Pluripotent Stem Cell Therapy for Diabetes
First Edition
Editors Lorenzo Piemonti
Diabetes Research Institute and Clinical Unit of Regenerative Medicine and Transplant
IRCCS San Raffaele Hospital/Vita-Salute
San Raffaele University
Milan, Italy
Timothy J. Kieffer
University of British Columbia Vancouver, BC, Canada
Eelco de Koning
Department of Medicine
Leiden University Medical Center
Leiden, The Netherlands
Jon Odorico
Department of Surgery
University of Wisconsin School of Medicine and Public Health Madison, WI, USA
Valeria Sordi
IRCCS Ospedale San Raffaele Diabetes Research Institute Milan, Italy
ISBN 978-3-031-41942-3 ISBN 978-3-031-41943-0 (eBook) https://doi.org/10.1007/978-3-031-41943-0
© The Editor(s) (if applicable) and The Author(s), under exclusive license to Springer Nature Switzerland AG 2023
This work is subject to copyright. All rights are solely and exclusively licensed by the Publisher, whether the whole or part of the material is concerned, specifcally the rights of translation, reprinting, reuse of illustrations, recitation, broadcasting, reproduction on microflms or in any other physical way, and transmission or information storage and retrieval, electronic adaptation, computer software, or by similar or dissimilar methodology now known or hereafter developed.
The use of general descriptive names, registered names, trademarks, service marks, etc. in this publication does not imply, even in the absence of a specifc statement, that such names are exempt from the relevant protective laws and regulations and therefore free for general use.
The publisher, the authors, and the editors are safe to assume that the advice and information in this book are believed to be true and accurate at the date of publication. Neither the publisher nor the authors or the editors give a warranty, expressed or implied, with respect to the material contained herein or for any errors or omissions that may have been made. The publisher remains neutral with regard to jurisdictional claims in published maps and institutional affliations.
This Springer imprint is published by the registered company Springer Nature Switzerland AG The registered company address is: Gewerbestrasse 11, 6330 Cham, Switzerland
Paper in this product is recyclable.
To all those who face the daily challenges of type 1 diabetes, this book is dedicated to you. In the pursuit of a world where insulin is no longer a necessity, you have been the unwavering beacon of hope and inspiration.
To the patients who have endured the relentless battle, you have shown remarkable resilience and strength, and your unwavering spirit fuels the drive for a better future.
To the families who have stood by their loved ones, your unwavering support and endless encouragement have been the cornerstones of their journey towards a healthier tomorrow.
To the researchers and scientists who have dedicated their lives, your relentless pursuit of knowledge and breakthroughs has brought us one step closer to an insulin-free world.
To all those who have contributed their time, resources, and expertise, your unwavering belief in the possibility of a brighter future has fuelled the momentum of progress.
As Helen Keller once said, “Alone we can do so little; together we can do so much.” It is through our collective efforts, our collaboration, and our shared vision that we can create a world where type 1 diabetes is no longer a barrier. This book stands as a testament to the power of unity, perseverance, and the unwavering belief in a future where treatment options abound.
With heartfelt gratitude and endless admiration, this book is dedicated to the patients, families, and believers, who have fuelled the journey towards an insulin-free world.
Preface
In recent years, the feld of diabetes research has witnessed remarkable progress in understanding and exploring novel avenues for the treatment of this chronic disease. Among the various approaches under investigation, beta cell replacement holds great promise as a potential cure for type 1 diabetes. The ability to restore functional beta cells, the insulin-producing powerhouses of the pancreas, could revolutionise the lives of millions affected by this condition. The aim of this book is to offer a comprehensive resource that brings together the vast knowledge and advancements in the feld of stem cell therapy for diabetes. From the historical milestones of insulin therapy to the recent breakthroughs in pluripotent stem cell research, this book strives to be a unique compilation that explores the subject matter from various angles. By encompassing a wide range of topics, it serves as a comprehensive guide for researchers, clinicians, and scientists seeking to deepen their understanding of the potential applications of stem cells in regenerative medicine. As we refect on the centenary of the discovery of insulin, we celebrate the remarkable success story that has saved countless lives over the past century. However, we must also acknowledge the limitations of insulin therapy and renew our commitment to strive for an insulin-free world. The convergence of advancements in stem cell research, tissue engineering, and regenerative medicine presents a timely opportunity to address these limitations and revolutionise diabetes treatment.
Part I sets the foundation by exploring the developmental journey and differentiation of beta cells. It elucidates the potential of human pluripotent stem cells to mimic islet development and discusses the prospects of beta cell replacement as a defnitive cure for type 1 diabetes. Additionally, it investigates the derivation of functional human beta cells in vitro and explores key events in islet development that serve as a blueprint for successful in vitro differentiation. Furthermore, it examines methods of enhancing stem cell-derived islets, pushing the boundaries of possibility even further.
Part II delves into the genetic regulatory networks that guide the intricate process of islet development. It unravels the regulatory logic behind biological processes, shedding light on the complex interplay of genes and signalling pathways during pancreas organogenesis. By comprehending these genetic regulatory networks, we
can gain a deeper understanding of the intricacies of beta cell development and harness this knowledge for therapeutic applications.
Part III explores the realm of bioengineering approaches for beta cell replacement. It discusses the selection of biocompatible biomaterials for stem cell-derived beta cell transplantation, the design of scaffolds for encapsulating these cells, and the creation of bioengineered vascularised insulin-producing endocrine tissues. Additionally, it examines the innovative concept of 3-D organoids composed of allogeneic mesenchymal stromal and pancreatic islet cells, offering a glimpse into the future of regenerative medicine.
