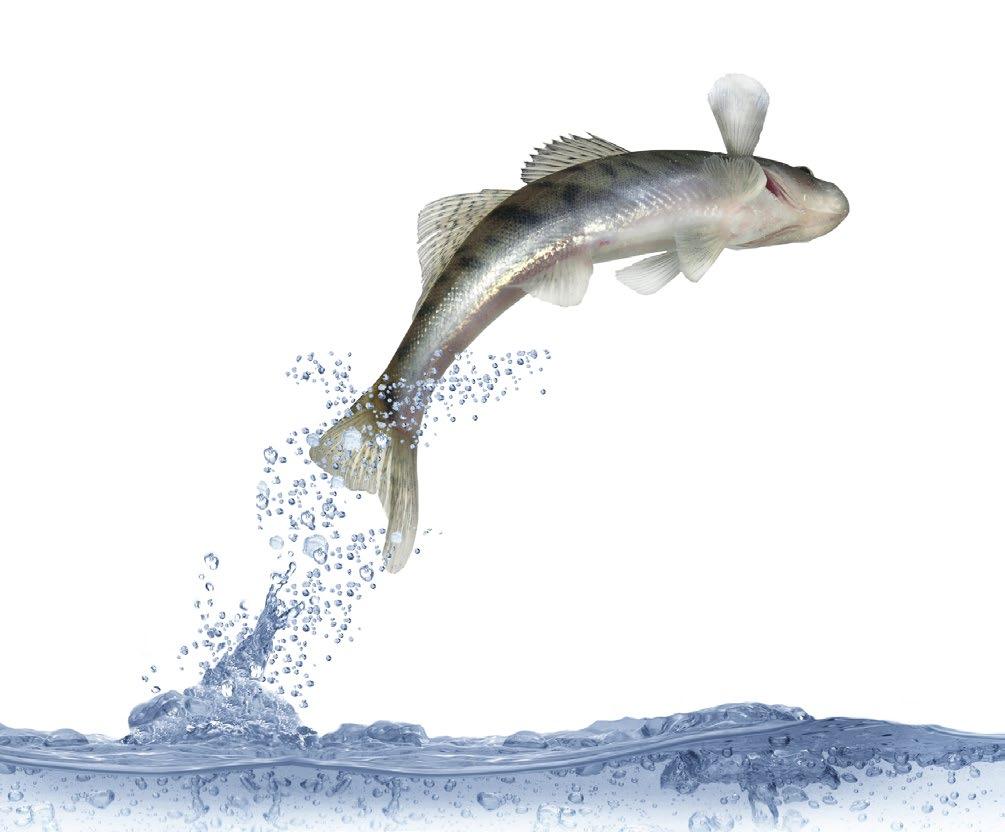
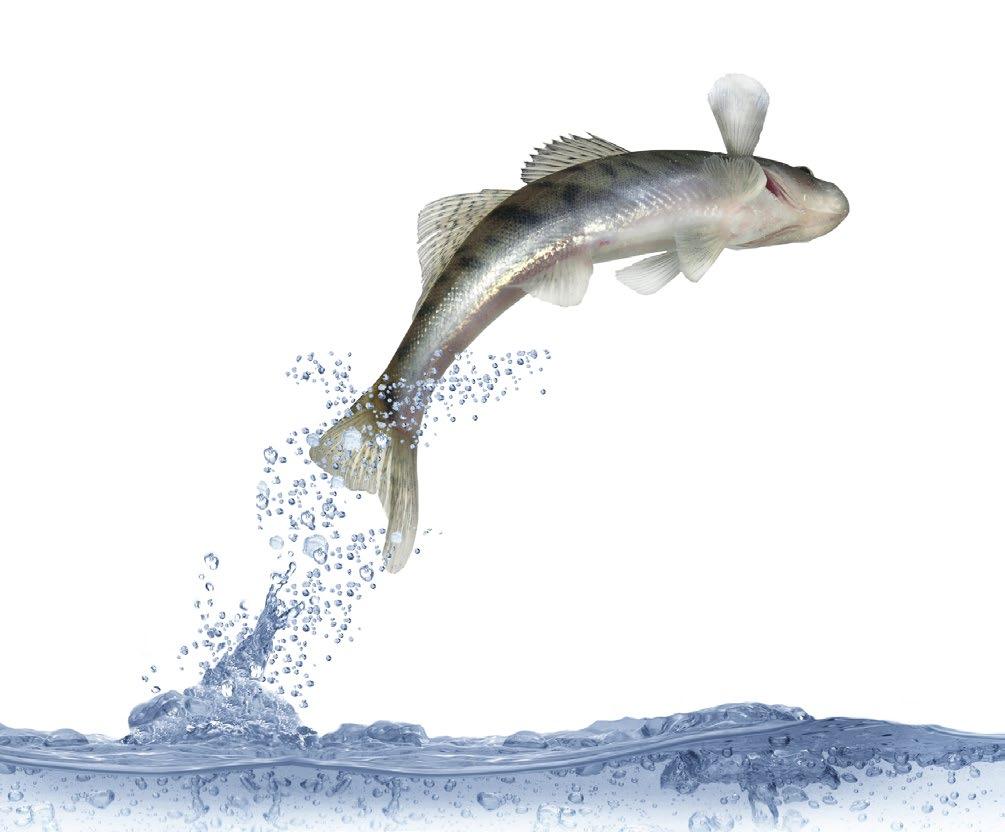
Could 4.0 TECHNOLOGY revolutionize THE FUTURE OF MYCOTOXIN CONTROL in aquaculture?
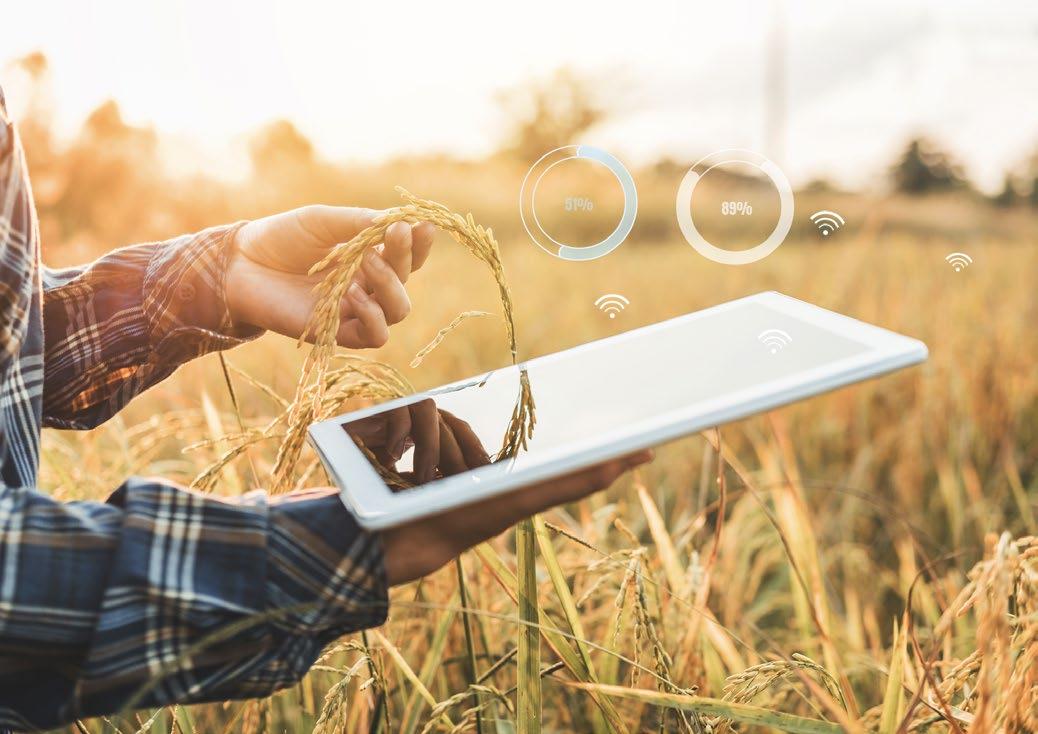
Ercument Genc1 and Evrim Genç Kumtepe2
1Department of Fisheries and Aquaculture Engineering, Faculty of Agriculture, Ankara University, Diskapi, Ankara, Turkiye
2Faculty of Open Education, Anadolu University, Eskisehir, Turkiye
Aquaculture, driven by the increasing global demand for food, is becoming increasingly important. However, mycotoxins commonly found in aquafeed pose a serious risk to the environment and human health.
Mycotoxin contamination threatens sustainable aquaculture practices.
Agricultural 4.0 technologies offering innovative solutions can address these challenges, such as:
Smart Monitoring Systems
Big Data Analysis
Automated Robots
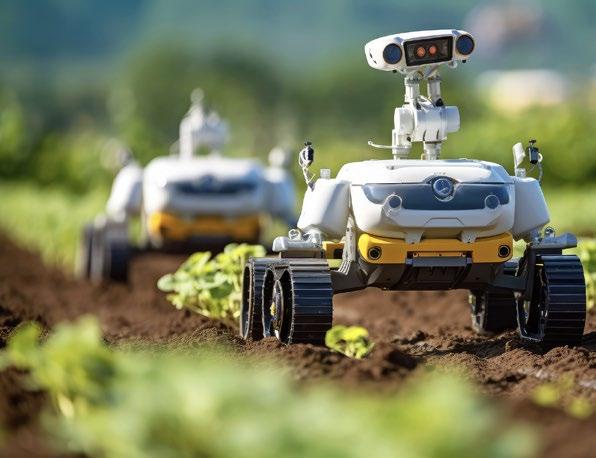
(Stentiford et al., 2017)
Aquaculture (farming aquatic organisms including fish, mollusks, crustaceans, and aquatic plants) offers a vital solution to the global demand for seafood.
As the world’s population continues growing, traditional capture fisheries remain under significant pressure, leading to stagnation or declines in many wild fish populations.
Consequently, aquaculture has emerged not only as an alternative but as an imperative to supplement the global seafood demand.
According to the Food and Agriculture Organization (FAO), aquaculture has been the fastest-growing food production sector for over half a century, currently providing almost 50% of all the fish consumed globally (FAO, 2020)
This rapid growth underscores its critical role in achieving food security and economic development in many regions worldwide.
The quality of the feed used in aquaculture plays a pivotal role in the sustainability and productivity of this sector, affecting not only the growth performance and health of aquatic animals but also the environmental impact of aquaculture practices.
High-quality feed contributes to the economic viability of aquaculture operations through:
Better feed conversion ratios
Lower waste outputs
Improved fish health
(Tacon & Metian, 2015)
However, one of the significant challenges aquaculture feed production is t mycotoxins, toxic compounds produced by certain molds in agricultural commodities such as wheat, and soybeans, which are common ingredients in aquafeed (Kumaretal.,2018).
Mycotoxins pose an important risk to the sustainability and profitability of aquaculture enterprises as they impair fish health leading to:
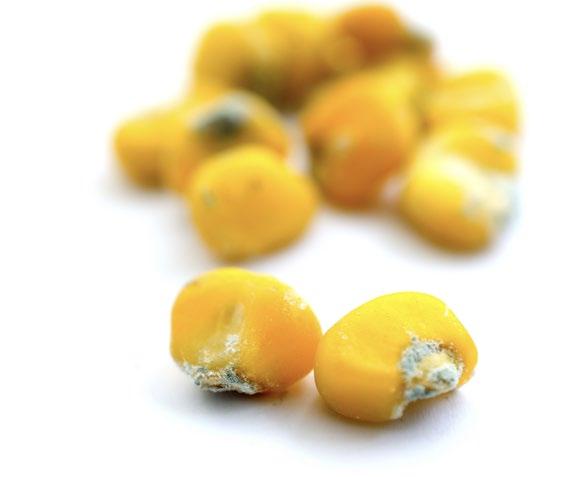
Decreased growth rates
Increased susceptibility to diseases
Death
Addressing the issue of mycotoxin contamination in aquafeed involves a comprehensive understanding of these toxins, the development of robust detection methods, and the implementation of effective mitigation strategies.
