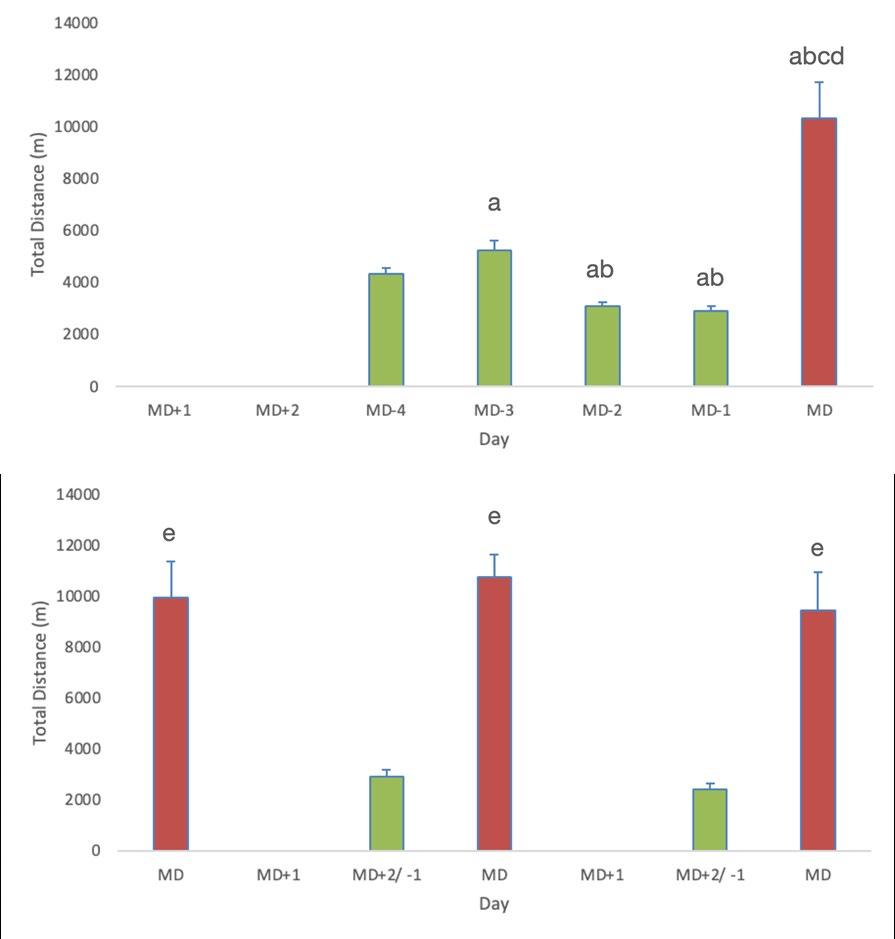
15 minute read
Fuelling for the Work Required in Professional Football: A Theoretical Model for Carbohydrate Periodisation
FUELLING FOR THE WORK REQUIRED IN
PROFESSIONAL FOOTBALL: A THEORETICAL MODEL FOR CARBOHYDRATE PERIODISATION
Advertisement
FEATURE / DR LIAM ANDERSON
Introduction
Professional football clubs are continuously posed with new questions that can often be answered through research and development. Whilst it is important to stay in tune with current literature to help answer these questions, it is also important to ask and answer questions that are specific to individual environments (i.e., players, management styles, training times, operating restrictions etc.). Going back almost 10 years, the questions we had before embarking on this research were “how much do these players need to eat each day?” and “what does players nutritional intake look like in comparison to recommended guidelines?”. We set about performing numerous investigations into the physical loading, energy expenditure and energy intake of professional football players with the end goal of being to provide more sophisticated nutritional programmes to meet energy expenditure and facilitate improvements in performance, recovery, and fitness adaptations.
Energy expenditure is a critical variable in human health and physiology 1, and implementing strategies to meet energy demands within elite football can be crucial for success and overall health of the player. Given that daily protein recommendations range from 1.6-2.2 g.kg-1 and recommended fat intakes equivalent to 30% energy intake 2, manipulation of carbohydrate intake would allow football players to meet differing daily energy demands. This type of periodised carbohydrate approach to sports nutrition has gained significant attention in endurance sports in recent years 3. Indeed, as part of answering the research questions mentioned above, our group and others have researched professional soccer players physical loading and energy requirements 2, 4-7. These new findings have significantly altered the approach that performance practitioners follow when providing training and nutritional recommendations.
