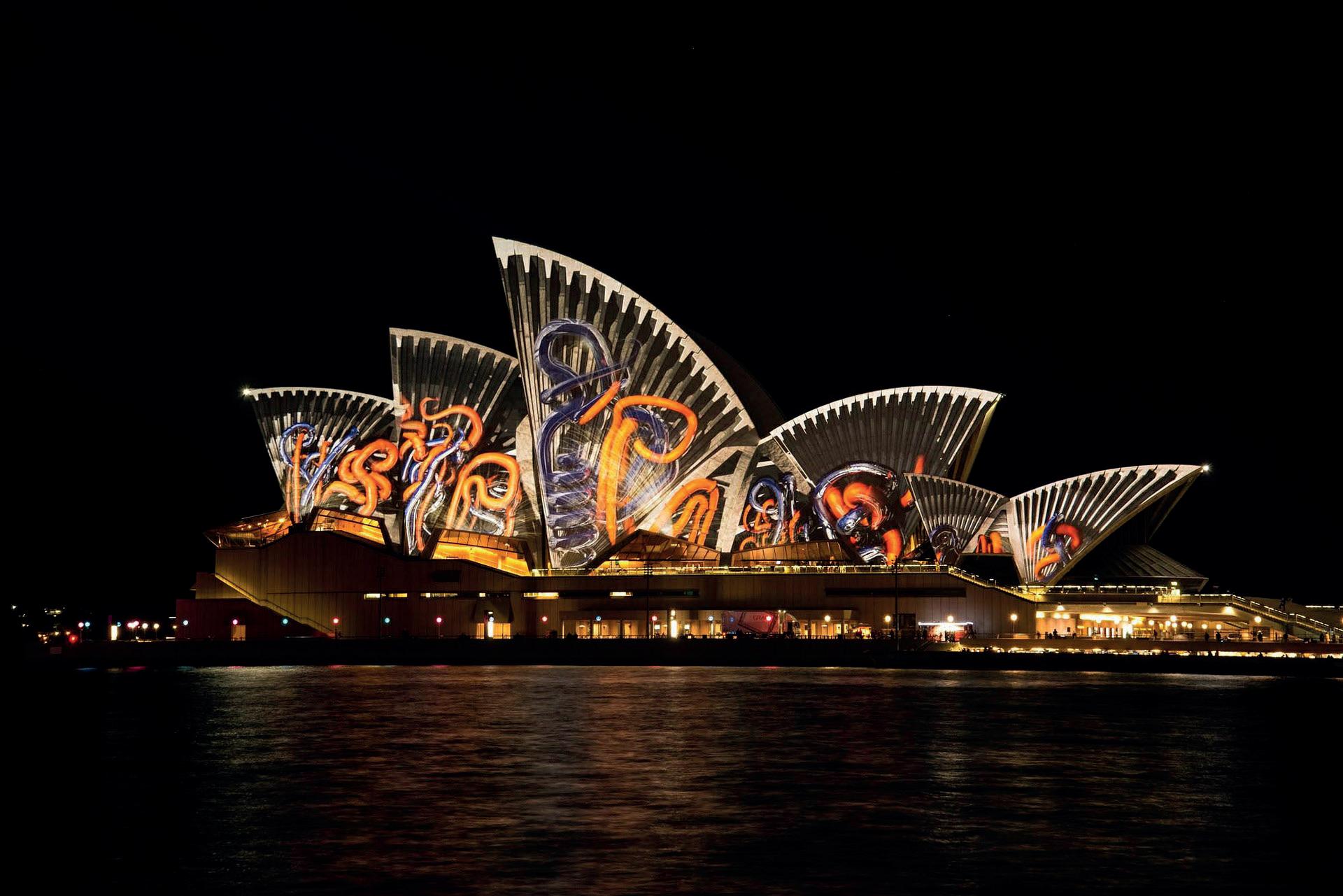
10 minute read
ON THE RIGHT WAVELENGTH
Alp Durmus outlines the concept of an adaptive optimisable lighting system and examines the applications
Worldwide energy consumption contributes to two of the biggest challenges facing humanity: resource scarcity and climate change. Pressured by the increasing energy demand of a growing population, governments, manufacturers and the lighting industry have been tackling this issue by promoting energy-efficient light sources, such as solid-state lighting (SSL) devices. SSL devices offer several advantages over previous lighting technologies, such as compact size, longer lifetime, directionality, high efficacy, ease of control of light output and high colour quality.