These strategies range from selecting mycotoxin-resistant feed ingredients to employing advanced feed processing technologies that reduce toxin levels.
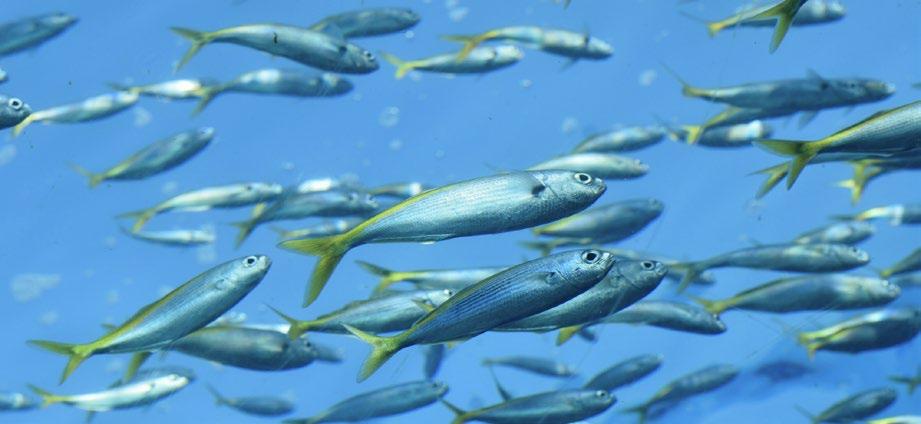
Furthermore, the adoption of Biofloc Technology and the use of specific feed additives, such as mycotoxin binders, have shown promise in managing mycotoxin risks (Nootong et al., 2021).
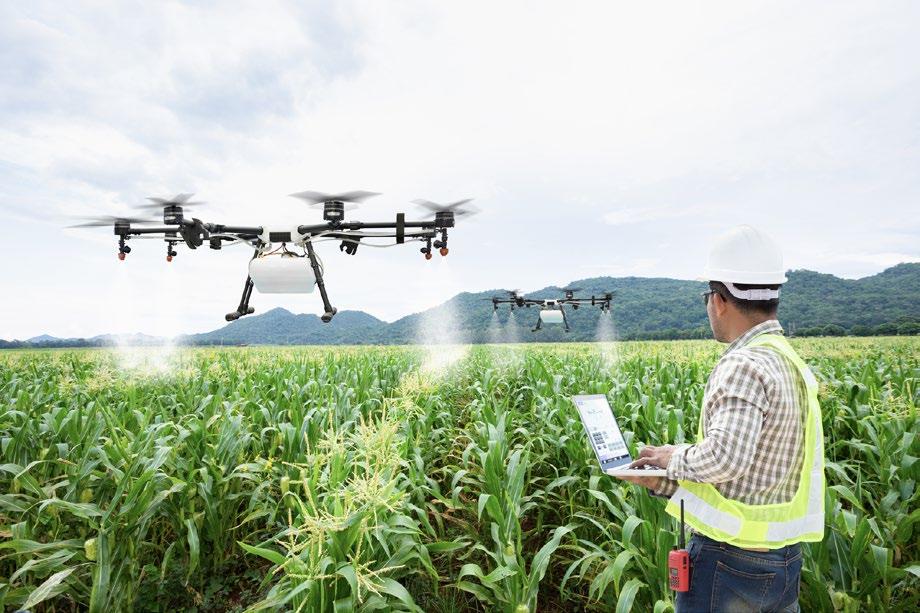
This review delves into multifaceted approaches used to protect fish health against mycotoxin contamination in formulated feeds and aquaculture systems.
It also draws attention to the potential of future 4.0 technologies, such as Smart Monitoring Systems, Big Data Analysis, and Automated Robots, to be utilized for this purpose.
We try to provide information on the future directions of research and development efforts in this critical area to create an opportunity for our valued readers to examine the effects of mycotoxins, discuss current strategies, and explore the potential of research and development activities conducted in this critical field.
Mycotoxins in aquaculture feeds
Mycotoxins are toxic compounds produced by specific molds commonly found in plant-based feed sources.
They cause health issues and economic losses when consumed by aquatic species, such as fish and shrimp, through contaminated feeds.
With the increasing use of plant-based feeds, awareness of mycotoxins in the aquaculture sector is growing. The need for plant-based materials is rising due to the high cost and limited availability of traditional protein sources like fishmeal in aquafeeds.
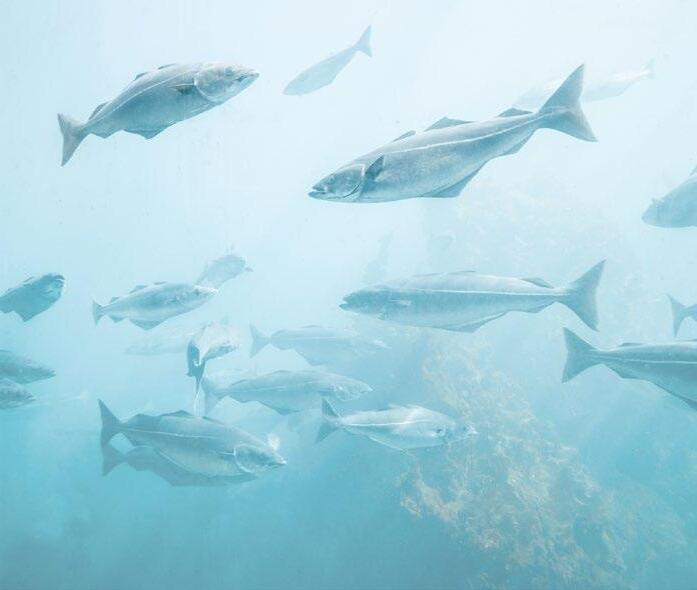
However, the effects of mycotoxins in the aquaculture sector are not easily observable, making their management challenging.
Additionally, there are concerns about the transfer of mycotoxins from feeds to aquatic products and the risk this poses to consumer health, as clear regulations are still lacking. This highlights the need for specific regulations on mycotoxin levels in aquaculture feeds and foods.
This approach should be supported by advancements in mycotoxin detection and mitigation technologies that can help maintain the integrity and safety of aquaculture operations.
Key types of mycotoxins affecting aquaculture and their impacts
Aflatoxins (AF), predominantly produced by Aspergillus species, are among the most dangerous mycotoxins. They are commonly found in maize and peanuts that have been stored under conditions favorable for mold growth.
Aflatoxins are highly toxic to the liver and recognized as potent carcinogens, posing a severe risk to aquatic animal health and human consumers.
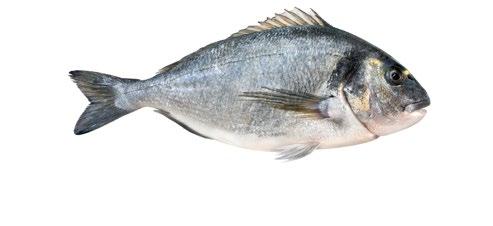
The International Agency for Research on Cancer (IARC, 2020) classifies aflatoxins as Group 1 carcinogens, underscoring their impact.
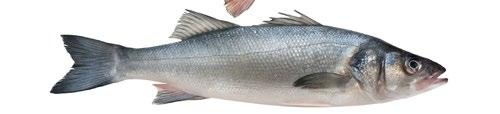
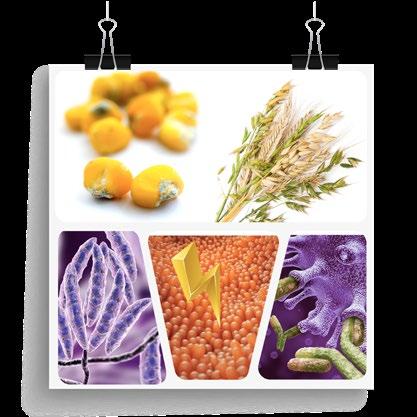
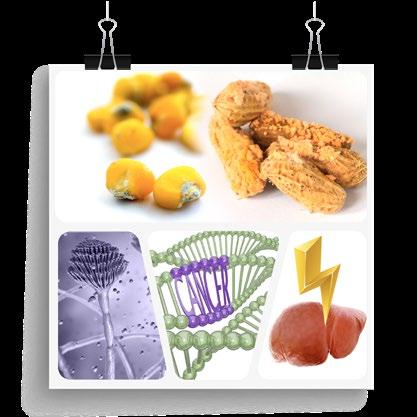
Fumonisins (FUM), predominantly produced by Fusarium species, are common in maize and other cereals.
They can cause severe damage to the gastrointestinal tract and compromise the immune system of aquatic species, impacting their health and reducing farm productivity (FDA 2019; FAO, 2021).
Ocratoxin A (OTA) is mainly produced by Aspergillus and Penicillium species, frequently contaminating cereals and grains.
This mycotoxin is known for its nephrotoxicity, adversely affecting kidney function, and it also possesses carcinogenic properties, which raises significant food safety concerns.
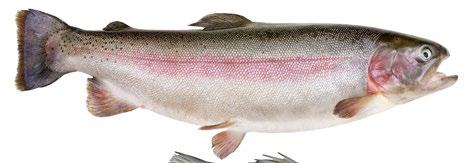
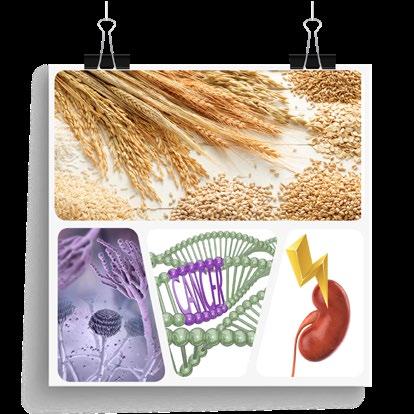
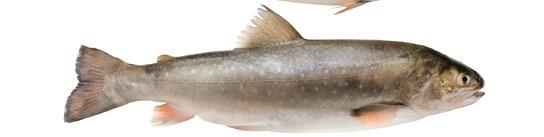
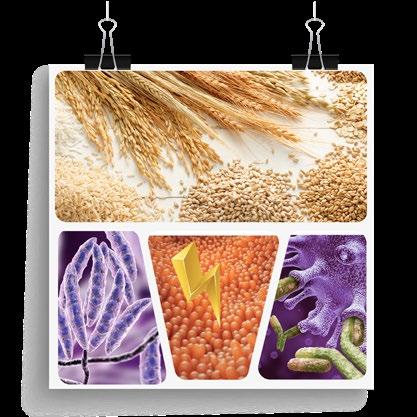
Deoxinivalenol (DON), also known as vomitoxin, is predominantly produced by Fusarium species, and found in infected wheat, barley, and other cereals.
It causes feed refusal and impairs immune function in fish, which can lead to widespread health issues and economic losses in aquaculture operations (EFSA, 2017).