The following article will summarise a theoretical model based off current scientific understanding of physical loading, energy expenditure and energy intake 8. This model, where carbohydrate is adjusted day-by-day and meal-by-meal according to the upcoming activity and the desired outcome of training sessions i.e., promoting exercise intensity vs stimulating metabolic adaptations, has been translated as a “fuel for the work required” model. Although this is a model that can be implemented into many football clubs, it is important to tailor to the individual training structure and demands (i.e., morning training vs. evening training, high vs. low demands on different days etc.).
Carbohydrate requirements for performance
Match demands have been widely accepted by practitioners and academics since the 1970s 9 . These demands have increased over time with distances covered at high-speed increasing ~30% from 2006-07 to 2012-13 in the English Premier League (EPL) and ~9% from 2012-13 to 2019-20 in the Spanish La Liga, heightening the importance of nutritional preparation for match play 10, 11. From a metabolic perspective, muscle glycogen is the major energy source to fuel these match demands. Krustup et al. 12 observed that pre-game muscle glycogen was 449 ± 23 mmol. kg-1 dry weight and decreased to 225 ± 23 mmol.kg-1 dry weight immediately after the match. This would suggest that there was sufficient glycogen available to continue exercising, however, analysis of individual muscle fibre (i.e., type IIa and IIx fibres) revealed that 50% of fibres could be classified as empty or almost empty. These fibres are responsible for sprinting and high-intensity activity. Therefore, muscle and liver glycogen are a potential contributing factor to fatigue and reduction in high intensity running towards the end of matches.
Figure 1: Playing squad average total distance completed in training sessions and matches during two different 7-day testing periods. Figure A = one game week, Figure B = three game week (games played with <72 hours apart) Red bars = matches and green bars = training. MD = Match day. a denotes difference from day MD-4, b denotes difference from day MD-3, c denotes difference from day MD-2, d denotes difference from day MD-1 and e denotes difference from MD+2/ -1, all P < 0.05. Figure adapted and redrawn from Anderson et al. 4.
Given the role of muscle glycogen promoting high-intensity intermittent exercise performance 13, 14, professional players should aim for high glycogen stores for a 24-hour period the day prior to match play 15. Daily intakes in this period should equate to 6-8 g.kg-1 to maximise their glycogen stores. This is providing that training intensity and duration is significantly reduced the day before match play (see Figure 1). In an analysis into players from the English Premier League we found players only consumed 4 g.kg-1 the day before a game (MD-1) 5 . Such values have shown reduced total distance covered on match day compared with 8 g.kg-1 16. Therefore, practitioners should be aware that MD-1 is a potential area where players can improve their current practise.
If players have correctly loaded muscle glycogen on the day prior to the game, they should arrive at the pre match meal with the goal to promote liver glycogen storage prior to match play. This is more evident in kick offs that are in the early afternoon or morning given that liver glycogen can be depleted by as much as 50% after an overnight fast and may not fully recover until early evening 17. The timing of this meal changes according to kick off time it is recommended that this meal be consumed 3-4 hours before kickoff and contain 1-3 g.kg-1 of carbohydrates. Professional players from the EPL reported intakes of 1-1.5 g.kg-1 in the pre-match meal, values that may be suboptimal for performance when considered with insufficient carbohydrate intakes the day prior to match play 6 .
Carbohydrate feeding during exercise is likely to improve elements of match day performance when fed at a rate of 3060g.hr-1 18, 19. Ingestion of carbohydrates at this rate improves physical performance 20, 21 as well as technical actions 22, 23 . Such rates of ingestion correspond to carbohydrate intake at the beginning (20-30g) and end of the warmup period (20-30g), the half-time period (2040 g) and if possible, the second half (20-30g). This strategy has not been experimentally tested and players should refine their individual strategy during training and friendly games. Similar to other aspects of carbohydrate intake for performance, players from the EPL do not readily achieve in-game carbohydrate guidelines and are certainly areas that can be addressed to further fuel for the demands of match play 6 .