Part IV delves into preclinical models and translational approaches, examining the crucial considerations involved in implant sites for cell-based insulin replacement therapies. It addresses the implementation of genetic safety switches for pluripotent stem cell-derived therapies, mitigating risks and ensuring patient safety. Furthermore, it delves into the concerns surrounding teratoma risk, emphasising the importance of rigorous safety protocols in the development of novel therapeutic approaches.
Finally, Part V provides an insightful glimpse into the clinical horizon of beta cell replacement. It explores the social value of cell-based technologies in type 1 diabetes and sheds light on emerging regulatory perspectives surrounding beta cell replacement products. Drawing on lessons learned from clinical trials of islet transplantation, this part highlights the challenges, successes, and future directions of translating beta cell replacement therapies from the laboratory to the clinic. Moreover, it identifes the minimal stem cell-derived beta cell properties required for successful transplantation and examines ongoing clinical trials involving stem cell-derived insulin-producing cells.
By traversing the diverse terrain of development, bioengineering, preclinical models, and clinical perspectives, this book aims to provide readers with a comprehensive overview of the multifaceted world of beta cell replacement. It is our hope that this collective knowledge will inspire researchers, clinicians, and policymakers alike, fostering collaboration and driving innovation towards a future where type 1 diabetes is no longer a lifelong burden but a conquered challenge.
Milan, Italy
Madison, WI, USA
Lorenzo Piemonti
Jon Odorico Vancouver, BC, Canada
Timothy J. Kieffer Milan, Italy
Valeria Sordi Leiden, The Netherlands Eelco de Koning
Part I Development and Differentiation of Beta Cells
Mimicking Islet Development with Human Pluripotent Stem Cells .
Aubrey L. Faust, Adrian Veres, and Douglas A. Melton
Genetic Regulatory Networks Guiding Islet Development
Xin-Xin Yu, Xin Wang, Wei-Lin Qiu, Liu Yang, and Cheng-Ran Xu
Pancreatic Cell Fate Specification: Insights Into Developmental Mechanisms and Their Application for Lineage Reprogramming
Sara Gonzalez Ortega, Anna Melati, Victoria Menne, Anna Salowka, Miriam Vazquez Segoviano, and Francesca M. Spagnoli
Factors Influencing In Vivo Specification and Function of Endocrine Cells Derived from Pancreatic Progenitors
Nelly Saber and Timothy J. Kieffer
The Promises of Pancreatic Progenitor Proliferation and Differentiation
Azuma Kimura and Kenji Osafune
Part II Bioengineering
Selecting Biocompatible Biomaterials for Stem Cell-Derived β-Cell Transplantation
Rick de Vries and Aart A. van Apeldoorn
Scaffolds for Encapsulation of Stem Cell-Derived β Cells
Rick de Vries and Aart A. van Apeldoorn
Bioengineered Vascularized Insulin Producing Endocrine Tissues
Francesco Campo, Alessia Neroni, Cataldo Pignatelli, Juliette Bignard, Ekaterine Berishvili, Lorenzo Piemonti, and Antonio Citro
3 D Organoids of Mesenchymal Stromal and Pancreatic Islet Cells
Christof Westenfelder and Anna Gooch
Extracellular Matrix to Support Beta Cell Health and Function
Daniel M. Tremmel, Sara Dutton Sackett, and Jon S. Odorico
Bioactive Materials for Use in Stem Cell Therapies for the Treatment of Type 1 Diabetes
Jonathan Hinchliffe and Ipsita Roy
Islet Macroencapsulation: Strategies to Boost Islet Graft
Oxygenation
Barbara Ludwig, Carolin Heller, Victoria Sarangova, and Petra B. Welzel
Part III Immunoescape
Immunogenicity of Stem Cell Derived Beta Cells
Nicoline H. M. den Hollander and Bart O. Roep
Immune Evasive Stem Cell Islets
Federica Cuozzo, Valeria Sordi, and Lorenzo Piemonti
Islet Immunoengineering
Leonor N. Teles, Chris M. Li, Zachary M. Wilkes, Aaron A. Stock, and Alice A. Tomei
Part IV Preclinical Model and Translational Approaches
Considerations Pertaining to Implant Sites for Cell-Based Insulin
Replacement Therapies
Braulio A. Marfl-Garza, Nerea Cuesta-Gomez, and A. M. James Shapiro
Genetic Safety Switches for Pluripotent Stem Cell-Derived Therapies for Diabetes
Dena E. Cohen and Jon S. Odorico
Safety Issues Related to Pluripotent Stem Cell-Based Therapies: Tumour Risk
Sanne Hillenius, Joaquin Montilla-Rojo, Thomas F. Eleveld, Daniela
C. F. Salvatori, and Leendert H. J. Looijenga
Part V Beta Cell Replacement: Clinical Horizon
An Ethical Perspective on the Social Value of Cell-Based Technologies in Type 1 Diabetes
Dide de Jongh and Eline M. Bunnik
Beta Cell Replacement Cellular Products: Emerging Regulatory Perspectives and Considerations for Program Development
Bruce S. Schneider
Lessons Learned from Clinical Trials of Islet Transplantation .
