Woodhead Publishing Series in Biomaterials
1 Sterilisation of tissues using ionising radiations
Edited by J. F. Kennedy, G. O. Phillips and P. A. Williams
2 Surfaces and interfaces for biomaterials
Edited by P. Vadgama
3 Molecular interfacial phenomena of polymers and biopolymers
Edited by C. Chen
4 Biomaterials, artificial organs and tissue engineering
Edited by L. Hench and J. Jones
5 Medical modelling R. Bibb
6 Artificial cells, cell engineering and therapy
Edited by S. Prakash
7 Biomedical polymers
Edited by M. Jenkins
8 Tissue engineering using ceramics and polymers
Edited by A. R. Boccaccini and J. Gough
9 Bioceramics and their clinical applications
Edited by T. Kokubo
10 Dental biomaterials
Edited by R. V. Curtis and T. F. Watson
11 Joint replacement technology
Edited by P. A. Revell
12 Natural-based polymers for biomedical applications
Edited by R. L. Reiss et al
13 Degradation rate of bioresorbable materials
Edited by F. J. Buchanan
14 Orthopaedic bone cements
Edited by S. Deb
15 Shape memory alloys for biomedical applications
Edited by T. Yoneyama and S. Miyazaki
16 Cellular response to biomaterials
Edited by L. Di Silvio
17 Biomaterials for treating skin loss
Edited by D. P. Orgill and C. Blanco
18 Biomaterials and tissue engineering in urology
Edited by J. Denstedt and A. Atala
19 Materials science for dentistry
B. W. Darvell
20 Bone repair biomaterials
Edited by J. A. Planell, S. M. Best, D. Lacroix and A. Merolli
21 Biomedical composites
Edited by L. Ambrosio
22 Drug–device combination products
Edited by A. Lewis
23 Biomaterials and regenerative medicine in ophthalmology
24
Edited by T. V. Chirila
Regenerative medicine and biomaterials for the repair of connective tissues
Edited by C. Archer and J. Ralphs
25 Metals for biomedical devices
Edited by M. Niinomi
26 Biointegration of medical implant materials: Science and design
Edited by C. P. Sharma
27 Biomaterials and devices for the circulatory system
Edited by T. Gourlay and R. Black
28 Surface modification of biomaterials: Methods analysis and applications
Edited by R. Williams
29 Biomaterials for artificial organs
Edited by M. Lysaght and T. Webster
30 Injectable biomaterials: Science and applications
Edited by B. Vernon
31 Biomedical hydrogels: Biochemistry, manufacture and medical applications
Edited by S. Rimmer
32 Preprosthetic and maxillofacial surgery: Biomaterials, bone grafting and tissue engineering
Edited by J. Ferri and E. Hunziker
33 Bioactive materials in medicine: Design and applications
Edited by X. Zhao, J. M. Courtney and H. Qian
34 Advanced wound repair therapies
Edited by D. Farrar
35 Electrospinning for tissue regeneration
Edited by L. Bosworth and S. Downes
36 Bioactive glasses: Materials, properties and applications
Edited by H. O. Ylänen
37 Coatings for biomedical applications
Edited by M. Driver
38 Progenitor and stem cell technologies and therapies
Edited by A. Atala
39 Biomaterials for spinal surgery
Edited by L. Ambrosio and E. Tanner
40 Minimized cardiopulmonary bypass techniques and technologies
Edited by T. Gourlay and S. Gunaydin
41 Wear of orthopaedic implants and artificial joints
Edited by S. Affatato
42 Biomaterials in plastic surgery: Breast implants
Edited by W. Peters, H. Brandon, K. L. Jerina, C. Wolf and V. L. Young
43 MEMS for biomedical applications
Edited by S. Bhansali and A. Vasudev
44 Durability and reliability of medical polymers
Edited by M. Jenkins and A. Stamboulis
45 Biosensors for medical applications
Edited by S. Higson
46 Sterilisation of biomaterials and medical devices
Edited by S. Lerouge and A. Simmons
47 The hip resurfacing handbook: A practical guide to the use and management of modern hip resurfacings
Edited by K. De Smet, P. Campbell and C. Van Der Straeten
48 Developments in tissue engineered and regenerative medicine products
J. Basu and J. W. Ludlow
49 Nanomedicine: Technologies and applications
Edited by T. J. Webster
50 Biocompatibility and performance of medical devices
Edited by J.-P. Boutrand
51 Medical robotics: Minimally invasive surgery
Edited by P. Gomes
52 Implantable sensor systems for medical applications
Edited by A. Inmann and D. Hodgins
53 Non-metallic biomaterials for tooth repair and replacement
Edited by P. Vallittu
54 Joining and assembly of medical materials and devices
Edited by Y. (Norman) Zhou and M. D. Breyen
55 Diamond-based materials for biomedical applications
Edited by R. Narayan
56 Nanomaterials in tissue engineering: Fabrication and applications
Edited by A. K. Gaharwar, S. Sant, M. J. Hancock and S. A. Hacking
57 Biomimetic biomaterials: Structure and applications
Edited by A. J. Ruys
58 Standardisation in cell and tissue engineering: Methods and protocols
Edited by V. Salih
59 Inhaler devices: Fundamentals, design and drug delivery
Edited by P. Prokopovich
60 Bio-tribocorrosion in biomaterials and medical implants
Edited by Y. Yan
61 Microfluidic devices for biomedical applications
Edited by X.-J. James Li and Y. Zhou
62 Decontamination in hospitals and healthcare
Edited by J. T. Walker
63 Biomedical imaging: Applications and advances
Edited by P. Morris
64 Characterization of biomaterials
Edited by M. Jaffe, W. Hammond, P. Tolias and T. Arinzeh
65 Biomaterials and medical tribology
Edited by J. Paolo Davim
66 Biomaterials for cancer therapeutics: Diagnosis, prevention and therapy
Edited by K. Park
67 New functional biomaterials for medicine and healthcare
E. P. Ivanova, K. Bazaka and R. J. Crawford
68 Porous silicon for biomedical applications
Edited by H. A. Santos
69 A practical approach to spinal trauma
Edited by H. N. Bajaj and S. Katoch
70 Rapid prototyping of biomaterials: Principles and applications
Edited by R. Narayan
71 Cardiac regeneration and repair Volume 1: Pathology and therapies
Edited by R.-K. Li and R. D. Weisel
72 Cardiac regeneration and repair Volume 2: Biomaterials and tissue engineering
Edited by R.-K. Li and R. D. Weisel
73 Semiconducting silicon nanowires for biomedical applications
Edited by J. L. Coffer
74 Silk biomaterials for tissue engineering and regenerative medicine
Edited by S. Kundu
75 Biomaterials for bone regeneration: Novel techniques and applications
Edited by P. Dubruel and S. Van Vlierberghe
76 Biomedical foams for tissue engineering applications