Carbohydrate requirements for recovery
It is generally accepted that acute muscle glycogen re-synthesis requires players to consume 1.2 g.kg-1h-1 of high glycaemic carbohydrate for 3-4 hours to optimally facilitate short-term glycogen re-synthesis 24. It is important to note that this should begin immediately after match play (i.e., in the changing room or on the bench after coming off as a substitute) as this is when the muscle is most receptive to glucose uptake and glycogen synthesis 25. In addition, to promote liver glycogen resynthesis ingestion of sucrose over glucose prevails 26. Such data suggests that fructose containing foods and drinks would be beneficial in acute recovery from match play. Despite the clear evidence and benefits of this type of carbohydrate feeding for optimising muscle and liver glycogen resynthesis, this is a key area for improvement in many professional players. For example, when recovering from an 8:15 pm kick off EPL players reported <1 g.kg-1h-1 in the initial 2 h recovery period whilst carbohydrate intake in similar timescales at the 4:15 pm kick off increased to 1-1.5 g.kg-1h-1 6. Whilst the reasons for this aren’t definitive, provisions are required to be put into place for players during such scenarios to obtain optimal recovery from match play (i.e., recovery pack or postmatch team meal).
Muscle glycogen can take up to 24-72 h after exercise to completely replenish after match play, therefore there is a requirement to consume adequate carbohydrate intake on the day(s) after the match. Players should be aware of this even if the day following match play is a day off or with reduced time within