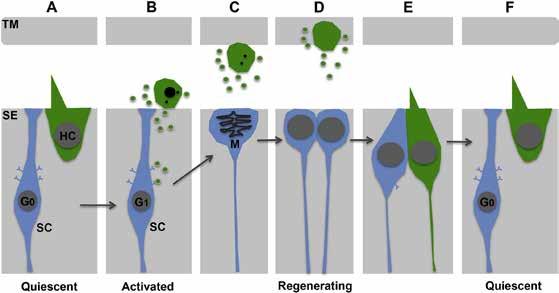
17 minute read
Research Recent Research by Hearing Health Foundation Scientists, Explained
Recent Research by Hearing Health Foundation Scientists, Explained
Looking to Other Sensory Organs to Better Understand Hair Cell Regeneration Scientists have learned an incredible amount since the discovery three decades ago that some animal species are able to regenerate hair cells, but they are still only beginning to understand the genes and signaling involved in tissue regeneration. In a Sept. 25, 2019, article published in the Annual Review of Cell and Developmental Biology, Hearing Restoration Project (HRP) consortium member Tatjana Piotrowski, Ph.D., and colleagues at the Stowers Institute for Medical Research in Missouri summarize the existing literature on hair cell regeneration in the context of sensory cell regeneration more broadly.
Advertisement
While there are many similarities in the development and regeneration of sensory cell types (those involved in vision, for example), recent research has highlighted significant differences. Cross-species comparisons are an important means of identifying these differences, which is why this approach is central to the HRP’s work. Studies suggest, for example, that the limited success that has been experienced in regenerating support cells in mammals may be because these cells are more differentiated in mammals than the analogous cells in zebrafish, a species that can regenerate.
The researchers identify the key question researchers in the field need to answer to significantly advance their efforts: “Which signal or signals trigger hair cell regeneration?” There are numerous hypotheses about the mechanisms through which dying sensory cells signal the need for repair and regeneration, including molecular triggering of the immune system and inflammation. Work in the field thus far indicates that in spite of numerous differences, the molecular response that drives stem cell activation is fundamentally similar. Despite the fact that early efforts to regenerate mammalian hair cells have resulted in modest numbers of hair cells that fail to survive, there is sufficient promise that a better understanding of so-called downstream signaling pathways, which scientists hypothesize are typically epigenetically silenced in postnatal mammals, will prove key to moving beyond these preliminary results.
In a separate study, Piotrowski and colleagues at Stowers and Cardiff University in the U.K. investigated the genes underlying two cell signaling pathways—PCP and Wnt—that are present in both humans and zebrafish and are known to affect the way in which sensory hair cells in the cochlea coordinate their orientations.
Hair cells are “tuned” to respond to different sounds based on pitch or frequency. This is due to a collective property called planar polarization, or the orientation in which the hair cells are laid out. When sound enters the ear, the hair cells change the sound vibrations into an electrical signal that is sent to the brain, allowing us to recognize it. Genetic factors are thought to cause more than 50 percent of all incidents of congenital hearing loss, with many attributed to the misalignment of or damage to hair cells.
Using zebrafish as a proxy, the team systematically switched off these genes in the zebrafish and were able to study the effects on hair cell direction. As published in Nature Communications on Sept. 6, 2019, the results showed that not only could the regularity of the hair cell pattern be destroyed, producing a random hair cell direction, but certain alterations to the genes could lead to the hair cells having circular or spiral patterns. —Christopher Geissler, Ph.D., and Cardiff University
A portion of this was adapted from a press release from Cardiff University on EurekAlert. HRP consortium member Tatjana Piotrowski, Ph.D., is an associate investigator at the Stowers Institute for Medical Research in Missouri.
A Key Molecule Required for the Regeneration of Auditory Hair Cells in the Avian Inner Ear
Hair cells in the cochlea are the only cells in our body specialized to encode the energy in sound waves. As a result, we lose our hearing when hair cells die, which occurs during aging and after exposure to excessive noise or ototoxic drugs. Research indicates that no adult mammals replace their auditory hair cells once they are lost. However, some mature non-mammals regenerate hair cells after damage. Of these animals, the bird’s auditory organ most closely resembles that of humans. Moreover, the regenerated hair cells in birds nearly restore hearing. Thus, birds are particularly important animal subjects for hair cell regeneration research.