Edited by P. Netti
77 Precious metals for biomedical applications
Edited by N. Baltzer and T. Copponnex
78 Bone substitute biomaterials
Edited by K. Mallick
79 Regulatory affairs for biomaterials and medical devices
Edited by S. F. Amato and R. Ezzell
80 Joint replacement technology Second edition
Edited by P. A. Revell
81 Computational modelling of biomechanics and biotribology in the musculoskeletal system: Biomaterials and tissues
Edited by Z. Jin
82 Biophotonics for medical applications
Edited by I. Meglinski
83 Modelling degradation of bioresorbable polymeric medical devices
Edited by J. Pan
84 Perspectives in total hip arthroplasty: Advances in biomaterials and their tribological interactions
S. Affatato
85 Tissue engineering using ceramics and polymers Second edition
Edited by A. R. Boccaccini and P. X. Ma
86 Biomaterials and medical-device associated infections
Edited by L. Barnes and I. R. Cooper
87 Surgical techniques in total knee arthroplasty (TKA) and alternative procedures
Edited by S. Affatato
88 Lanthanide oxide nanoparticles for molecular imaging and therapeutics
G. H. Lee
89 Surface modification of magnesium and its alloys for biomedical applications
Volume 1: Biological interactions, mechanical properties and testing
Edited by T. S. N. Sankara Narayanan, I. S. Park and M. H. Lee
90 Surface modification of magnesium and its alloys for biomedical applications
Volume 2: Modification and coating techniques
Edited by T. S. N. Sankara Narayanan, I. S. Park and M. H. Lee
91 Medical modelling: The application of advanced design and rapid prototyping techniques in medicine Second edition
Edited by R. Bibb, D. Eggbeer and A. Paterson
92 Switchable and responsive surfaces and materials for biomedical applications
Edited by Z. Zhang
93 Biomedical textiles for orthopaedic and surgical applications: Fundamentals, applications and tissue engineering
Edited by T. Blair
94 Surface coating and modification of metallic biomaterials
Edited by C. Wen
95 Hydroxyapatite (HAP) for biomedical applications
Edited by M. Mucalo
96 Implantable neuroprostheses for restoring function
Edited by K. Kilgore
97 Shape memory polymers for biomedical applications
Edited by L. Yahia
98 Regenerative engineering of musculoskeletal tissues and interfaces
Edited by S. P. Nukavarapu, J. W. Freeman and C. T. Laurencin
99 Advanced cardiac imaging
Edited by K. Nieman, O. Gaemperli, P. Lancellotti and S. Plein
100 Functional marine biomaterials: Properties and applications
Edited by S. K. Kim
101 Shoulder and elbow trauma and its complications Volume 1: The shoulder
Edited by R. M. Greiwe
102 Nanotechnology-enhanced orthopedic materials: Fabrications, applications and future trends
Edited by L. Yang
103 Medical devices: Regulations, standards and practices
Edited by S. Ramakrishna, L. Tian, C. Wang, S. L. and T. Wee Eong
104 Biomineralisation and biomaterials: Fundamentals and applications
Edited by C. Aparicio and M. Ginebra
105 Shoulder and elbow trauma and its complications Volume 2: The elbow
Edited by R. M. Greiwe
106 Characterisation and design of tissue scaffolds
Edited by P. Tomlins
107 Biosynthetic polymers for medical applications
Edited by L. Poole-Warren, P. Martens and R. Green
108 Advances in polyurethane biomaterials
Edited by S. L. Cooper
109 Nanocomposites for musculoskeletal tissue regeneration
Edited by H. Liu
110 Thin film coatings for biomaterials and biomedical applications
Edited by H. J. Griesser
111 Laser surface modification of biomaterials
Edited by R. Vilar
112 Biomaterials and regenerative medicine in ophthalmology Second edition
Edited by T. V. Chirila and D. Harkin
113 Extracellular matrix-derived medical implants in clinical medicine
Edited by D. Mooradian
114 Wound healing biomaterials Volume 1: Therapies and regeneration
Edited by M. S. Ågren
115 Wound healing biomaterials Volume 2: Functional biomaterials
Edited by M. S. Ågren
116 Materials for the direct restoration of teeth
J. Nicholson and B. Czarnecka
117 Science and principles of biodegradable and bioresorbable medical polymers
Edited by X. Zhang
118 Medical biosensors for point of care (POC) applications
Edited by R. Narayan
119 Biocompatibility of dental biomaterials
Edited by R. Shelton
120 Bioresorbable polymers for biomedical applications: From fundamentals to translational medicine
Edited by G. Perale
121 Bioinspired materials for medical applications
Edited by L. Rodrigues and M. Mota
122 Chitosan based biomaterials, Volume 1: Fundamentals
Edited by J. A. Jennings and J. D. Bumgardner
123 Chitosan based biomaterials, Volume 2: Tissue engineering and therapeutics
Edited by J. A. Jennings and J. D. Bumgardner
124 Material-tissue interfacial phenomena
Edited by P. Spencer
125 Mechanical testing of orthopaedic implants
Edited by E. Friis
126 Management of periprosthetic joint infections (PJIs)
Edited by A. J. Arts and J. A. P. Guerts
127 Bone response to dental implant materials
Edited by A. Piattelli
128 Orthodontic applications of biomaterials: A clinical guide
Edited by T. Eliades & W. A. Brantley
129 Biofilms and implantable medical devices: Infection and control
Edited by Y. Deng & W. Lv
Fundamentals of chitosan for biomedical applications 1
E. Vunain, A.K. Mishra, B.B. Mamba
University of South Africa, Johannesburg, South Africa
1.1 Introduction
