Tropospheric Ozone variability in Delhi during Pre & Post Monsoon
periods: Decoding influence of Seasonal variation, diurnal variation, shortrange and long-range transport
Sachin Dhawan a, Mohan P George b, K S Jayachandran b, Mukesh Khare a, *
a Department of Civil Engineering, Indian Institute of Technology, Delhi, 110016
b Delhi Pollution Control Committee, Delhi, 110006
*Corresponding Author – Email address: mukeshk@civil.iitd.ac.in, phone: +91-11-2659-1212
Electronic copy available at: https://ssrn.com/abstract=4160065
1 Tropospheric Ozone variability in Delhi during Pre & Post Monsoon 2 periods: Decoding influence of Seasonal variation, diurnal variation, short3 range and long-range transport 4 Sachin Dhawan a, Mohan P George b, K S Jayachandran b, Mukesh Khare a, * 5 a Department of Civil Engineering, Indian Institute of Technology, Delhi, 110016 6 b Delhi Pollution Control Committee, Delhi, 110006 7 * mukeshk@civil.iitd.ac.in, +91-11-2659-1212 8 9 Abstract 10 This study examines the contribution of various monitoring sites of Delhi categorized as Green Areas, Urban 11 Areas, Industrial/Commercial Areas, Traffic Areas, and Residential Areas. The mean hourly O3 12 concentration during the pre-monsoon season varies from 2630 to 8569 g/m3 and in post-monsoon 13 season varies from 1348 to 4958 g/m3. Maximum O3 concentration is found at sites categorized as Green 14 Areas and minimum at Industrial/Commercial Areas for both the pre-and post-monsoon seasons. The 15 contribution of O3 from short-and long-range transport, downward draft, biogenic emissions are found to be 16 maximum at green Areas which act as a sink for the O3. HYSPLIT cluster analysis was done to see the 17 influence of long-range transport on the O3 concentration across all sites. The air masses originating from 18 3500m or above contributed more to O3 in green Areas only. Air masses originating from S-W during pre19 monsoon and East during post-monsoon contributed to high concentration events in industrial/commercial 20 Areas only. The stubble burning episode in N-W contributed to high concentration events in urban areas 21 and residential areas. The contribution of O3 in Residential Areas can be attributed to long-range transport 22 from the N-W direction with air-masses originating at height <1500 m and the local influences. 23 Keywords: Tropospheric Ozone, HYSPLIT back trajectory analysis, Long-range transport, short-range 24 transport, Meteorological parameters 25 26 1.0 Introduction Electronic copy available at: https://ssrn.com/abstract=4160065
27 The tropospheric Ozone (O3) present in the ambient air, is a greenhouse gas (GHG) which has detrimental impacts 28 on human health and is also linked to climate change (Kumari et al., 2020; Ghude et al., 2016; Londhe et al., 29 2008). The concentration of O3 in ambient air is continuously increasing due to growing consumption of fossil 30 fuels for transportation, increase in power generation and industries (Chand & Lal, 2004; Debaje & kakade, 2006). 31 The concentration of O3 in ambient air is increasing at a rate 1-2% per year for the O3 present in troposphere as 32 well as the O3 near the earth surface (Lal et al., 1999; Kumari et al., 2020; Verma et al., 2017). 33 The O3 levels present in the troposphere are majorly attributed to two phenomena, one, stratosphere to troposphere 34 exchange of the O3 and two, photochemical generation from precursors such as CH4, CO, and VOCs, in presence 35 of NOx from anthropogenic and natural processes. Further, long-range transport of O3 may also be one of the 36 reasons for O3-rich air masses. The contribution of O3 from the stratosphere to troposphere exchange is 23%; and 37 the contribution of O3 production from the photochemical generation is the major source of O3 in ambient air 38 (Verma et al., 2017; Yadav et al., 2016; Sinha et al., 2021; Lal et al., 2017, Mahapatra et al., 2014). The 39 concentration of O3 in ambient air is either dependent on VOCs or NOx. The areas where the concentration of O3 40 in ambient air is dependent on VOCs, termed as VOCs-sensitive regime; where the concentration of O3 is 41 dependent on the NOx concentration, termed as NOx-sensitive regime. However, In India, it is considered to be 42 NOx limited for the photochemical production of O3 because anthropogenic emissions of NOx from combustion 43 activitiesaredominant(Vermaetal.,2018;Debaje&kakade,2006).TheincreaseintheO3 levelscanbeattributed 44 to various reasons such as the meteorological conditions, the ventilation coefficient, the precursor emissions, 45 decreased O3 titration due to limited NOx emissions, and rapid production of ozone due to the local photochemical 46 reactions in warm and calm wind conditions. Further, the long-range transport of O3 at regional scale may also be 47 possible if wind speeds are higher resulting into its advective transport to the local receptor (Pont & Fontan, 2001; 48 Verma et al., 2017; Yadav et al., 2016; Mahapatra et al., 2012; Kumari et al., 2020). Further, it has been observed 49 that the concentrations of O3 during weekends are relatively higher than those on weekdays. It may be due to 50 lower ambient concentration of particulate matter during weekends, which results in less absorption of sunlight 51 and so enhanced concentration of O3 during weekends. Besides, the heavy-duty vehicles number are higher on 52 weekends as there is no peak hour time restrictions which leads to higher NOx concentrations resulting into higher 53 O3 production (Dreher & Harley, 1998; Marr & Harley, 2002a, b; Debaje & kakade, 2006). 54 Ozone is also a major source of OH radical in the troposphere. The self-cleansing mechanism of the atmosphere 55 is also dependent on the OH radical (Mahapatra et al., 2018). The NO2 present in the air acts as a catalyst, which 56 dissociates photochemically to form NO and atomic oxygen, O(3P) (Eq. 1) in the presence of solar radiation. In
57 the following paragraph, the ozone photochemistry has been briefly explained (Lal et al., 1999; Chand & Lal, 58 2004; Yadav et al., 2016; Biswas et al., 2019; Kumari et al., 2020 Nelson et al., 2021): 59 (E1) ����2+ℎ��→����+��(3��) 60 The reaction between the oxygen atom and oxygen molecule leads to the formation of O3 (Eq. 2). The NO 61 produced in Eq. 1 reacts with HO2 radical and converts back into NO2 (Eq. 3), which acts as a catalyst for the O3 62 production process. 63 (E2) ��(3��)+��2+��→��3+�� 64 (E3) ����2+����→����2+���� 65 However, in the absence of sunlight i.e., at night, O3 reacts with NO2 to form nitrate radical (NO3) (Eq. 4) in the 66 ambient air, which in turn aids in the removal of NOx from the atmosphere. 67 (E4) ��3+����2→����3 68 69 The present study includes analysis and description of O3 ambient concentrations at 23 different locations of the 70 Delhi city, having different land use characteristics, and traffic density during the periods, February – June 2018 71 (pre-monsoon) and October 2018 – January 2019 (post-monsoon). Besides, the source identification has also been 72 carried out using backward trajectory cluster analysis at few selected sites. 73 74 2.0 Materials
75
and Methods
2.1 Study Region
76 The study region is the National Capital Territory (NCT) of Delhi, NCT comprises the capital city of Delhi and 77 surrounding cities in states of Haryana, Uttar Pradesh, and Rajasthan (Figure 2). It is located at an altitude of 215m 78 above mean sea level and is among the 132 declared non-attainment cities (NCAP, 2021). It is landlocked by state 79 of Haryana and Punjab from the west side, where crop burning activities are maximum during the months of 80 October-November and state of Uttar Pradesh which is the most populated state of India from east side (Kalbande 81 et al., 2021). As per the WHO (2018) report, the cities with high ambient air pollution levels across the world,
82 India has thirteen cities in it including Delhi. Delhi ranks highest in the economic loss per capita due to premature 83 deaths morbidity attributable to air pollution (Pandey et al., 2021).
