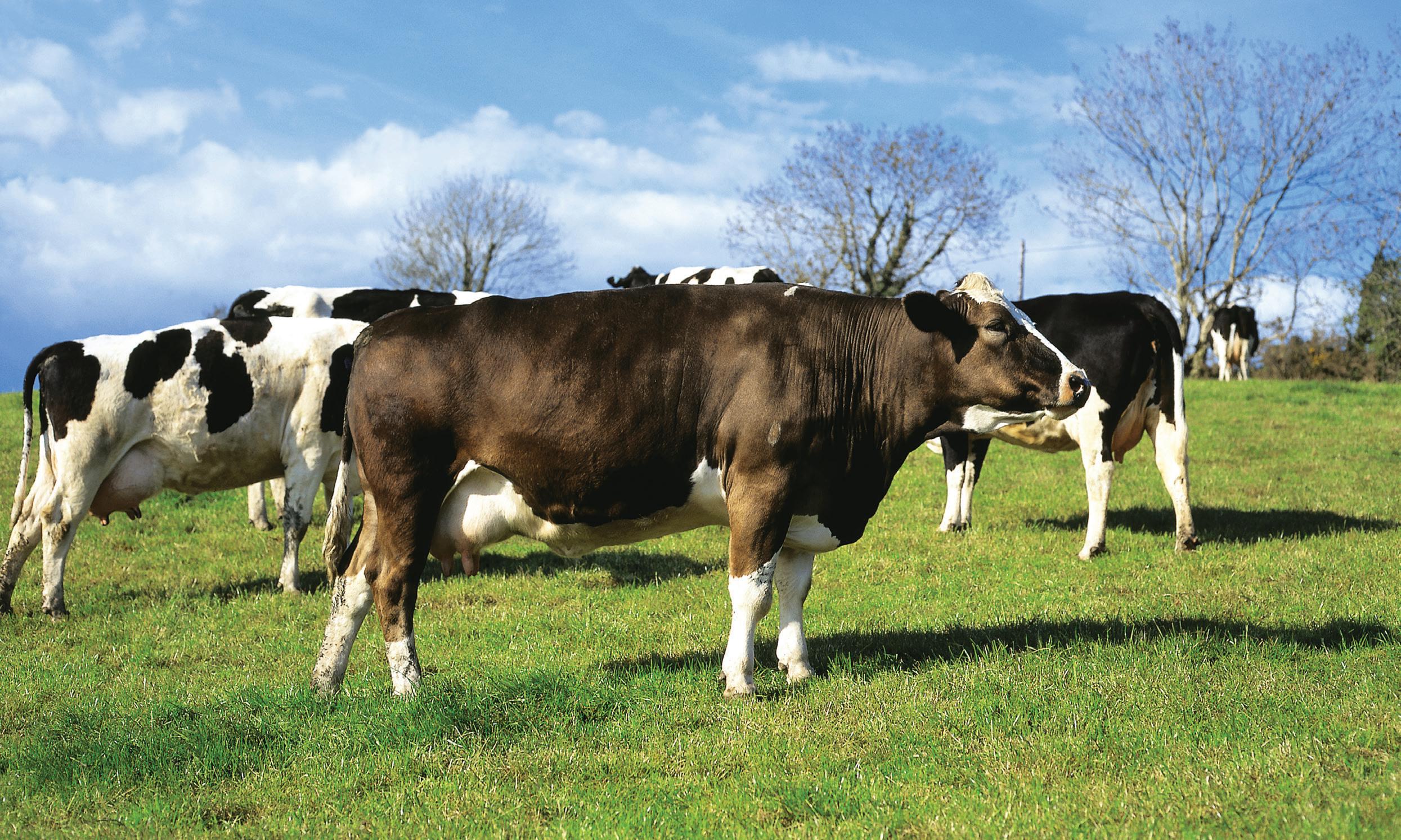
4 minute read
Feed Solutions
CHELATED MINERALS, COMPLEXES AND INORGANIC SALTS
Authors:
Advertisement
Giovanni Predieri Roberto Barea Silvia Peris
During the first decade of this century, a large number of studies have shown that the bioavailability of trace elements can be improved when they are supplemented as chelates (Jondreville and Revy, 2003; Ballarini and Predieri, 2007; Liu et al., 2014). The benefits provided by chelated minerals with respect to inorganic sources can be attributed to: (a) absence of free metal cations (Fe2+, Mn2+, Cu2+ , etc.) responsible for feed deterioration through free radicals formation; (b) protection of minerals from interaction with phytates and oxalates and from antagonism with other minerals; and (c) less mineral excretion into the environment.
