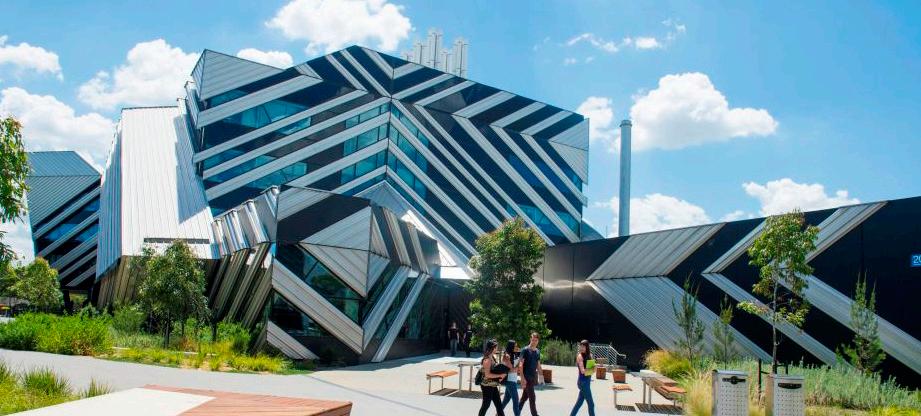
11 minute read
Firing Up the Next Generation: Monash University Puts Students in the Spotlight
Source: Sally Wood
Australia’s future relies on selecting materials that boast strength, durability and longevity.
From building roads and public transport to recycling and renewable energy; scientists are paving the way for the next generation.
These engineering projects, and the decisions behind them would not be possible without innovation in materials science.
At Monash University, researchers and students alike are focussed on this journey.
The Department of Materials Science and Engineering works on making things stronger, lighter, more functional, sustainable, and costeffective to meet the demands of the future.
Scientists work across six research themes:
• Additive manufacturing
• Biomaterials
• Functional and energy materials
• Metals and alloys
• Polymers
• Materials theory, modelling and characterisation
Professor Neil Cameron is the Head of the Department of Materials Science and Engineering.
His research focuses on materials chemistry and biology, specifically on the development of novel polymeric biomaterials for applications in regenerative medicine and drug delivery.
Bringing these bold ideas to life requires access to state-of-the-art facilities and partnerships.
Solving Real-World Challenges
Monash University has partnered with Woodside Energy to work towards a lower-carbon economy.
The $40 million partnership transforms research from the lab into commercially viable outcomes.
At the globally-connected FutureLab, researchers are tasked with three main priorities: developing affordable, bulk clean energy; profitable carbon abatement; and thought leadership as Australia transitions to renewable energy sources.
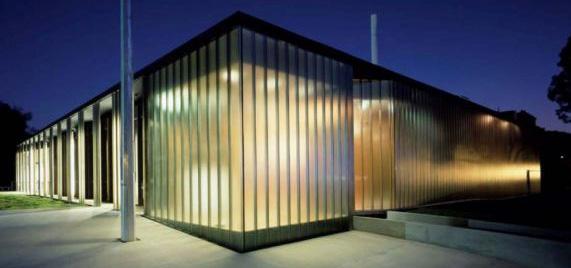
This interactive environment is ideal for researchers and practitioners who work together towards end-user driven research.
Australia is on track to become the world’s second largest net-exporter of low-emissions hydrogen by 2030.
As such, the Woodside-Monash Energy Partnership is well-positioned to leverage their expertise and take advantage of this sector.
The partnership is focussing on the forces influencing hydrogen energy adoption for business customers, and the future role that hydrogen hubs may play in the energy transition process.
Solid Oxide Electrolysis (SOEC) is one of the techniques studied at Monash.
The SOEC method uses water splitting and carbon dioxide conversion to bring hydrogen production to life. It offers exciting potential for future research, which seeks to fill the gaps in Australia’s current energy market.
The Minster for Climate Change and Energy Chris Bowen said Australia is ideally placed to help power the world with renewable energy.
“The whole world needs renewable hydrogen, and regional Australia is ready to provide it.”
“Renewable hydrogen is a game changer, opening the door to green metals, green fertiliser, green power and supporting industrial decarbonisation,” he said.