Advertisement
However, one of the most significant contributions of SSL devices could be considered system and control compatibility due to their semiconductor nature. Generating light through semiconductor devices introduced us to a whole new range of capabilities, generally known as smart lighting.
Smart lighting is a growing industry with the potential of reaching a market value of US$21bn by 2023 1 . A smart lighting system aims to reduce the energy consumed by lighting by sensing environmental factors (for example, occupant presence) and controlling the light output (for example, dimming). The earlier smart lighting applications were rudimentary in terms of the light output control and integration of sensor data, but an increasing number of different technologies has been proposed to resolve connectivity and control issues. Despite the complexity of intelligent lighting systems, the basic functions are very simple: quality lighting, user satisfaction and energy efficiency.
Lighting quality and energy efficiency can be measured through the physical properties of a light source. The spectral power distribution (SPD, aka spectrum) is the radiant power emitted from a light source as a function of wavelength. It is possible to calculate the colour quality of light sources, such as chromaticity (for example, CCT, Duv, chromaticity coordinates) and colour rendering metrics (CRI, ANSI/IES TM-30), as well as energy efficiency (or the luminous efficacy of radiation) using light source SPD. The colour appearance of an object is a product of interactions between the light source spectrum, the object’s spectral reflectance and the human visual system. An object reflects some of the incident light on its surface, and the reflected light reaches observers to be perceived as the colour of the object.
However, the light that is not reflected is absorbed by the object and turns into heat; it is wasted for lighting purposes. Since the output of the SSL devices, such as LEDs, can be adjusted, it is possible to create a lighting system by optimising the light source SPD to reduce the amount of light absorbed by objects. For example, a red object reflects light in the longer wavelengths (perceived red), but the shorter wavelength radiation (perceived blue) is absorbed by the object. Therefore, eliminating the light absorption by not emitting the short wavelengths can tremendously increase energy efficiency.
A spectrally optimisable absorptionminimising lighting system consists of sensors that detect the 'colours' (reflectance characteristics) of objects and emit optimised light to each coloured part of an object, as shown in Figure 1. Although a 3D real-time implementation of this concept would be the ultimate goal, an early adaptation of such a lighting system could be used for illuminating 2D structures, such as paintings and facades.
ARCHITECTURAL APPLICATIONS: SAVING ENERGY Earlier investigations of the absorptionminimisation concept were based on computational simulations that identify the maximum theoretical energy savings. These simulations were not limited to the commercially available light source SPDs. Results indicate that the energy consumed by lighting to illuminate a series of coloured test samples could be reduced by up to 71 per cent if theoretical light source SPDs with two spectral peaks were generated2.
Energy savings gained by light optimisation did not compromise the colour quality of the objects. In this study, reflectance characteristics (object colours) were
Fig 1: a spectrally optimisable lighting system can detect the colours of objects through a sensor (top right corner) and emit optimised lighting to each coloured part of an object in the environment
grouped according to their spectral shapes. Expectedly, green objects resulted in relatively higher energy savings, due to the increased sensitivity of the human visual system to the middle wavelengths.
Extending on the computation work, we tested the spectral optimisation concept by using real light sources and everyday objects to quantify energy savings and colour shifts. Nine commercially available narrow-band LEDs were optimised to illuminate commonly found objects that are known for their colour appearance (for example, tomato representing red hue)3. A balanced set of objects were chosen that circulate the whole hue circle.
In the visual experiments, observers found the colour appearance of the fruits and vegetables equally natural and attractive under both optimised lighting conditions and reference phosphor-coated white LEDs. While energy savings were not as high as the previous studies, the visual experiments showed that the absorption-minimisation concept can deliver energy-efficient lighting without causing visual distortions. Higher energy savings could also be achieved if the saturation of the objects were increased since people prefer saturated objects (to a certain extent) and remember the colour of the objects to be more saturated than their original appearance4.