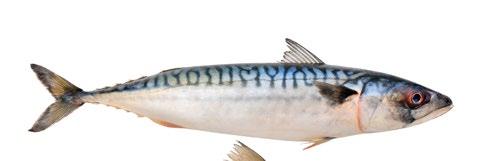
ZEARALENONE
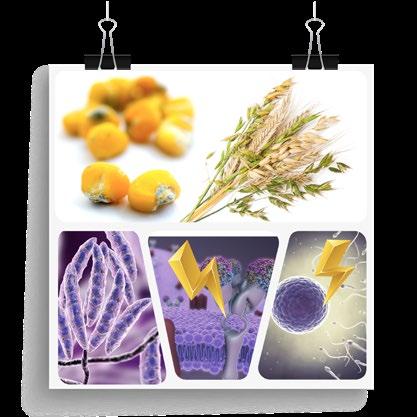
Prevalence of mycotoxins in aquaculture feed
The prevalence of mycotoxins in aquaculture feeds exhibits significant variability across regions, with particularly high incidences observed in areas characterized by hot and humid climates, which are ideal conditions for fungal proliferation.
Studies conducted by Gonçalvesetal.(2020) and Alinezhad et al. (2010; 2011; 2017; 2018) have extensively documented the widespread contamination of aquaculture feed in Asia and Europe.
Zearalenone (ZEN), frequently found in grains and cereals, is produced by Fusarium species, mimicking estrogen hormones and disrupting reproductive and developmental processes in aquatic organisms.
Its presence in feeds can lead to significant reproductive failures, affecting the sustainability of aquaculture operations (WHO, 2018).
These studies underscore a consistent risk across various feed types and ingredients, emphasizing the urgent need for stringent feed management and quality control protocols to mitigate mycotoxin exposure.
The detrimental effects of mycotoxins in aquaculture extend beyond direct impacts on the health of aquatic species to encompass profound economic ramifications, including reduced productivity and heightened mortality rates.
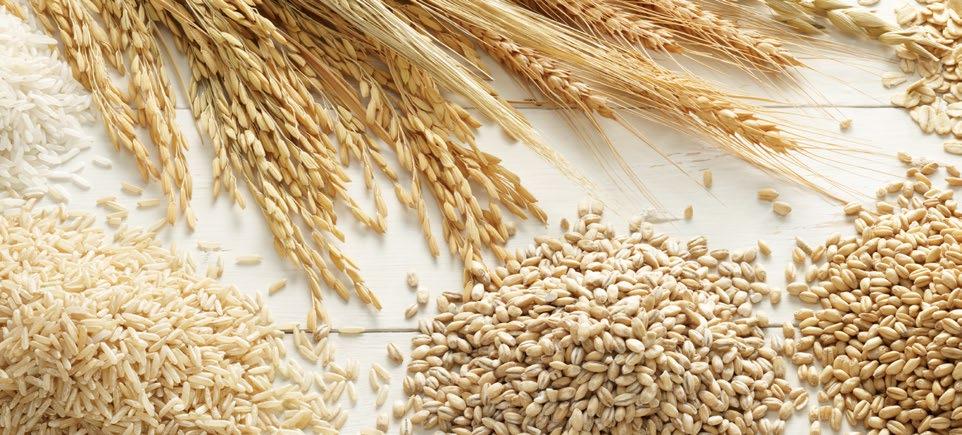
Consequently, there is a pressing imperative to adopt integrated management strategies that encompass the selection of resistant crop varieties, optimization of storage conditions, and incorporation of mycotoxin binders or detoxifiers into feed formulations.
In general, mycotoxins pose a persistent challenge in aquaculture, necessitating ongoing vigilance, research, and innovation in feed management practices to ensure the safety and sustainability of fish production systems.
Challenges in detecting and controlling mycotoxin levels in feed
Detecting and controlling mycotoxin levels in aquaculture feed presents significant challenges, primarily due to the complexity and variability of mycotoxin contamination.
Mycotoxins can occur in various feed components at any stage, from crop cultivation to feed processing and storage, and the heterogeneity in the distribution of mycotoxins within a batch of feed compounds increases the difficulty of effectively detecting them (Gonçalves et al., 2017).
Environmental factors, such as temperature and humidity, significantly influence the occurrence of mycotoxins, varying widely across different geographic regions and seasons, complicating consistent monitoring efforts.
Current practices for mycotoxin detection often rely on sampling methods that may not capture the sporadic nature of mycotoxin contamination (Vasanthi & Bhat, 1998).
Traditional methods, such as chromatography and spectroscopy, while precise, are time-consuming and require sophisticated equipment and skilled personnel (Cheli et al., 2013).
Rapid detection methods, such as immunoassays and biosensors, offer potential improvements but still face challenges regarding sensitivity and specificity (Marasas, 1991; Ellis et al., 2000).
The control of mycotoxins in feed is further complicated by the limited effectiveness of current mitigation strategies.
While strategies such as crop rotation and the use of fungicides can reduce fungal infections and subsequent mycotoxin production, their effectiveness is not absolute (Fegan & Spring, 2007).
Mycotoxin binders and adsorbents in feed show promise but do not eliminate mycotoxins from the feed matrix and can interfere with nutrient absorption (Dawson et al., 2001; Hussein & Brasel, 2001; Alvarado et al. 2017; Zahran et al., 2020; Waithaka Ng’ang’a et al 2022).
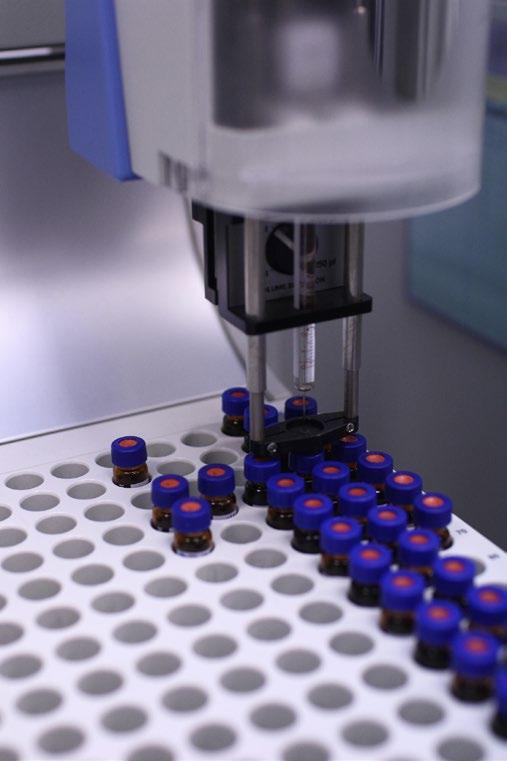
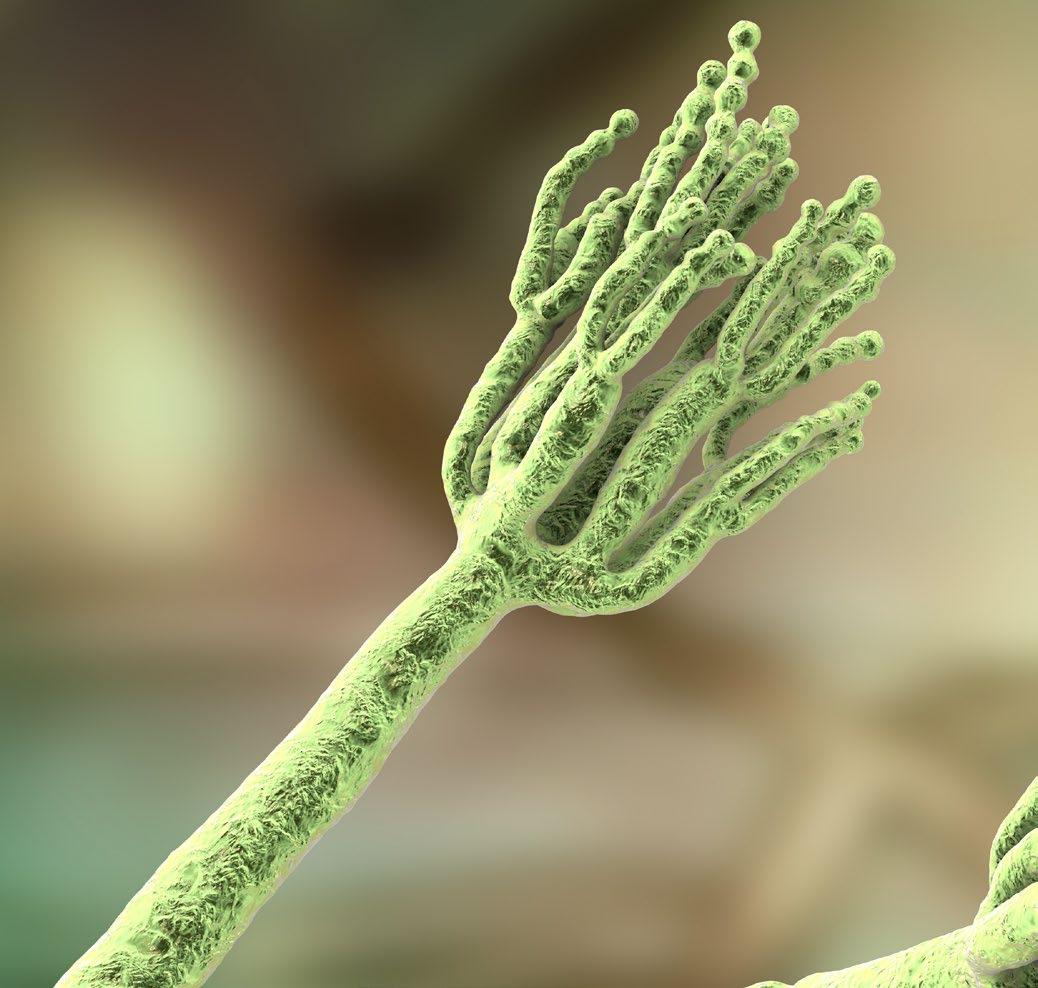
To address these challenges, several avenues for research and innovation are proposed:
Advancements in detection technologies
Exploring emerging technologies for rapid, accurate, and cost-effective mycotoxin detection (Paterson&Lima,2010;2011).
Biocontrol and biodegradation approaches
Investigating biological control measures and microbial biodegradation as promising strategies to counteract mycotoxins directly in feeds (Jestoi,2008;Karlovskyetal.,2016).
Regulatory and policy frameworks
Analyzing the role of enhanced regulatory frameworks and global collaboration in standardizing safe practices for mycotoxin management in aquaculture feeds(EFSA,2004).
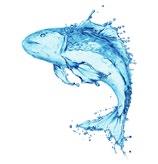
By addressing these objectives, this article aims to provide comprehensive insights into the current state of mycotoxin management in aquaculture feeds and offer guidance on adopting and developing effective strategies to mitigate these risks.
Preventive measures to mitigate mycotoxin risks in aquaculture feed
Aquaculture, as a rapidly growing sector, faces significant challenges in maintaining feed quality, particularly concerning mycotoxin contamination.
Effective mycotoxin management in aquaculture feed is pivotal, beginning with the selection of raw materials and extending through agricultural and storage practices.