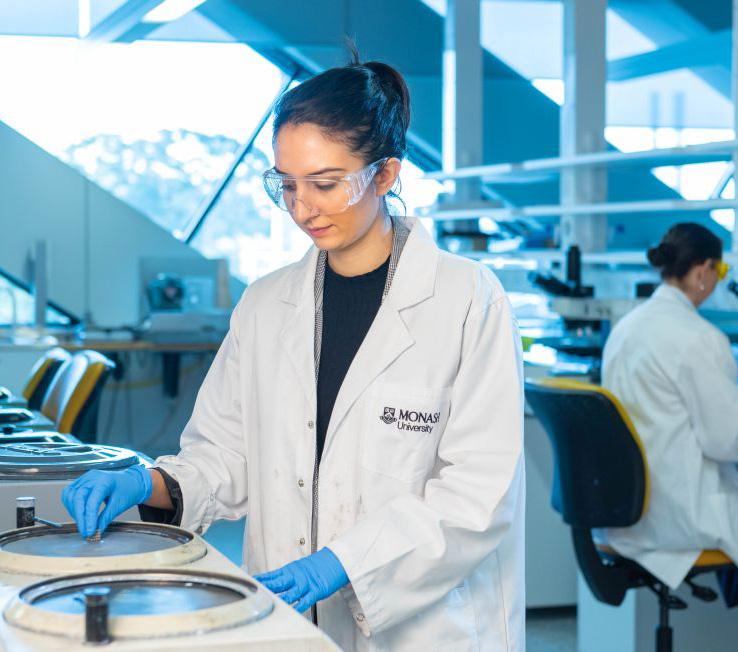
The project is one piece of the puzzle when it comes to developing innovative responses to real-world challenges.
Students Leading the Way
The Department of Materials Science and Engineering is one of the few institutions offering undergraduate courses in Australia. This is crucial to secure and promote a fresh pipeline of researchers into this field.
The science and engineering sectors are multi-disciplinary in nature. As such, Monash students learn to collaborate with their peers to challenge existing ways of thinking and push boundaries to deliver outcomes.
Students learn about a variety of materials science, including:
• Metals and alloys
• Biomaterials
• Nanomaterials
• Polymers
• Ceramics
• Corrosion and composites
• Materials characterisation and modelling
The Biomedical Science and Materials Engineering degree is a popular choice for incoming students, as the department is well-aligned with research centres and end-user organisations.
Many students find comfort knowing their degree will lead to employment. In fact, many students attain a job during their studies, usually in their final year. This is where students put their skills to the test in a real-world scientific environment.
Researchers “Bioprint” Living Brain Cell Networks in the Lab
Monash University Engineering researchers recently used ‘bioinks’ containing living nerve cells (neurons) to print 3D nerve networks.
Grown in the laboratory, these networks can transmit and respond to nerve signals.
The engineers used a tissue engineering approach, and bioprinting with two bioinks containing living cells and non-cell materials respectively.
Then, they were able to mimic the arrangement of grey matter and white matter seen in the brain.
Professor John Forsythe from the Department of Materials Science and Engineering, who is leading the research, said two-dimensional nerve cell cultures have previously been used to study the formation of nerve networks and disease mechanisms.
However, those relatively flat structures do not reflect the way neurons grow and interact with their surroundings.
“The networks grown in this research closely replicated the 3D nature of circuits in a living brain, where nerve cells extend processes called neurites to form connections between different layers of the cortex.”
“We found that the projections growing from neurons in the printed ‘grey matter’ or cellular layer readily grew through the ‘white matter’ layer and used it as a ‘highway’ to communicate with neurons in other layers,” Professor Forsythe said.
Researchers found the neurons behaved and performed in a similar manner.
Sensitive electrophysiological measurements confirmed spontaneous nerve-like activity taking place in the 3D neuronal networks in addition to responses evoked by electrical and drug stimulation.
The presence of detectable electrical activity in tissue engineered 3D networks represents a significant step forward in the field of neuroscience and bioprinting.
Bioprinted 3D neural networks are likely to be a promising platform for studying how nerves and nerve networks form and grow, investigating how some diseases affect neurotransmission, and screening drugs for their effects on nerve cells and the nervous system.
The study was recently published in Advanced Healthcare Materials.