84 Delhihassemi-arid climateandexperiencesfourmajorseasonsWinter(Dec-Feb),Summer(Mar-May),Monsoon 85 (Jun-Aug) and Post-Monsoon (Sep-Nov) (Sharma et al., 2021; Kumar et al., 2017). During summer the city is 86 influenced by the dry weather, during the season with temperature reaching up to as high as 500C. This season is
87 accompanied by low humidity, scorching heat and hot stormy winds comprising of the dust from the Thar desert 88 in West. The dry season can be categorized into two periods, i.e., summer, and post-monsoon, during which the 89 solar radiation is high (Sinha et al., 2021). The rainfall experienced during the monsoon season comprises of the 90 80% of the annual rainfall (500-800 mm) of the city, it begins with the arrival of southwestern winds travelling 91 from the Arabian sea (Sharma et al., 2021; Perrino et al., 2011). The southwest monsoon is dominant over this 92 region during the monsoon season, precipitation, heavy rainfall, and high humidity is common during this season. 93 After the post-monsoon season, the air masses originate from the west and northwest direction as there is shift of 94 the southwest to northwest monsoon (Sinha et al., 2021). Comparative assessment of temperature and relative 95 humidity (RH) for pre- and post-monsoon season are given in Table 1. During pre-monsoon, the temperature of 96 the city reached up to 460C or sometimes more with mean-minimum temperature 150C during the month of March 97 and mean maximum temperature of 450C during the month of May (Warmest-month of Identified period); during 98 post-monsoon the temperature of the city goes down to 30C or sometimes less with mean minimum temperature 99 5.160C for the month of December (coldest month of the identified period) and mean maximum temperature 260C 100 for the same month due to frequent ground-based inversion conditions; the cold winds travel from the Himalayas 101 in the North-West (N-W) during post-monsoon season. The occurrence of haze, several fog and poor visibility 102 is common during this season. The high contrast in the summer and winter temperatures are attributes of the 103 Delhi’s Climate (Kalbande et al., 2021; Sharma et al., 2021; Kumar et al., 2017). The average RH during post104 monsoon was 43%, with 6% as the mean-minimum RH and 96% as the mean-maximum RH of all sites; the 105 average RH during post-monsoon was 66%, with 20% as the mean-minimum RH and 95% as the mean-maximum 106 RH of all sites. 107
Table 1: Seasonal comparative assessment of Temperature and Relative Humidity for pre-monsoon, and post108 monsoon seasons
Season Temperature (0C) Mean (Min. – Max.)
Relative Humidity (%) Mean (Min. – Max.)
Pre-Monsoon 30.58 (14.61- 45.15) 43.06 (5.73 – 96.37) Post-Monsoon 18.33 (5.16 – 35.9) 64.77 (19.58 – 95.30) 109 110 Figure 1 shows the diurnal variation of Solar Radiation (SR) in pre- and post-monsoon seasons, the mean111 maximum SR was 720 Wm-2 and mean-minimum SR of all sites was 4 Wm-2 during the pre-monsoon season; the
112 mean-maximum SR of all sites was 559 Wm-2 and mean-minimum SR of all sites was 4 Wm-2 during the post113 monsoon season.
(a) (b) 114 Figure 1: Diurnal variation assessment of Solar Radiation (SR) for (a) pre-Monsoon, and (b) post-Monsoon 115 season 116 2.2 Monitoring stations, data collection & analysis 117 The local air quality is influenced by the local emission sources contributions of the long-range transport, 118 meteorology, topographical factors, and Land use (Tombette and Sportisse, 2007; Mensink et al., 2007; Vautard 119 et al., 2007); which directly influences the concentration of pollutant across different locations. The ambient air 120 quality stations are categorized into different categories based on the location of the station to see the influence of 121 local sources on to the concentration of pollutant. In various studies, the average concentration of a specific 122 pollutant across all the stations is considered as the city average; however, it is not the case as the stations installed 123 are disproportionately located. The selected stations for this study are scattered throughout the city having 124 different surrounding environment. The sites selected for the analysis comprises of different types of areas such 125 as urban areas, industrial/commercial areas, traffic areas, residential areas, and green areas. The sites are selected 126 to compare the concentration of O3 in different types of areas and inter-comparison of O3 concentration in same 127 type of areas. The selection of monitoring stations was done based on data availability of O3, temperature, wind 128 speed (WS), wind direction (WD), relative humidity (RH), & solar radiation (SR). Continuous hourly average 129 data for all the selected parameters were selected from the whole datasets to assess the effect of meteorological 130 parameters at different sites on the O3 concentration. The missing values in the dataset are removed from the 131 analysis to properly understand the relationship of all meteorological variables with O3, replacing the missing 132 values with the median values will trade the original value of the relation between all variables. The outliers are 133 not removed from the study to understand about the influence of variables and location characteristics on to the 134 pollutant concentration. Table A1 describes the ambient air quality stations and their characteristics based on their Electronic copy available at: https://ssrn.com/abstract=4160065
