Source: email
“Thermalization” of sunlight
Tom Shula
November 28, 2024
I found the exchange between David Burton and Will Happer in this thread most interesting. This predates the works initiated by van Wijngaarden and Happer by 5 years, and Markus Ott and I have studied this process in detail. I strongly recommend the reader watch Markus Ott’s video Convection and Thermalization Kills the Greenhouse Effect and read our essay The "Missing Link" in the Greenhouse Effect , recently submitted for posting on this site.
Before delving into that, I digress briefly on the concept of the photon because it plays a major role and is one source of many misconceptions in this arena. I submitted an earlier post on this topic but in the context of some of Burton’s and Happer’s comments I’ll go a bit further. There is no wave function for the position of a photon. While it is one of the “particles” in the Standard Model of Particle Physics, the descriptions I have found relative to Quantum Field Theory and Quantum Electrodynamics indicate it is “a quantized excitation of the electromagnetic field” which is “governed by Maxwell’s equations and photons correspond to specific states of these fields." It is not a particle in the classical sense. It only manifests itself in interactions with matter. In the case of emission and absorption, the quantization arises because of the allowed energy states of the matter particle(s). While it is convenient in some respects to think of photons as particles/packets of radiant energy with trajectories, they are not. Quantization is a property of matter, not the electromagnetic field. The photon is a useful concept as long as we understand what it is and what it is not.
Proceeding, I will focus on the role and mechanisms of thermalization as they apply to outward energy transport in the atmosphere referring to radiation/photons only as necessary. A discussion of radiative transfer theory as applied in contemporary climate science will be another topic in CDGG2024 Box 1 Section C3.
One misconception I have noted in various discussions is the “thermalization of sunlight.” The sunlight is not thermalized, at least not directly. Thermalization generally refers to the redistribution of energy amongst an ensemble of particles such that they reach a distribution approaching thermodynamic equilibrium. In the case of our planet, sunlight heats the surface. The heat energy can be transferred from the surface to the atmosphere in two ways.
The first is via conduction when air molecules collide with the warmer earth surface and increase their energy via transfer of heat increasing their velocity. At STP, about 50 kg of molecules/sq-meter/sec collide with the surface. The energy transferred will be proportional to the difference in temperature.
The second mechanism for transfer of heat is via the thermal radiation from the surface which is absorbed by IR active molecules. This absorption is effectively complete within ~ 10 meters above the surface. The balance between these two processes will change with varying levels of solar insolation.
We now have an ensemble of air molecules near the surface, some heated via conduction and others in excited states from absorption of IR radiation.
The troposphere is in a continuous state of attempting to reach equilibrium (but it never gets there.) The molecules at a given altitude will all have a
distribution of kinetic energy following a Maxwell-Boltzmann distribution according to the local temperature. The excitation and de-excitation (thermalization) of the IR active molecules are driven not by absorption and emission, but by collisions with other molecules. It is replenished by, but independent of, the energy received from conduction and absorbed radiation at the surface.
Referring now to the Happer/Burton discussion, some correction of the numbers is needed. The collision rate of air molecules is about 7 billion collisions/second, so the mean time between collisions is around 150 picoseconds. The de-excitation/thermalization that Happer refers to does not occur for every collision. Non-radiative deactivation rates have been measured very precisely by Siddles, Wilson and Simpson( The vibrational deactivation of the (00o1) and (0110): Modes of CO2 measured down to 140 K - ScienceDirect ), and at STP the average deactivation time for the bending vibration of CO2 is about 10 microseconds. This is discussed in detail in both of our references linked at the beginning of this comment. This means each CO2 molecule will experience about 50,000 thermalizations/second. This is a difference of four orders of magnitude relative to what Happer alluded to in the discussion. These thermalizations of excited CO2 transfer heat to the non-IR active molecules and together with the heat from direct conduction this drives convection in the troposphere from the bottom up.