Chitosan is a natural polysaccharide obtained from partial or full deacetylation of chitin (Hu et al., 2015; Thein-Han and Misra, 2009). Chitin, the source material for chitosan, is the most naturally abundant polysaccharide after cellulose (Dutta et al., 2004). Chitin is an important component of the cell wall of fungi, the exoskeleton of crustaceans, and insects. Chitosan is the N-deacetylated derivative of chitin, a linear and semicrystalline polysaccharide composed of glucosamine and N-acetyl glucosamine units linked by β(1→4) glycosidic bonds (Rinaudo, 2006) (Fig. 1.1). When the fraction of glucosamine units is greater than 50%, the polymer is commonly called chitosan and the number of glucosamine units is termed degree of deacetylation (DDA). Chitin, on the other hand, is the term when the N-acetyl glucosamine units are greater than 50% and the number of acetamido groups is termed the degree of acetylation (DA). Mathematically, DA = 1 − DDA (Dornish et al., 2001; VandeVord et al., 2002). It should be noted here that when the DDA of chitin reaches about 50% or greater (depending on the origin of the polymer and processing conditions), it becomes soluble in aqueous acidic media. Chitosan is insoluble in aqueous solution above pH 7, in its crystalline form. However, in dilute acids (pH < 6), the protonated free amino groups on glucosamine facilitate solubility of the polymeric molecule (Madihally and Matthew, 1999). In acidic media, the polysaccharide is converted into a polyelectrolyte. A polyelectrolyte is a polymer carrying either positively or negatively charged ionizable groups. Solubilization occurs by protonation of the –NH2 group on the C-2 position of the d-glucosamine repeat unit. Chitosan is the second most abundant natural biopolymer after cellulose in terms of availability to the extent of over 10 gigatons (1 × 1013) annually (Harish Prashanth and Tharanathan, 2007). Chitosan is a nontoxic, biodegradable polymer of high molecular weight and very similar to cellulose, a plant
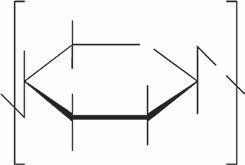
Figure 1.1 Molecular structure of chitosan polymer.
Chitosan Based Biomaterials, Volume 1. http://dx.doi.org/10.1016/B978-0-08-100230-8.00001-7
fiber. Depending on the source and preparation procedure, its molecular weight may range from 300 to over 1000 kDa with a DDA from 30% to 95%, though by convention, only polymers with greater than 50% DDA are referred to as chitosan. The functional properties of chitosan such as film formation, antimicrobial activity, and use as a thickening agent are related to its molecular weight and DDA. These two parameters are very important because they affect the potential applications on chitosans in various fields. For example, low molecular weight chitosan has low viscosity, which limits its applications, and oligomers of chitosan do not form films. Furthermore, the antimicrobial effect of chitosan is stronger if the molecular weight is greater than 100 kDa and has high DDA (No et al., 2002; Zheng and Zhu, 2003) (see Chapters 5 and 6 regarding changing DDA and MW). Additionally, chitosan is highly amenable to chemical modification via one of its three reactive functional groups: the amino group as well as both primary and secondary hydroxyl groups on C(2), C(3), and C(6) respectively (Fig. 1.1).
The presence of these functional groups allows for easy modification of the chitosan to attach molecules through procedures such as graft polymerization for specific applications. Furthermore, chitosan offers several advantages in biomedical applications, such as biocompatibility, biodegradability (Kenawy et al., 2015; Kumar et al., 2004), and that degradation products do not produce inflammatory reactions or toxic degradation products (i.e., its degradability products are nontoxic, noncarcinogenic, and nonimmunogenic) (Swetha et al., 2010). Due to its unique polycationic nature, controlled biodegradability, biocompatibility, nontoxicity, and bioresorbable nature, chitosan has wide application in medical fields for applications such as gene therapy (drug or gene delivery), wound dressing, tissue engineering, blood anticoagulant, hypocholesterolemic agents, antithrombogenic agents, bone regeneration biomaterials, and antimicrobial agents (Agnihotri et al., 2004; Danielsen et al., 2005; Di Martino et al., 2005; Ishihara et al., 2002; VandeVord et al., 2002). In addition, chitosan has been used in other industrial fields such as cosmetic preparations, paper and pulp, wastewater treatment, and food and feed additives (Balicka-Ramisz et al., 2007; Struszczyk et al., 2002).
Chitosan is a white to light red solid powder depending on processing conditions and levels of residual pigments. Its physiological functions include decreasing cholesterol, decreasing high blood pressure, inhibition of fat absorption, and improvement of intestinal microflora/environment. It has long been included in food and food additives due to its safety in humans, animals, and microbes (Zou et al., 2016; Ristić et al., 2015; Muzzarelli et al., 2012). Chitosan has several key properties that make it one of the most promising natural substances for application in medical and pharmaceutical fields. This chapter provides an insight into the fundamental applications of chitosan in the biomedical field.
1.1.1 Production/processing of chitin and chitosan
Shellfish processing results in large quantities of waste materials, which can be further processed into chitin, or its more valuable derivative, chitosan. The production of chitosan involves an acid treatment (decalcification), an alkaline treatment
(deproteinization), and a decolorization process and subsequent deacetylation typically with strong alkali under high temperature and pressure or via enzymatic processes (Puvvada et al., 2012). In the production process, there are several factors involved that are known to cause variability in the physicochemical properties of chitosan, such as alkaline concentration, incubation time, chitin alkali ratio, atmosphere, temperature, chitin source (including polymorphism), particle size, N-deacetylation conditions, and use of single or multiple step N-deacetylation processes (Van Den Broek et al., 2015). Although methods of production of chitin and chitosan may vary, they all invariably involve deproteinization and demineralization of crustacean shells. A number of factors such as the type of shell (e.g., shrimp, crab, lobster), shell freshness, and the portion of the shell used influence the amount of chitin present in a shell as well as chitosan yields (Arbia et al., 2013; Bough et al., 1978).
The chitin/chitosan processing involves the crushing and drying of shellfish waste (e.g., shrimp, crab, lobster, or any other suitable species of crustacean). The product is processed with acidic steps first and alkaline second to remove calcium mineral and protein, respectively. The treated shell obtained after this process is chitin, which is dried and packed. Chitin can further be treated with concentrated sodium hydroxide (NaOH) in a process known as deacetylation to yield chitosan (Fig. 1.2) (see Chapter 5).