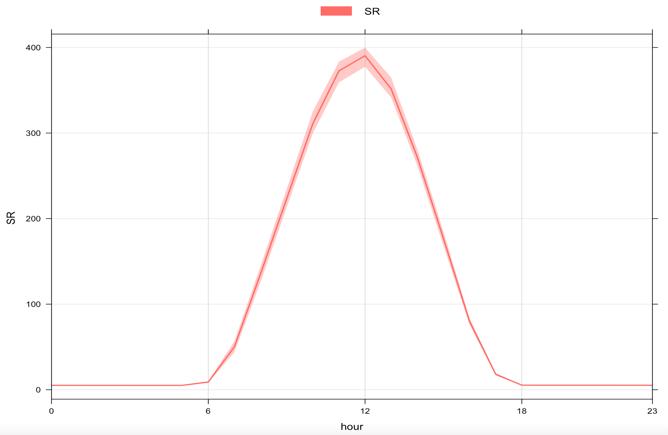
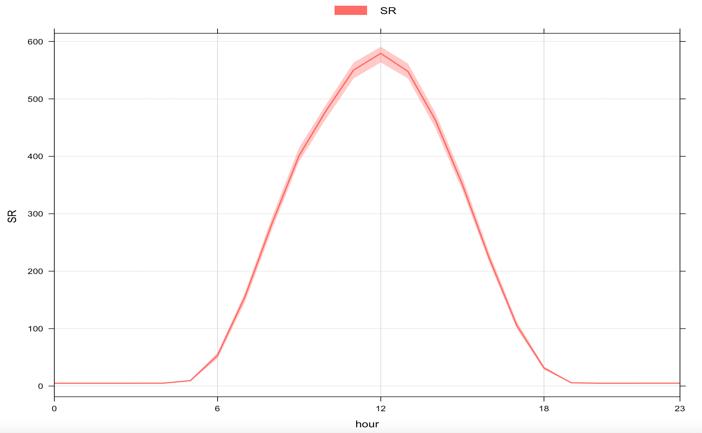
135 respective surroundings. Figure 2 shows the geographical locations of the selected monitoring sites for the 136 analysis. 137 138 Figure 2: Selected Delhi CAAQMS sites with varying characteristics 139
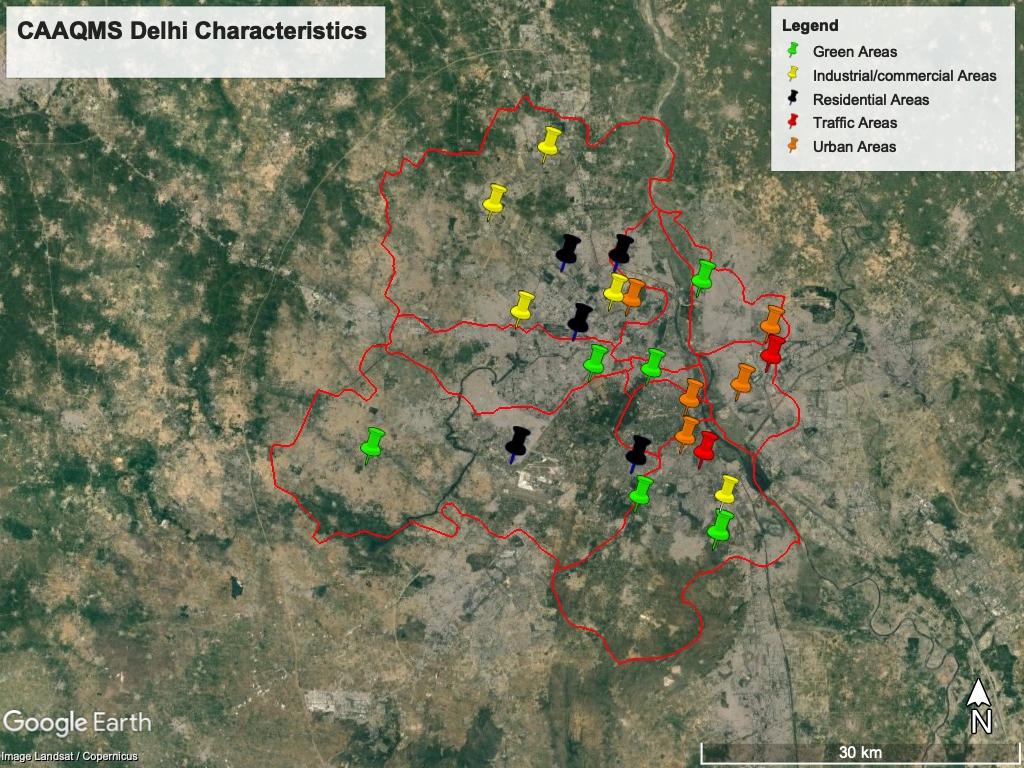
2.3
HYSPLIT back-trajectory and Cluster Analysis
140 Hybrid Single-Particle Lagrangian Integrated Trajectory (HYSPLIT) model was used to understand the role of 141 transport of O3. The origin of air parcels can be discovered using the back trajectory analysis and these simulations 142 can be used for understanding the relationship between source and receptor by demarking the transport path to 143 monitoring locations (Draxler and Hess, 2004). The Global Assimilation Data System (GDAS 10) met-file was 144 included in the simulation for the back trajectory analysis, and simulations was done for 96-h back trajectory 145 arriving at the monitoring sites. These back trajectories provide a 3D air parcel path into the receptor sites as 146 derived from 3D gridded wind/pressure field. Single trajectories do not provide clear understanding of the 147 pathways for air parcel reaching the receptor locations, however, clustering of the trajectories using k-means 148 clustering provides better understanding and a better continuous delineation of the pathways can be achieved by 149 including large number of trajectories (Camalier et al., 2007; Saini et al., 2014; Verma et al., 2017; Kaspargolu et 150 al., 2018; Mukherjee et al., 2018). The monthly back trajectory cluster analysis was performed at height of 50 m 151 through vertical velocity model to understand the influence of long-range transport on the monitoring sites (Tu et 152 al., 2007; Mahapatra et al., 2012). The monthly back trajectory cluster is given in figures 3 and 4.
Clustermeans-Standard 622backwardtrajectories GDASMeteorologicalData
April
Clustermeans-Standard 622backwardtrajectories GDASMeteorologicalData
May June 153 Figure 3: Month wise HYSPLIT Back Trajectory Cluster Analysis for Pre-Monsoon Season 154 Figure 3 shows the month wise back trajectory cluster analysis for the pre-monsoon season which shows that the 155 predominant wind direction for the month of March, April and May is N-W with sometimes air masses originating 156 at height more than 3000m. During April and May, the influence of air masses originating from Indo-Gangetic 157 Plain(East(IGP))atheightoflessthan500mandreachingthestudylocationcanbeobserved.Theclusteranalysis 158 for the month of June reveals that the air masses originating from South-West and East i.e., IGP at height of less 159 than 500m; and air masses from N-W originating at height of 1000m, are equally distributed i.e., South-West 160 (30%), East (36%), and N-W (34%). 161 Figure 4 shows the month wise back trajectory cluster analysis for the post-monsoon season which shows that the 162 predominant wind direction for the month of October, November and January is N-W with air masses originating 163 at height of 1500m for few clusters in October and January, and at height of 2500m for few clusters in November. 164 The influence of air masses originating from east i.e., IGP at height of less than 500m and reaching the study
165 location can be observed in the month of October. The back trajectory cluster analysis shows that the air masses 166 reaching the study site is composed of various clusters, majorly from N-W direction and east for the month of 167 December. Further, it has been observed that for most of the clusters, the air masses originating at height of 2000m 168 or less except cluster 1 which originates at a height of more than 3500m and contributes almost 8% of the total air 169 masses; regional re-circulation of air masses is also observed.
177 the post-monsoon (1348 to 4958 g/m3) season as observed by Sharma et al., 2021.; the high mean O3 178 concentration is observed in areas categorized as green areas as compared to the other areas. The reasons for high 179 O3 concentration at green areas may be due to (i) green areas act as sink for the O3 emissions from urban 180 areas/traffic areas/commercial areas/residential areas due to higher vehicular activities and combustion sources, 181 (ii) O3 has a longer lifetime and it is possible that the higher concentration at green areas could be attributed to the 182 long-range or short-range transport of O3 from the precursor locations, (iii) the biogenic volatile organic 183 compounds (VOCs) emitting from the flora contributing to the formation of O3, as they are more reactive than 184 anthropogenic VOCs (Paoletti, 2009; Verma et al., 2017; Yadav et al., 2016; Sinha et al., 2021; Lal et al., 2017, 185 Mahapatra et al., 2014). The mean O3 concentration in traffic areas is low, but high concentration events are 186 observed in these areas during both day and night. (Yadav et al., 2016; Verma et al., 2017; Sharma et al., 2021; 187 Sinha et al., 2021). The lower concentration of O3 during the post-monsoon season can be attributed to low mixing 188 layer height during these months, low solar radiation as compared to the pre-monsoon season, low average 189 temperature and high relative humidity (Mahapatra et al., 2012; Sinha et al., 2021). 190 191