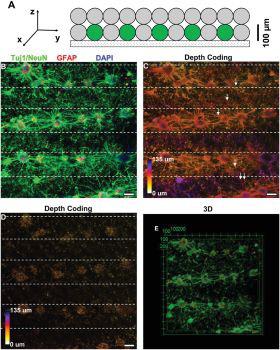
Bioprinted cortical neurons and astrocytes after in vitro culture at 7DIV. A) Cross-section view of bioprinted structure consisting of cellular (green) and acellular (grey) strands. B) Maximum intensity projection of patterned structure: cellular–acellular–cellular. Neurons (NeuN, green), astrocytes (GFAP, red), and nuclei (DAPI, blue) were colocalized and developed complex structures. Axons (Tuj1, green) originating from the proximal cellular strands projected across the distal cellular strand. C) Depth-coding of Tuj1/NeuN showed the development of axonal projections across strands. White arrows indicate neurites that belong to different focal planes. D) Depth-coding of DAPI shows localization of cell nuclei. E) Confocal image in 3D. Depth-coding was represented using a color bar: red, closest to the glass substrate, and blue, 135 µm away from the substrate. Scale bar, 100 µm.
Nanodiamonds Could Hold Key to Cool Clothing
Researchers from RMIT University are using nanodiamonds to create smart textiles that can cool people down faster.
The study found fabric made from cotton coated with nanodiamonds, using a method called electrospinning, showed a reduction of 2-3 degrees Celsius during the cooling down process compared to untreated cotton. They do this by drawing out body heat and releasing it from the fabric as a result of the incredible thermal conductivity of nanodiamonds.
Project Lead and Senior Lecturer, Dr Shadi Houshyar, said there was a big opportunity to use these insights to create new textiles for sportswear and even personal protective clothing, such as underlayers to keep fire fighters cool.
The study also found nanodiamonds increased the UV protection of cotton, making it ideal for outdoor summer clothing. The use of this fabric in clothing was projected to lead to a 20-30% energy saving due to lower use of air conditioning.
Based in the Centre for Materials Innovation and Future Fashion (CMIFF), the research team is made up of RMIT engineers and textile researchers.
“They’re actually cheap to make cheaper than graphene oxide and other types of carbon materials,” Dr Houshyar said.
Cotton material was first coated with an adhesive, then electrospun with a polymer solution made from nanodiamonds, polyurethane and solvent. This process creates a web of nanofibres on the cotton fibres, which are then cured to bond the two.
Further research will study the durability of the nanofibres, especially during the washing process.
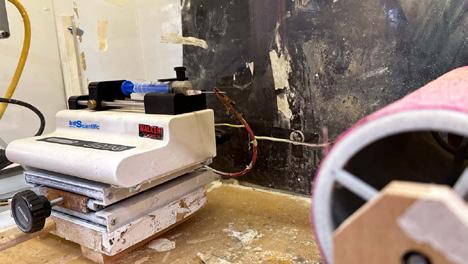
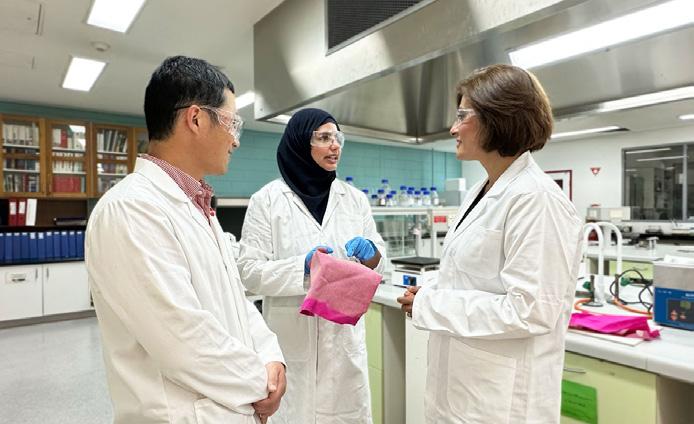
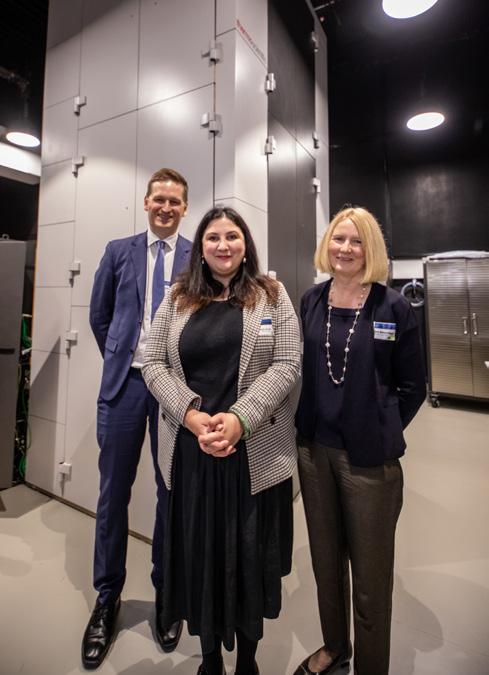
Melbourne Home to One-Of-A-Kind Electron Microscope
A large Australian team led by Monash University has devised an approach to killing antibiotic-resistant bacteria. Researchers used lipid nanoparticles that target specific layers on the surface of the bacterial cell.