Commercially, chitosan usually has a DDA in the range of 70–95% and a molecular weight of 104–106 g/mol (De Moura et al., 2011). Characterizing the relative
Figure 1.2 Deacetylation of chitin to chitosan.
proportion of N-deacetylated groups or the NH2 content is very important in the assessment of the efficacy of the chitin deacetylation process as well as suitability for any other chemical modification and medical use (Kasaai, 2009). Therefore, chitosan samples may present different characteristics in terms of deacetylation degree and molar weight distributions, which in turn define its application and influence the final performance of the biopolymer (de Moura et al., 2011; Tolaimate et al., 2000; Youn et al., 2007). For instance, studies have shown that the antimicrobial effect of chitosan is related to both molecular weight and DDA (Arancibia et al., 2015; Chen and Zhao, 2012). Also, the antibacterial activity of chitosan against Gram negative bacteria increases with decreasing molecular weight and pH (Younes et al., 2014).
1.2 Chemical and biological properties of chitosans
The chemical nature of chitosan provides many possibilities for covalent and ionic modifications, which allow for extensive adjustment of the biological and mechanical properties of chitosan-based medical devices. Unlike plant fibers, chitosan possesses positive ionic charges, which give it the ability to chemically bind with negatively charged fats, lipids, proteins, cholesterol, macromolecules, and metal ions (Li et al., 1992). Combining chitosan and simple aldehydes produces N-alkyl chitosan upon hydrogenation. The presence of the bulky substituents weakens the hydrogen bonds between chitosan chains. Therefore, N-alkyl chitosan swells in water in spite of the hydrophobicity of alkyl chains. The N-alkyl chitosan retains the film-forming property of chitosan (d’Ayala et al., 2008). The presence of the amino groups at the C-2 positions in chitosan makes it more versatile in comparison to chitin. Some chemical and biological properties (Dutta et al., 2004) of chitosan are described further. The presence of certain functionalities like –OH and –NH2 groups in the chitosan molecules provides a basis for interaction with other polymers and biological molecules.
1.2.1 Chemical properties
The chemical properties of chitosan are due to its
1. reactive amino groups, 2. linear polyamine structure, 3. available reactive hydroxyl groups, and 4. ability to chelate many transition metal ions.
1.2.2
Biological properties
Biological properties of chitosan (Kumar, 2000) are as follows:
1. biocompatible (Muzzarelli and Muzzarelli, 2005)
a. natural polymer (Mogosanu and Grumezescu, 2014)
b. safe and nontoxic (research in chitinase is an example in this respect) (Hector et al., 2016)
c. biodegradable to normal body constituents (Zou et al., 2016), 2. binds to mammalian and microbial cells
3. accelerates the formation of osteoblast responsible for bone formation
4. regenerative effect on connective gum tissue
5. central nervous system depressant
6. hemostatics
7. fungistatic
8. spermicidal
9. antitumor, and 10. immunoadjuvant.
These versatile properties of chitosan allow them to be easily processed into scaffolds (Duarte et al., 2010), nanoparticles (Csaba et al., 2009), beads (Gandhi et al., 2011), microparticles (Lameiro et al., 2006), nanofibers (Wijesena et al., 2015), membranes (Beppu et al., 2007; Song et al., 2015), and other forms (Fig. 1.3).
1.3 Modification of chitosans
From a pharmaceutical viewpoint, the modification of polymers has received much attention (Kenawy et al., 2015). Chitosan is the only cationic polysaccharide in
Figure 1.3 Schematic representation of the possibilities of processing chitin and chitosan into different forms (Anitha et al., 2014).
nature derived from biomass. Its unique physicochemical and biological properties give additional function over other polymeric biomaterials in pharmaceutical and biomedical applications. However, chitosan exhibits some limitations in its reactivity and processability. The scarce water solubility is a major limiting factor in its application. Therefore, surface modification of the chitosan to facilitate solubility and other properties has been sought for breakthroughs in utilization. Surface modification is a technique to improve physicochemical properties of polymers. A number of publications, books, and book chapters have reported different methods used for the modification of chitosan in the literature (Wang et al., 2016; Jiang et al., 2016). Modifications to the chitosan biopolymer can be achieved via chemical modification, physical interactions (inorganic composites and polyelectrolyte complexes), and other methods such as the use of avidin–biotin interaction. Although chitosan alone offers some excellent chemical properties for medical applications, some specially desired properties, such as targeting specific tissues, controlled drug delivery, and increased antimicrobial efficacy, can be achieved via chemical modification of the chitosan backbone (Sashiwa et al., 2003; Park et al., 2010; Kim et al., 2003; Venter et al., 2006).
1.3.1 Chemical modifications of chitosan
Despite the vast applications of chitosan, its utilization is substantially limited by its poor solubility in most solvents at neutral or high pH except in organic solvents. This is due to its inter- and intramolecular hydrogen bond network, low concentration of antioxidant molecules owing to the lack of H-atom donors, rigid physical properties, and high brittleness (Aljawish et al., 2015; Kanatt et al., 2008). To address this limitation, investigations into chitosan functionalization by chemical or enzymatic methods have been studied. A literature search reveals that chemical modifications of chitosan have been extensively investigated. A primary goal of modifying chitosan chemically is to provide derivatives that are soluble at neutral and basic pH values. In addition, chemical modifications control hydrophobic, cationic, and anionic properties as well as the attachment of various functional groups and ligands to the chitosan backbone. Chemical modification of chitosan is possible due to the amenable functional groups such as primary hydroxyl, secondary hydroxyl, and amino functional groups (Mourya and Inamdar, 2008) found in chitosan. Chemical modification of chitosan is known to alter the physicochemical properties such as electrostatic charge and permeability of polymer network but would not change the fundamental backbone of the chitosan polymer chain. It is noteworthy to mention that chemical modification brings new and improved properties such as biocompatibility of films and excellent physicochemical properties. Chemical modification of chitosan takes place by chemical linkages or blending with synthetic biopolymers, cross-linking with different physical or chemical reagents, radiation, photochemical, plasma-induced hydrophobization through alkylation reactions, surface coating of micro- or nanospheres with biocompatible synthetic polymers, and enzymatic grafting (d’Ayala et al., 2008; Kim et al., 2008). For the purpose of this chapter, we focus only on blending or chemical linkages of chitosan with synthetic biopolymers, chemical grafting, and enzymatic grafting of chitosans.