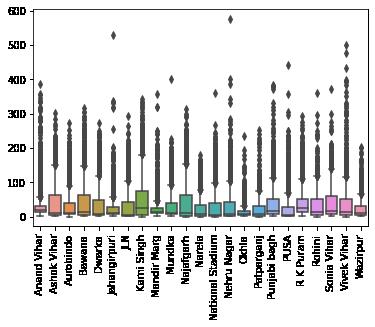
Figure 5: Boxplot for hourly O3 concertation for pre-monsoon (left) and post-monsoon (right) season 192 193 3.2 Correlation Analysis of ozone and meteorological parameters 194 195 The correlation between the hourly O3 concentration and meteorological parameters on different sites for pre196 monsoon and post-monsoon season are given in figure 6. The hourly O3 concentration shows positive correlation 197 with the wind speed, temperature & solar radiation for both seasons, however, the relative humidity shows 198 negative correlation with the hourly O3 concentration. The wind direction has not much influence on the hourly
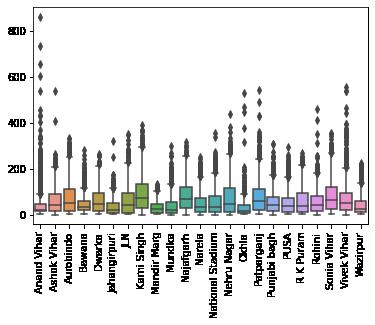
199 O3 concentrationonmostofthesitesforbothseasons.Figure6showsthecorrelationofozonewithmeteorological 200 variables for different sites across Delhi city. All the sites categorized as green areas show strong positive 201 correlation with temperature & solar radiation, and strong negative correlation with the relative humidity for both 202 the pre-monsoon (Temp: 0.18-0.73, RH: (-0.43) - (-0.72), SR: 0.57-0.69) and post-monsoon season (Temp: 0.14203 0.76, RH: (-0.19) - (-0.77), SR: 0.15-0.70); the correlation for industrial/commercial correlation for post-monsoon 204 (Temp: 0.38-0.71, RH: (-0.41) - (-0.78), SR: 0.39-0.62) is season is stronger than that of pre-monsoon season 205 (Temp: 0.40-0.58, RH: (-0.38) - (-0.57), SR: 0.48-0.64); in urban areas the correlation for pre-monsoon (Temp: 206 0.46-0.67, RH: (-0.41) - (-0.78), SR: 0.42-0.69) and post-monsoon season (Temp: 0.43-0.67, RH: (-0.47) - (207 0.74), SR: 0.42-0.76), positive correlation between temperature & solar radiation with O3, and relative humidity 208 shows a strong negative correlation, Weak positive correlation is observed between wind speed and O3 209 concentration (0.08 - 0.56); in traffic areas Anand Vihar site do not show correlation with any of the 210 meteorological parameters (Temp: 0.17 - 0.20, RH: (-0.063) - (-0.24), SR: 0.11 – 0.12), the lower correlation with 211 solar radiation indicates the high nighttime O3 concentrations. Nehru Nagar site shows correlation similar to that 212 of urban areas; in residential areas the correlation for pre-monsoon (Temp: 0.37-0.65, RH: (-0.35) - (-0.61), SR: 213 0.44-0.67) and post-monsoon season (Temp: 0.25 - 0.65, RH: (-0.34) - (-0.74), SR: 0.33-0.54), the strong positive 214 correlation between temperature & solar radiation with O3 and strong negative correlation with relative humidity 215 is observed, except Punjabi Bagh site during pre-monsoon season and R. K. Puram during post-monsoon and the 216 correlation between wind speed and O3 concentration (0.22 – 0.54) on residential sites is better than all other sites (a) (b) 217 Figure 6: Correlation plot between Ozone and meteorological Parameters for (a) pre-monsoon, and (b) post218 monsoon season 219
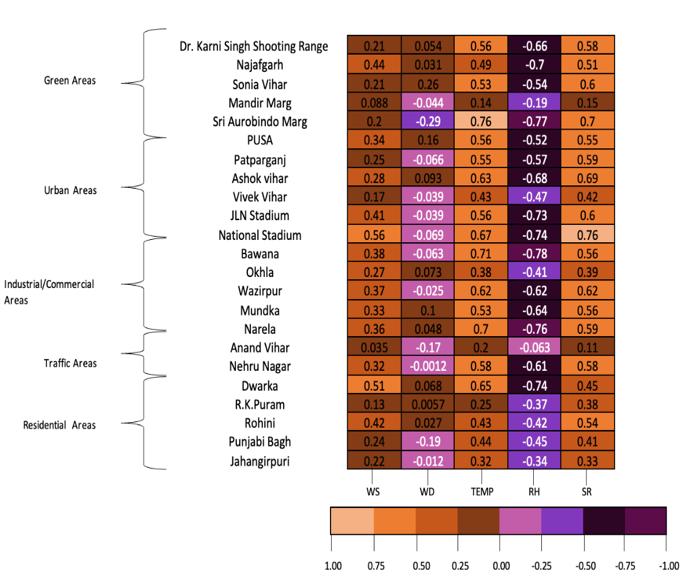
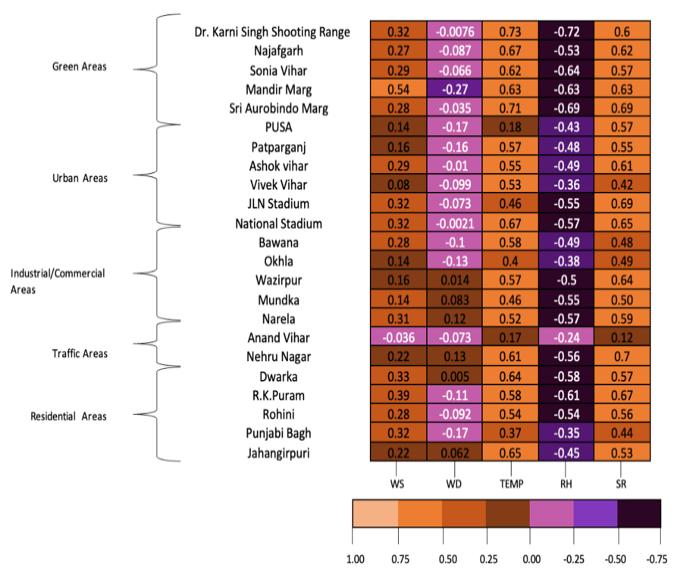
220 In green areas, during the pre-monsoon season the correlation between wind speed and O3 is weak positive (0.28-
221 0.54), but during post-monsoon season the wind speed and O3 shows no correlation or weak correlation (0.088-
222 0.44) due to less wind speed during the post-monsoon season. All sites categorized as green areas show similar
223 trend between O3 and meteorological parameters except Mandir Marg (figure 2 & table A1). Mandir Marg is in 224 New Delhi with most of the surrounding areas are covered by forests; however, a connecting road between two
225 major roads is in proximity which experiences heavy traffic during peak hours; NOx and particulate matter from
226 vehicles can influence the concentration of O3 on this site. The dispersion of pollutants in the post-monsoon is
227 limited due to lower pressure gradient which indicates minimal horizontal mixing (Verma et al., 2017).
228 229
3.3 Diurnal & Seasonal Variation of Ozone
230 The mean O3 concentration varied from 29-85 g/m3 during the pre-monsoon season and 23-49 g/m3 during
231 post-monsoon season in green areas; 49-72 g/m3 during the pre-monsoon season and 20-39 g/m3 during post-
232 monsoon season in urban areas; 26-48 g/m3 during the pre-monsoon season and 13-40 g/m3 during post-
233 monsoon season in industrial/commercial areas; 38-67 g/m3 during the pre-monsoon season and 31 g/m3 on
234 both sites (Anand Vihar & Nehru Nagar) during post-monsoon season in traffic areas; 33-59 g/m3 during the
235 pre-monsoon season and 24-41 g/m3 during post-monsoon season in residential areas. The seasonal, diurnal,
236 monthly and day-wise variation of O3 for pre- and post-monsoon season are given in figure 7 and further in A1-