While the colour quality of the visual environment is critical for user satisfaction, other aspects of the human visual experience can also be incorporated into an intelligent lighting system. For example, a light projection system can be optimised to reduce visual clarity, scene preference and perceived brightness. Quantifying the visual aspects beyond colour requires evidencebased models that estimate human visual response to the visual environment. For example, image quality assessment measures have been adopted from imaging applications to the architectural domain to quantify the visual clarity of scenes5.
MUSEUM APPLICATIONS: REDUCING DAMAGE TO ARTWORK Absorption-minimisation lighting can offer advantages beyond energy efficiency. Light that is absorbed by objects turns into heat and can cause irreversible damage to light-sensitive objects, such as paintings. The change in the chemical composition of the molecules due to light absorption (photochemical action) can be decelerated, if not completely prevented, by optimising the light spectrum for object reflectance.
Earlier applications of the absorption minimisation concept on artwork suggest that it is possible to reduce damage caused by optical radiation and energy consumption by up to 55 per cent with perceptible but small colour shifts 6 . More advanced optimisation techniques can enable similar damage and energy consumption reductions without causing any perceptible colour shifts in the appearance of mono-coloured paintings7.
These studies were conducted using single-coloured paintings, and artworks are typically more complex in terms of colour, form, and structure, so the intelligent lighting system should address spatial qualities of the stimuli as well as spectral qualities. To address the spatial complexity issue, a light projection prototype has been developed, consisting of a CCD camera, an RGB projector and a computer 8 . Joaquin Sorolla’s painting, Women Walking on the Beach, was illuminated pixel by pixel with an RGB projector to restore its appearance and minimise future damage. Damage caused by optical radiation was reduced by up to 67 per cent in certain parts of the painting, and 37 per cent on average. There is more work to be done to further reduce the light absorption, and assess the visual appearance of artwork under optimised lighting by expert (conservators, curators) and non-expert observers.
CHALLENGES AND FUTURE IMPLICATIONS The intelligent lighting systems that are based on environmental sensing and light optimisation offer unprecedented opportunities. However, an advanced integrative lighting system will require computational power, precision optics and reflectance recovery – in real-time. Although the precision, spectral control and speed needed for this projection system seem enormous, there are encouraging advancements in the field of sensing and projection technologies.
For example, some of the functionalities of the proposed lighting system already exist in different forms. Indoor positioning through visible light positioning (VLP) allows the location of objects and people inside a building by using sensory information collected from mobile devices, while visible light communication (VLC) can be used to transmit data via modulating LED outputs9.
Fig 2: 3D projection mapping at the Vivid Sydney light festival in Australia
While the position, orientation and speed of objects in space are important factors, the light source spectrum and optical surface characteristics of objects are more fundamental to the proposed system. It is possible to 'extract' the spectral characteristics of light sources and object spectral reflectance by using common CCD cameras, or even colour encoded projectors 10,11 . These devices can offer Wi-Fi or Bluetooth connectivity to cloud storage and enable real-time operation and connection to other semiconductor devices. Real-time motion, object and surface recognition, object tracking and light-mapping systems are already realised in various forms, and they can be used by advanced integrative lighting systems.
The light output control complements the sensing technologies in an integrative lighting system. Tunable, high-precision light projection systems are crucial for the spectrally optimisable lighting systems.
Projection mapping, also known as video mapping, is a popular method, used to illuminate complex objects in the real world. Examples of projection mapping are commonly found in festivals in urban environments all over the globe. One of the most well-known examples is the Sydney Opera House at the Vivid Sydney lighting festival in Australia, as shown in Figure 2 (previous page). Designers of the projection mapping create a spatial map of the surface of a target area (a building facade, for example), and generate images or animations that may result in optical illusions, highlight dimensions or depth, or add a notion of movement to static surfaces.