The classification of the trace elements according to the Register of Feed Additives of the EU is not the ideal since the chemical nature of the different mineral additives is not taken into account. In fact, not all amino acid (AA) and mineral aggregations lead to chelated compounds (Ballarini and Predieri, 2007). This forces us to make a better distinction between mineral complexes and true chelates.
The nature of the chemical bond
The chemical bond is the mutual attraction between atoms that allows the formation of chemical compounds. Depending on the nature of the atomic interactions, three categories of chemical bonds exist in nature: ionic, covalent, and metallic (the latter will not be described in this article). An ionic bond forms when oppositely charged ions (anions and cations) attract each other. This type of bond occurs between metals (cations: Zn, Ca, Mn, etc.) and non-metal (anions: S, O, etc.). A covalent bond is characterized by the exchange of one or more pairs of electrons between atoms. A special type of this bond is the dative, or coordinated, covalent bond, which occurs when one atom gives both electrons (donor) to another atom that receives them (acceptor).
Chelates are special complexes
Coordinated covalent bonds can produce chelates (chele = clamps), if the arrangement of donor atoms around the mineral forms a chelation ring (Figure 1). From the chemical point of view, there are four main classes of “organic” minerals marketed in feed
animal industry: (a) mineral complexes with AA or peptides derived from protein lysates, called proteinates; (b) mineral complexes of a single AA such as glycine (Gly) with a welldefined formula (eg, Zn(HGly) SO4), called improperly glycinates or “mono-chelates”; (c) minerals chelated with a single AA, with well-defined formula (eg Zn(Gly)2), known as AA bis-chelates; and (d) minerals chelated with hydroxy analogue of methionine (HMTBa) of well defined formula (eg, Zn(HMTBa)2, which are bis-chelates of HMTBa (MINTREX®, Novus International, Inc.).
Class (a) contains simple complexes since oligopeptides cannot form a double chelation ring: mineral cations can only interact with terminal amino or carboxylate groups. Class (b) is based on molecules such as Zn(HGly)SO4, whose crystalline structure clearly shows a nonchelating behavior in which glycine is bound to Zn only through the carboxylate oxygen (Figure 2A). Class (c) refers to true AA chelates but whose stability decreases when the pH of the medium diminishes. Finally, Class (d) includes true HMTBa chelates (Figure 2B), which resist better than AA to the low pH value found, for example, in the upper parts of the gastrointestinal tract of the animal. This is due to the stronger acid characterisctic of HMTBa, which is less susceptible to be protonated in acid media (Predieri et al., 2003; 2005) (Figure 2B). Fouriertransform infrared spectroscopy (FTIR) allows the identification and quantification of the products from classes (b) to (d), being able to distinguish simple complexes (as in class b) from true chelates (classes c and d) (Predierietal., 2009).
Trace mineral bioavailability in livestock animals
The bioavailability of a mineral is generally defined as a proportion of the total mineral in feed that is utilized for normal body functions. It has been traditionally estimated as the mineral retention in the animal tissues (Underwood and Suttle, 1999). More recently, the use of biomarkers has allowed to know the bioavailability of a mineral in a more precise way. An example is that of the metallothionein protein, whose expression in the intestinal mucosa is regulated by the amount of mineral (mainly Zn and Cu) absorbed. Figure 3 shows the result of an experiment that compared the bioavailability of various Zn sources by measuring metallothionein mRNA expression in the intestine of broilers (Richards et al., 2007; 2008). The birds were fed a control diet or diets supplemented with 70 ppm of Zn from different sources. The results showed a higher metallothionein mRNA expression in animals supplemented with MINTREX®Zn, indicating that it was the source that provided the highest bioavailability.