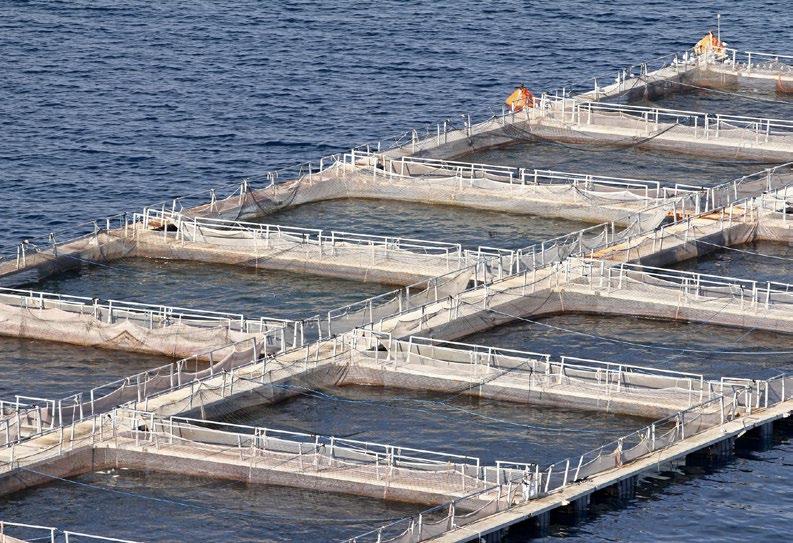
Selection of raw materials
Choosing high-quality, low-mycotoxin ingredients is the first critical step in preventing mycotoxin contamination in aquaculture feeds.
Research emphasizes the necessity of stringent quality control measures right from the procurement stage. Tacon and Metian (2008) highlight the importance of using certified suppliers who adhere to rigorous safety standards to minimize mycotoxin risks.
Agricultural practices
Effective agricultural practices play a crucial role in minimizing the presence of mycotoxins.
Crop rotation, proper fertilization, and irrigation practices, as suggested by FAO guidelines, are fundamental to reducing crop stress and fungal proliferation (FAO, 2021).

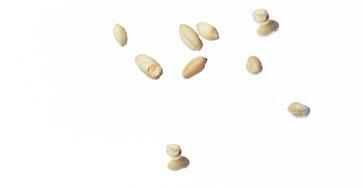
The adoption of integrated pest management (IPM) practices during cultivation can significantly reduce fungal infections and subsequent mycotoxin production (Puri et al., 2019; Chakraborty et al., 2019).
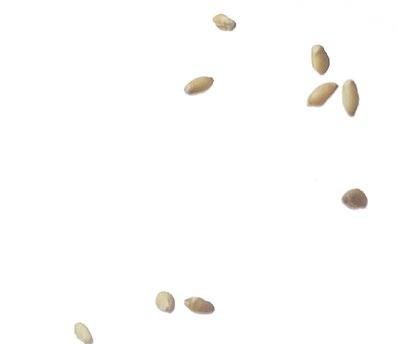
Timely harvesting crops under optimal conditions is essential to prevent the overgrowth of mycotoxin-producing fungi (Olorunfemi et al., 2013).
Storage practices
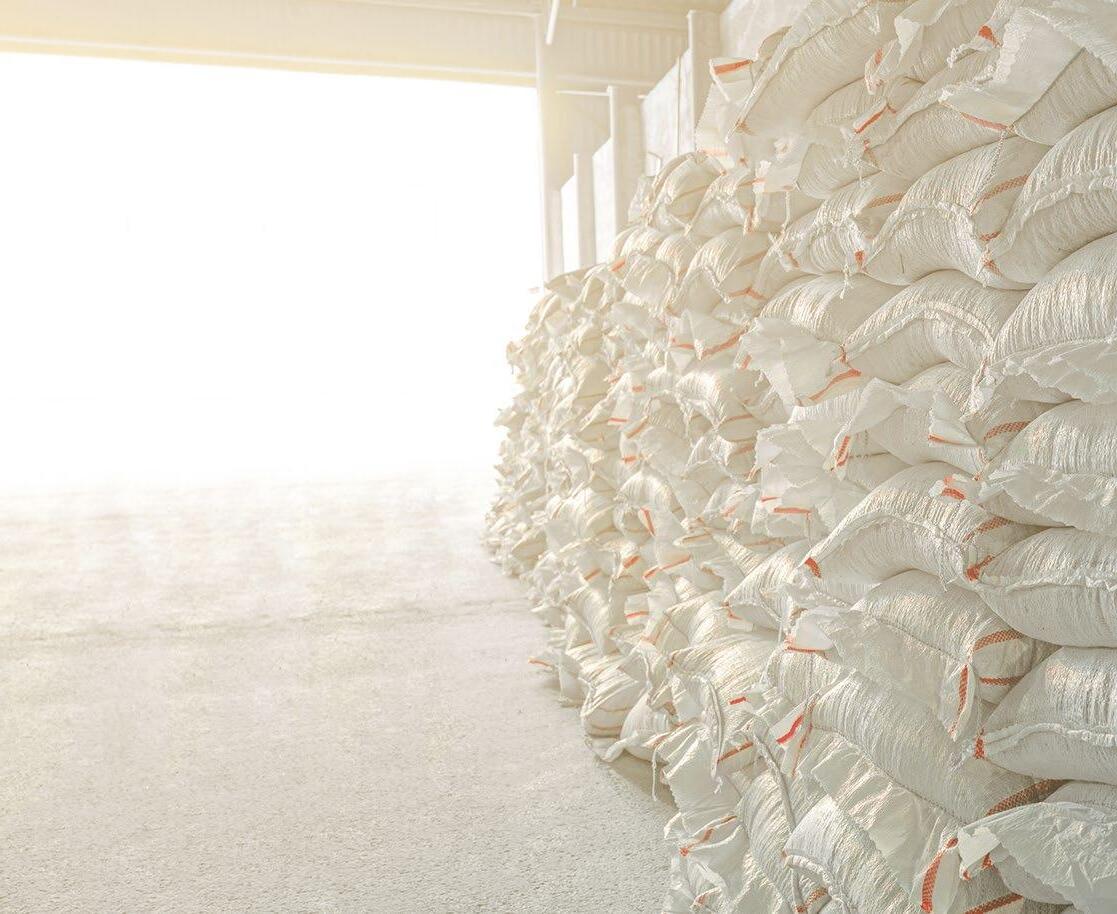
Once the crops are harvested, proper storage conditions are crucial to prevent the growth of mycotoxin-producing fungi.
Studies have shown that maintaining low moisture levels, adequate ventilation, and cool temperatures can drastically reduce mycotoxin levels in stored grains and other feed components (Marijani et al., 2019).
Technologies that allow for hermetic storage can also play a significant role in inhibiting fungal growth and mycotoxin production during storage (Speijers & Speijers, 2004).
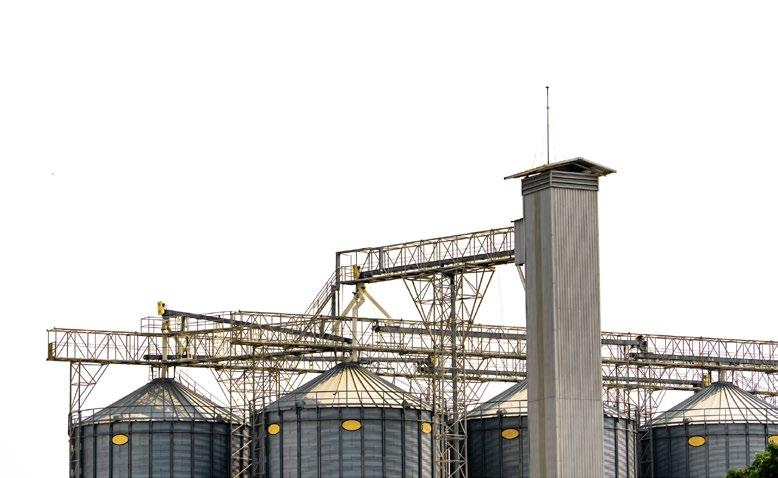
Integrated mycotoxin management
Beyond these preventative measures, integrated mycotoxin management (IMM) approaches are recommended to ensure comprehensive control across all stages of feed production.
This includes regular mycotoxin testing, using reliable and sensitive analytical methods, to monitor and ensure feed safety before it reaches the farm (Zychowski et al., 2013; Woźny et al., 2015).
The incorporation of feed additives that bind or deactivate mycotoxins is also gaining traction. Some products containing adsorbent minerals, clays, yeast derivatives, and other innovative substances have shown promise in reducing mycotoxin bioavailability in feeds (Tuan et al., 2002; Deng et al., 2010).
Technological innovations and feed management
Feed processing technologies
Advanced feed processing technologies such as extrusion and pelleting are critical tools in mitigating mycotoxin contamination in aquaculture feeds.
These processes involve cooking feed at high temperatures and pressures, which can effectively reduce the levels of certain mycotoxins.
For example, the extrusion process has been shown to decrease the levels of mycotoxins like deoxynivalenol and fumonisins due to the mechanical and thermal stresses applied during manufacturing (Tacon et al., 2010)
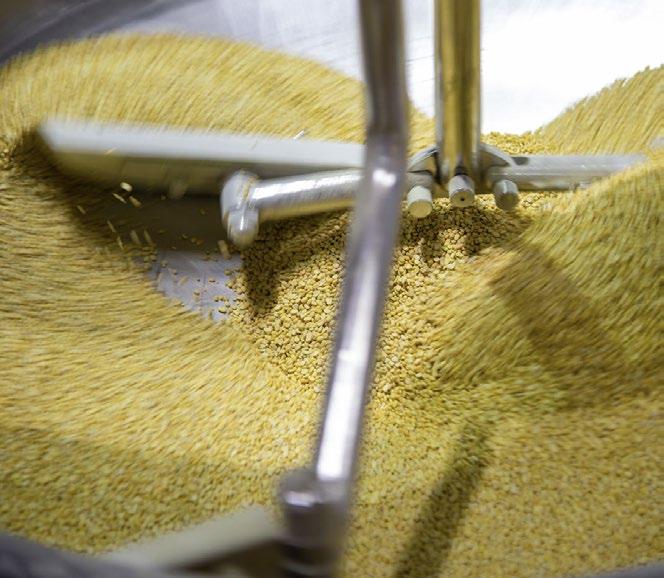
Studies indicate that pelleting can alter the structure of mycotoxins, making them less bioavailable and less toxic to fish (Puri et al., 2019; FAO, 2021).
Feed additives
The use of mycotoxin binders and other feed additives plays a pivotal role in protecting aquaculture species from the adverse effects of mycotoxins.
Mycotoxin binders, such as clays, yeast derivatives, and activated carbons, are added to fish feeds to adsorb and sequester mycotoxins, preventing their absorption in the gut of the fish (Bennett y Klich, 2003; WHO, 2018; FAO, 2021)
Research has demonstrated that these additives do not only bind mycotoxins but can also enhance immune responses in fish, potentially offsetting some of the immunosuppressive effects of mycotoxins (Naylor et al., 2000; El-Sayed & Khalil, 2009)
Antioxidants like vitamin E and selenium have been used in aquafeed to counteract the oxidative stress induced by mycotoxins, further supporting fish health and resilience (Tacon & Metian, 2009).
These combined strategies underscore the importance of integrating multiple approaches to effectively manage mycotoxin risks in aquaculture, ensuring both feed safety and the overall health of aquatic organisms.
Applications of 4.0 Technology in mycotoxin control: Forecasts for the future
The integration of 4.0 Technology holds a transformative potential in managing mycotoxin risks in aquaculture. These technologies enable real-time monitoring of mycotoxin levels and automate interventions to prevent their formation.