the club due to only performing recovery exercises. In the example of EPL players carbohydrate intakes in the days following match play were ~4 g.kg-1 5. Intakes as low as this would be considered insufficient in facilitating glycogen resynthesis as it took cohorts of Danish 2-3 days to fully recover after 6-9 g.kg-1 carbohydrate intake 27, 28. This clearly highlights the requirement for high carbohydrate intake for recovery from match play, in particular when time between matches is limited (i.e., congested fixture periods; see Figure 1).
Carbohydrate requirements of soccer training
In contrast to the match demands, typical training loads of soccer players have only recently been examined with a variety of metrics now common place in the literature and used within the applied environment 8. A review of the current available literature identifies that absolute training loads are not near as high as experienced in match play with the highest load being performed in the middle of the week (i.e., MD-4, MD-3; see Figure X). Unlike match play (and potentially due to the highly variable demands), the glycogen cost of actual training sessions has not yet been studied. In a soccer-simulated training protocol, Jeong et al. 29 reported glycogen depletion of only 20%. Although this protocol is limited due to the omission of technical actions and changes of direction etc. Such data considered in combination with real-world training load data suggest that the glycogen cost of training is not near that of match play. Therefore, the principle of “fuelling for the work required” from endurance sports, where carbohydrate intake is adjusted on a day-by-day and meal-by meal manner according to the metabolic demands of the upcoming training sessions seems a relatable model for professional football players 3 .