1.3.1.1
Blending or chemical linkages of chitosan with synthetic biopolymers
Blending of chitosan with other polymers is widely investigated. Blends with synthetic and natural polymers can imbibe the wide range of physicochemical properties and processing techniques of synthetic polymers as well as the biocompatibility and biological interactions of natural polymers (Kim et al., 2008). The combination of chitosans with other materials appears to be a common theme in many reports. Hsieh et al. (2005) blended chitosan with a hydrophilic and biodegradable polymer, γ-poly (glutamic acid) (γ-PGA), to modify chitosan matrices. The γ-PGA/chitosan composite matrix was found to have enhanced hydrophilicity and serum protein adsorption over chitosan alone, as well as increased maximum strength through the addition of γ-PGA. These composite properties could be useful in tissue engineering applications.
Jiang et al. (2006) synthesized poly(ethylene glycol)-grafted chitosan (chitosan-gPEG) by using the reaction between methoxy PEG nitrophenol carbonate and chitosan for the purpose of developing a gene therapy device. The average size of nanoparticles prepared from chitosan/DNA was slightly smaller than that of chitosan-g-PEG/DNA complexes in the media. However, the chitosan–DNA complexes were highly susceptible to aggregation in the presence of serum and bile. In this work, the PEG-grafted chitosans effectively shielded the positively charged chitosan surface when compared to unmodified chitosans. Moreover, the PEG-grafted chitosans were able to maintain complex sizes in the presence of bile and serum as well as providing protection to the complexed DNA against enzymatic degradation, thus leading to an improvement in the transfection efficiency in vivo. Lameiro et al. (2006) and Thein-Han et al. (2009) blended chitosan with gelatin scaffolds to improve biological activity for tissue engineering applications. Incorporation of gelatin into chitosan altered the stiffness of the scaffolds and biocompatibility of the scaffolds. In comparison with pure chitosan, cell spreading and proliferation were greater in chitosan–gelatin composites. Similarly, Chung et al. (2002a, 2002b) prepared galactosylated chitosan-based scaffolds by combining with alginate to improve mechanical properties and biocompatibility.
A highly porous sponge composed of calcium alginate and galactosylated chitosan (ALG-GC) sponge was fabricated through simple freeze-drying techniques. The morphology of the ALG/GC sponge could be controlled by varying the content of galactosylated chitosan, molecular weight, and the freezing temperature. Results show that the incorporation of alginate into chitosan improved mechanical properties for the ALG sponge and offered improved morphology of the pores and shape, which resulted in higher viability than the pure alginate. The scaffolds could be used for hepatocyte culture and transplantation.
1.3.1.2 Chemical grafting of chitosan
The possibility of grafting synthetic polymer (graft copolymerization) to chitosan has been explored in recent literature as a new way to modify the polysaccharide. Graft copolymerization reactions introduce side chains and lead to the formation of new derivatives (hybrid materials) composed of natural and synthetic polymers. Grafting of chitosan has been performed through various routes such as ceric ion initiation,
energy radiation, Fenton’s reagent, addition of various radicals, photo-induced methods, and nonradical-based mechanisms such as ring-opening methods and “grafting onto” methods (Jenkins and Hudson, 2001). Grafting chitosan is a common method to improve chitosan properties through modifications such as the formation of inclusion complexes (Chen and Wang, 2001), bacteriostatic effect (Jung et al., 1999), or to improve adsorption properties (Borchard et al., 1996; Lueβen et al., 1996). The method alters the polysaccharide backbone and modifies chitosan physicochemical properties. The modified chitosan retains many useful characteristics for biomedical applications such as biocompatibility (Ono et al., 2000), biodegradability (Guo et al., 2015), and mucoadhesivity (Roldo et al., 2004).
Homopolymers and copolymers of lactic acid have been widely utilized in drug release and suture systems due to their biodegradability (Sashiwa and Aiba, 2004), and copolymers of chitosan and lactic acid derivatives have been explored for medical applications. Luckachan and Pillai (2006) synthesized chitosan/ oligo l-lactide graft copolymers by ring-opening polymerization of l-lactide in dimethyl sulfoxide solution at 90°C under nitrogen using Ti(OBu)4 as catalyst. A graft copolymer with increased hydrophilicity and controlled degradation rate was obtained with possible application in wound dressing and in controlled drug delivery. By using this method of synthesis, the combined advantages of synthetic and natural polymers such as biodegradation and bioactivity were obtained. In a related study, Wu et al. (2005) synthesized amphiphilic chitosan–polylactide graft copolymers and evaluated their potential for biomedical applications. Results showed the chitosan–polylactide polymeric micelles have a hydrophobic core which could be used as promising carrier for the entrapment and controlled release of hydrophobic drugs. Grafted copolymerization of vinyl monomers onto chitosan using free radical initiation has been investigated by many researchers (d’Ayala et al., 2008). Furthermore, due to the electrically conductive nature of grafted chitosan materials, they have been employed in the field of biosensors (de Souza Costa-Junior et al., 2009; Luo et al., 2005). There are several reports regarding the use of grafted chitosans employed in biomedical applications such as tissue engineering, wound dressing, and cardiovascular applications and all these will be discussed in detail under biomedical application of chitosans.
1.3.1.3 Enzymatic grafting of chitosan
The chemical functionalization of chitosans often uses reactive agents and harsh conditions and requires chemical cross-linking reagents. More often, the use of some of these chemical reagents is environmentally harmful and may result in adverse effects on the human body when the polymer derivatives are used in foodstuffs and medicine ( Aljawish et al., 2015 ). Furthermore, the use of chemical cross-linking agents may lead to toxic effects and to secondary reactions with unwanted products ( Lin et al., 2005 ). Thus, considering environmental concerns, the functionalization of natural polymers using enzymes has attracted much interest as an alternative to toxic, environmentally harmful, and nonspecific chemical approaches ( Couto et al., 2005 ).