Thierry Berney, Lionel Badet, Ekaterine Berishvili, Fanny Buron, Philippe Compagnon, Fadi Haidar, Emmanuel Morelon, Andrea Peloso, and Olivier Thaunat
Minimal SC-β-Cell Properties for Transplantation in Diabetic Patients
Veronica Cochrane, Yini Xiao, Hasna Maachi, and Matthias Hebrok
Clinical Trials with Stem Cell-Derived Insulin-Producing Cells
Ji Lei and James F. Markmann
Modelling of Beta Cell Pathophysiology Using Stem Cell-Derived Islets
Tom Barsby, Hossam Montaser, Väinö Lithovius, Hazem Ibrahim, Eliisa
Vähäkangas, Sachin Muralidharan, Vikash Chandra, Jonna SaarimäkiVire, and Timo Otonkoski
Contributors
Lionel Badet Department of Urology and Transplantation Surgery, Hospices Civils de Lyon, Lyon, France
Tom Barsby Stem Cells and Metabolism Research Program, Faculty of Medicine, University of Helsinki, Helsinki, Finland
Ekaterine Berishvili Laboratory of Tissue Engineering and Organ Regeneration, Department of Surgery, University of Geneva, Geneva, Switzerland
Cell Isolation and Transplantation Center, Department of Surgery, Geneva University Hospitals and University of Geneva, Geneva, Switzerland
Faculty Diabetes Center, University of Geneva Medical Center, Geneva, Switzerland
Institute of Medical and Public Health Research, Ilia State University, Tbilisi, Georgia
Division of Transplantation, Department of Surgery, University of Geneva Hospitals, Geneva, Switzerland
School of Natural Sciences and Medicine, Ilia State University, Tbilisi, Georgia
Thierry Berney Division of Transplantation, Department of Surgery, University of Geneva Hospitals, Geneva, Switzerland
Department of Transplantation, Nephrology and Clinical Immunology, Hospices Civils de Lyon, Lyon, France
School of Natural Sciences and Medicine, Ilia State University, Tbilisi, Georgia
Juliette Bignard Laboratory of Tissue Engineering and Organ Regeneration, Department of Surgery, University of Geneva, Geneva, Switzerland
Cell Isolation and Transplantation Center, Department of Surgery, Geneva University Hospitals and University of Geneva, Geneva, Switzerland
Faculty Diabetes Center, University of Geneva Medical Center, Geneva, Switzerland
Eline M. Bunnik Erasmus MC, Department of Medical Ethics, Philosophy and History of Medicine, Rotterdam, The Netherlands
Fanny Buron Department of Transplantation, Nephrology and Clinical Immunology, Hospices Civils de Lyon, Lyon, France
Francesco Campo Diabetes Research Institute and Clinical Unit of Regenerative Medicine and Transplant, IRCCS San Raffaele Hospital, Milan, Italy
Vikash Chandra Stem Cells and Metabolism Research Program, Faculty of Medicine, University of Helsinki, Helsinki, Finland
Antonio Citro Diabetes Research Institute and Clinical Unit of Regenerative Medicine and Transplant, IRCCS San Raffaele Hospital, Milan, Italy
Veronica Cochrane Diabetes Center, Department of Medicine, University of California, San Francisco, CA, USA
Dena E. Cohen Regenerative Medical Solutions, Inc, Madison, WI, USA
Philippe Compagnon Division of Transplantation, Department of Surgery, University of Geneva Hospitals, Geneva, Switzerland
Nerea Cuesta-Gomez Alberta Diabetes Institute, Edmonton, AB, Canada
Department of Surgery, University of Alberta, Edmonton, AB, Canada
Federica Cuozzo Diabetes Research Institute, IRCCS San Raffaele Hospital, Milan, Italy
Dide de Jongh Erasmus MC, Department of Medical Ethics, Philosophy and History of Medicine, Department of Nephrology and Transplantation, Rotterdam, The Netherlands
Rick de Vries Maastricht University, MERLN Institute for Technology-Inspired Regenerative Medicine, Maastricht, The Netherlands
Nicoline H. M. den Hollander Department of Internal Medicine, Section Immunomodulation & Regenerative Medicine, Leiden University Medical Center, Leiden, The Netherlands
Thomas F. Eleveld Princess Máxima Center for Pediatric Oncology, Utrecht, The Netherlands
Aubrey L. Faust Department of Stem Cell and Regenerative Biology and Harvard Stem Cell Institute, Harvard University, Boston, MA, USA
Sara Gonzalez Ortega Centre for Gene Therapy and Regenerative Medicine, King’s College London, Guy’s Hospital, London, UK
Anna Gooch Department of Medicine, University of Utah, Salt Lake City, UT, USA
SymbioCellTech, Salt Lake City, UT, USA
Fadi Haidar Division of Transplantation, Department of Surgery, University of Geneva Hospitals, Geneva, Switzerland
Contributors
Matthias Hebrok Diabetes Center, Department of Medicine, University of California, San Francisco, CA, USA
Center for Organoid Systems (COS), Technical University Munich (TUM), Garching, Germany
Institute for Diabetes Organoid Technology (IDOT), Helmholtz Center Munich, Helmholtz Diabetes Center (HDC), Neuherberg, Germany
Carolin Heller Department of Medicine III, Paul Langerhans Institute Dresden (PLID) of the Helmholtz Center Munich at the University Hospital Carl Gustav Carus, CRTD/DFG-Center for Regenerative Therapies, Technische Universität Dresden, Dresden, Germany
Leibniz-Institut für Polymerforschung Dresden e.V., Max Bergmann Center of Biomaterials Dresden, Dresden, Germany
Sanne Hillenius Princess Máxima Center for Pediatric Oncology, Utrecht, The Netherlands
Jonathan Hinchliffe Department of Materials Science and Engineering, University of Sheffeld, Sheffeld, UK