It shows antibacterial lipids can be successfully used in combination with nanocarrier lipids to form nanoparticles that kill gram-negative bacteria.
Instrument scientist and co-author Dr Anton Le Brun contributed to the research with measurements on the neutron reflectometer Platypus and analysis of the data.
“Neutron reflectometry is a useful tool for understanding the structure of cell membranes at the nanometre length scale.”
The Platypus instrument at the Australian Centre for Neutron Scattering was used to elucidate the mechanism at work in a combined ML-niosome/polymyxin B treatment at the molecular level.
Niosomes are vesicles with special properties that are used to deliver drugs.
Meanwhile, polymyxin B is an antibiotic of last resort for treating infections from gram-negative bacteria. Some bacteria have started to show signs of resistance even to this antibiotic.
By making artificial membranes that mimic the properties of the gram-negative bacterial cell surface, the team discovered the ML-niosomes target the outer layer of the outer membrane, which is mainly composed of polysaccharides.
The binding of the ML-niosomes to the surface of the outer membrane exposes the membrane.
This grants polymyxin B better access to attack and breakdown the protective outer membrane and then the inner membrane, which ultimately kills the bacterial cell.
Future work will investigate how this is achieved in detail at the molecular level and why the combination with polymyxin B is more effective.
Mini-brains and Lab-On-A-Chip Wearables: Jumar Bioincubator Officially Opens Within Melbourne Biomedical Precinct
Australia’s newest biotech incubator Jumar Bioincubator has officially opened its doors and revealed the first 16 innovative early-stage ventures to take up residency in its much awaited Melbourne based facility.
The state-of-the-art facilities, infrastructure, and support offered by Jumar creates a world class hub for biotech innovation that will help to progress discoveries towards realworld patient treatments.
The official opening was hosted by founding partners CSL, WEHI, and The University of Melbourne, as well as initial investor Breakthrough Victoria and operator Cicada Innovations.
Ken Jefferd, Managing Director of Research, Innovation and Commercialisation at the University of Melbourne, said Jumar’s launch was a great example of how Melbourne was continuing to evolve support to world-class researchers.
Guests at the launch, including biotech leaders who were introduced to residents working on health issues across pharmaceuticals, diagnostics, medical devices, bioinformatics, health-related AI and more.
Some of these leaders included biotech company Denteric, which is developing a therapeutic vaccine for the one billion people globally suffering from periodontal gum disease.
Ovulation bio-sensing startup Symex Labs, which has developed a wearable “lab-on-a-chip” solution that provides continuous “set-and-forget” monitoring of hormones to more conveniently and effectively predict ovulation for people wanting to conceive.
Regenerative medicine company Tessara Therapeutics is creating “mini brains” in test tubes through 3D neural microtissues that mimic the human brain and offer all the essential requirements for drug discovery, which will help fast-track the ability to find cures for neurodivergent diseases like dementia.
Through its support of the next generation of biotech startups bringing innovative health solutions like these to market, Jumar is enabling the research translation that will ensure Australia’s world-leading research results in real-world patient outcomes.