237 A2, A5-A6, A9-A10, A13-A14, A17-A18.
238 Mean monthly maximum O3 concentration was observed in month of May for pre-monsoon season for all areas 239 except for Anand Vihar and Punjabi Bagh site (figure 2 & table A1). Anand Vihar site is dominated by traffic and 240 diurnal pattern at this site is entirely different from all other sites; thus, high concentration is observed during 241 month of April. Punjabi Bagh site experiences very high concentration in month of March as compared to other 242 sites. Mean monthly maximum O3 concentration was observed in month of October for post-monsoon season for 243 all areas except residential areas. Apart from Dwarka and Jahangirpuri (figure 2 & table A1) all other sites have 244 maxima during different months. While R. K. Puram (figure 2 & table A1) site has maxima during month of 245 December, Punjabi Bagh and Rohini site had maxima in month of November and January respectively. The 246 seasonal variation suggests that the ozone at these areas is influenced more by the local sources, as visualized 247 using the polar plots given in figure A19 & A20. 248 The diurnal pattern of ozone concentration during pre-monsoon season shows a unimodal peak which is consistent 249 with the finding from Varshney and Aggarwal, 1992; the high O3 concentration for pre-monsoon season was
250 observed between 11:00-18:00 h, it starts increasing after 6:00 h and the maximum concentration is observed 251 around 13:00-14:00 h for all areas except traffic and residential areas. Residential areas show bimodal peak with 252 another peak during the night-time between 00:00-5:00 h. At traffic areas the maximum hourly concentration is 253 observed during 11:00 - 18:00 h, 21:00 – 23:00 h, and 00:00 - 03:00 h; it shows a trimodal peak. Anand Vihar 254 (traffic area) is located in east Delhi; it is in close vicinity to an Inter State Bus Terminus (ISBT) with heavy traffic 255 load mostly consisting of Light Commercial Vehicles (LCVs), and Heavy Commercial Vehicles (HCVs), mainly 256 buses. The site is in neighborhood of Industrial and Commercial Areas and lies on the border of Delhi and Uttar 257 Pradesh (Goyal et al., 2019). The maxima during the night hours at Anand Vihar site can be due to the presence 258 of high vehicular activity that increases the NO titration effect and reduces the O3 concentration during night.
259 High concentration events are observed for few days every month in pre-monsoon season. However, during post260 monsoon season the high nighttime concentration events are attenuated in the month of October and December. 261 The Lower O3 concentrations during nighttime allows for the downdraft at night, which gets associated with the 262 surface to the lower troposphere and brings down air with high O3. This is more distinct during the night than in 263 the afternoon (Betts et al., 2002).
264 The O3 concentration for post-monsoon season also shows unimodal peak feature, the high O3 concentration was 265 observed between 12:00-15:00 h; it starts increasing after 09:00-10:00 h and the maximum concentration is 266 observedaround14:00hforallsitesexcepttraffic and residential areas. Traffic&Residentialareasshowbimodal 267 peak feature with another peak during the night-time between 01:00-06:00 h & 00:00-5:00 h, respectively. The 268 unusual peaks during the early morning hours can be due to urban heat island effect and carry over of O3, the 269 reduction in NO from vehicles leading to dilution of titration effect, and downdraft at night (Betts et al., 2002; 270 Matt and Harley, 2002; Taha 2008; Mahapatra et al., 2012). 271 It is interesting to note that the mean monthly O3 concentration at Mundka site for the month of June is 272 approximately 10 g/m3 which is 80% less than the month of May and 73% less than the average pre-monsoon 273 concentration on the site. The mean monthly O3 concentration of Okhla during the month of November is 5 g/m3 , 274 which is 75% less than the concentration in month of October and 62% less than the average of post-monsoon 275 season. The sudden change in the monthly mean concentration at these are a matter of intensive investigation. The 276 mean monthly O3 concentration of Industrial/commercial sites is least during the pre-monsoon season followed 277 by urban areas, residential areas, traffic areas and green areas, respectively. However, during post-monsoon the 278 mean monthly O3 concentration at Industrial/commercial sites is equivalent to that of urban areas followed by 279 traffic, residential and green areas.
281 3.4 Contribution of O3 from long-range transport and short-range transport 282
283 3.4.1 Green Areas
284 The bivariate polar plots for O3 in green areas have been shown in figures A3 & A4. The bivariate polar plots 285 show that during the pre- and post-monsoon seasons, the high O3 concentration is associated with higher wind 286 speeds (>2 m/s), indicating regional movement of O3 to the monitoring sites except at the Mandir Marg site 287 (Mukherjee et al., 2018; Grange et al., 2016). The long-range transport of O3 and emission of biogenic VOCs 288 from the vegetation in urban green areas lead to the formation of O3 in green areas. The relative high concentration 289 of O3 in green areas qualify the role of vegetation as the sink for O3 (Manes et al., 2008). 290 Figure 7 shows the variation of ozone at Dr. Karni Singh shooting range site for the pre-monsoon (Fig. 7(a)) and 291 post-monsoon season (Fig. 7(c)) along with the bivariate polar plots for pre-monsoon (Fig. 7(b)) and post292 monsoon season (Fig. 7(d)). Figure 7(e) shows the ozone air mass concentration Hysplit plots for the month of 293 march, indicating that maximum concentration episodes are attributed to cluster 1 (shown in figure 3) originating 294 from N-W direction at a height of approximately 1000m. However, Cluster 3 (13%) is also contributing high 295 concentration episodes as a result of the air masses originating from N-W direction at an approximate height of 296 4000m. Figure 7(f) shows the ozone air mass concentration Hysplit plots for the month of December. It clearly 297 showsthemaximumconcentrationepisodesareattributedtocluster1,cluster4andcluster7.Cluster1contributes 298 8%, cluster 4 contributes 15%, and cluster 7 contributes 7% (figure 4). The plots for remaining sites are given in 299 supplementary material for further reference. 300 The prevailing wind direction is similar as the respective sites for both seasons, especially for the low301 concentration events indicating that the locally originated O3 is similar for both seasons. The contribution of short302 range and long-range transport of O3 is higher than locally sourced O3. The 96-h back trajectory cluster analysis 303 for the month of December reveals that the O3 concentration events linked with air masses originating from N-W 304 more than 3500 m height are relatively higher than any other cluster, indicating the contribution of ozone from 305 long-range transport (Jain et al., 2007; Verma et al., 2017). Further, air masses originating from cluster 3 (13%) 306 during the month of March clearly shows the contribution of O3 episodes at the site. The polar plots also confirm 307 this fact (figure 7, A3 & A4). The hysplit plots show high-concentration events occurring during nights due to NO 308 titration. During daytime the tropospheric O3 concentration becomes considerably less due to dominance of 309 convective transfer (Betts et al., 2002; Khaiwal et al., 2019; Saxena et al., 2020).
a. b. c. d. e. f.
310 Figure 7: (a) & (b) Time variation and Bivariate plots for ozone at Dr. Karni Singh Shooting Range site for 311 pre-monsoon season, (c) & (d) Time variation and Bivariate plots at Dr. Karni Singh Shooting Range site 312 for post-monsoon season, (e) & (f) Ozone Air mass concentration split plots for the month of March (Pre313 monsoon), and December (Post-monsoon).