Hazem Ibrahim Stem Cells and Metabolism Research Program, Faculty of Medicine, University of Helsinki, Helsinki, Finland
Timothy J. Kieffer Laboratory of Molecular and Cellular Medicine, Department of Cellular & Physiological Sciences, Life Sciences Institute, University of British Columbia, Vancouver, BC, Canada
School of Biomedical Engineering, University of British Columbia, Vancouver, BC, Canada
Department of Surgery, University of British Columbia, Vancouver, BC, Canada
Azuma Kimura Center for iPS Cell Research and Application (CiRA), Kyoto University, Kyoto, Japan
Ji Lei Division of Transplantation and Center for Transplantation Sciences, Department of Surgery, Massachusetts General Hospital, Harvard Medical School, Boston, MA, USA
Chris M. Li Diabetes Research Institute and Department of Microbiology and Immunology, University of Miami School of Medicine, Miami, FL, USA
Väinö Lithovius Stem Cells and Metabolism Research Program, Faculty of Medicine, University of Helsinki, Helsinki, Finland
Leendert H. J. Looijenga Princess Máxima Center for Pediatric Oncology, Utrecht, The Netherlands
Barbara Ludwig Department of Medicine III, Paul Langerhans Institute Dresden (PLID) of the Helmholtz Center Munich at the University Hospital Carl Gustav Carus, CRTD/DFG-Center for Regenerative Therapies, Technische Universität Dresden, Dresden, Germany
Hasna Maachi Diabetes Center, Department of Medicine, University of California, San Francisco, CA, USA
Braulio A. Marfl-Garza Alberta Diabetes Institute, Edmonton, AB, Canada Clinical Islet Transplant Program, University of Alberta, Edmonton, AB, Canada
CHRISTUS-LatAm Hub – Excellence and Innovation Center, Monterrey, Mexico
James F. Markmann Division of Transplantation and Center for Transplantation Sciences, Department of Surgery, Massachusetts General Hospital, Harvard Medical School, Boston, MA, USA
Anna Melati Centre for Gene Therapy and Regenerative Medicine, King’s College London, Guy’s Hospital, London, UK
Douglas A. Melton Department of Stem Cell and Regenerative Biology and Harvard Stem Cell Institute, Harvard University, Boston, MA, USA
Victoria Menne Centre for Gene Therapy and Regenerative Medicine, King’s College London, Guy’s Hospital, London, UK
Hossam Montaser Stem Cells and Metabolism Research Program, Faculty of Medicine, University of Helsinki, Helsinki, Finland
Joaquin Montilla-Rojo Anatomy and Physiology, Department Clinical Sciences, Faculty of Veterinary Medicine, Utrecht University, Utrecht, The Netherlands
Emmanuel Morelon Department of Transplantation, Nephrology and Clinical Immunology, Hospices Civils de Lyon, Lyon, France
Sachin Muralidharan Stem Cells and Metabolism Research Program, Faculty of Medicine, University of Helsinki, Helsinki, Finland
Alessia Neroni Diabetes Research Institute and Clinical Unit of Regenerative Medicine and Transplant, IRCCS San Raffaele Hospital, Milan, Italy
Jon S. Odorico Transplantation Division, University of Wisconsin-Madison Department of Surgery, Madison, WI, USA
Kenji Osafune Center for iPS Cell Research and Application (CiRA), Kyoto University, Kyoto, Japan
Timo Otonkoski Stem Cells and Metabolism Research Program, Faculty of Medicine, University of Helsinki, Helsinki, Finland
Andrea Peloso Division of Transplantation, Department of Surgery, University of Geneva Hospitals, Geneva, Switzerland
Lorenzo Piemonti Diabetes Research Institute and Clinical Unit of Regenerative Medicine and Transplant, IRCCS San Raffaele Hospital, Milan, Italy
Cataldo Pignatelli Diabetes Research Institute and Clinical Unit of Regenerative Medicine and Transplant, IRCCS San Raffaele Hospital, Milan, Italy
Wei-Lin Qiu School of Basic Medical Sciences, Department of Human Anatomy, Histology, and Embryology, Peking University Health Science Center, PekingTsinghua Center for Life Sciences, Peking University, Beijing, China
Bart O. Roep Department of Internal Medicine, Section Immunomodulation & Regenerative Medicine, Leiden University Medical Center, Leiden, The Netherlands
Ipsita Roy Department of Materials Science and Engineering, University of Sheffeld, Sheffeld, UK
Jonna Saarimäki-Vire Stem Cells and Metabolism Research Program, Faculty of Medicine, University of Helsinki, Helsinki, Finland
Nelly Saber Laboratory of Molecular and Cellular Medicine, Department of Cellular & Physiological Sciences, Life Sciences Institute, University of British Columbia, Vancouver, BC, Canada
Sara Dutton Sackett Transplantation Division, University of Wisconsin-Madison Department of Surgery, Madison, WI, USA
Anna Salowka Centre for Gene Therapy and Regenerative Medicine, King’s College London, Guy’s Hospital, London, UK
Daniela C. F. Salvatori Anatomy and Physiology, Department Clinical Sciences, Faculty of Veterinary Medicine, Utrecht University, Utrecht, The Netherlands
Victoria Sarangova Department of Medicine III, Hospital Carl Gustav Carus, Technische Universität Dresden, Dresden, Germany
Leibniz-Institut für Polymerforschung Dresden e.V., Max Bergmann Center of Biomaterials Dresden, Dresden, Germany
Bruce S. Schneider Schneider BIO Consultancy LLC, New York, NY, USA
A. M. James Shapiro Clinical Islet Transplant Program, University of Alberta, Edmonton, AB, Canada