For instance, smart monitoring systems equipped with sensors can continuously assess feed storage conditions and automatically adjust humidity and temperature to minimize mycotoxin development (Zhang et al. 2018; Muangprathub et al., 2019; Colizzi et al., 2020; Kim et al., 2020; Kumar et al., 2021; Xu et al., 2022).
Big data analytics play a crucial role by analyzing historical data and current inputs to predict and mitigate future mycotoxin contamination risks (Coble et al., 2018)
Robotic automation enhances precision in feed handling, reducing human error that could lead to mycotoxin contamination. Robots equipped with advanced sensors can:
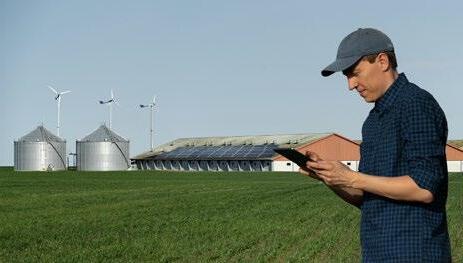
Identify and segregate contaminated feed components quickly
Maintain stringent control over feed storage environments
Perform regular cleaning and maintenance of storage facilities to prevent fungal growth
(Ren et al., 2020)
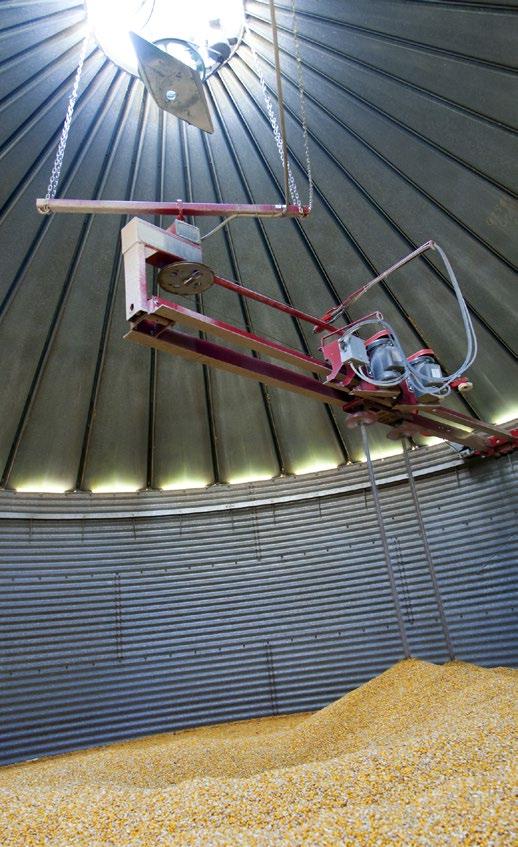
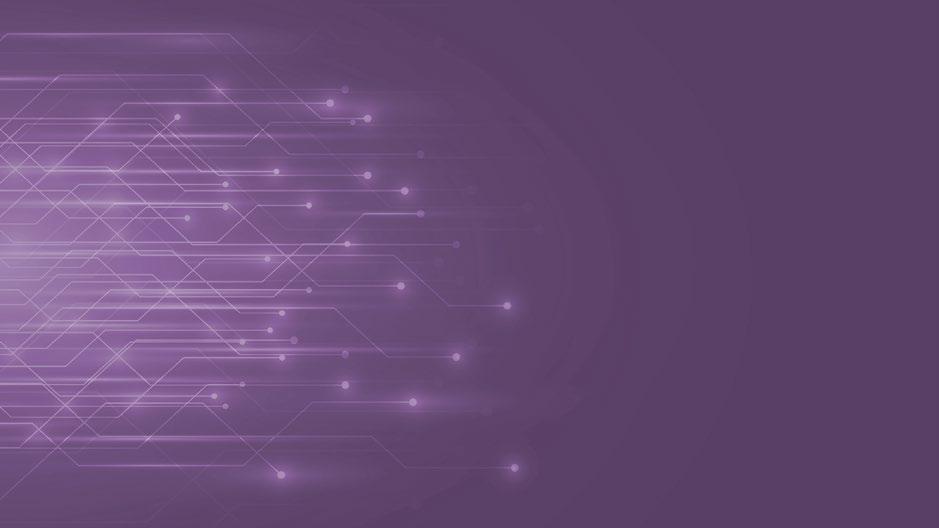
Furthermore, these technologies promote eco-friendly practices by optimizing resource use and reducing waste at various stages of feed production.
Implementing such systems aligns with sustainable agricultural practices, significantly reduce the ecological footprint of aquaculture operations (Balafoutis et al., 2020; Bacenetti et al., 2020; Lieder & Schröter-Schlaack, 2021; Javaid et al., 2022; Papadopoulos et al. 2024)
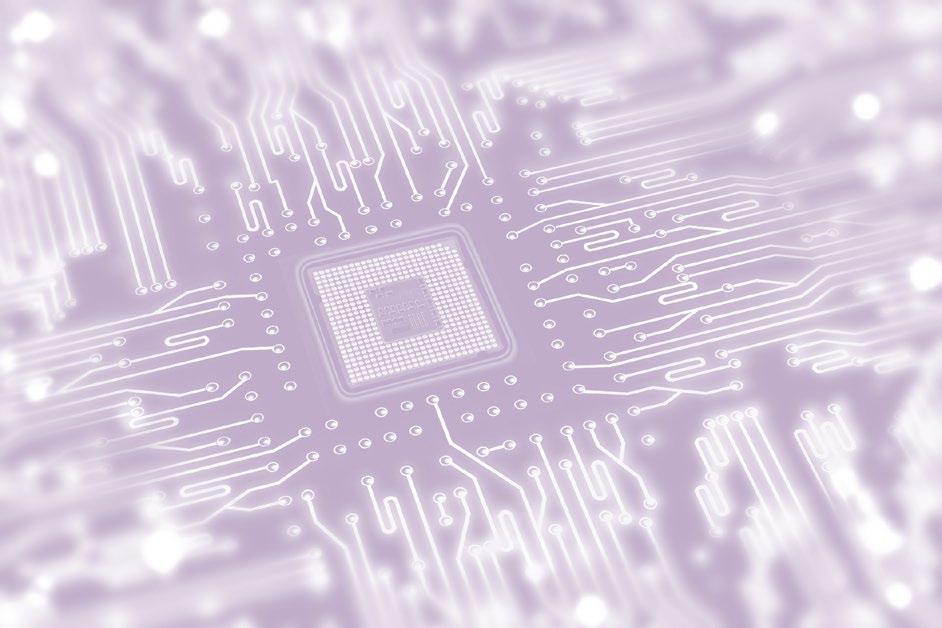
The potential of 4.0 Technology extends beyond immediate mycotoxin management, as it can accelerate broader sustainable transformations in aquaculture. These systems enhance productivity, optimize resource utilization, and mitigate environmental impacts.
For example, smart irrigation and automated fertilization systems optimize water use and nutrient delivery, thereby increasing efficiency while minimizing runoff and pollution (Bwambale et al., 2022)
The sustainability of aquaculture can also benefit from the deployment of intelligent monitoring systems. These technologies: Ensure consistent water quality
Monitor health parameters of aquatic species
Support the ecological balance within aquaculture systems
This proactive environmental management approach can help maintain the long-term viability of aquaculture facilities and enhance resilience against climate variability and other environmental stressors (Kassem et al., 2021; Ruiz-Vanoye et al., 2022).
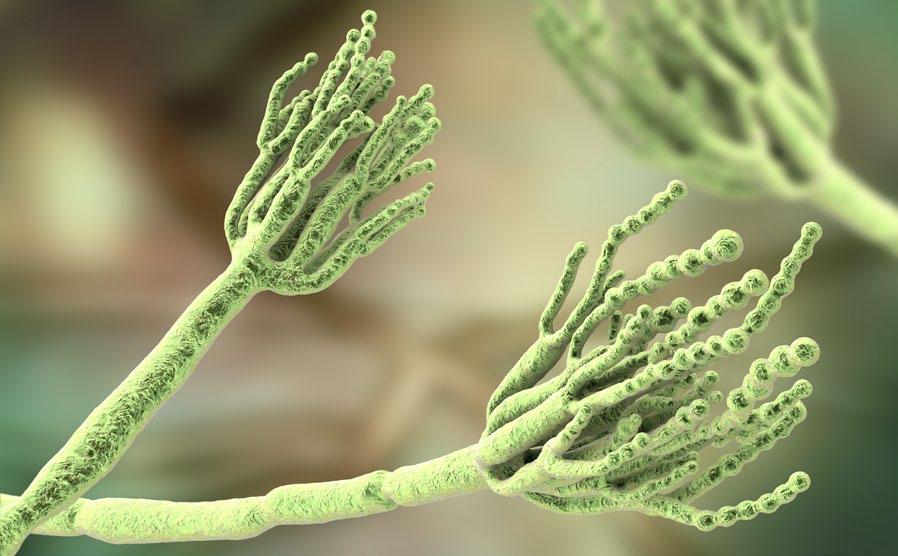
Recommendations for enhancing mycotoxin management
Rigorous feed ingredient selection
Implement strict quality control measures using 4.0 Technology tools such as blockchain and IoT sensors to ensure the use of high-quality, low-mycotoxin raw materials (Klerkx & Rose, 2020)
Establishing strong partnerships with suppliers who adhere to good agricultural practices is crucial (Gonçalves-Nunes et al., 2015; Tacon & Metian, 2009).
Adoption of advanced feed processing techniques
Aquaculture operations should embrace 4.0 Agriculture principles and integrate advanced feed processing techniques, such as extrusion and pelleting augmented by automation and artificial intelligence (Qin et al., 2020)
These techniques have been shown to effectively reduce mycotoxin contamination.
Regular use and evaluation of feed additives
Incorporate proven feed additives into aquaculture diets routinely, utilizing precision agriculture technologies for precise dosage control and evaluate their effectiveness regularly under operational conditions (Marijani et al., 2019).
This approach ensures the additives remain effective against mycotoxins present in the feeds.
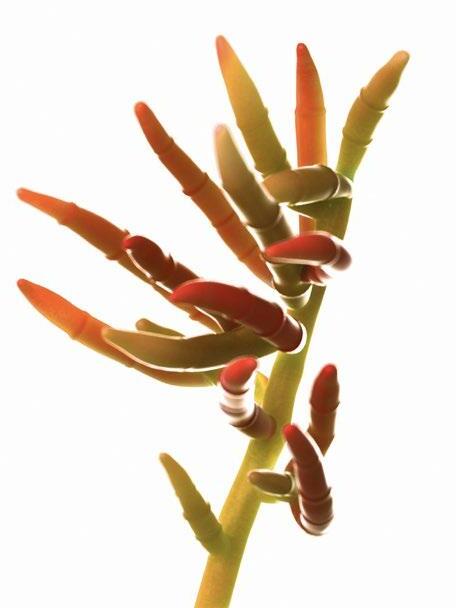
Comprehensive monitoring programs
Establish routine monitoring programs augmented by sensor technology and big data analytics to regularly test feeds and raw materials for mycotoxins (Wolfert et al., 2017).
Utilize reliable, sensitive detection methods integrated with AI algorithms for data analysis (Marijani et al., 2019).
This proactive approach enables timely intervention and management practices to mitigate risks.
