound, which is soluble in water, readily reacts with chlorine to produce an aqueous solution of chlorine dioxide. It was adopted on a full-scale basis in Niagara Falls in 1944 for control of phenolic tastes and odors. Chlorine dioxide has the ability to disinfect water without the formation of trihalomethanes.
Chlorine disinfection was finally established on a scientific basis in the period from 1917 to
1919 when the suitability and reliability of the orthotolidine test was demonstrated. It was recommended that water systems vary the dose of chlorine to maintain some residual chlorine after treatment.
Minimum Bactericidal Residuals
These residuals were investigated from 1944 to 1948 by the Public Health Service. Data were examined from experiments at temperatures from 68 to 77°F, using 10 minutes of exposure to free available chlorine and 60 minutes exposure for combined available chlorine.
With free chlorine residual at a pH of 6 to 8, a safe residual for complete destruction of bacteria after 10 minutes contact time would be not less than 0.2 mg/l (This should be familiar to all of you as the minimum free residual allowed in your distribution system under today’s disinfection regulations).
As the pH increases the minimum residual must also increase. For combined residual chlorine at a pH of 6 to 7 a safe residual after 60 minutes contact time would be not less than 1 mg/l. The minimum required residual must be increased with the increase of pH in this case as well. Additional studies in 1965 examined data based on a 30-minute contact time.
The results confirmed a free residual of 0.2 mg/l as being adequate up to a pH of 9.2. This study indicated that higher residuals for combined chlorine would be required. A pH of below 6.8 would require a minimum combined residual of 2 mg/l and as much as 3 mg/l at a pH of 10.
Principles of Water Chlorination
Disinfection is the principle and mostcommon objective of chlorination. Chlorine also acts as an oxidizer to modify the chemical characteristics of water.
Oxidation-Reduction Potentials
Chlorine is a strong oxidizer and can be dissipated in reactions with various inorganic and organic materials in water before significant disinfection is accomplished. The oxidation-reduction (redox) potential can be considered a measure of the tendency chlorine has to react with other materials. As a general rule, chlorine reacts with fewer materials as the pH increases, and with those materials with which it reacts, the reaction rate increases with increasing temperature, although the redox potential is not a measure of the rate at which chlorine compounds react.
The oxidation reactions of chlorine with inorganic-reducing substances, such as sulfides, sulfites, ferrous iron, and nitrites, are generally very rapid. Some dissolved organic materials also react with chlorine rapidly, but most reactions with organics take hours. The redox potential for various chlorine compounds varies, but in all cases, has a positive net charge.
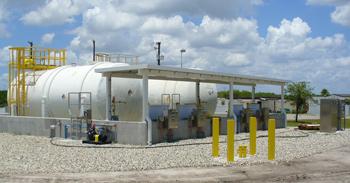
Reactions With Water
When chlorine is added to water it’s a mixture of HOCl and HCl. At ordinary water temperatures this reaction is essentially completed in a few seconds. The oxidation property of the chlorine, as well as the disinfection capacity, is contained in the HOCl formed in the reaction. The pH of chlorinated water supplies is normally within the range where chlorine may exist, both as HOCI and hypochlorite ion.
Chlorine existing in water as HOCI and hypochlorite ions is defined as free available chlorine.
Chlorine-Ammonia Reactions
These reactions are of great significance in the water chlorination processes, especially disinfection. When chlorine is added to water containing natural ammonia, or added ammonia, the ammonia reacts with the HOCl to form various chloramines which, like HOCl, retain the oxidation power of the chlorine. These reactions form monochloramine and dichloramine, depending on the pH, temperature, time, and initial ratio of chlorine to ammonia. Common ratios are a 3:1 to 5:1 relationship of chlorine to ammonia. Chlorine existing in water in chemical combination with ammonia or organic nitrogen compounds is defined as combined available chlorine.
Chlorine Demand
Chlorine demand is defined as the difference between the amount of chlorine applied to water and the amount of free, combined, or total available chlorine remaining at the end of the specified contact period. The portion of the remaining available chlorine is the residual.