Department of Surgery, University of Alberta, Edmonton, AB, Canada
Alberta Diabetes Institute, Edmonton, AB, Canada
Valeria Sordi Diabetes Research Institute, IRCCS San Raffaele Hospital, Milan, Italy
Francesca M. Spagnoli Centre for Gene Therapy and Regenerative Medicine, King’s College London, Guy’s Hospital, London, UK
Aaron A. Stock Diabetes Research Institute, University of Miami Miller School of Medicine, Miami, FL, USA
Leonor N. Teles Diabetes Research Institute and Department of Biomedical Engineering, University of Miami, Miami, FL, USA Contributors
Olivier Thaunat Department of Transplantation, Nephrology and Clinical Immunology, Hospices Civils de Lyon, Lyon, France
Alice A. Tomei Diabetes Research Institute, University of Miami Miller School of Medicine, Miami, FL, USA
Department of Biomedical Engineering, University of Miami Miller School of Medicine, Miami, FL, USA
Department of Surgery, University of Miami Miller School of Medicine, Miami, FL, USA
Daniel M. Tremmel Boston Children’s Hospital/Harvard Medical School Department of Cardiac Surgery, Boston, MA, USA
Eliisa Vähäkangas Stem Cells and Metabolism Research Program, Faculty of Medicine, University of Helsinki, Helsinki, Finland
Aart A. van Apeldoorn Maastricht University, MERLN institute for technologyinspired regenerative medicine, Maastricht, The Netherlands
Miriam Vazquez Segoviano Centre for Gene Therapy and Regenerative Medicine, King’s College London, Guy’s Hospital, London, UK
Adrian Veres Department of Stem Cell and Regenerative Biology and Harvard Stem Cell Institute, Harvard University, Boston, MA, USA
Xin Wang College of Life Sciences, Peking University, Beijing, China
Petra B. Welzel Leibniz-Institut für Polymerforschung Dresden e.V., Max Bergmann Center of Biomaterials Dresden, Dresden, Germany
Christof Westenfelder Department of Medicine, University of Utah, Salt Lake City, UT, USA
SymbioCellTech, Salt Lake City, UT, USA
Zachary M. Wilkes Diabetes Research Institute and Department of Biomedical Engineering, University of Miami, Miami, FL, USA
Yini Xiao Diabetes Center, Department of Medicine, University of California, San Francisco, CA, USA
Cheng-Ran Xu School of Basic Medical Sciences, Department of Human Anatomy, Histology, and Embryology, Peking University Health Science Center, Peking-Tsinghua Center for Life Sciences, Peking University, Beijing, China
Liu Yang School of Basic Medical Sciences, Department of Human Anatomy, Histology, and Embryology, Peking University Health Science Center, PekingTsinghua Center for Life Sciences, Peking University, Beijing, China
Xin-Xin Yu School of Basic Medical Sciences, Department of Human Anatomy, Histology, and Embryology, Peking University Health Science Center, PekingTsinghua Center for Life Sciences, Peking University, Beijing, China Contributors
3 Deriving
Functional Human Beta Cells In Vitro
Studies of pancreatic development in model organisms described the stages and genes involved in specifying pancreatic endoderm and endocrine differentiation [84]. Those studies formed the basis for subsequent work on the in vitro differentiation of pluripotent stem cells. In 2006, D’Amour and colleagues developed a protocol to progressively specify SOX17+ defnitive endoderm, then posterior foregut, then PDX1+ pancreatic endoderm [12]. Numerous publications followed similar protocols, but comparing their results is challenging given a lack of standardized cell type quantifcation. A notable advance by Nostro et al. employed factors that increased endocrine cell abundance, and this study provided more rigorous quantifcations of percentages of cells expressing particular markers, including C-peptide [46]. However, in all these reports, the resulting beta or beta-like cells were not able to secrete insulin in response to glucose challenges in vitro.
Early efforts to induce beta cells from pancreatic progenitors yielded a cell type of ambiguous identity, co-expressing insulin and glucagon. Because this hormone co-expression is not seen in mature islets, these cells were not defnitively classifed as a specifc islet cell type and were instead called “polyhormonal” cells. The hypothesis that these represented a beta cell progenitor was pursued but attempts to mature polyhormonal cells into a mono-hormonal insulin expression state, or to achieve the glucose-responsive insulin secretion expected of a beta cell, proved unsuccessful. Mimicking
A. L. Faust et al.
A pivotal advance came in 2014 with the publication of a detailed protocol that described both effcient beta cell differentiation and the frst compelling evidence for beta cell function in vitro [48]. Physiological function by sequential glucosestimulated insulin secretion (GSIS) is signifcant as that is what leads to glucose homeostasis in vivo. This advance, including all details on inducing factors, timing, and other experimental methods, was shared prior to publication with Rezania et al., who independently verifed the fndings [56]. The protocol to produce functional stem cell-derived beta cells and islets using human stem cells (essentially described in [48]) was patented and licensed to Semma Therapeutics, now Vertex Pharmaceuticals. The protocol has since been expanded, industrialized, and used in clinical trial.