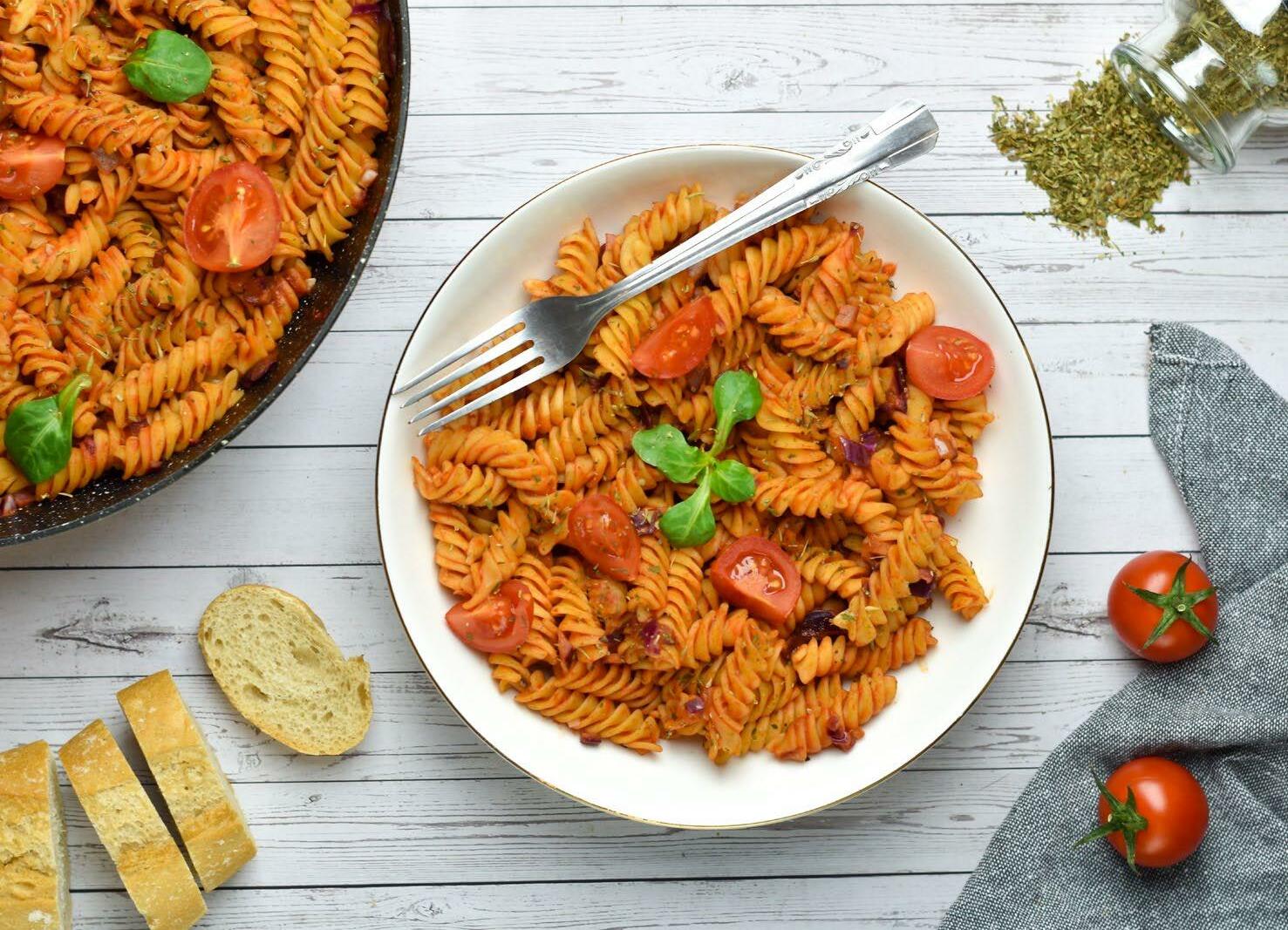
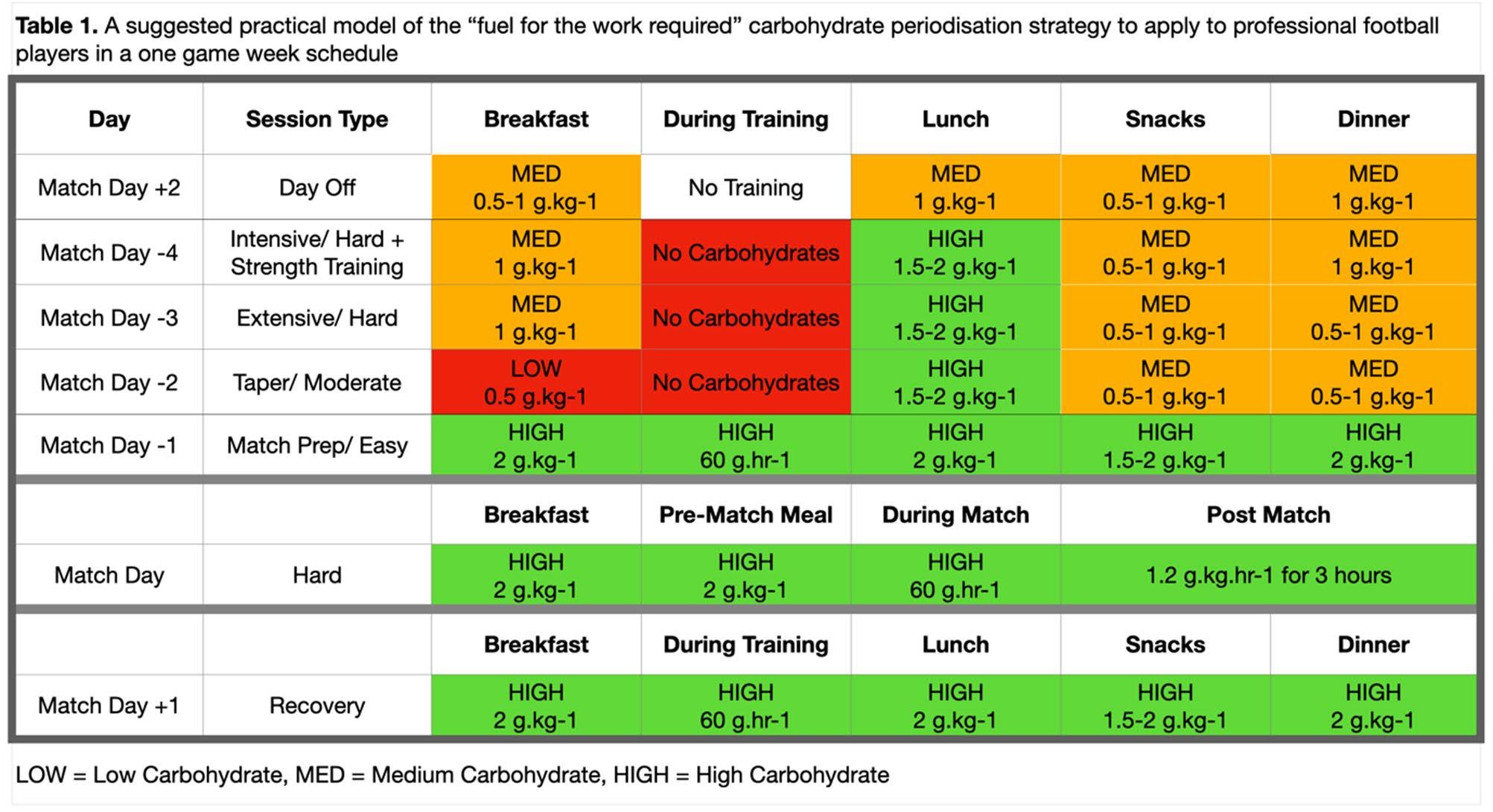
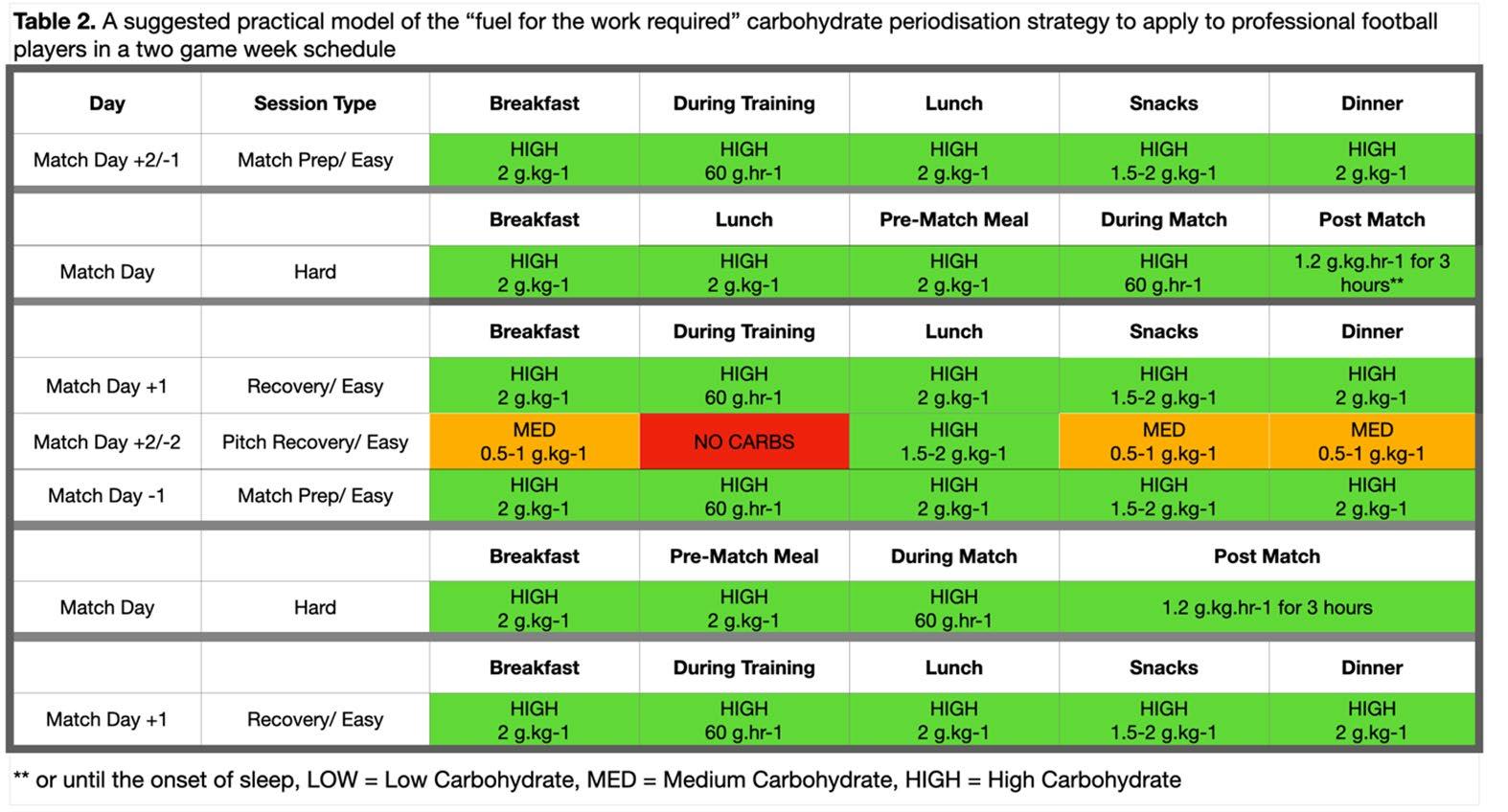
The rationale for the development of carbohydrate periodisation strategies is further enhanced on the premise that commencing and/or recovering from exercise with reduced carbohydrate availability up-regulated cell signalling pathways that regulate oxidative adaptations of human skeletal muscle 30, 31, the result of which may culminate in enhanced training adaptations and improved exercise performance. It is noteworthy that professional players tend to already adopt a form of carbohydrate periodisation across the weekly microcyle. For example, we observed intakes of 4 g.kg-1 on training days and X g.kg-1 on match days in EPL players 5. These data are comparable with Dutch Eredivisie Players 7. Additionally, utilising the gold standard for assessing free living energy expenditure, the energy expenditure of professional players are approximately 3000-3500 kcal.d-1, equivalent to ~4855 kcal.kg-1 lean body mass 6, 7. Given that daily protein recommendations (i.e., 1.6-2 g.kg-1) and recommended fat intakes equivalent to 30% of total energy intake 2, it is reasonable to suggest that average daily carbohydrate intakes of 3-6 g.kg-1 during training days (that are not aligned to MD-1 or MD+1) would be sufficient to meet daily energy requirements that encompass the typical range in daily training intensity and duration associated with in season training schedules.
Practical carbohydrate periodisation strategy
Based on the fuel for the work required approach and assessments of energy expenditure in professional players, we provide a theoretical overview of day-by-day and meal-by-meal carbohydrate intakes in both a 1 game week (see Table 1) and 2 game week scenario (see Table 2). This model intends to provide players who partake in a “morning” training session and only one on field session per day. Both weeks have the aim of facilitating performance and recovery with nutritional strategies in the 1-game week also aiming to augment fitness adaptations during training days. It is also of note that this strategy is for adult male players who play a significant portion (i.e., >60 minutes) of match play and are involved in every training session. If players are not receiving significant match minutes and/ or are not involved in all training sessions, then training and nutritional strategies are to be revised.
Conclusion
Professional football clubs should aim to answer their own performance questions to evolve and develop practises. It is important to set out on a journey to answer these questions in a thorough but efficient manner. Presented in this article are some theoretical nutritional guidelines that operate on a day-by-day and meal-by-meal approach to carbohydrate feeding in both a 1 and 2 game week. Although this theoretical model is based on scientific understanding, it should be tested in both in the applied and laboratory environments. Practitioners may also tweak the model to suit their own individual scenarios (i.e., rest day in the middle of the week and/ or evening training sessions).