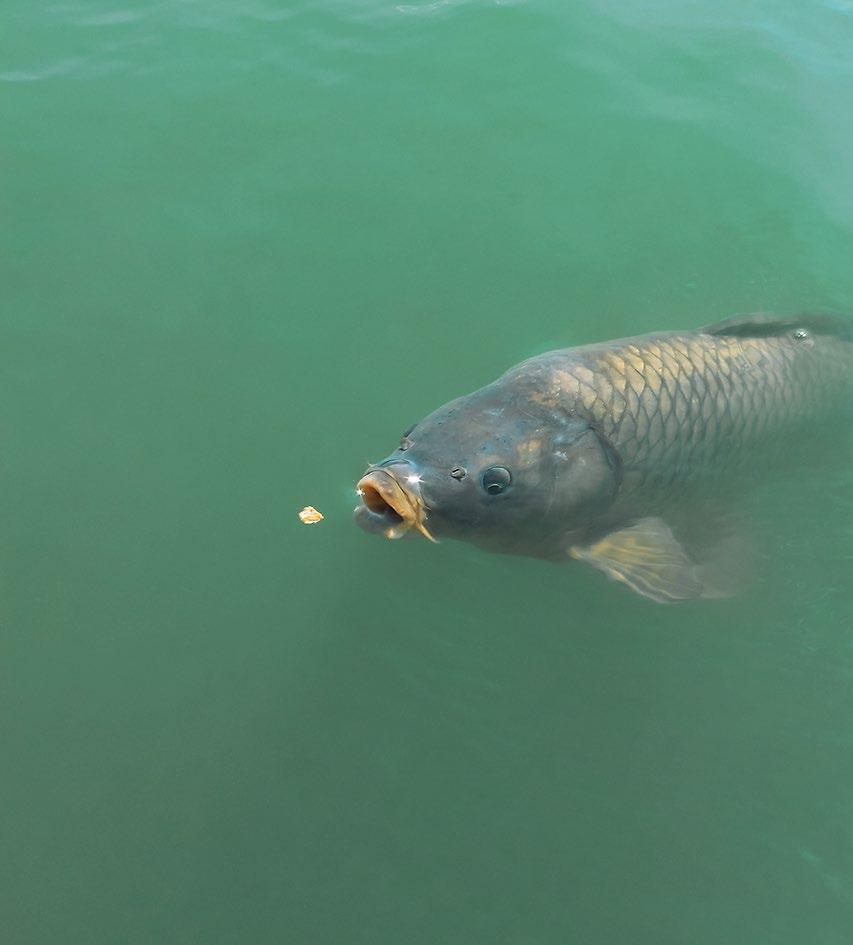
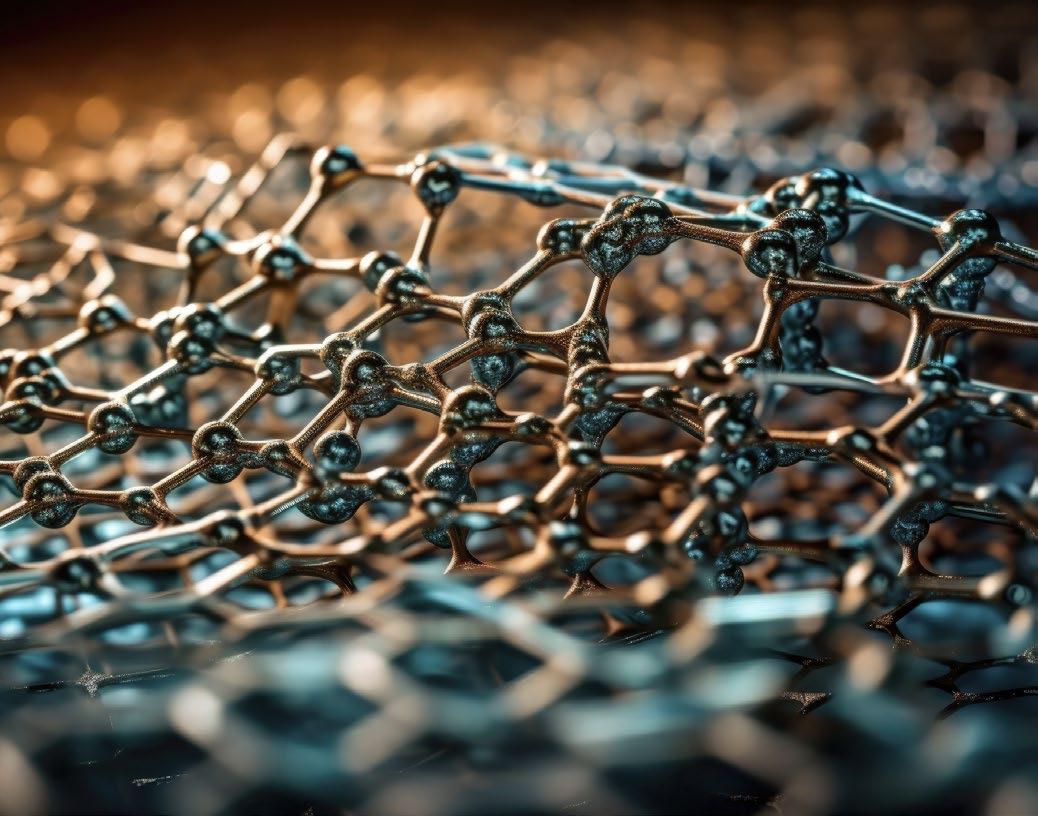
Future research directions
To advance mycotoxin management in aquaculture and integrate it with 4.0 Technology and 4.0 Aquaculture, research efforts should focus on specific areas:
Development of novel mycotoxin binders
The synthesis of optimized mycotoxin binders tailored for 4.0 Aquaculture systems should be explored.
The efficacy of nanomaterials and smart polymers for targeted mycotoxin adsorption and detoxification within aquafeed matrices needs to be investigated.
Machine learning algorithms should be employed to design and optimize binder structures for enhanced performance and specificity (Tacon & Metian, 2009; Klerkx & Rose, 2020).
Selective breeding for resistance
Genomic selection and precision breeding techniques should be utilized to enhance the genetic resilience of aquaculture species against mycotoxin exposure in 4.0 Aquaculture systems.
Data-driven approaches should be implemented to identify genetic markers associated with mycotoxin tolerance traits and accelerate breeding programs for resilient fish and shrimp varieties (Gonçalves et al., 2018; Qin et al., 2020).
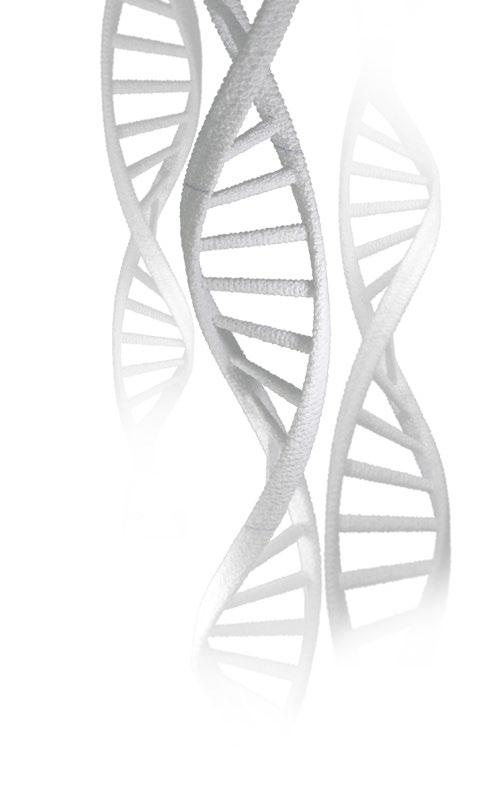

Economic impact assessment
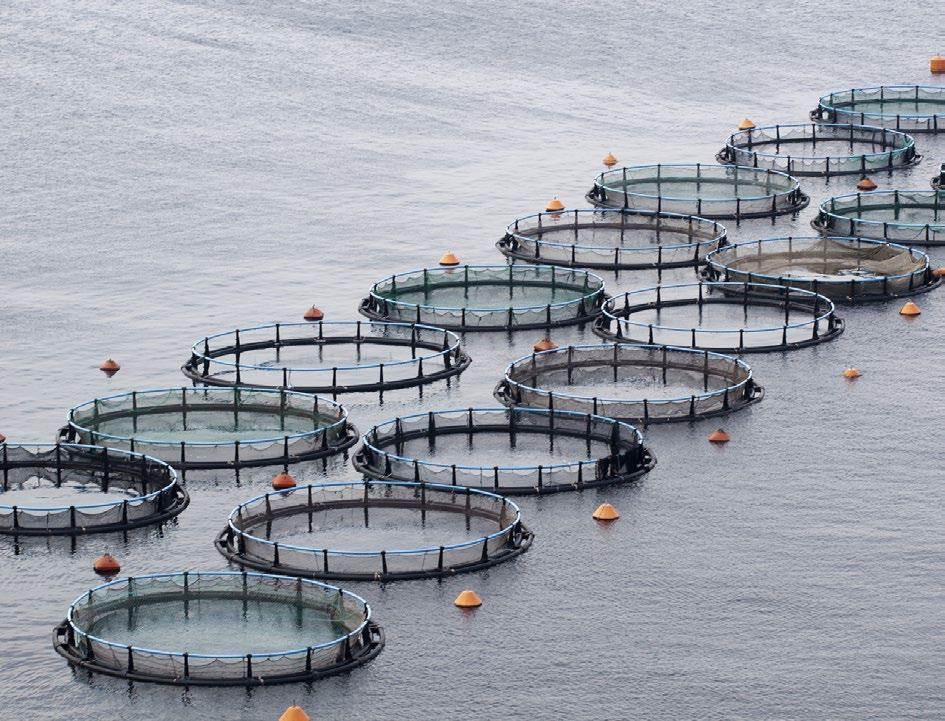
Comprehensive economic evaluations should be conducted to quantify the costs of mycotoxin contamination in Aquaculture 4.0 settings, considering both direct losses and indirect impacts on consumer health.
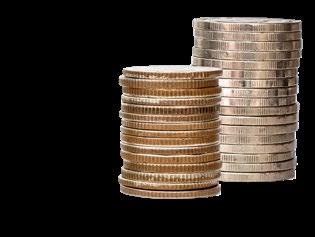
Decision support systems and blockchain technology should be employed to trace mycotoxin contamination along the aquaculture value chain and assess the economic implications of mitigation strategies (Gonçalves et al., 2018; Wolfert et al., 2017).
Effects of climate change
The synergistic effects of climate change and mycotoxin contamination in 4.0 Aquaculture environments need to be investigated.
Predictive modeling and risk assessment frameworks should be employed to anticipate future shifts in mycotoxin prevalence and toxicity under changing climatic conditions.
Adaptive management strategies informed by holistic risk assessments should be developed to mitigate climate-related mycotoxin risks in aquaculture (Manning & Lawrence 2005; Qin et al., 2020)
In addition to technological advancements in aquaculture, societal awareness and education on mycotoxin risks play a crucial role.
Educated individuals, particularly women, often serve as key influencers in rural communities, advocating for the adoption of good agricultural practices in aquaculture.
Societal awareness can serve as a constant reminder to the industry to monitor, combat, and prevent mycotoxin contamination. Moreover, the rapid dissemination of information through social media and industry periodicals facilitates knowledge sharing and awareness-raising on mycotoxin risks in aquaculture.