Enzymes are known to catalyze biological reactions with high rate and selectivity/ specificity without any permanent changes in their structure (Silavi et al., 2012). There are many advantages to the use of enzymes in polymer synthesis or functionalization. Considering environmental health and safety, enzymes offer the potential of eliminating the hazards linked with the use of reactive reagents. The main environmental benefit for the use of enzymes is the fact that their selectivity may be exploited to eliminate the need for full protection and deprotection steps during chemical synthesis (Bertoni et al., 2006; Gubitz and Paulo, 2003). Furthermore, specificity of enzymes may offer the potential for precise modification of macromolecular structure for a better control of polymer function (Aljawish et al., 2015; Cunha and Gandini, 2010). Chen et al. (2000) used an enzymatic method to graft hexyloxyphenol onto chitosan mediated by tyrosinase to alter its surface and rheological properties. The method employs tyrosinase to convert phenol into a reactive o-quinone, which undergoes subsequent nonenzymatic reaction with chitosan. Galactose molecules were coupled to chitosan using lactobionic acid bearing the galactose groups to obtain galactosylated chitosan grafted-poly(vinyl pyrrolidone). The prepared polymers were grafted with dextran and its physicochemical properties examined (Park et al., 2003). The polymer could be used as a hepatocyte-targeting gene delivery system since galactose functions as a specific ligand to asialoglycoprotein receptors (ASGRs) and dextran sulfate serves as the hydrophilic ligand. Furthermore, Aberg et al. (2004) employed an enzymatic method to graft peptides from casein hydrolysate to chitosan. Tyrosinase was used to initiate the grafting of peptides onto the amine-containing polysaccharide chitosan. This could be contrasted with chemical modification of polysaccharides that lack amine groups (e.g., cellulose). The tyrosinase provided a simple and safe way to convert food processing by-products such as polysaccharides into environmentally friendly and biodegradable products. Indeed, these products were even safe to consume considering the fact that all the components such as chitosan, casein hydrolysates, and mushroom tyrosinase are all derived from food manufacturing. Therefore, tyrosinase-catalyze grafting of peptides onto chitosan could be considered as a “green” alternative for conferring viscoelasticity to aqueous solutions (Aberg et al., 2004).
1.4 Some chitosan derivatives
1.4.1
Carboxymethylchitosan (CM-chitosan) is an amphoteric polymer whose solubility depends on pH. It stands as the most explored derivation of chitosan. Under controlled reaction conditions (with sodium monochloroacetate in the presence of NaOH), Oand N-carboxymethylation is obtained (Rinaudo, 2006). The reactive sites for the carboxymethylation of chitosan are the amino and hydroxyl groups present in its chain. The properties and applications of carboxymethylchitosan are dependent on its structural characteristics such as the average degree of substitution and the locus (amino or hydroxyl groups) of the carboxymethylation (Broerman et al., 1985). Chitosan and carboxymethylchitosan are used in agriculture for inhibiting the growth of fungi and
bacteria during storage of fruits and vegetables and in the medical and pharmaceutical areas mainly for the controlled release of drugs, orthopedic devices, and tissue adhesion (Broerman et al., 1985; Zhao et al., 2001).
1.4.2 Quaternized chitosan
Quaternized chitosan is prepared by introducing a quaternary ammonium group on a dissociative hydroxyl group or amino group of the chitosan. The quaternized chitosan derivative exhibits improved water solubility and stronger antibacterial activity over a broad pH range relative to chitosan ( Tan et al., 2013a ). Therefore, quaternary modification may increase the potential biomedical applications of chitosan in the field of anti-infective drugs and biomaterials. Examples are quaternized chitosan, N-substituted carboxymethylchitosan derivative, and water-soluble quaternized chitosan (for further details on quaternization and other derivatization reactions of chitosan, see Chapter 9 on antimicrobial applications of chitosan in volume 2).
1.4.3 Sugar-modified chitosan
Hall and Yalpani were the first to report the modification of chitosan with sugars (Mourya and Inamdar, 2008; Sashiwa and Aiba, 2004). They synthesized sugar-bound chitosan by reductive N-alkylation using sodium cyanoborohydride (NaCNBH3) and unmodified sugar or sugar–aldehyde derivative. The synthesis of sugar-bound chitosan, such as chitosan bound with D- and l-fucose, was studied for specific interaction with lectin and cells in studies by Morimoto et al. (Li et al., 1999, 2000; Morimoto et al., 2001). Lactose-modified chitosan for application in the repair of the articular cartilage was synthesized by Donati et al. (2005) using the same method of preparation. Kato et al. (2001a) prepared lactosaminated N-succinyl chitosan and its fluorescein thiocarbonyl derivative as a liver-specific drug carrier in mice through ASGR. Furthermore, lactosaminated N-succinyl chitosan was found to be a possibly advantageous drug carrier for mitomycin C treatment of liver metastasis (Kato et al., 2001b). Sialic acid is a sugar of the glycolipids and glycoproteins on the mammalian cell surface and is the key epitope recognized as essential for a number of pathogenic infections. Sialic acid-containing polymers are potent inhibitors of hemagglutination of human erythrocytes by influenza viruses (Roy and LaferriLaferriè, 1998; Sashiwa and Aiba, 2004). Other sugar derivatives include amylase-grafted chitosan and products obtained from ascorbic acid, among others.
1.4.4 Alkyl chitosans
Alkylated chitosans are amphiphilic polymers based on polysaccharides. The first reported derivative having these characteristics was a C-10-alkyl glycoside-branched chitosan with a high degree of substitution (DS = 1.5), which gelled when heated over 50°C (Rinaudo, 2006). Another synthesis approach was used for selective Nand O-palmitoylation, which gives a derivative with two or three long alkyl chains
Another random document with no related content on Scribd:
manier waarop de ouders met hun jongen omgingen, maakte, dat Piet nooit achterbaks behoefde te zijn.
Toen mijnheer uit zijn studeerkamer kwam, kon zijn vrouw niet nalaten hem te vragen, of hij niets bizonders aan Piet had opgemerkt.
„Aan Piet!” zei mijnheer heel verbaasd. „De jongen was net als anders. Wat haal je je nu weer voor muizenissen in ’t hoofd?”
Het antwoord bevredigde mevrouw maar matig en zonder er verder op door te gaan, bleven haar gedachten nog lang bij haar jongen verwijlen.