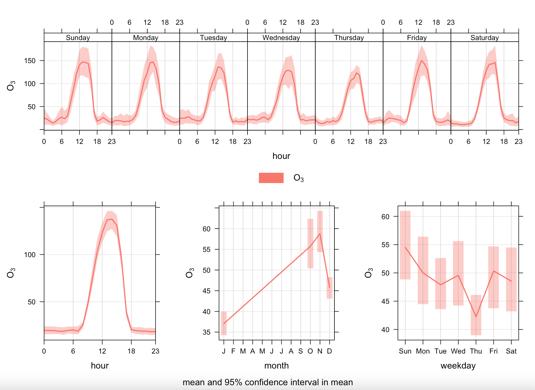
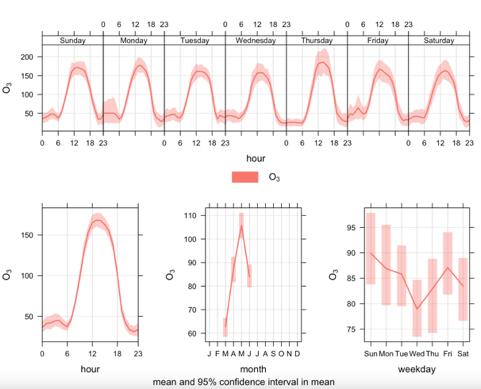
314
3.4.2 Urban Areas
315 The bivariate polar plots for O3 in urban areas are given in figures A7 & A8. The bivariate polar plots show that 316 during the pre- and post-monsoon season the high O3 concentration is associated with low wind speeds (< 2 m/s) 317 indicating the presence of locally sourced O3 at the monitoring sites. The high concentration at lower wind speed 318 indicates that the dispersion of pollutants is lacking at the urban sites (Grange et al. 2016). The contribution of
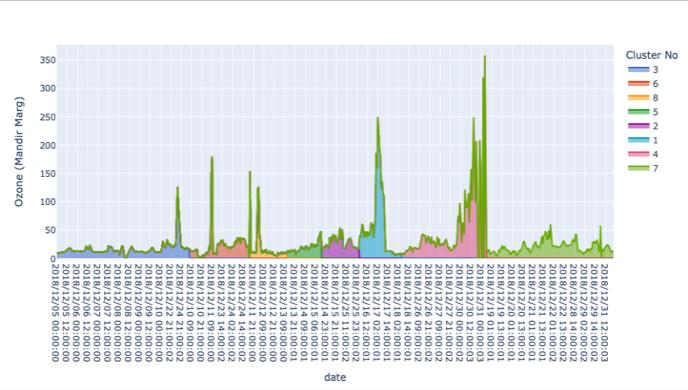
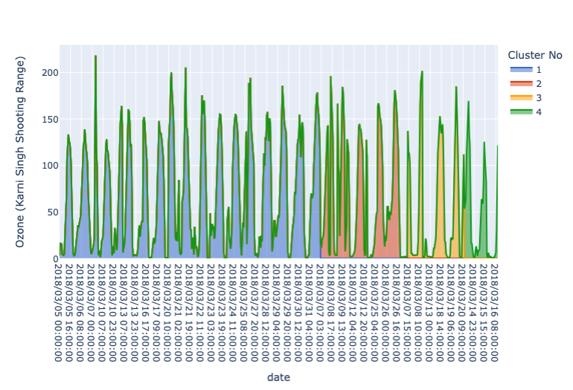
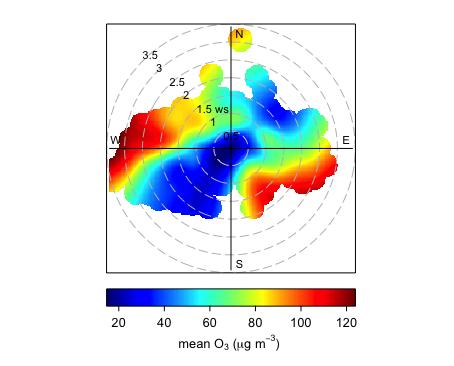
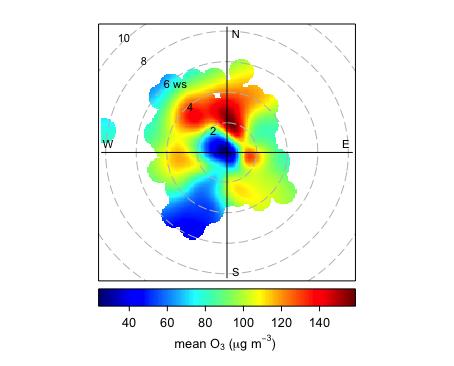
319 short-range and long-range transport to the urban sites is low at higher wind speeds (> 2 m/s). The contribution of 320 long-range transport at urban areas during the pre-monsoon and post-monsoon is limited, except for few high321 concentration events as shown in figures A21-A28. During the month of May, the air masses originating from N322 W at height above 3000m is contributing to the high concentration events. During the post-monsoon season, the 323 contribution of O3 is linked with air-masses originating from N-W direction in the month of October, November 324 and January. The concentration of O3 at urban sites is higher in the month of October and November due to stubble 325 burning in N-W regions. The releases of VOCs during the stubble burning i.e., Benzene and Toluene increase the 326 O3 formation potential in Delhi and result in higher concentrations (Kalbande et al., 2021). However, it has been 327 observed that during the month of December, the air masses originating from east i.e., IGP, and N-W region is 328 affecting the concentration of O3 at urban areas. The wind speeds are higher resulting into its advective transport 329 to the local receptor (Pont & Fontan, 2001; Chelani, 2013). 330 3.4.3
Industrial/commercial Areas
331 The bivariate polar plots for O3 in industrial/commercial areas are given in figures A11 & A12. The bivariate 332 polar plots show that during the pre-monsoon season the high concentration of episodes are linked with high wind333 speeds except at the Okhla site, whereas in post-monsoon season the higher concentration of O3 is associated with 334 both high and low wind speeds, indicating the transport of pollutants to the monitoring site and locally sourced 335 O3, respectively. The predominant wind direction during the pre-monsoon season were North, North-East or N336 W, whereas during the post-monsoon season all the sites had different predominant wind direction indicating 337 contribution of diffused local sources to O3 (Ramli et al., 2010). During the pre-monsoon season the contribution 338 of air-masses originating from the N-W direction is higher in the month of March, April, and May. However, 339 during the month of June the air masses originating from the Arabian sea in South-West direction influence the 340 O3 concentration. In the month of October, the air masses originating from east i.e., IGP at a height <500 m is 341 contributing more to the high O3 concentration events, as this region is also influenced by the stubble burning 342 (Kalbande et al., 2021). In the month of January, the contribution of long-range transport is negligible as shown 343 in figures A21-S28. It can be due to lower mixing height during the month and very high humidity. The ozone 344 formed near the ground can be assorted into the higher levels of mixing height during the day, or ozone trapped 345 upwards in the residual layer during the night. Alternatively, it can be appropriated downwards into the ground 346 level on the succeeding day (Singh et al., 1997; Pont & Fontan, 2001; Kim et al., 2007; Chelani, 2013 ; Mahaptra 347 et al., 2018) 348
349 3.4.4
Traffic Areas
350 The bivariate polar plots for O3 in traffic areas are given in figures A15 & A16. These show high concentration 351 episodes at the Anand Vihar site (figure 2 and Table A1) during the pre- and post-monsoon season, linked with 352 high wind-speeds transporting pollutants to the monitoring site. The contribution of pollutants during high353 concentration events from N-W is predominant during pre-monsoon season, however, the direction changes to 354 South-East during post-monsoon. The contribution of O3 at Nehru Nagar site (figure 2 and Table A1) from 355 localized sources is more as compared to transport of pollutants from other areas. The predominant wind direction 356 during the pre- and post-monsoon season were same i.e., East or N-W. N-W direction is associated with high 357 concentration events whereas East direction is associated with low-concentration events signifying a common 358 source during both the seasons which can be vehicles as this area is traffic dominated. The trend shown at this site 359 is different from Anand Vihar site, since it is not much impacted by LCVs and HCVs. The back trajectory cluster 360 analysis and O3 air mass concentration hysplit plots (Figures A21-A28), during the pre-monsoon season show that 361 the major contribution is locally sourced at the sites. During the post-monsoon season, the contribution of O3 via 362 long-range transport is similar as observed in urban areas (section 3.4.2) having major contribution of O3 from 363 local sources.