Acknowledgements
It is important to acknowledge Professors James Morton, Barry Drust and Graeme Close in the creation of the published manuscript 8. Although they weren’t directly involved in the creation of this article, they were integral for the completion of the publication.
1. Pontzer H, Yamada Y, Sagayama H, et al. Daily energy expenditure through the human life course. Science 2021; 373: 808-812. DOI: 10.1126/science.abe5017. 2. Collins J, Maughan RJ, Gleeson M, et al. UEFA expert group statement on nutrition in elite football. Current evidence to inform practical recommendations and guide future research. Br J Sports Med 2021; 55: 416. 20201023. DOI: 10.1136/ bjsports-2019-101961. 3. Impey SG, Hammond KM, Shepherd SO, et al. Fuel for the work required: a practical approach to amalgamating trainlow paradigms for endurance athletes. Physiol Rep 2016; 4. DOI: 10.14814/phy2.12803. 4. Anderson L, Orme P, Di Michele R, et al. Quantification of training load during one-, two- and three-game week schedules in professional soccer players from the English Premier League: implications for carbohydrate periodisation. J Sports Sci 2016; 34: 1250-1259. 2015/11/05. DOI: 10.1080/02640414.2015.1106574. 5. Anderson L, Orme P, Naughton RJ, et al. Energy Intake and Expenditure of Professional Soccer Players of the English Premier League: Evidence of Carbohydrate Periodization. Int J Sport Nutr Exerc Metab 2017; 27: 228-238. 2017/01/05. DOI: 10.1123/ijsnem.2016-0259. 6. Anderson L, Naughton RJ, Close GL, et al. Daily Distribution of Macronutrient Intakes of Professional Soccer Players From the English Premier League. Int J Sport Nutr Exerc Metab 2017; 27: 491-498. 20170628. DOI: 10.1123/ijsnem.2016-0265. 7. Brinkmans NYJ, Iedema N, Plasqui G, et al. Energy expenditure and dietary intake in professional football players in the Dutch Premier League: Implications for nutritional counselling. J Sports Sci 2019; 37: 2759-2767. 20190216. DOI: 10.1080/02640414.2019.1576256. 8. Anderson L, Drust B, Close GL, et al. Physical loading in professional soccer players: Implications for contemporary guidelines to encompass carbohydrate periodization. J Sports Sci 2022; 40: 1000-1019. 20220306. DOI: 10.1080/02640414.2022.2044135. 9. Reilly T and Thomas V. A motion analysis of work-rate in different positional roles in professional football match-play. Journal of Human Movement Studies 1976; 2: 87-97. 10 . Barnes C, Archer DT, Hogg B, et al. The evolution of physical and technical performance parameters in the English Premier League. Int J Sports Med 2014; 35: 1095-1100. 20140710. DOI: 10.1055/s-0034-1375695. 11. Lago-Penas C, Lorenzo-Martinez M, Lopez-Del Campo R, et al. Evolution of physical and technical parameters in the Spanish LaLiga 2012-2019. Sci Med Footb 2022: 1-6. 20220307. DOI: 10.1080/24733938.2022.2049980. 12. Krustrup P, Mohr M, Steensberg A, et al. Muscle and blood metabolites during a soccer game: implications for sprint performance. Med Sci Sports Exerc 2006; 38: 1165-1174. DOI: 10.1249/01.mss.0000222845.89262.cd. 13. Balsom PD, Wood K, Olsson P, et al. Carbohydrate intake and multiple sprint sports: with special reference to football (soccer). Int J Sports Med 1999; 20: 48-52. DOI: 10.1055/s-2007-971091. 14. Saltin B. Metabolic fundamentals in exercise. Med Sci Sports 1973; 5: 137-146. 15. Bussau VA, Fairchild TJ, Rao A, et al. Carbohydrate loading in human muscle: an improved 1 day protocol. Eur J Appl Physiol 2002; 87: 290-295. 20020528. DOI: 10.1007/s00421-002-0621-5. 