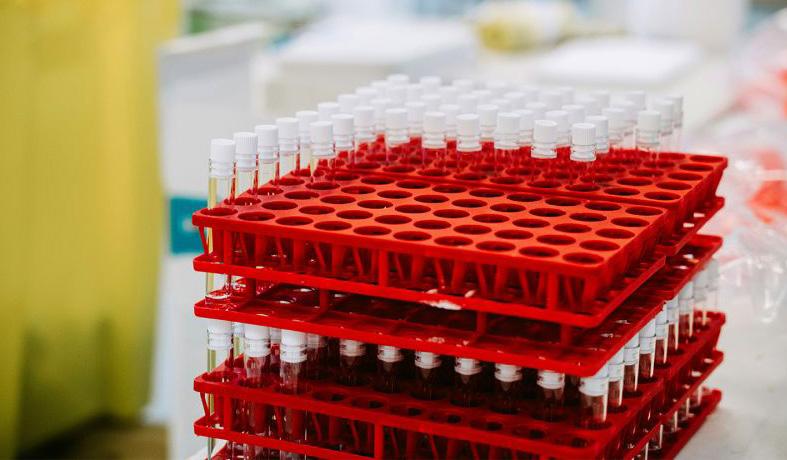
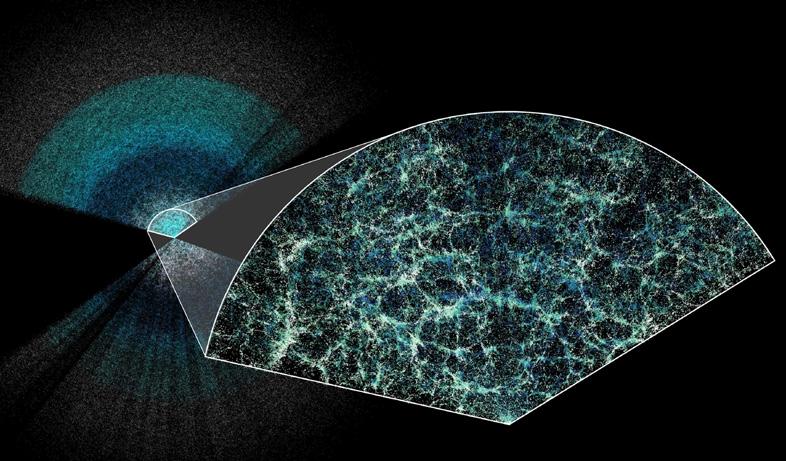
Scientists have created the largest 3D map of our universe to date. Earth is at the centre of this thin slice of the full map and the magnified section shows the underlying structure of matter in our universe. Each dot is a different galaxy similar in size to our own Milky Way. Image Credit: Claire Lamman/DESI collaboration; custom colormap package by cmastro.
Scientists Create 3D Map of Universe
An international team of researchers has created the world's largest and most detailed 3D map of the universe measuring the expansion of the cosmos over the past 11 billion years.
Dr Cullan Howlett from The University of Queensland helped develop pivotal software used for analysing data collected as part of the Dark Energy Spectroscopic Instrument (DESI).
Galaxies and massive celestial objects have been mapped with unprecedented detail, creating the largest map of the universe ever constructed. UQ researchers developed the key software used for analysing and modelling remnant sound waves from the early universe. The information allowed researchers to study how the universe has evolved over time and measure the effect of dark energy.
Using the new data, the DESI collaboration has made the most precise measurements to date of how fast the universe has expanded throughout history.
“The team at UQ was responsible for developing one of the key pieces of software used for analysing the survey data, which helps search for a very specific feature in the map,” Dr Howlett said.
The 3D map is comprised of the spatial coordinates and distances of millions of galaxies. Researchers can measure the longitudinal and latitudinal position of each galaxy, as well as its unique light ‘fingerprint’ observed by measuring the presence of chemical elements like hydrogen, oxygen, and nitrogen.
“We decoded that fingerprint, identified the individual elements, and compared the measured frequencies to those in a lab on Earth to get the distance from us,” Dr Howlett said.
The 3D map is comprised of the spatial coordinates and distances of millions of galaxies.
CSIRO Achieves Record Efficiency for Next-Gen Roll-ToRoll Printed Solar Cells
Scientists from Australia’s national science agency, CSIRO, have led an international team to a clean energy breakthrough by setting a new efficiency record for fully roll-to-roll printed solar cells.
Printed onto thin plastic films, this lightweight and flexible solar technology will help meet the growing demand for renewable energy, expanding the boundaries of where solar cells can be used.
Where silicon solar panels are rigid and heavy, the printed solar cells are flexible and portable, being used in previously unimaginable ways across urban construction, mining operations and many other key industries.
CSIRO’s Renewable Energy Systems Group Leader, Dr Anthony Chesman, said the achievement was the result of more than a decade’s research and development. “CSIRO’s thin and light-weight solar cells are now on the cusp of emerging from the lab to create clean energy in the real world.”
The results were achieved in collaboration with researchers from the University of Cambridge, Monash University, the University of Sydney and the University of New South Wales and have been published in the leading journal Nature Communications.
CSIRO Principal Research Scientist Dr Doojin Vak said that an automated system produced a comprehensive dataset that will pave the way to use machine learning in future research.
“We developed a system for rapidly producing and testing over ten thousand solar cells a day – something that would have been impossible to do manually,” Dr Vak said.