364 3.4.5 Residential Areas
365 The bivariate polar plots for O3 in residential areas are given in figures A19 & A20. All the sites have mostly one 366 predominant direction of O3 during pre-monsoon season except Punjabi Bagh (figure 2 and Table A1), where the 367 high-concentration and low-concentration events are both linked by two different directions 1800 opposite of each 368 other i.e., South-West and North-East. During post-monsoon season only R. K. Puram site (figure 2 and Table 369 A1) experienced O3 from the locally sources whereas all the other sites attribute high-concentration events during 370 high wind speeds implying the contribution of O3 via advective transport (Mukherjee et al., 2018). The air mass 371 O3 concentration split plots show that during the pre-monsoon season the high O3 concentration event are linked 372 with air masses originating from N-W direction and east i.e., IGP. The O3 contributing air masses originate from 373 heights <1500m during all the months. During the months of October and November, the residential site 374 experiences high O3 concentration events when the air masses are originating from the N-W region. It can also be 375 linked with the stubble burning during October and November months. Further, the contribution of O3 during the 376 months of December and January is associated with air masses originating from N-W and local sources.
377 4.0 Conclusion
378 The present study highlights that the areas categorized as green areas, urban areas, industrial/commercial areas, 379 traffic areas, and residential areas show different O3 concentrations and are influenced by various sources ranging 380 from locally sourced to long-range transport. The O3 concentration shows strong positive correlation with 381 temperature and solar radiation; strong negative correlation with relative humidity; and weak positive correlation 382 with wind speed. There is no evidence of any relationship between wind direction and O3 concentration. Almost 383 all the sites show similar correlations with the meteorological factors except the Anand Vihar site (figure 2 and 384 Table A1). 385 The O3 concentration are highest on the stations categorized as green areas. These stations experience high O3 386 concentration during both pre- and post-monsoon seasons. The lowest O3 concentration is observed at 387 industrial/commercial areas. The O3 concentration at green areas are primarily due to long-range & short-range 388 transport as well as local biogenic sources. The back trajectory cluster analysis also this. Further, the green areas 389 act as a sink for O3 as well. Besides, the stubble burning in Haryana-Punjab regions (N-W) influences the O3 390 concentration in urban and residential areas. Whereas the stubble burning in east i.e., IGP, influences the O3 391 concentration in industrial/commercial areas. The traffic areas are not much influenced by the long-range 392 transport of O3. The high concentration at night in traffic areas can be attributed to the downdraft of O3. 393 394 Acknowledgements 395 The author/authors gratefully acknowledge the ozone and meteorological data provided by the Delhi Pollution 396 Control Committee. 397 Appendix A. Supplementary Data 398 399 References 400 401 Agrawal, S. B., Singh, A., & Rathore, D. (2005). Role of ethylene diurea (EDU) in assessing impact of ozone 402 on Vigna radiata L. plants in a suburban area of Allahabad (India). Chemosphere, 61(2), 218-228. 403 Betts, A. K., Gatti, L. V., Cordova, A. M., Dias, M. A. S., & Fuentes, J. D. (2002). Transport of ozone to the 404 surface by convective downdrafts at night. Journal of Geophysical Research: Atmospheres, 107(D20), LBA405 13. Electronic copy available at: https://ssrn.com/abstract=4160065
406 Biswas, M. S., Ghude, S. D., Gurnale, D., Prabhakaran, T., & Mahajan, A. S. (2019). Simultaneous 407 observations of nitrogen dioxide, formaldehyde and ozone in the Indo-Gangetic Plain. Aerosol and Air 408 Quality Research, 19(8), 1749-1764.
409 Camalier, L., Cox, W., & Dolwick, P. (2007). The effects of meteorology on ozone in urban areas and their 410 use in assessing ozone trends. Atmospheric environment, 41(33), 7127-7137. 411 Chand, D., & Lal, S. (2004). High ozone at rural sites in India. Atmospheric Chemistry and Physics 412 Discussions, 4(3), 3359-3380.
413 Chelani, A.B., 2013. Study of extreme CO, NO2 and O3 concentrations at a traffic site in Delhi: Statistical 414 persistence analysis and source identification. Aerosol and Air Quality Research, 13(1), pp.377-384.
415 Debaje, S. B., & Kakade, A. D. (2006). Measurements of surface ozone in Rural site of India. Aerosol and 416 Air Quality Research, 6(4), 444-465.
417 Debaje, S. B., & Kakade, A. D. (2006). Weekend ozone effect over rural and urban site in India. Aerosol and 418 Air Quality Research, 6(3), 322-333. 419 Dreher, D. B., & Harley, R. A. (1998). A fuel-based inventory for heavy-duty diesel truck emissions. Journal 420 of the Air & Waste Management Association, 48(4), 352-358. 421 Elkamel, A., Abdul-Wahab, S., Bouhamra, W., & Alper, E. (2001). Measurement and prediction of ozone 422 levels around a heavily industrialized area: a neural network approach. Advances in environmental 423 research, 5(1), 47-59. 424 Escudero, M., Lozano, A., Hierro, J., del Valle, J., & Mantilla, E. (2014). Urban influence on increasing 425 ozone concentrations in a characteristic Mediterranean agglomeration. Atmospheric Environment, 99, 322426 332. 427 Ghude, S. D., Chate, D. M., Jena, C., Beig, G., Kumar, R., Barth, M. C., ... & Pithani, P. (2016). Premature 428 mortality in India due to PM2. 5 and ozone exposure. Geophysical Research Letters, 43(9), 4650-4658. 429 Gorai, A. K., Tchounwou, P. B., & Mitra, G. (2017). Spatial variation of ground level ozone concentrations 430 and its health impacts in an urban area in India. Aerosol and air quality research, 17(4), 951. 431 Goyal, P., Gulia, S., Goyal, S. K., & Kumar, R. (2019). Assessment of the effectiveness of policy 432 interventions for Air Quality Control Regions in Delhi city. Environmental Science and Pollution 433 Research, 26(30), 30967-30979.
434 Grange, S. K., Lewis, A. C., & Carslaw, D. C. (2016). Source apportionment advances using polar plots of 435 bivariate correlation and regression statistics. Atmospheric Environment, 145, 128-134. 436 Jain, S. L., Arya, B. C., Kumar, A., Ghude, S. D., & Kulkarni, P. S. (2005). Observational study of surface 437 ozone at New Delhi, India. International Journal of Remote Sensing, 26(16), 3515-3524. 438 Kalbande, R., Bano, S., & Beig, G. (2021). Benzene and Toluene from Stubble Burning and Their 439 Implications for Ozone Chemistry and Human Health in the Indo-Gangetic Plain Region. ACS Earth and 440 Space Chemistry, 5(11), 3226-3233. 441
Kasparoglu, S., Incecik, S., & Topcu, S. (2018). Spatial and temporal variation of O3, NO and NO2 442 concentrations at rural and urban sites in Marmara Region of Turkey. Atmospheric Pollution Research, 9(6), 443 1009-1020. 444 Khemani, L. T., Momin, G. A., Rao, P. S. P., Vijayakumar, R., & Safai, P. D. (1995). Study of surface ozone 445 behaviour at urban and forested sites in India. Atmospheric Environment, 29(16), 2021-2024. 446 Kumar, P., Gulia, S., Harrison, R. M., & Khare, M. (2017). The influence of odd–even car trial on fine and 447 coarse particles in Delhi. Environmental Pollution, 225, 20-30. 448 Kumari, S., Lakhani, A., & Kumari, K. M. (2020). First observation-based study on surface O3 trend in Indo449 Gangetic Plain: Assessment of its impact on crop yield. Chemosphere, 255, 126972. 450 Lal, S., Naja, M., & Subbaraya, B. H. (2000). Seasonal variations in surface ozone and its precursors over an 451 urban site in India. Atmospheric Environment, 34(17), 2713-2724. 452 Lal, S., Venkataramani, S., Naja, M., Kuniyal, J. C., Mandal, T. K., Bhuyan, P. K., ... & Kumar, M. K. S. 453 (2017). Loss of crop yields in India due to surface ozone: an estimation based on a network of 454 observations. Environmental Science and Pollution Research, 24(26), 20972-20981. 455 Li, L., An, J., Huang, L., Yan, R., Huang, C., & Yarwood, G. (2019). Ozone source apportionment over the 456 Yangtze River Delta region, China: Investigation of regional transport, sectoral contributions and seasonal 457 differences. Atmospheric environment, 202, 269-280. 458 Londhe, A. L., Jadhav, D. B., Buchunde, P. S., & Kartha, M. J. (2008). Surface ozone variability in the urban 459 and nearby rural locations of tropical India. Current science, 1724-1729. 460 Mahapatra, P. S., Jena, J., Moharana, S., Srichandan, H., Das, T., Chaudhury, G. R., & Das, S. N. (2012). 461 Surface ozone variation at Bhubaneswar and intra-corelationship study with various parameters. Journal of 462 earth system science, 121(5), 1163-1175. Electronic copy available at: https://ssrn.com/abstract=4160065
463 Mahapatra, P. S., Kumar, R., Mallik, C., Panda, S., Sahu, S. C., & Das, T. (2019). Investigation of a regional 464 ozone reduction event over eastern India by integrating in situ and satellite measurements with WRF-Chem 465 simulations. Theoretical and Applied Climatology, 137(1), 399-416.