Free Chlorine Residual (Breakpoint Chlorination)
When chlorine is added to water the characteristics of the water will determine the formation of free chlorine residual. When chlorine is first added to water the inorganic compounds act as reducing agents to consume the chlorine. As more chlorine is added the chlorine begins to react with the ammonia and organic matter to form chloramines and chlororganic compounds. This is the combined chlorine residual.
Adding more chlorine to the water actually decreases the combined residual as the chlorine oxidizes some of the chlororganic compounds and ammonia. The additional chlorine also changes some of the monochloramines to dichloramine and trichloramine. As additional chlorine is added, the amount of chloramine reaches a minimum value. Beyond this point further addition of chlorine produces free chlorine residual.
Points of Application
When chlorination was first employed for disinfection, terminal treatment of the purification plant effluent was almost always employed. Now chlorine is used at various stages of water treatment, as well as in the distribution system.
Plain or Simple Chlorination
This involves the application of chlorine to water that receives no other treatment. More than half of the water systems in the U.S. use this type of chlorination process.
Prechlorination This involves the application of chlorine to Continued on page 46 Florida Water Resources Journal • July 2021 45
Continued from page 45 water prior to any other treatment process. This process aids in the treatment of water by oxidizing inorganic and some organic compounds prior to coagulation/flocculation treatment or filtration. Also, the primary disinfection takes place in the prechlorination process.
Postchlorination
This involves the addition of chlorine to the water after the treatment process, usually after filtration. Chlorine is added in quantities sufficient to maintain chlorine residuals in the distribution system.
Rechlorination
This involves adding chlorine to water after previous chlorine treatment. It’s usually done at distribution pumping stations, elevated storage tanks, and in some cases, where large distribution systems need chlorine residuals boosted due to long retention times.
Dechlorination
This is the partial or complete reduction of residual chlorine in water by any chemical or physical treatment. Sometimes high levels of chlorine are required to complete the desired treatment results; when this happens, it’s necessary to reduce the chlorine residual before the water can be sent to the distribution system. Sometimes the chlorine residual must be completely eliminated or reduced substantially if storage tanks are to be drained to natural estuaries. A common compound use for dechlorination is the use of sulfur dioxide. Activated carbon is very effective for the purpose of dechlorination. Aeration is another method that has been employed. Sodium thiosulfate is the common compound for dechlorination of water samples for bacteriological analysis.
I hope you find this information on the history and methods of disinfection interesting and informative. Next month’s C Factor will take a look at the practical application of disinfection in the operation and maintenance of our potable water systems.
FWPCOA Training Update
The training office is in need of proctors for online courses in all regions. If you are available to be a proctor, please contact the training office at 321-383-9690.
In the meantime, and as always, our Online Training Institute is up and running. You can access our online training by going to the FWPCOA website at www.fwpcoa.org and selecting the “Online Institute” button at the upper right-hand area of the home page to open the login page. You then scroll down to the bottom of this screen and click on “View Catalog” to open the catalog of the many training programs offered. Select your preferred training program and register online to take the course.
For more information, contact the Online Institute program manager at onlinetraining@ fwpcoa.org or the FWPCOA training office at training@fwpcoa.org.
Fall Short School
I want to remind everyone that we will be holding the FWPCOA Fall Short School at the Indian River State College in Ft. Pierce from August 9 through 13. If you have not already registered for your classes now is the time to do it. Some classes will fill up quickly.
That’s all I have for this C Factor. Everyone take care and, as usual, keep up the good work! S
Got Leaks?
Interested in a proactive approach to fighting water loss and the ability to actually see how much you can gain if you could reliably detect leaks within your distribution network?
Contact your local Kamstrup Regional Sales Manager to learn more.
kamstrup.com/ald-us
Christian McGarrigle Regional Market Sales Manager CMG@kamstrup.com 678-727-6863 Jody Cline Regional Distribution Sales Manager JOCL@kamstrup.com 850-557-0145