CSIRO is actively seeking industry partners to further develop and commercialise this technology.
This activity received funding from ARENA as part of ARENA’s Research and Development Program – Solar PV Research.
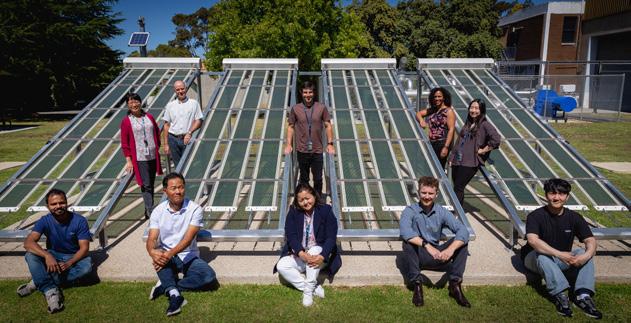
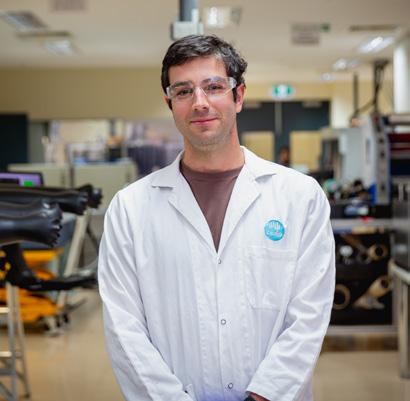
More than a decade’s research and development has culminated in this world-first efficiency outcome for CSIRO’s printable flexible solar team.
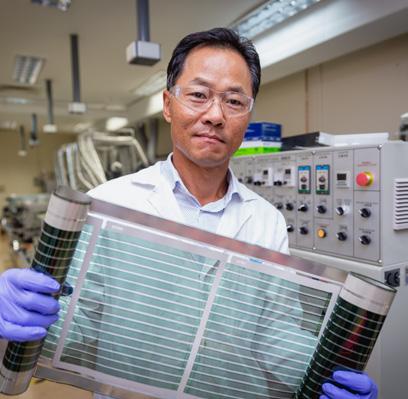
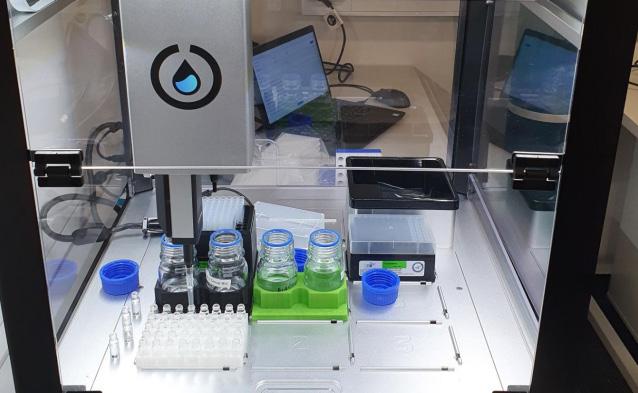
Dr Nicole Rijs and her team used robotics, including custom designed parts, to mix high loads of chemical reactions to form supramolecules.
Photo: Oscar Lloyd Williams.
The Chemistry AI Revolution: Developing a High Throughput Technique to Analyse Complex Chemical Structures
A new method for analysing the complexity of chemical reactions, using a technique known as ion mobility mass spectrometry, coupled with simple machine learning, has been developed by scientists from the UNSW School of Chemistry.
The new method could be used by scientists who study supramolecules in a range of research fields from drug discovery to material design.
“In our case, we’re building supramolecules using molecular subunits,” said Dr Nicole Rijs, a lead author on the study.
Dr Rijs and her team set out to develop a way to analyse the complexity of these selfassembling supramolecules that was efficient and high throughput. And to achieve such a feat, they turned to AI.
Dr Rijs, together with PhD candidate Oscar Williams and their team, used a liquid-handling robot to prepare over 800 reactions between different metals and other reactants, which spontaneously produced more supramolecular assemblies.
This data was then fed into an machine learning algorithm which determines how different the supramolecules are from each other, developing a technique which identifies parts of complex chemical mixtures that are often invisible to other techniques.
“We also can get that chemical information out and digitise it, and we made an efficient way that lets the information be fed in to machine learning.
“Such data can now be generated at scale and used as a training set for AI, which is important because these systems currently lack good chemical training sets.”