466 Mahapatra, P. S., Panda, S., Walvekar, P. P., Kumar, R., Das, T., & Gurjar, B. R. (2014). Seasonal trends, 467 meteorological impacts, and associated health risks with atmospheric concentrations of gaseous pollutants at 468 an Indian coastal city. Environmental Science and Pollution Research, 21(19), 11418-11432. 469 Manes, F., Salvatori, E., La Torre, G., Villari, P., Vitale, M., Biscontini, D., & Incerti, G. (2008). Urban green 470 and its relation with air pollution: ecological studies in the Metropolitan area of Rome. italian Journal of 471 public health, 5(4), 278-283.
472 Marr, L. C., & Harley, R. A. (2002). Modeling the effect of weekday− weekend differences in motor vehicle 473 emissions on photochemical air pollution in central California. Environmental science & technology, 36(19), 474 4099-4106. 475 Marr, L. C., & Harley, R. A. (2002). Spectral analysis of weekday–weekend differences in ambient ozone, 476 nitrogen oxide, and non-methane hydrocarbon time series in California. Atmospheric Environment, 36(14), 477 2327-2335. 478 Mukherjee, A., Agrawal, S. B., & Agrawal, M. (2018). Intra-urban variability of ozone in a tropical city— 479 characterization of local and regional sources and major influencing factors. Air Quality, Atmosphere & 480 Health, 11(8), 965-977.
481 Nelson, B. S., Stewart, G. J., Drysdale, W. S., Newland, M. J., Vaughan, A. R., Dunmore, R. E., ... & Lee, J. 482 D. (2021). In situ ozone production is highly sensitive to volatile organic compounds in the Indian megacity 483 of Delhi. Atmospheric Chemistry and Physics Discussions, 1-36. 484 Paoletti, E., (2009). O3 and urban forests in Italy. Environmental pollution, 157(5), pp.1506-1512. 485 Perrino, C., Tiwari, S., Catrambone, M., Dalla Torre, S., Rantica, E., & Canepari, S. (2011). Chemical 486 characterization of atmospheric PM in Delhi, India, during different periods of the year including Diwali 487 festival. Atmospheric Pollution Research, 2(4), 418-427. 488 Pont, V. and Fontan, J., 2001. Comparison between weekend and weekday ozone concentration in large cities 489 in France. Atmospheric Environment, 35(8), pp.1527-1535.
490 Ramli, N.A., Ghazali, N.A. & Yahaya, A.S., (2010). Diurnal fluctuations of O3 concentrations and its 491 precursors and prediction of O3 using multiple linear regressions. Malaysian Journal of Environmental 492 Management, 11(2), pp.57-69. 493 Ravindra, K., Singh, T., Mor, S., Singh, V., Mandal, T.K., Bhatti, M.S., Gahlawat, S.K., Dhankhar, R., Mor, 494 S. and Beig, G.,2019. Real-timemonitoringofairpollutantsinseven citiesof NorthIndiaduring crop residue 495 burning and their relationship with meteorology and transboundary movement of air. Science of the total 496 environment, 690, pp.717-729 497 Saini,R.,Singh,P.,Awasthi,B.B.,Kumar,K.,&Taneja,A.(2014).Ozonedistributionsandurbanairquality 498 during summer in Agra–a world heritage site. Atmospheric Pollution Research, 5(4), 796-804. 499 Saxena, P., Srivastava, A., Verma, S., Singh, L. and Sonwani, S., 2020. Analysis of atmospheric pollutants 500 during fireworks festival ‘Diwali’at a residential site Delhi in India. In Measurement, analysis and 501 remediation of environmental pollutants (pp. 91-105). Springer, Singapore. 502
Sharma, A., Sharma, S. K., & Mandal, T. K. (2021). Ozone sensitivity factor: NOX or NMHCs?: a case study 503 over an urban site in Delhi, India. Urban Climate, 39, 100980. 504 Singh, A., Sarin, S.M., Shanmugam, P., Sharma, N., Attri, A.K. and Jain, V.K., 1997. Ozone distribution in 505 the urban environment of Delhi during winter months. Atmospheric Environment, 31(20), pp.3421-3427. 506
Sinha, P., Singh, S., Saroj, P., Beig, G., & Murthy, B. S. (2021). Study of surface ozone (O3) and its 507 relationship with NO, NO2, NOx, OX and CO at five different locations in New Delhi, India from 2013 to 508 2019.
509 Taha, H. (2008). Meso-urban meteorological and photochemical modeling of heat island 510 mitigation. Atmospheric Environment, 42(38), 8795-8809 511 Tu, J., Xia, Z. G., Wang, H., & Li, W. (2007). Temporal variations in surface ozone and its precursors and 512 meteorological effects at an urban site in China. Atmospheric Research, 85(3-4), 310-337. 513 Varshney, C.K. and Aggarwal, M., 1992. Ozone pollution in the urban atmosphere of Delhi. Atmospheric 514 Environment. Part B. Urban Atmosphere, 26(3), pp.291-294.
515 Verma, N., Lakhani, A., & Kumari, K. M. (2017). High ozone episodes at a semi-urban site in India: 516 photochemical generation and transport. Atmospheric Research, 197, 232-243.
517
Verma, N., Satsangi, A., Lakhani, A., & Kumari, K. M. (2018). Characteristics of surface ozone in Agra, a 518 sub-urban site in Indo-Gangetic Plain. Journal of Earth System Science, 127(3), 1-16..
Electronic copy available at: https://ssrn.com/abstract=4160065
519 Yadav, R., Sahu, L. K., Beig, G., & Jaaffrey, S. N. A. (2016). Role of long-range transport and local
520 meteorology in seasonal variation of surface ozone and its precursors at an urban site in India. Atmospheric
521 Research, 176, 96-107.
522 Zhu, X., Ma, Z., Qiu, Y., Liu, H., Liu, Q., & Yin, X. (2020). An evaluation of the interaction of morning
523 residual layer ozone and mixing layer ozone in rural areas of the North China Plain. Atmospheric
524 Research, 236, 104788.