It has been known for years that supporting cells— also located in the hearing organ—are the source of the new hair cells in birds, but the molecules that enable supporting cells to regenerate hair cells are not understood. A January 2020 Hearing Research study by Liangcai Wan, M.D., and HRP consortium members Michael Lovett, Ph.D., Mark Warchol, Ph.D., and Jennifer Stone, Ph.D., explored the roles of a molecule called vascular endothelial growth factor (VEGF) in auditory hair cell regeneration in chickens.
The team suspected that VEGF might be required for regeneration because the levels of molecules in the VEGF signaling pathway changed significantly after damage. When they silenced VEGF signaling, they found that hair cell regeneration ceased. Conversely, when they added excess VEGF, they found regeneration was enhanced. The investigators theorize that VEGF, which is abundant in typical hair cells, is released when hair cells die, promoting supporting cells to form new hair cells. This study reveals a novel mechanism controlling hair cell regeneration. Future studies should test if VEGF can enhance hair cell regeneration in adult mammals. —Jennifer Stone, Ph.D.
This figure shows a model for how vascular endothelial growth factor (VEGF) signaling may trigger hair cell regeneration in the auditory organ of birds. VEGFA (green), present in hair cells (A), may be secreted as hair cells die. VEGF may bind its receptors (B) on supporting cells (blue cells), which triggers them to divide (C) and form new hair cells and supporting cells. VEGF may also stimulate some supporting cells to directly convert into hair cells (not shown).
HRP consortium member Jennifer Stone, Ph.D., is the director of research in the department of otolaryngology–head and neck surgery at the Virginia Merrill Bloedel Hearing Research Center at the University of Washington. Learn more about the HRP at hhf.org/hrp and hhf.org/spotlight.
Support our research: hhf.org/donate
E M E R G I N G RESEARCH GRANTS
Size Control of the Inner Ear Through Fluid Pressure
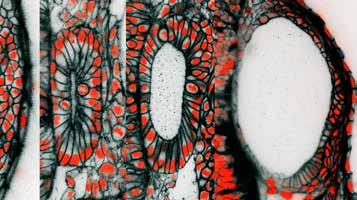
These are cross-sectional pictures from live 3D microscopic images of the developing zebrafish ear. The images are from time-points at 12, 16, 24, and 45 hours after egg fertilization, a span of time during which the ear rudiment grows more than four times in volume. The black coloring is a fluorescent protein targeted to the outer membrane of individual cells. The red is from a fluorescent protein targeted to the nucleus of individual cells where it binds to DNA. At 45 hours post-fertilization, the length of the embryonic ear’s long axis is approximately 1/10th the thickness of a penny.
The inner ear senses sound for hearing and body movement for balance. In the embryo, the rudimentary ear grows from a fluid-filled balloon that is enveloped in a tight layer of cells. In our paper published in the journal eLife on Oct. 1, 2019, we examined how this balloon grows into the more complex ear. Our work helped us formulate a new mathematical theory on how ear growth in animals is controlled.
To do the research, we took advantage of the zebrafish embryo’s transparency by using a high-resolution microscope to take detailed 3D pictures of the inner ear as it grew during the first two days of fish development. We observed that the ear grows dramatically and increases its volume by four times over a period of 24 hours. Most of the increase in size originates from the accumulation of fluid and not because of cell division to increase the tissue mass. This was a very surprising finding since most tissues in development grow by increasing the number or size of cells.
A second observation was that the ear pushed out the neighboring brain structures, and that the ear cells that envelop the balloon appear stretched.
Both of these observations suggested to us that pressure within the developing ear was increasing. We wondered if cells control this pressure directly or if it is just a byproduct of the growth process. We decided to dive deeper.
We designed and built a nanoscale pressure probe small enough to insert into the tiny ear of the fish embryo and sensitive enough to detect the first increase in fluid pressure. To examine if the pressure is monitored and controlled, we popped the ear with needles and observed that the ear would collapse, much like a balloon that has been popped. To our amazement, we watched the popped embryonic ear recover and rapidly catch up in size to the unpopped ear by briefly accelerating its inflation with fluid. Just as an engineer may design a thermostat to maintain the temperature of a room, we began to think
that the developing ear has a way to tell itself when it has reached the correct size by monitoring the internal fluid pressure. We developed a mathematical model of this process.