In architectural spaces, the projection mapping applications would not require the same resolution, luminous flux, or contrast, as used by these outdoor examples where light is projected at high distances. The colour quality of the projection system is an essential factor when it is used in the built environment. It is possible to control a projection system independent of the existing lighting conditions, object colour and texture, projector position and brightness, by using several projectors 12 . A multi-projector approach can enable high consistency of image geometry, intensity and colour, which are crucial for the perceived quality of the environmental scenes. Increasing progress in the field of optics will hopefully provide even finer control over the light output of a projection system. FINAL THOUGHTS Optimising light source output by considering the environmental characteristics has the potential to create efficient and good quality lighting. The absorptionminimisation concept is a technology-neutral way of reducing energy consumption without causing perceptual distortions in the visual perception. Although today LEDs are widely used in the lighting industry, emerging technologies, such as quantum dots and lasers, can also be used in an integrative adaptive lighting system. Despite the current engineering limitations, there is enough experimental data supporting the successful application of the proposed concept in architectural spaces. As often stated, future studies should investigate the spatial and temporal characteristics of the system in real-time, and the preference and acceptability of the results by conducting visual experiments.
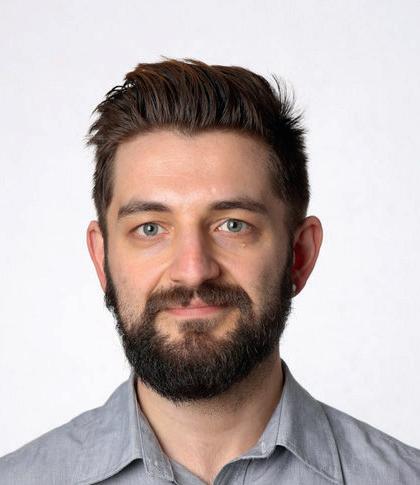
Alp Durmus is assistant professor at the Department of Architectural Engineering, Pennsylvania State University
References
1 Rohan, S (2018) Smart Lighting
Market worth 20.98 Billion USD by 2023. Retrieved from https:// www.marketsandmarkets.com/
PressReleases/smart-lighting.asp 2 Durmus, D and Davis, W (2015)
Absorption-Minimizing Spectral
Power Distributions. Light, Energy and the Environment (Optical
Society of America, OSA Technical
Digest (online), 2015), paper JTu5A.2. 3 Durmus, D and Davis, W (2017)
Object color naturalness and attractiveness with spectrally optimized illumination. Optics
Express, 25(11), 12839-12850. 4 Siple, P and Springer, RM (1983).
Memory and preference for the colors of objects. Perception and psychophysics, 34(4), 363-370. 5 Durmus, D (2020). Spatial frequency and the performance of imagebased visual complexity metrics.
IEEE Access, 8, 100111-111119. 6 Abdalla, D, Duis, A, Durmus, D and
Davis, W (2016). Customisation of light source spectrum to minimise light absorbed by artwork. In
Proc. CIE Lighting Quality Energy
Efficiency (pp. 22-31). 7 Durmus, D, Abdalla, D, Duis, A and Davis, W (2020). Spectral optimization to minimize light absorbed by artwork. Leukos, 16(1), 45-54. 8
9
10
11
12 D Vázquez, AA FernándezBalbuena, H Canabal, C Muro, D Durmus, W Davis, A Benítez and S Mayorga. (2020) Energy optimization of a light projection system for buildings that virtually restores artworks. Digital Applications in Archaeology and Cultural Heritage, 16, e00128. Chew, I, Karunatilaka, D, Tan, CP and Kalavally, V (2017). Smart lighting: The way forward? Reviewing the past to shape the future. Energy and Buildings, 149, 180-191. Botero-Valencia, JS, ValenciaAguirre, J, Durmus, D and Davis, W (2019). Multi-channel low-cost light spectrum measurement using a multilayer perceptron. Energy and Buildings, 199, 579-587. Chen, SY, Li, YF, Guan, Q and Xiao, G (2006). Real-time three-dimensional surface measurement by color encoded light projection. Applied Physics Letters, 89(11), 111108. Bimber, O and Emmerling, A (2006). Multifocal projection: A multiprojector technique for increasing focal depth. IEEE Transactions on Visualization and Computer Graphics, 12(4), 658-667.