4 Key Events in Islet Development as a Blueprint for In Vitro Differentiation
Efforts to differentiate beta cells in vitro have relied on an understanding of their normal development in vivo. Endocrine cells comprise approximately 2% of the pancreas by mass and are aggregated in structures called islets, which are interspersed throughout the exocrine tissue. Islets consist of fve endocrine cell types. The most abundant are insulin-secreting beta and glucagon-secreting alpha, whose hormone products have opposite metabolic effects and, in tandem, govern glucose homeostasis. The next most abundant are somatostatin-secreting delta cells, which regulate beta and alpha-cell activity through paracrine signaling. Finally, pancreaticpolypeptide-secreting gamma and ghrelin-secreting epsilon are the least abundant. No changes in glycemia or body weight are associated with gamma cell ablation in mice [51], and epsilon cells are absent from the adult pancreases of most species and constitute less than 1% of human islets [81]. Islet structure varies across species. In mice and most mammals, islets have a core-mantle structure with a shell of alpha and other non-beta cells and a beta cell interior [70]. In humans, islet structure is more complex, appearing superfcially disorganized but proposed to consist of sheets of alpha and beta cells folded into complex shapes to generate both homotypic and heterotypic contacts between these two cell types [16].
Based on similarities in gene expression patterns, it was hypothesized that pancreatic endocrine cells are derived from neural crest cells. This mistaken view was widely taught and included in many medical texts despite the lack of direct evidence [2]. It is the case that there are many similarities between neurons and islet endocrine cells: both cell types depolarize upon receiving input signals, using the resulting infux of Ca2+ ions to release the contents of secretory granules in specifc locations. There are also extensive gene expression commonalities, including many genes that are otherwise largely restricted to neurons. In Drosophila and other invertebrates, insulin-expressing cells are in fact neurons [59], spurring speculation that the ancestral cell type may have been an insulin-secreting neuron whose expression
programs have subsequently been activated in the gut tube. Regardless of their evolutionary history, the developmental origin of vertebrate islets is decidedly endodermal [53].
The pancreas is generated through branching morphogenesis, with epithelial branches organized into acinar tips and ductal trunks. Endocrine cells develop as scattered cells in the ductal epithelium. After endocrine induction, cells exit the ductal epithelium to form islets. One model to explain islet formation proposes that individual endocrine cells undergo a partial epithelial-to-mesenchymal transition and are delaminated from the ductal epithelium to enter developing islets through preferential adhesion. More recently, an alternative model was proposed whereby large duct segments initiate endocrine induction in concert, initially forming a “peninsula” that subsequently separates into islets as the cells gain mature endocrine identities [67].
At the cellular level, endocrine induction is initiated by the transient expression of Neurog3 [14, 22, 64]. Induction of suffcient levels of Neurog3 to drive endocrine induction requires low levels of Notch signaling [3], with the Notch target Hes1 suppressing transcription of Neurog3 [30, 38]. Downstream of Neurog3, a set of transcription factors (TFs) shared across islet cells maintains endocrine fate; these include Neurod1, Insm1, and Nkx2-2 [63]. After Neurog3 is downregulated, markers of individual cell types including hormones turn on and cell identity becomes apparent. When and how specifcation of the fve different islet cell types occurs, however, is unknown, and this knowledge gap has carried through to in vitro differentiation.
5 Applying Technologies for Cell Characterization and Perturbation
In vitro differentiation poses a practical test for defnitions of cell identity: when can we consider a cell type produced in vitro equivalent to its in vivo counterpart? Which genes are defnitional to a cell type’s identity, and how much can gene expression vary as a consequence of cell state such as age or environment without altering the underlying cell type identity? Perfect concordance in expression of every gene between directed differentiation derivatives and their in vivo counterparts is too high a bar. Differing metabolic or cellular environments must induce differences in gene expression, differences we can safely attribute to state not identity. Similarly, human fetal cells are classifed as the same cell types as their adult counterparts, despite an immature state that lacks expression of genes referred to as maturation markers. Consequently, cell function may be a better parameter for comparison of cells formed by in vitro differentiation to their natural counterparts. In practice, the feld progressed despite this puzzle by combining small panels of marker genes with a strong emphasis on functional assays. For beta cells, the bar was set at expression of insulin and the transcription factor NKX6.1, paired with the
A. L. Faust et al.
functional ability to secrete insulin in response to sequential glucose challenges in vitro and separately rescue murine diabetes models after transplantation in vivo. As noted above, these criteria were met in 2014, which produced the frst stem cellderived (SC-) beta cells that performed glucose-stimulated insulin secretion in vitro [48].
Various methods have been deployed to measure the similarity of in vitro and in vivo beta cell gene expression. These methods include biased, hypothesis-based approaches with single-cell resolution (such as immunofuorescence read out by microscopy or fow cytometry) or unbiased, whole-transcriptome approaches (micro-arrays and bulk RNA-seq) limited by an unknown degree of cellular heterogeneity in the input. While these methods gave insight into questions such as whether the resulting beta cells more closely resemble fetal or adult beta cells [27], they left unresolved whether unexpected cell populations might be present, and the extent to which SC-beta transcriptomes might diverge from those in islets.