Next, we began to use this model to predict and test if the pressure also shapes the ear. The mechanical properties of the enveloping cells offer resistance to growth much like a belt worn around the waist. If the belt’s elasticity is locally changed, then growth rates can be controlled locally. This helps explain how the inner ear changes from a spherical shell to an elongated football-like shape.
To summarize, our paper demonstrates how biology does not limit itself to gene regulation and protein activities. Mechanics matter. To function in a 3D world where there is inertia and resistance to growth, an embryo and its developing organs actually control physical forces and collect feedback on these forces to inform regulatory processes. This is yet another reason why animal development is so orchestrated, robust, and precise. Additionally, the crosstalk between mechanical forces and the behavior of cells in the ear is important to understand when investigating hearing and balance diseases where inner ear fluid pressure is out of control, such as Pendred syndrome and Ménière’s disease. —Kishore Mosaliganti, Ph.D., and Ian Swinburne, Ph.D.
Ian Swinburne, Ph.D., is a postdoctoral researcher in the department of systems biology at Harvard Medical School, Massachusetts, where his colleagues included Kishore Mosaliganti, Ph.D. Swinburne is a 2013, 2018, and 2019 Emerging Research Grants (ERG) recipient.
Changes in the Tiny Vessels of the Inner Ear’s Balance Organ Reveal Links to Ménière’s Disease
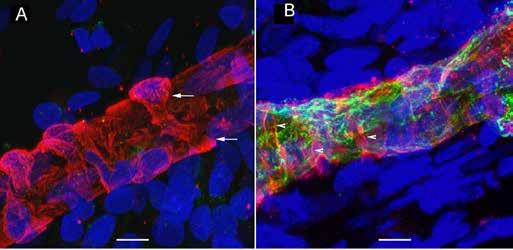
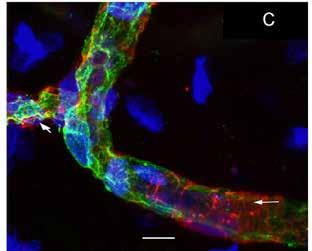
(A) Uniformly thick vessels (red) in a vestibular schwannoma utricle with arrows pointing to a wrapping pericyte cell. (B) In a thick vessel from a Ménière’s patient, there is disorganization of the pericyte processes (white arrows) and evidence of degeneration of the vascular endothelial cells and thin areas of the vessel wall (green). (C) This zoomed-out image shows constriction in the blood vessels (left arrow).
The integrity and permeability of the blood labyrinthine barrier (BLB) in the inner ear is important to maintain an adequate blood supply and to control the passage of fluids, molecules, and ions. Identifying the cellular and structural components of the BLB is critical to understanding inner ear microvasculature (micro vessels) and designing the efficient delivery of therapeutics across the BLB, potentially to treat hearing and balance disorders such as Ménière’s disease.
My team and I used fluorescence microscopy to study the microvasculature in the utricular macula, which detects the body’s linear movement, of patients who had undergone surgery for Ménière’s disease or vestibular schwannoma. As published in Frontiers in Cellular Neuroscience on Oct. 4, 2019, we found a significantly decreased number of junctions, total vessel length, and average vessel length in the microvasculature in Ménière’s disease specimens compared with vestibular schwannoma and control specimens.
The vessels in Ménière’s specimens appeared disorganized with abnormal, uneven, or constricted shapes, atypical branching, and decreased coverage and thinning, leaving vascular endothelial cells (VECs) exposed and unprotected. Our prior research had shown that in Ménière’s disease, VECs are damaged and that they contain oxidative stress markers. Our new study underscores possible mechanisms behind BLB disruption in Ménière’s and the subsequent signs of edema (excess fluid), which disrupts the homeostasis of the hearing and balance structures. The report indicates that interventions aimed at preventing damage to the microvasculature may help stop the progression of damage to the vestibular system, restoring balance and preventing vertigo spells. It could be that decreasing vessel constriction and BLB leakage will help prevent chronic damage to balance structures; this may help explain how steroids administered to Ménière’s patients provide temporary relief from dizziness symptoms.
The paper also shows that human inner ear tissue can be used to compare and contrast findings in animal models to design better therapies for vestibular and auditory disorders. We hope that the deeper understanding of the anatomy of the BLB and its changes during disease will enable the development of noninvasive delivery strategies for treating hearing and balance disorders. —Gail Ishiyama, M.D.