En zoo gebeurde het, dat, toen reeds alles in diepe rust was, zij nog met open oogen lag en den slaap niet kon vatten.
Ze had de klok reeds elf en half twaalf hooren [104]slaan, keerde zich om en om en voelde zich hoogst onrustig.
Plotseling hoort zij in de stilte van den nacht een geluid als het piepen van een raam dat opengeschoven wordt.
Even denkt ze nog dat het bij de buren is, doch dan, als gedreven door een voorgevoel, staat ze haastig op, en begeeft zich ijlings naar Piet’s kamertje.
Ze opent de deur.…! Daar staan moeder en zoon tegenover elkaar. Piet, met jas aan en pet op, juist gereed om het raam uit te klimmen, blijft als versteend staan.
Zijn moeder kijkt hem met een mengeling van verbazing en verdriet aan en dit stille kijken treft den jongen dieper dan hevige verwijten hadden kunnen doen.
En Piet, het Opperhoofd der Inka’s, de ontdekker van het Hol van Kaan, barst in snikken uit, terwijl hij, net als toen hij nog klein was, z’n hoofd tegen haar schouder aan verbergt.
„Wàt wilde je doen, jongen, nu mag je ’t me toch vertellen,” vraagt ze zacht.
Ze gaat op den rand van zijn bed zitten en Piet vertelt haar met horten en stooten het geheele plan.
Hij ziet er zóó ontdaan en verdrietig uit, dat moeder medelijden met hem heeft, want ze voelt heel goed, wat ’t voor hem is, zijn makkers te verraden.
„Dàt was ’t dus, Piet. Ja, moeder voelde wel, [105]dat er iets gaande was. Ik wil je verder geen verwijten maken, alleen wil ik je zeggen, dat je een domme, roekelooze jongen bent geweest. Want, denk je er eens even in, dat ik wat later was gekomen en je bed leeg had gevonden. Kun je voelen, vent, hoe ’n radelooze angst je ons dan bezorgd had?”
Weer ging Piet’s hoofd schuil in moeder’s schouder.
„En luister nu eens, jongen; diezelfde angst kunnen vannacht de ouders van drie jongens hebben. Mogen we dit toelaten?”
Bij die woorden kijkt Piet verschrikt op.
„Niet ze verraden,” wil hij zeggen. Maar moeder kijkt hem ernstig in de oogen en zegt:
„En als er nu vannacht, eens een ongeluk met een van je vriendjes gebeurt, Piet!… Denk eens aan Bob van Eest …”
„Dat is waar, moeder,” zegt Piet haastig. „Ze mogen niet gaan. Ik ga ’t ze zeggen.”
„Neen baas, dat zullen we vader laten doen. ’t Is wel hard, om hem uit z’n rust te halen, maar ’t is noodig.”
In minder dan geen tijd is mijnheer Kaan in de kleeren. Het was een heel werk geweest, hem uit z’n vasten slaap te halen en hem in korte trekken de toedracht der zaak duidelijk te maken.
Gelukkig nam hij het geval nogal niet te zwaar op. Piet kreeg een voorloopigen uitbrander met het bevel onmiddellijk naar bed te gaan.
En toen toog mijnheer er in den nacht op uit [106]om de drie andere roekelooze knapen op het rechte pad te brengen.
Het is precies vijf minuten over twaalven als mijnheer op den Nieuwen Binnenweg aankomt. Even vóór het huis waar Ambro woont, ziet hij drie kleine, donkere gedaanten heen en weer loopen.
„De schelmen,” bromt mijnheer in zichzelf. „Ze hebben ’t dan toch gewaagd.”
Hij besluit hen achterop te loopen. De jongens hebben er geen flauw idee van, dat ze bespied worden. Ambro, die tusschen Puckie en Chris loopt, raadpleegt z’n horloge en zegt ongeduldig:
„Piet komt niet, hoor! Dat valt me van hem tegen, hij is toch anders altijd van de partij.”
„Laten we een steentje tegen z’n raam gooien, misschien is ie in slaap gevallen,” zegt Chris.
„De wekker zal ie niet hebben laten afloopen,” grinnikt Puckie.
De laatste woorden werden gehoord door den heer Kaan, die het drietal zachtjes is genaderd.
„Zoo heeren,” klinkt z’n stem tamelijk hard door de stille straat. „Nog zóó laat op ’t pad?”
De drie nachtbrakers blijven als versteend staan.
„Waar gaat de tocht heen?”
„Wandelen naar Overschie,” zei Ambro, die als altijd de moedigste was.
„Een vreemd uur om zoo’n wandeling te maken. Weten jullie ouders daar wel van?”
„Natuurlijk niet, mijnheer, u begrijpt, dat we dan zooiets niet zouden mogen doen. Die slapen [107]nu allen gerust en hebben geen flauw idee, dat we niet in ons bed liggen.”
„Behalve de moeder van Piet,” zegt mijnheer. „Die slaapt niet gerust.”
„Nou mijnheer,” zegt Chris. „Piet is toch niet meegegaan.”
„Neen, dank zij de oplettendheid van zijn moeder, die hem op heeterdaad betrapte toen hij al met één been buiten het raam stond.”
„Zie je wel,” verdedigde Puckie, „Piet heeft ons niet verraden.”
Hiermede gaf hij eigenlijk een antwoord op de gedachten van alle drie.
„Neen jongens, je begrijpt ’t verkeerd. Over Piet is m’n vrouw niet meer in onrust, wel over drie andere roekelooze bengels.”
Het begon tot de jongens door te dringen en ze keken verlegen naar den grond. Ambro schopte denkbeeldige steentjes van de straat weg.
Er volgde een pijnlijke stilte, die eindelijk onderbroken werd door meneer Kaan.
„Nu, wat denken jullie te doen? Wordt het plan doorgezet?”
„We zijn stom geweest, meneer,” bekende Ambro volmondig. „We hebben aan alles wat u daar zegt, niet gedacht.”
„Wie weet, of mijn moeder nou ook niet in onrust zit,” zei Puckie, wiens geweten begon te spreken.
„Als ik jullie een goeden raad mag geven, kruip dan weer net zoo zacht je bed in, als je er uitgekomen [108]bent en vertel morgen aan je ouders wat je hadt willen doen. Ik hoop, dat jullie in het vervolg verstandiger zult zijn en ik vertrouw jullie nu verder.”