These limitations changed dramatically with the advent of single-cell RNA sequencing, which enables comprehensive transcriptomic profling at single-cell resolution. Multiplexed single-cell RNA-seq emerged in 2011, initially with throughputs of fewer than 100 cells [29]. Adapting the molecular biology steps from these techniques to be performed within microscopic droplets created by microfuidics devices enabled the routine application of these techniques to characterize thousands of cells [35, 41]. Applying inDrops, we generated one of the frst wholetranscriptome profles of pancreatic islet cell identity [9]. This provided a reference dataset against which stem cell-derived islet cells can be benchmarked.
During this same period, the application of Cas9 to precisely edit genomes transformed our ability to systematically probe gene function. Forward genetics is an approach to mapping genes that control a phenotype and has been applied at scale in a number of biological contexts for decades. For mammalian genetics, mapping genes that control a phenotype had been accomplished by knocking out one gene at a time or using knockdown libraries of short hairpin RNAs, but variation in ontarget effciency and substantial off-target effects in the latter limited their use as a tool across biology. Genome-wide Cas9 knockout libraries share the concept of shRNA knockdown libraries but beneft from higher on-target and lower off-target activity to provide a new way to systematically study human biology. These approaches depend on next-generation DNA sequencing, which allows simultaneous sequencing of millions of short sequences to reveal which elements of a perturbation library (e.g., specifc sgRNAs) drive a phenotype of interest.
6 Stem Cell Differentiation Recapitulates Islet Development
The most commonly used approach for generating a cell type through directed differentiation is to guide cells through successive progenitor states by manipulating signaling pathway activities. While this method succeeded in generating
functional beta cells from human pluripotent stem cells, it does not produce beta cells in isolation. Applying droplet-based single-cell RNA-sequencing [76], we determined the identities of the several other cell types that co-develop with SC-beta cells, including SC-alpha and SC-enterochromaffn (EC) populations whose abundance rivals that of SC-beta cells. While the SC-alpha cells may enhance SC-islets due to their complementary role in glucose homeostasis, SC-EC cells are not typically found in islets and are unlikely to contribute to the desired function of SC-islets.
Our reclassifcation of polyhormonal cells as SC-alpha cells transiently expressing insulin resolved an earlier debate. Previously, population identities in SC-beta differentiations had been approximated by how marker gene immunostaining mapped to the characteristic expression in islet endocrine cell types. Because insulin and glucagon co-expression is not seen in adult islets, the identity and potential of so-called polyhormonal cells was an open question. Demonstrating close concordance between SC-alpha cells and their islet alpha counterparts connects to a broader question of whether in vitro differentiation can derive aberrant cell types that do not map to cells that naturally develop in vivo. In this case, a cell type initially viewed as an in vitro artifact due to insulin and glucagon co-expression was found instead to be a close analog of a canonical cell type and demonstrated to reach this canonical state over time even without additional exogenous factors.
Since our initial single-cell publication, rare populations observed in our and others’ data have been classifed as analogs of somatostatin-expressing delta and ghrelin-expressing epsilon cells. This demonstrates the derivation of nearly all islet cell types in what is effectively a self-assembly process following directed differentiation to pancreatic endoderm. Pancreatic polypeptide-expressing gamma cells are the sole islet endocrine population that has not been conclusively observed in stem cell differentiation. While this fact remains puzzling, gamma cell function remains a mystery, and their absence in a stem cell-derived islet is unlikely to be detrimental [51].
Non-endocrine cells mature over time in culture into approximations of exocrine acinar and ductal populations. Rather than lingering in a stalled progenitor state, they lose expression of transient developmental markers and gain expression of genes related to their functional roles in secreting and transporting digestive enzymes. Non-endocrine cells are considered undesirable in a graft setting. One reason relates to limited space, especially for SC-islets transplanted in a device. While endocrine cells rarely replicate, exocrine cells retain proliferative potential. Thus, nonendocrine cells occupy space that could be devoted to SC-beta cells instead and are expected to expand further through replication. Second, mature acinar cells secrete proteases that could physically damage the graft. To reduce exocrine cell abundance, we introduced a reaggregation process that depletes these populations (Fig. 1).
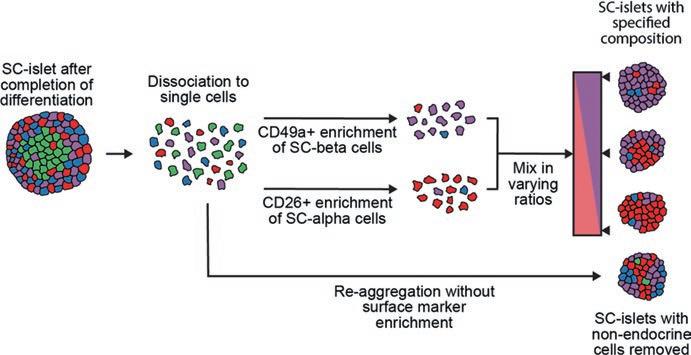
Fig. 1 Controlling SC-islet composition through a combination of surface marker sorting and reaggregation
7 A Piece Out of Place: The Puzzle of Enterochromaffn Cells
Among this near-complete representation of pancreatic exocrine and endocrine cell types, there is the puzzling emergence of enterochromaffn cells. Enterochromaffn cells are the most abundant enteroendocrine cell type and emerge in both the stomach and intestine but have not been identifed in the pancreas historically or in recent single-cell sequencing profles of human and mouse islets. Since our initial description of SC-EC cells in stem cell-derived islets, they have been observed across several protocol variants, cell lines, and research groups [4, 6, 26, 50, 79, 83] and no single-cell RNA-seq study of stem cell-derived beta cell cultures has reported their absence. The mechanisms driving SC-EC differentiation remain elusive. One study identifed a compound that gradually reduces SC-EC abundance during extended culture, but this timing suggests selective toxicity rather than developmental control [6].