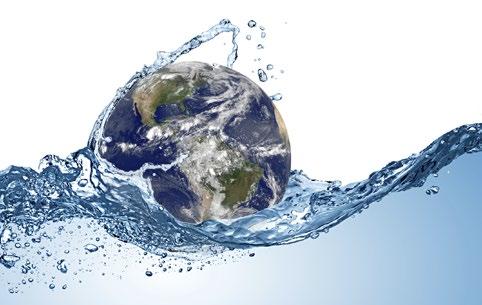
However, it is essential to ensure that information is obtained from reliable sources and expert consultations to avoid misinformation and ensure effective mycotoxin management strategies.
Conclusion and recommendations
Agricultural 4.0 technologies offer a groundbreaking opportunity to combat mycotoxin contamination in aquaculture and promote sustainable farming practices.
These advanced technologies, including smart monitoring systems, big data analytics, and automated robotics, can provide transformative solutions to address mycotoxin risks and enhance the resilience of aquaculture operations.
The potential presence of mycotoxins in aquaculture feeds poses significant threats to the health of aquatic species and consumers alike.
Mycotoxin contamination can jeopardize the immune function, growth, and overall health of aquaculture species, leading to economic losses and potential health risks for consumers.
Therefore, tackling mycotoxin contamination is crucial to ensuring the safety and quality of aquaculture products and protecting consumer health.
In this context, the role of educated individuals, especially women, in society becomes crucial. We express concern that our commentary may be perceived as gender discrimination.
However, throughout human history, women have played a more active and decisive role in shaping dietary preferences compared to men, especially during the transition to settled life and the formation of agricultural communities. When educated, women can activate decision-making mechanisms in improving public health and agricultural product health by directly and indirectly influencing human development, which is fundamentally important in all societies.
Communities composed of educated women and men are often knowledgeable about farmers’ responsibilities and advocate for good agricultural practices in aquaculture.
Their scientific and sectoral understanding of aquaculture products and production processes enables them to play a constant reminder role in monitoring, combating, and preventing mycotoxins, both in aquaculture and in other branches of agriculture.
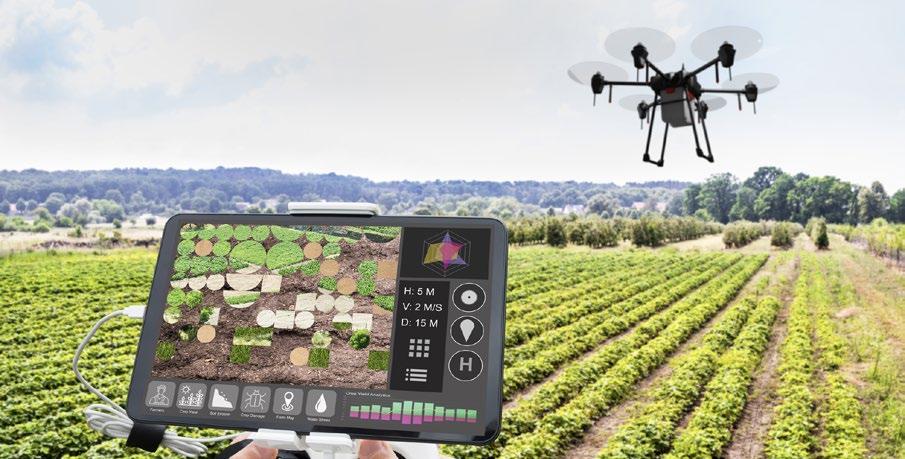
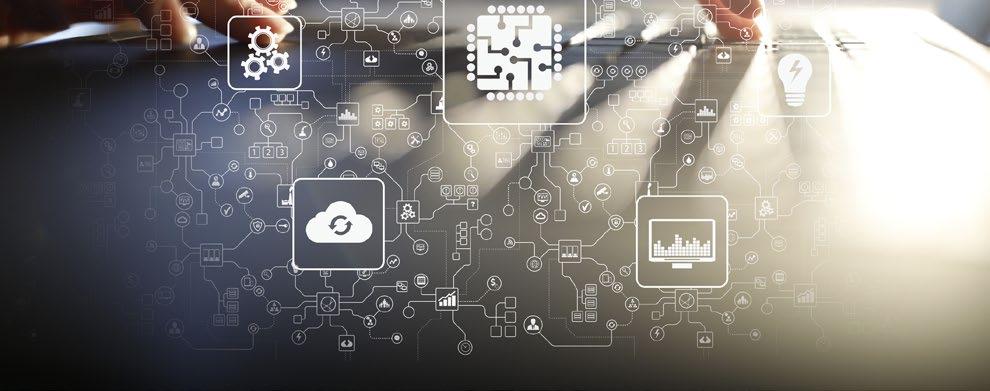
Informed consumers can promote sustainable agricultural practices, disseminate information to society and decision-makers for the widespread adoption of environmentally friendly production technologies, and exert pressure.
In this way, conscious consumers can significantly increase food safety and quality standards in aquaculture by developing both local and global understanding.
In recent years, accurate and scientific information has rapidly spread through industry journals, which serve as the voice of the industry, as well as through social media platforms and the platform where you are reading the article.
This encourages awareness about mycotoxin risks and management strategies.
However, ensuring the accuracy of information is essential. Obtaining information from experts or documented consultations with professionals is crucial to prevent misinformation that could compromise intervention efforts and pose additional risks to aquaculture production and consumer health.
The article titled “World Mycotoxin Forum: Toward a Resilient and Toxin-Free Food System” has been published, summarizing the discussions held at the 14th World Mycotoxin Forum.
The forum took place in Antwerp, Belgium, from October 9-11, 2023. Discussions focused on current challenges facing the food system and strategies to ensure the safety and security of feed and food systems, with particular emphasis on mycotoxin management strategies. While food safety has improved significantly, as noted in the forum, low levels of exposure to contaminants such as aflatoxins can still pose health risks (Dairy Global, 2023).
There is currently no significant activity related to 4.0 Technology applications for mycotoxin monitoring in aquaculture feeds and final products. However, with rapid technological advancements, significant progress in this area is expected in the future.
Advanced technology solutions can offer more sensitive and effective methods for monitoring, detecting, and controlling mycotoxins.
For example, smart sensors and data analytics can enable real-time detection and intervention of mycotoxin contamination in production processes. Additionally, autonomous systems and artificial intelligence-based solutions can provide automatic and continuous monitoring for mycotoxin detection and control.
We foresee that all these technological developments will offer great potential for reducing mycotoxin contamination in agriculture and the aquaculture industry and increasing food safety. Therefore, we believe that advanced technology applications will be a significant tool for mycotoxin monitoring and control in the aquaculture industry in the future.
In conclusion, the adoption of 4.0 Technology, along with efforts in education and knowledge dissemination, will become crucial for effectively managing mycotoxin contamination in aquaculture. By leveraging advanced technologies, increasing awareness, and promoting collaboration among stakeholders, the aquaculture industry can enhance its resilience and ensure the safety and sustainability of its products.
REFERENCES
Alinezhad, S. (2010). Study on the inhibitory effects of native medicinal plants on growth and aflatoxin production by toxigenic Aspergilli isolated from rainbow trout feed (Doctoral dissertation, Islamic Azad University, Science and Research Branch, Tehran, Aquatic Animal Health).
Alinezhad, S., Faridi, M., Falahatkar, B., Nabizadeh, R., & Davoodi, D. (2017). Effects of nanostructured zeolite and aflatoxin B1 in growth performance, immune parameters and pathological conditions of rainbow trout Oncorhynchus mykiss. Fish & shellfish immunology, 70, 648-655.
Alinezhad, S., Irajian, G., & Ghaemmaghami, S. S. (2018). Evaluation of Fungal and Aflatoxin Contamination in Factory-made Feed in Rainbow trout Farms in Damghan City. Iranian Journal of Medical Microbiology, 12(1), 33-42.
Alinezhad, S., Tolouee, M., Kamalzadeh, A., Motalebi, A. A., Nazeri, M., Yasemi, M., ... & Razzaghi-Abyaneh, M. (2011). Mycobiota and aflatoxin B1 contamination of rainbow trout (Oncorhinchus mykiss) feed with emphasis to Aspergillus section Flavi. Iranian Journal of Fisheries, 10(3), 363-374.
Alvarado, A. M., Zamora-Sanabria, R., & Granados-Chinchilla, F. (2017). A focus on aflatoxins in feedstuffs: levels of contamination, prevalence, control strategies, and impacts on animal health. Aflatoxin-Control, Analysis, Detection and Health Risks, 2017, 116-152.
Bacenetti, J., Paleari, L., Tartarini, S., Vesely, F. M., Foi, M., Movedi, E., ... & Confalonieri, R. (2020). May smart technologies reduce the environmental impact of nitrogen fertilization? A case study for paddy rice. Science of the total environment, 715, 136956.
Balafoutis, A. T., Evert, F. K. V., & Fountas, S. (2020). Smart farming technology trends: economic and environmental effects, labor impact, and adoption readiness. Agronomy, 10(5), 743.
Bennett, J. W., & Klich, M. (2003). Mycotoxins. Clinical Microbiology Reviews, 16(3), 497-516.
Bryden, W. L. (2012). Mycotoxin contamination of the feed supply chain: Implications for animal productivity and feed security. Animal Feed Science and Technology, 173(1-2), 134-158.
Bwambale, E., Abagale, F. K., & Anornu, G. K. (2022). Smart irrigation monitoring and control strategies for improving water use efficiency in precision agriculture: A review. Agricultural Water Management, 260, 107324.
Chakraborty, R., Mandal, S. M., Dey, S., & Chakraborty, S. (2019). Management of mycotoxin contamination in agricultural crops: a review. Journal of Plant Protection Research, 59(1), 1-13.
Cheli, F., Battaglia, D., Gallo, A., & Dell’Orto, V. (2013). EU legislation on cereal safety: An update with a focus on mycotoxins. Food Control, 32(2), 414-419.
Coble, K. H., Mishra, A. K., Ferrell, S., & Griffin, T. (2018). Big data in agriculture: A challenge for the future. Applied Economic Perspectives and Policy, 40(1), 79-96.
Colizzi, L., Caivano, D., Ardito, C., Desolda, G., Castrignanò, A., Matera, M., ... & Shi, H. (2020). Introduction to agricultural IoT. In Agricultural internet of things and decision support for precision smart farming (pp. 1-33). Academic Press.
Dairy Global (2023). World Mycotoxin Forum: The path to a resilient and toxin-free food system. Retrieved from https://www.dairyglobal.net/ health-and-nutrition/health/world-mycotoxin-forum-the-path-to-a-resilient-and-toxin-free-food-system/ Dawson, K. A., Evans, J. E. F. F., & Kudupoje, M. A. N. O. J. (2001). Understanding the adsorption characteristics of yeast cell wall preparations associated with mycotoxin binding. CABI. cabidigitallibrary.org
Deng, J., Liao, X., & Wang, L. (2010). Aflatoxin B1, zearalenone and deoxynivalenol in feed ingredients and complete feed from central China. Food Additives & Contaminants: Part B, 3(1), 44-49.
EFSA (European Food Safety Authority) (2004). Opinion of the Scientific Panel on Contaminants in the Food Chain on a request from the European Commission related to mycotoxins in food. EFSA Journal, 2(3), 1-27.
EFSA (European Food Safety Authority) (2017). Risks for animal health related to the presence of zearalenone and its modified forms in feed. EFSA Journal, 15(7), e04854. https://doi.org/10.2903/j.efsa.2017.4854
Ellis, W. O., & Smith, J. P. (2000). Using immunoassays for mycotoxins in animal feed. In E. De Saeger (Ed.), Immunoassays for Residue Analysis: Food Safety (pp. 223-236). Elsevier.