In his paper “The Quantum theory of Radiation”, Einstein showed that the radiation field in this scenario will follow a Planck distribution. At STP, approximately 4.4% of the CO2 molecules will be in an excited state because of collisional excitation (sometimes called reverse thermalization). The collisional de-excitation time is ~ 10 microseconds and the half-life of the excited state for emission is ~0.5 seconds, so only about 1:50,000 CO2 molecules will radiate without being thermalized. Nonetheless, this creates a significant random radiation field that can be measured in any direction. This is the source of the so-called "back radiation”, a topic for another discussion. I do find concerning Happer’s comment that the pulse
from an emitting CO2 molecule if uninterrupted would have a length equal to the radiative lifetime. Photonic confusion.
A similar process occurs for all IR active species. In the case of water vapor, it emits across almost the entire IR band and so can be excited by a larger population of collision partners. I have not found a reference estimate for H2O but would expect it to be considerably larger than the 4.4% for CO2.
This random radiation field does not transport energy in any specific direction. The energy transport upwards in the atmosphere is the result of convection. The thermal (kinetic) energy is distributed amongst ALL molecules. In addition, there is the latent heat of water vapor. The transport of this energy is performed by the mass transport of convection, not by the propagation of a radiation field.
Getting back to CO2 for now, the radiative emission to escape the atmosphere is suppressed by the thermalization at a ratio of 50,000:1, and the radiation from the small fraction of molecules that do emit is reabsorbed either by CO2 or water vapor which has a strong absorption spanning the CO2 band.
What is the condition for radiation from these CO2 molecules to escape to space? The deactivation rate is proportional to the overall density of molecules. At the tropopause for example, it is decreased from ~50,000:1 to ~30,000:1 for CO2. In order for an excited state to escape deactivation and allow emission into space, that ratio needs to approach 1:1. This occurs at a pressure of ~1/50,000 pressure at STP, ~2 Pa, at the mesopause. That is why we see a tiny CO2 emission peak at the center of the bottom of the “dip”. It is tiny because most of the energy has been released via water vapor emission which is described next. The emission altitude is not determined by the concentration of the IR active gas species. It is determined the relative rates of collisional excitation and spontaneous
emission.
Because water vapor is a condensing gas, its concentration in the troposphere drops precipitously with altitude. It may surprise you that water vapor emits across almost the entire spectral range, even in the socalled atmospheric window. You can see this in Herman Harde’s paper from 2013 Radiation and Heat Transfer in the Atmosphere: A Comprehensive Approach on a Molecular Basis - Harde - 2013International Journal of Atmospheric Sciences - Wiley Online Library. His figures 17 and 18 are relevant here. The tiny peak at the center of the CO2 band is not present in his figure 18 because the spectrum is modeled at 12.5 km altitude, well below the emission altitude of 86 km for CO2. I find his treatment considerably more rigorous and transparent than van Wijngaarden and Happer, though it predates them by a few years. More comments on the differences at the end of this discussion.