Two underlying explanations for the presence of enterochromaffn cells in isletdirected differentiation have emerged: (1) incomplete pancreatic specifcation yields an intestinal progenitor that generates an enteroendocrine cell type, or (2) enterochromaffn identity is a previously undescribed cell state in the developing human pancreas. The frst theory is that differentiations contain gastric or intestinal progenitors whose presence is revealed by their non-pancreatic progeny. Because SC-EC cells express the marker CDX2, an intestinal progenitor is more likely than a gastric one. Additionally, the standard method of evaluating whether directed differentiations generate pancreatic endoderm–immunostaining for PDX1–does not exclude the possibility of intestinal identity. Although the loss of PDX1 causes pancreatic agenesis [72], this gene is expressed in adjacent tissues: the duodenum
A.
contains Pdx1+/Cdx2+ cells, and the gastric antrum contains Pdx1+/Sox2+ cells [23, 43]. Further confounding progenitor classifcation, a recent study found that, in a divergence from mouse embryos, the human fetal pancreas contains CDX2+ cells [83]. VIL1, whose promoter is used in mouse models to drive gene expression in the intestinal epithelium [60], is also expressed in the human fetal pancreas [47]. Consequently, marker genes have been insuffcient to classify our in vitro progenitors, leaving unresolved the question of whether SC-EC cells derive from intestinal or gastric progenitors. Given the ambiguous picture painted by progenitor markers, an alternative is to consider whether the cell types made in our differentiations are consistent with such a progenitor pool. Other than SC-EC cells researchers have not identifed populations that are characteristically gastric or intestinal, but this claim, too, is confounded by marker ambiguity.
A second explanation for the emergence of SC-EC cells is that an equivalent state is present in the human pancreas either during development or under certain physiological conditions. Especially if this represents a divergence between human and mouse, these cells may not have been profled. Recent evidence supporting this view stems from the discovery of CDX2 expression during human fetal pancreatic development, and enterochromaffn cells may emerge from this progenitor state [83]. From their observation of a possible enterochromaffn population in fetal pancreas and its absence in adult islets, Zhu et al. conclude that this is a transient expression state that resolves toward a beta cell identity, and that such a change would likely occur over time in SC-islets as well. This hypothesis is challenged by data from single-cell sequencing of SC-islets 6 months after transplantation into diabetic mouse models, which found that EC cells persist and even increase their expression of EC identity markers while reducing expression of islet markers [5].
An intermediate explanation is the hypothesis that enterochromaffn cells are a default endocrine cell type in the intestine, stomach, and pancreas, but that conditions during normal pancreatic development effciently suppress their emergence. Enterochromaffn cells have been observed throughout the stomach and intestine with only subtle gene expression variation across sites [11]. An unusual feature of enterochromaffn cells observed through single-cell sequencing is the overlap of their marker genes with those of the shared expression program of endocrine progenitors. In the stomach, intestine, and pancreas, endocrine induction is driven by transient expression of NEUROG3, and markers that distinguish individual endocrine cell types are upregulated after NEUROG3 turns off. The notable exceptions to this are EC markers, which are co-expressed with NEUROG3 both in the intestine in vivo [20] and in our SC-islet differentiation in vitro.
EC cell emergence may be controlled by a small set of transcription factors that are shared across tissues. In the intestine, EC cells are the only endocrine cell type that does not express Isl1 [20], and intestine-specifc deletion of Isl1 increases enterochromaffn abundance while reducing differentiation of all other enteroendocrine cell types [75]. In the adult pancreas, all islet endocrine cells express ISL1. It is possible that effcient induction of ISL1 and similar genes in the pancreas repress EC cell emergence, and that these genes are not induced as highly, rapidly, or effciently in vitro. Supporting this hypothesis, knockout of ISL1 in our differentiations Mimicking Islet Development with Human Pluripotent Stem Cells
reduces alpha and beta differentiation relative to EC, and its overexpression achieves the opposite (Veres et al., unpublished). The cause of SC-EC differentiation may be as simple as a developmental event that drives robust ISL1 induction in vivo that is absent in vitro, and identifying and mimicking these cues would enable suppression of the EC fate.
There may be a deep similarity and interconvertibility between beta and EC cells. They are each the most abundant endocrine cell type in their respective tissues, yet they have disjointed tissue distributions. There is also plasticity in both cell types to acquire functional elements of the other. Subsets of beta cells synthesize serotonin during pregnancy [62], which has been described as a reversible cell state but-since serotonin-synthesizing beta cells have not been profled by scRNA-seqmay yet prove to be transdifferentiation. For EC cells, the evidence is stronger: Foxo1 inhibitors cause them to gain insulin expression and to downregulate genes related to serotonin synthesis and secretion, likely acquiring a beta-like identity [10]. Similarities are also prominent in SC-islet differentiations, with SC-beta and EC cells differentiating simultaneously with gene expression commonalities. Under suitable conditions such as Foxo1 inhibition, SC-EC cells may in the future be induced to transdifferentiate into SC-beta cells. Finally, it is worth noting that the presence of EC cells has not been demonstrated in any assay to adversely affect SC-islet function. These cells can be eliminated or greatly reduced in numbers by alterations to the protocol.