El-Sayed, Y. S., & Khalil, R. H. (2009). Toxicity, biochemical effects and residue of aflatoxin B1 in marine water-reared sea bass (Dicentrarchus labrax L.). Food and chemical toxicology, 47(7), 1606-1609.
FAO (2021). Mycotoxins in cereals and cereal products: Ochratoxin A. Food and Agriculture Organization, FAO Food and Nutrition Paper No. 74. http://www.fao.org/3/y1928e/y1928e00.htm
FAO. (2020). Food and Agriculture Organization,The State of World Fisheries and Aquaculture 2020. Rome, FAO. FDA (2019). U.S. Food and Drug Administration. Guidance for industry: Fumonisin levels in human foods and animal feeds. https://www.fda. gov/regulatory-information/search-fda-guidance-documents/guidance-industry-fumonisin-levels-human-foods-and-animal-feeds Fegan, D., & Spring, P. (2007). Mycotoxins: Occurrence, toxicology, and management strategies. Journal of Livestock Science, 109(1-3), 13-32.
Gonçalves, R. A., Schatzmayr, D., Albalat, A., & Mackenzie, S. (2020). Mycotoxins in aquaculture: Feed and food. Reviews in Aquaculture, 12(1), 145-175.
Gonçalves, R. A., Schatzmayr, D., Hofstetter, U., & Santos, G. A. (2017). Occurrence of mycotoxins in aquaculture: Preliminary overview of Asian and European plant ingredients and finished feeds. World Mycotoxin Journal, 10(2), 183-194.
Gonçalves, R. A., Silva, F. C. D., Boas, L. V., & Lopes, T. S. (2018). Mycotoxins in aquaculture: Occurrence in feeds, toxicity, and mitigation strategies. Aquaculture, 491, 1-10.
Gonçalves-Nunes, E. C., Takahashi, J. A., & Gonçalves, J. L. M. (2015). Mycotoxins in aquaculture: occurrence in feeds, toxicity, and management strategies. Reviews in Aquaculture, 7(3), 218-237.
Hussein, H. S., & Brasel, J. M. (2001). Toxicity, metabolism, and impact of mycotoxins on humans and animals. Toxicology, 167(2), 101-134. International Agency for Research on Cancer. (2020). IARC Monographs on the Evaluation of Carcinogenic Risks to Humans: Some Naturally Occurring Substances: Food Items and Constituents, Heterocyclic Aromatic Amines and Mycotoxins. World Health Organization. https:// publications.iarc.fr/Book-And-Report-Series/Iarc-Monographs-On-The-Identification-Of-Carcinogenic-Hazards-To-Humans/Some-Naturally-Occurring-Substances-Food-Items-And-Constituents-Heterocyclic-Aromatic-Amines-And-Mycotoxins-2012
Javaid, M., Haleem, A., Singh, R. P., & Suman, R. (2022). Enhancing smart farming through the applications of Agriculture 4.0 technologies. International Journal of Intelligent Networks, 3, 150-164.
Jestoi, M. (2008). Emerging Fusarium-mycotoxins fusaproliferin, beauvericin, enniatins, and moniliformin: A review. Critical Reviews in Food Science and Nutrition, 48(1), 21-49.
Karlovsky, P., Suman, M., & Berthiller, F. (2016). A critical review of the role of gut microbiota in the metabolism of mycotoxins. Archives of Toxicology, 90(10), 2309-2323.
Kassem, T., Shahrour, I., El Khattabi, J., & Raslan, A. (2021). Smart and sustainable aquaculture farms. Sustainability, 13(19), 10685.
Kim, W. S., Lee, W. S., & Kim, Y. J. (2020). A review of the applications of the internet of things (IoT) for agricultural automation. Journal of Biosystems Engineering, 45, 385-400.
Klerkx, L., & Rose, D. (2020). Dealing with the game-changing technologies of Agriculture 4.0: How do we manage diversity and responsible innovation in the digital revolution for more sustainable food futures? Global Food Security, 24, 100355.
Kumar, L., Ahlawat, P., Rajput, P., Navsare, R. I., & Singh, P. K. (2021). Internet of things (IOT) for smart precision farming and agricultural systems productivity: A review. IJEAST, 5, 141-146.
Kumar, V., Basu, M. S., & Rajendran, T. P. (2018). Mycotoxin research and mycoflora in some commercially important agricultural commodities. Crop Protection, 57, 21-29.
Lieder, S., & Schröter-Schlaack, C. (2021). Smart farming technologies in arable farming: Towards a holistic assessment of opportunities and risks. Sustainability, 13(12), 6783.
Manning, B. B., & Lawrence, A. L. (2005). Impact of climate change on mycotoxins in aquaculture feed. Aquaculture Research, 36(3), 369-377.
Marasas, W. F. (1991). Fumonisins: History, world-wide occurrence and impact. In K. D. Jr. Vesper & W. L. D. Nesheim (Eds.), Mycotoxins and Animal Foods (pp. 332-335). CRC Press.
Marijani, E., Ding, X., Ma, Y., Liu, D., Wang, J., & Huang, X. (2019). Occurrence and spatial distribution of aflatoxin B1 in feed ingredients and complete feeds from different provinces in China. Animal Nutrition, 5(2), 189-194.
Muangprathub, J., Boonnam, N., Kajornkasirat, S., Lekbangpong, N., Wanichsombat, A., & Nillaor, P. (2019). IoT and agriculture data analysis for smart farm. Computers and electronics in agriculture, 156, 467-474.
Naylor, R. L., Goldburg, R. J., Primavera, J. H., Kautsky, N., Beveridge, M. C., Clay, J., ... & Troell, M. (2000). Effect of aquaculture on world fish supplies. Nature, 405(6790), 1017-1024.
Nootong, K., Pavasupree, S., & Suktham, K. (2021). Biofloc technology: A review of water quality management through biofloc formation. Bioresource Technology, 125, 112-118.
Olorunfemi, O. B., Banwo, O. O., & Adeyeye, S. A. O. (2013). Effects of agricultural practices on mycotoxin contamination of maize in storage. Food Control, 33(1), 215-219.
Papadopoulos, G., Arduini, S., Uyar, H., Psiroukis, V., Kasimati, A., & Fountas, S. (2024). Economic and Environmental Benefits of Digital Agricultural Technologies in Crop Production: A review. Smart Agricultural Technology, 100441.
Paterson, R. R., & Lima, N. (2010). How will climate change affect mycotoxins in food? Food Research International, 43(7), 1902-1914.
Pietsch, C. (2019). Food safety: the risk of mycotoxin contamination in fish. Mycotoxins and Food Safety. IntechOpen
Pietsch, C. (2020). Risk assessment for mycotoxin contamination in fish feeds in Europe. Mycotoxin research, 36(1), 41-62.
Pietsch, C., Müller, G., Mourabit, S., Carnal, S., & Bandara, K. (2020). Occurrence of fungi and fungal toxins in fish feed during storage. Toxins, 12(3), 171.
Puri, M., Sharma, D., & Barman, U. (2019). Management of mycotoxins in food and feed: An economic analysis. Food Control, 95, 302-309.
Qin, Z., Wang, Z., Liu, J., & Ma, X. (2020). Agricultural 4.0: Background, challenges and opportunities. Journal of Integrative Agriculture, 19(1), 1-8.
Ren, G., Lin, T., Ying, Y., Chowdhary, G., & Ting, K. C. (2020). Agricultural robotics research applicable to poultry production: A review. Computers and Electronics in Agriculture, 169, 105216.
Ruiz-Vanoye, J. A., Barrera-Cámara, R. A., Fuentes-Penna, A., Díaz-Parra, O., Trejo-Macotela, F. R., Campero-Jurado, I., ... & Toledo-Navarro, Y. (2022). Internet of Things on sustainable aquaculture system. In AI, Edge and IoT-based Smart Agriculture (pp. 487-503). Academic Press.
Speijers, G. J., & Speijers, M. H. (2004). Combined toxic effects of mycotoxins. Toxicology Letters, 153(1), 91-98.
Stentiford, G. D., Bateman, I. J., & Shields, R. J. (2017). Recirculating aquaculture systems: Challenges and opportunities. Environmental Conservation, 44(1), 24-36.
Tacon, A. G. J., & Metian, M. (2015). Feed matters: Improving the nutritional quality of aquaculture feeds. In D. Allen Davis (Ed.), Advances in aquaculture nutrition (pp. 1-31). Wiley.
Tacon, A. G. J., Hasan, M. R., Allan, G., El-Sayed, A. F., Jackson, A., Kaushik, S. J., ... & Viana, M. T. (2010). Aquaculture feeds: addressing the long-term sustainability of the sector. In Proceedings of the global conference on aquaculture (pp. 193-232).
Tacon, A. G., & Metian, M. (2008). Global overview on the use of fish meal and fish oil in industrially compounded aquafeeds: Trends and future prospects. Aquaculture, 285(1-4), 146-158.
Tacon, A. G., & Metian, M. (2009). Feed additives for sustainable aquaculture. FAO Fisheries and Aquaculture Technical Paper, 547.
Tuan, N. A., Smulders, F. J., & Van Campenhout, L. (2002). Heat stability and in vitro digestibility of casein as influenced by addition of bixin or curcumin. Food Chemistry, 78(2), 159-163.
Vardali, S., Papadouli, C., Rigos, G., Nengas, I., Panagiotaki, P., & Golomazou, E. (2023). Recent advances in mycotoxin determination in fish feed ingredients. Molecules, 28(6), 2519.
Vasanthi, S., & Bhat, R. V. (1998). Mycotoxins in foods—Occurrence, health & economic significance & food control measures. Indian Journal of Medical Research, 108, 212-224.
Waithaka Ng’ang’a, Z., & Niyonshuti, E. (2022). Animal Feeds Mycotoxins and Risk Management. In Mycotoxins and Food Safety-Recent Advances. IntechOpen.
WHO (2018) World Health Organization. Safety evaluation of certain food additives and contaminants: Prepared by the Seventy-sixth meeting of the Joint FAO/WHO Expert Committee on Food Additives (JECFA). WHO Food Additives Series No. 63. https://www.who.int/foodsafety/ publications/chem/zearalenone/en/
Wolfert, S., Ge, L., Verdouw, C., & Bogaardt, M. J. (2017). Big data in smart farming–A review. Agricultural Systems, 153, 69-80.
Woźny, M., Obiedziński, M. W., & Jóźwik, A. (2015). Application of enzyme-linked immunosorbent assay (ELISA) for the assessment of mycotoxins in the feed. Journal of Animal and Plant Sciences, 25(6), 1573-1582.
Xu, J., Gu, B., & Tian, G. (2022). Review of agricultural IoT technology. Artificial Intelligence in Agriculture, 6, 10-22.
Zahran, E., Risha, E., Hamed, M., Ibrahim, T., & Palić, D. (2020). Dietary mycotoxicosis prevention with modified zeolite (Clinoptilolite) feed additive in Nile tilapia (Oreochromis niloticus). Aquaculture, 515, 734562.
Zhang, L., Dabipi, I. K., & Brown Jr, W. L. (2018). Internet of Things applications for agriculture. Internet of things A to Z: technologies and applications, 507-528.
Zychowski, K. E., Hoffmann, A. R., Ly, H. J., Pohlenz, C., & Buentello, A. (2013). Development of a multianalyte LC-MS/MS method for quantitation of mycotoxins in fish feed. Journal of Agricultural and Food Chemistry, 61(30), 7393-7402.