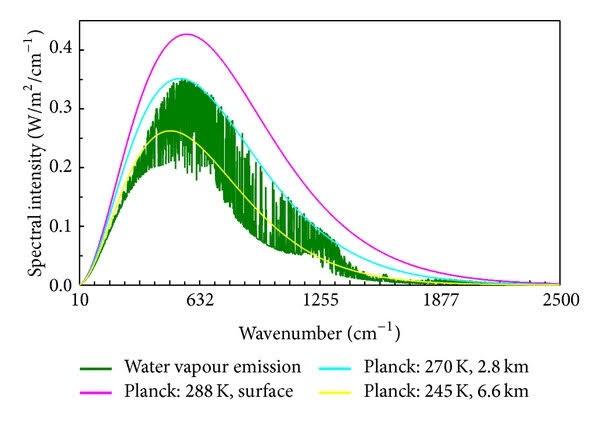
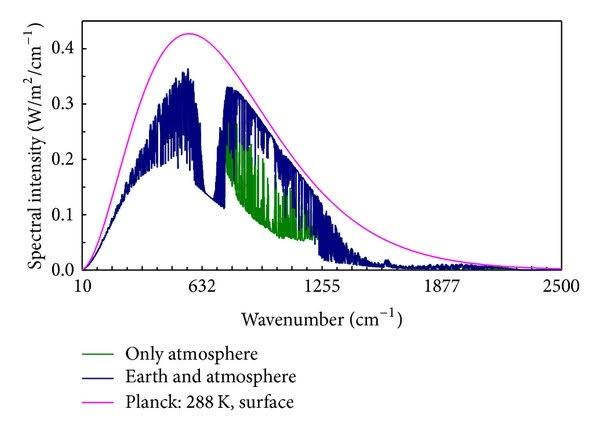
Harde Figures 17 & 18
Due to the reduction of partial pressure because of condensation, the condition for water vapor emissions to escape to space are satisfied at a much lower altitude than for CO2. Harde’s model would indicate ~2.8-6.6 km at subtropical latitudes with a surface H2O concentration of 1.46%. The relatively dense atmosphere and broad emission band means that water vapor emits fiercely in this range and accounts for almost all the radiative energy emission into space. The condensation also results in the release of latent heat which is absorbed by collisions and that energy further drives excitation and emission. The tropopause exists because it is where the cooling of the lower atmosphere via radiation is complete. The radiation emitted by ozone in the stratosphere and CO2 at the mesopause is insignificant. The atmosphere is cooled by emissions from water vapor.
Where does the “dip” around the CO2 peak come from? In the band of water vapor emission (mid to upper troposphere), there is still considerable CO2 which is non-condensing. The CO2 absorbs part of the water vapor in its active band centered around 667/cm. If it absorbed all of it, the dip would go to the baseline. This absorption by CO2 is commonly misinterpret as “trapping” heat. On the contrary, at that altitude the CO2 does not emit but is still subject to collisional deactivation which redistributes the energy (thermalization) and that energy continues to drive collisional excitation of water vapor.
The less obvious conclusion one can draw from this is that IR absorbing gases are solely responsible for removing heat from the atmosphere, contrary to the so-called GHE. It is the emissive properties of IR-active gases that cool the earth, contrary to the “conventional wisdom” that the absorptive properties cause the earth to warm.
I find contrast between the Harde work and that of van Wijngaarden and Happer (vWH) interesting. In Harde, he provides a much more complete picture including what is effectively a derivation of Einstein’s Quantum Theory of Radiation paper with recognition and a complete treatment of the role of collisional excitation and de-excitation as the driving process in the dense lower atmosphere. This is discussed in passing at best by vWH. Harde includes convection specifically in his model, though only the minimal 3 W/m^2 promoted by the IPCC at that time. vWH states in some papers that convective energy transport is dominant in the troposphere but provide no treatment of it and proceed with using the RTE alone to describe energy transport. The one comment in the Burton/Happer that surprised me and that I agree with is that the emissions from the atmosphere a function of the temperature and composition, independent of the heat source for the atmosphere.
In both cases the authors are driven by a radiation-centric perspective and
a desire to reconcile the concept of the GHE which cannot exist. The calculations of the equations that they present are correct. It is perspective that limits the interpretation. More on this in another post.
Attachments area
Preview YouTube video Markus Ott: Convection and Thermalisation Kill The Greenhouse Effect
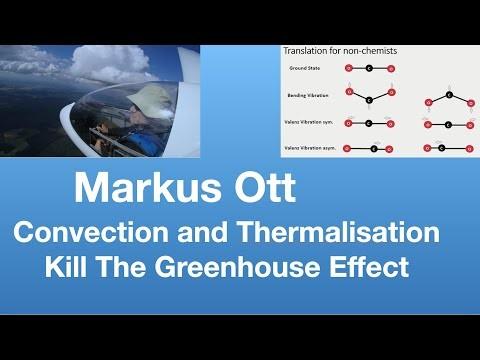
Markus Ott: Convection and Thermalisation Kill The Greenhouse Effect