Gail Ishiyama, M.D., a 2016 and 2018 ERG recipient, is a clinician-scientist who is a neurology associate professor at UCLA’s David Geffen School of Medicine.
E M E R G I N G RESEARCH GRANTS
Children’s Working Memory and Phonological Awareness Benefit From Hearing Earlier
Literacy—the ability to read and write—is key for a child’s success in school. This ability requires phonological awareness, an understanding of a language’s sounds, and how to combine sounds into words. Children who are deaf or hard of hearing often experience difficulties with literacy given their limited access to sounds and the resulting poorer phonological awareness skills. In the September 2019 issue of Frontiers in Psychology, 2017 Emerging Research Grants scientist Christina Reuterskiöld, Ph.D., and team detail their study of the relationship among rhyme awareness (the first phonological skill children develop), vocabulary size, working memory, and linguistic characteristics of words in children with typical hearing and children with cochlear implants.
Studies have shown that cochlear implants improve speech and language skills in children with hearing loss, but the researchers were more specifically interested in these children’s phonological awareness skills. They tested this by presenting the children with groups of words and asking them to identify the one that did not rhyme with the others. In contrast to previous studies on typically developing children, they found that in children with cochlear implants, it made little difference if a target word belonged to a group of many rhyming words or few rhyming words, or whether a child’s vocabulary was large or small.
Instead, it was working memory that proved most important. Working memory allows individuals to temporarily store or process information. The group found that there were no significant differences in working memory skills between children with typical hearing and children with cochlear implants with similar hearing experience. (These children had been implanted at an early age and were slightly older than the typical hearing children in the study.)
The authors caution that their findings must be interpreted in light of the small number of children (six subjects) with cochlear implants who participated in the study. Nevertheless, this research suggests a potential association between hearing experience and working memory skills, further underscoring the importance of early treatment for hearing loss. —Christopher Geissler, Ph.D.
Christina Reuterskiöld, Ph.D., is an associate professor of communicative sciences and disorders at New York University’s Steinhardt School of Culture, Education, and Human Development. Her co-principal investigator was Katrien Vermeire, Ph.D., an assistant professor of communication sciences and disorders at Long Island University, New York. Reuterskiöld’s 2017 ERG grant was generously funded by the General Grand Chapter Royal Arch Masons International.
Pinpointing How the Temporal Processing of Nerve Cell Signals Is Important in Hearing
As many as 10 percent of preschool children exhibit some form of developmental speech processing disorder that cannot be attributed to known causes, such as hearing loss or neurological problems. Such listening disorders are accompanied by typical hearing, as determined by traditional hearing tests that assess inner ear function, indicating that these processing deficits are located within the brain. Studies of central auditory processing disorders (CAPD) have identified degraded timing of midbrain activity as a predictor of listening difficulties in adults and children, but the source of dysfunction is unresolved.
Genetically engineered knockout mouse models in which a specific gene is knocked out, or removed, can be used to discover how the processing of timing information may be altered in the developing auditory system and, as a result, how problems with temporal processing impacts listening in humans.
Our mouse model involves the disruption of cholinergic signaling. Nerve cells that are cholinergic use the neurotransmitter acetylcholine to send messages (in contrast to adrenergic nerve cells that use epinephrine or related substances to transmit signals). The CHRNA7 gene, which affects the function of acetylcholine, is considered to be a primary candidate gene causing a wide spectrum of neurodevelopmental disorders, including autism spectrum disorder. Genome-wide studies have found that CHRNA7 is one of a few genes associated with reading disability and specific language impairment, supporting the hypothesis that CHRNA7 is an important gene for the development of language.
In our study published in the Journal of Neurophysiology in July 2019, we examined the temporal processing of knockout mice whose cholinergic signaling is disrupted compared with wild-type mouse controls. We found degraded, abnormal timing of neural activity in the knockout mice at the level of the auditory brainstem and midbrain. These findings underscore the importance of cholinergic signaling in types of neurodevelopmental and auditory processing disorders.
Understanding the contribution of cholinergic signaling to auditory processing can help us increase the understanding of language impairment and assist in the development or refinement of noninvasive diagnostic tests, pharmacological treatments, and behavioral therapeutic strategies. —Richard A. Felix II, Ph.D.