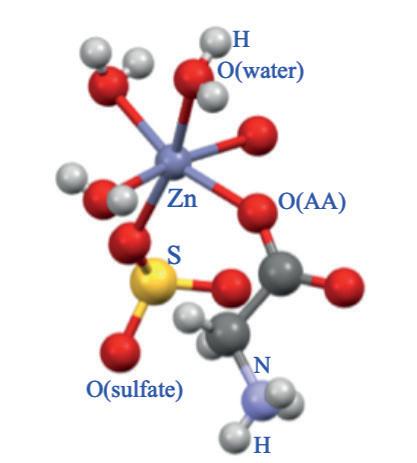
Conclusions
Feeding a chemically welldefined, stable and highly bioavailable trace mineral source is an important key to optimize the growth potential and animal welfare. True chelated minerals, such as MINTREX®, have the potential to deliver trace minerals more effectively to the tissues of the animal, improving its metabolic functions and, consequently, its productive performance.
For more information, contact info.europe@novusint.com
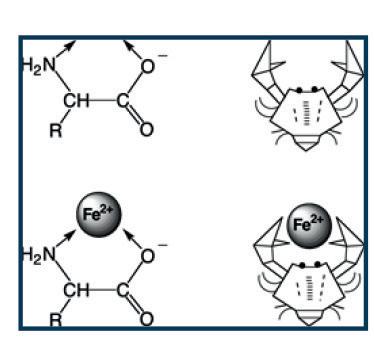
Figure 1. Sketches of an amino acid (AA) acting as a chelated ligand (crab claws); (bottom) an iron (II) cation chelated by a deprotonated AA (the other AA forming the bis-chelate is omitted for simplicity) (Ballarini and Predieri, 2007).
A
B
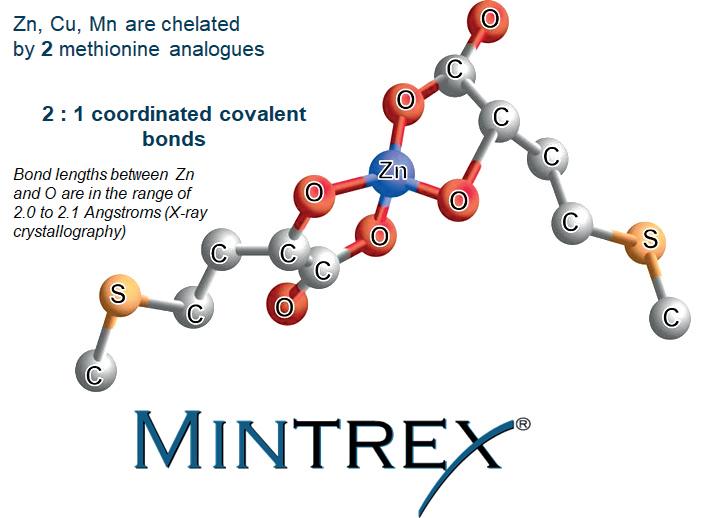
Figure 2. Molecular structures in the solid state of: (a) Zn glycine complex (Zn(HGly)(H2O)3SO4) (Cambridge Structural Database); (b) Zn(HMTBa)2.2H2O, showing the two chelating rings between the HMTBa anions around Zn (Novus International, Inc.).
Figure 3. Metallothionein mRNA expression in the intestinal mucosa of broilers as an indicator of Zn bioavailability (Richards et al., 2007; 2008).
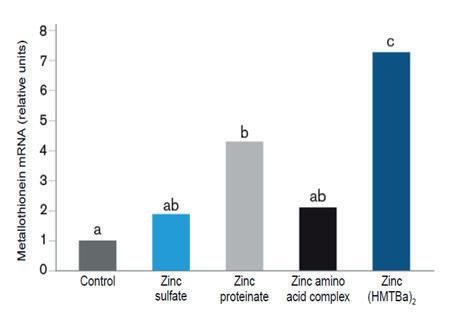