16. Souglis AG, Chryssanthopoulos CI, Travlos AK, et al. The effect of high vs. low carbohydrate diets on distances covered in soccer. J Strength Cond Res 2013; 27: 2235-2247. DOI: 10.1519/ JSC.0b013e3182792147. 17. Iwayama K, Tanabe Y, Tanji F, et al. Diurnal variations in muscle and liver glycogen differ depending on the timing of exercise. J Physiol Sci 2021; 71: 35. 20211121. DOI: 10.1186/s12576-021-00821-1. 18. Baker LB, Rollo I, Stein KW, et al. Acute Effects of Carbohydrate Supplementation on Intermittent Sports Performance. Nutrients 2015; 7: 5733-5763. 20150714. DOI: 10.3390/nu7075249. 19. Russell M and Kingsley M. The efficacy of acute nutritional interventions on soccer skill performance. Sports Med 2014; 44: 957-970. DOI: 10.1007/s40279-014-0184-8. 20. Rodriguez-Giustiniani P, Rollo I, Witard OC, et al. Ingesting a 12% Carbohydrate-Electrolyte Beverage Before Each Half of a Soccer Match Simulation Facilitates Retention of Passing Performance and Improves High-Intensity Running Capacity in Academy Players. Int J Sport Nutr Exerc Metab 2019; 29: 397-405. 20190701. DOI: 10.1123/ ijsnem.2018-0214. 21. Harper LD, Stevenson EJ, Rollo I, et al. The influence of a 12% carbohydrate-electrolyte beverage on self-paced soccer-specific exercise performance. J Sci Med Sport 2017; 20: 1123-1129. 20170421. DOI: 10.1016/j.jsams.2017.04.015. 22. Currell K, Conway S and Jeukendrup AE. Carbohydrate ingestion improves performance of a new reliable test of soccer performance. Int J Sport Nutr Exerc Metab 2009; 19: 34-46. DOI: 10.1123/ ijsnem.19.1.34. 23. Russell M, Benton D and Kingsley M. Influence of carbohydrate supplementation on skill performance during a soccer match simulation. J Sci Med Sport 2012; 15: 348-354. 20120109. DOI: 10.1016/j.jsams.2011.12.006. 24. Burke LM, van Loon LJC and Hawley JA. Postexercise muscle glycogen resynthesis in humans. J Appl Physiol (1985) 2017; 122: 1055-1067. 20161027. DOI: 10.1152/ japplphysiol.00860.2016. 25. Ivy JL, Katz AL, Cutler CL, et al. Muscle glycogen synthesis after exercise: effect of time of carbohydrate ingestion. J Appl Physiol (1985) 1988; 64: 1480-1485. DOI: 10.1152/ jappl.1988.64.4.1480. 26. Fuchs CJ, Gonzalez JT, Beelen M, et al. Sucrose ingestion after exhaustive exercise accelerates liver, but not muscle glycogen repletion compared with glucose ingestion in trained athletes. J Appl Physiol (1985) 2016; 120: 1328-1334. 20160324. DOI: 10.1152/japplphysiol.01023.2015. 27. Gunnarsson TP, Bendiksen M, Bischoff R, et al. Effect of whey protein- and carbohydrate-enriched diet on glycogen resynthesis during the first 48 h after a soccer game. Scand J Med Sci Sports 2013; 23: 508-515. 20111123. DOI: 10.1111/j.16000838.2011.01418.x. 28. Krustrup P, Ortenblad N, Nielsen J, et al. Maximal voluntary contraction force, SR function and glycogen resynthesis during the first 72 h after a high-level competitive soccer game. Eur J Appl Physiol 2011; 111: 2987-2995. 20110330. DOI: 10.1007/s00421-011-1919-y. 29. Jeong TS, Bartlett JD, Joo CH, et al. Acute simulated soccerspecific training increases PGC-1alpha mRNA expression in human skeletal muscle. J Sports Sci 2015; 33: 1493-1503. 20141223. DOI: 10.1080/02640414.2014.992937. 30. Morton JP, Croft L, Bartlett JD, et al. Reduced carbohydrate availability does not modulate training-induced heat shock protein adaptations but does upregulate oxidative enzyme activity in human skeletal muscle. J Appl Physiol (1985) 2009; 106: 1513-1521. 20090305. DOI: 10.1152/ japplphysiol.00003.2009. 31. Bartlett JD, Hawley JA and Morton JP. Carbohydrate availability and exercise training adaptation: too much of a good thing? Eur J Sport Sci 2015; 15: 3-12. 20140619. DOI: 10.1080/17461391.2014.920926.