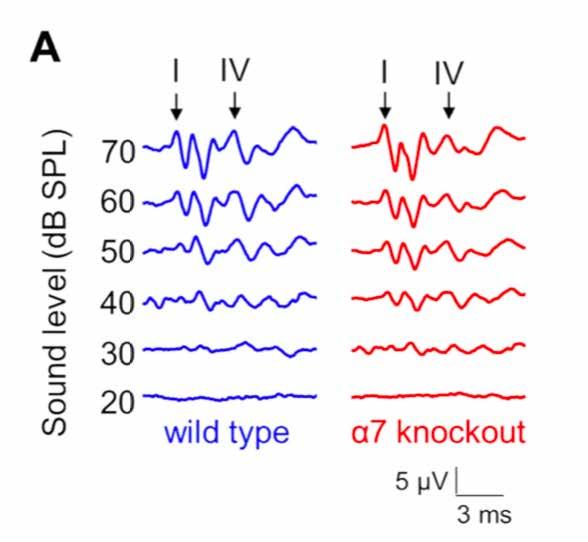
Genetically modified “knockout” mice (red, right) show differences in the timing of auditory brainstem responses compared with wild-type (blue, left) mice.
Richard A. Felix II, Ph.D., is a postdoctoral researcher in the Hearing and Communications Lab at Washington State University Vancouver. His 2016 ERG grant was generously funded by the General Grand Chapter Royal Masons International.
E M E R G I N G RESEARCH GRANTS
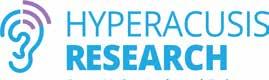
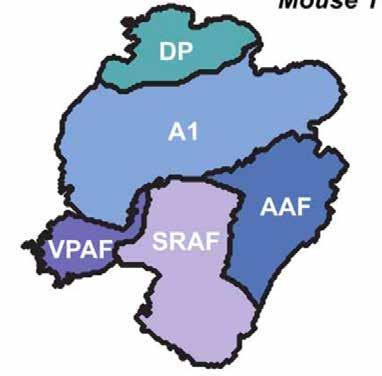
The five fields of the mouse auditory cortex: the primary auditory cortex (A1), anterior auditory field (AAF), suprarhinal auditory field (SRAF), ventral posterior auditory field (VPAF), and dorsal posterior (DP).
Unraveling Sound Frequency Organization in the Mouse Auditory Cortex
Tonotopy refers to the spatial arrangement of where sound is perceived, transmitted, or received. “Tono” is from the Greek for frequency, and “topos,” place. There is tonotopy of the cochlea, in that higher frequencies stimulate neurons in the base of the cochlea, while lower frequencies stimulate neurons in the apex, or center. The orientation and placement of sensory hair cell bundles that react to sound waves and transmit that information to the brain are also examples of tonotopy.
It is known that different regions of the brain respond to varying frequencies. But while the mouse is among the most popular model systems for studies of cortical sound processing and brain plasticity, fundamental aspects of core and higher order cortical field organization have been unclear. The bestfrequency organization (the frequency at which a neuron is most responsive) and naming scheme for additional higher order fields remain a matter of debate. In a study published in Cerebral Cortex on Oct. 30, 2019, a group of researchers, including 2017 ERG scientist Jennifer Resnik, Ph.D., and past ERG reviewer Daniel B. Polley, Ph.D., add to the knowledge of the tonotopy of the mouse auditory cortex.
Using special imaging techniques on transgenic mice to gather cellular-level, high-resolution data for mapping and analysis, the team identified five fields. One is a previously unidentified area of the mouse auditory cortex at the ventral posterior extreme of the auditory cortex. The imaging data suggests that in the mouse there is no ultrasonic field (which the researchers argue is a misnomer anyway in the mouse as it is an anthropomorphic term) or a secondary auditory cortex (as in other animals, including humans). The team makes recommendations for tweaking the nomenclature of brain regions for better uniformity with other animal models and identifying brain areas for future investigation. —Yishane Lee
Jennifer Resnik, Ph.D., is a postdoctoral fellow in the Polley Lab, part of the Eaton Peabody Laboratories, Massachusetts Eye and Ear/Harvard Medical School. Her 2017 ERG grant was generously funded by Hyperacusis Research Ltd. Daniel B. Polley, Ph.D., has been a past reviewer of ERG grants.