Meneer reikt ze daarop alle drie de hand en de druk van die drie jongenshanden bewijst hem, dat hij naar hun hart gesproken heeft en dergelijke grapjes niet weer zullen voorkomen.
Mijnheer verdwijnt in den donkeren nacht en haast zich huiswaarts, teneinde niets meer te verliezen van zijn kostbare nachtrust.
De jongens gaan op een drafje naar huis en ’t gelukte hen allen even vlug hun slaapvertrek te bereiken als ze dit verlaten hadden.
Den volgenden morgen, aan het ontbijt, vertelde Ambro de geschiedenis aan zijn ouders, die het eerst niet gelooven wilden en hem zeiden: „je hebt vast gedroomd.”
Maar toen het dienstmeisje, dat de thee binnenbracht, met een verbaasd gezicht vertelde, dat vanmorgen de knippen van de straatdeur waren en het raampje open stond, moesten zij het wel gelooven.
Nadat hij Ambro flink de les had gelezen, zei de heer Verbrugge:
„We kunnen meneer Kaan niet dankbaar genoeg zijn en ik wil niet wachten, hem onzen dank te gaan betuigen.”
Zoo dachten ook de ouders der andere jongens erover en meneer Kaan ontving dien dag drie dankbare vaders, die ’t er met hem over eens [109]waren, dat hun zoontjes schelmen van ’t zuiverste water waren.
De heer Kaan vond het noodig nog even tot hun verdediging aan te voeren, dat het wel rakkers waren, maar dat bij geen van het ondeugende stel een kwaad hart zat.
[Inhoud]
HET GEHEIMZINNIGE APPARAAT.
Ambro en Karel loopen langs de Schiekade. Ze komen van gymnastiek en daar ’t Woensdagmiddag is, willen ze nog naar het Hol, waar ze weten dat de jongens hen wachten.
Een steenen paal, langs den weg, lokt ze echter eerst tot haasjeover springen. De paal is hooger dan Ambro’s schouder.
Niettegenstaande dit, weet hij er met een flinken afzet handig over heen te komen.
Karel heeft er meer moeite mee en al eenige malen heeft zijn buik op een onaangename manier kennis gemaakt met den harden paal.
Eindelijk gelukt het hem zijn aanloop groot genoeg te nemen en hij vliegt met een vaart over den paal heen, waarbij echter dezen keer zijn zitvlak in zoo’n hevige botsing komt met den paal, dat dit een eind maakt aan het spel.
Ambro staat te gieren van pret om de pijnlijke grimassen die Karel maakt bij het wrijven langs bovengenoemd lichaamsdeel.
„Je bent ook een reuzen-os,” zegt Ambro.
„Je moet je aanloop grooter nemen.”
„Ik vertik ’t langer,” zegt Karel kwaad. [110]
„Als je nu niet meegaat, ga ik alleen.”
„Goed,” zegt Ambro. „En dan nemen we den paal mee.” Hij doet alsof hij den paal met alle geweld uit den grond wil trekken.
„Maciste, de groote krachtpatser is er niks bij,” lacht Karel. „Schiet op,” laat hij er op volgen, „anders komen we nooit verder.”
Het tweetal vervolgt zijn weg.
Maar daar krijgt Ambro het pontje in de gaten, dat voor twee centen de liefhebbers naar den overkant brengt.
„Nou weet ik een goeie,” zegt hij.
„Wat dan?”
„We zullen dien pontjes-baas er tusschen nemen.”
„Hoe dan?”
„Ik loop zoo hard als ik kan om, en als ik aan den overkant ben, wacht jij af aan welken kant het pontje ligt en dan doen we net of we elkaar niet kennen. Ligt het nou aan mìjn kant, dan doe jij net of je mee wil. Ik doe natuurlijk net zoo als ie aan joùw kant ligt. Vaart hij nou over, dan smeer je ’m! In dien tusschentijd sta ik aan de andere kant en probeer hetzelfde. Als dàt lukt, is ie volmaakt.”
„Potverdrie, ja! dàt zullen we ’m lappen,” zegt Karel.
In vollen draf rent Ambro de Heulbrug over en nu loopt het tweetal op gelijke hoogte, ieder aan een kant van de Schie. Het pontje komt juist, met twee juffrouwen als vracht naar Karel’s kant toe. [111]
Karel vervolgt kalm zijn weg.
Het pontje is intusschen aangekomen en Ambro staat aan den overkant kalm en geduldig te wachten, tot de pontjesbaas hem komt halen.
Deze keert dan ook onmiddellijk terug en zonder dat de man het in de gaten heeft, is Ambro reeds verdwenen en houdt hij zich schuil in het portiek van een huis.
De man begrijpt er niets van en zal wel gedacht hebben, dat hij droomde. Nog net ziet hij een jongetje staan en—weg is ie!
„Zoo’n aap,” bromt de man. „Laat me daar voor niks overvaren! Dat zal me geen tweeden keer gebeuren!”
Hij blijft nog even wachten en als hij eenige passagiers bij elkaar in z’n bootje heeft, onderneemt hij de reis naar den overkant.
Dit is een groote teleurstelling voor Karel, want nu het bootje vol is, kan hij hem den tocht niet te vergeefs laten maken. Dùs blijft hem niets anders over, dan Ambro te fluiten en hem toe te schreeuwen weer samen te komen op het Hofplein.
„Jammer, dat ie de tweede keer mislukte,” zegt Ambro, als ze weer bij elkaar zijn.
„Zeg, jôh, wat is ’t al laat; ga je mee naar ’t hol?”
„Mij best, de anderen zijn er nu ook.”
Nu gaat het in looppas naar den Dierentuin. Bij het hol gekomen, laat Ambro een zacht fluitsignaal hooren, dat onmiddellijk wordt beantwoord en, na even goed rond gekeken te hebben, schieten zij als konijnen weg tusschen de dichte bladeren. [112]
Op hun buik voortkruipend, komen ze eindelijk op hun zorgvuldig in orde gehouden paadje en bevinden zich alras in het hol, waar zij Chris en Puckie aantreffen. Beiden liggen te lezen.
„Wat zijn jullie laat,” zegt Chris.
„We hebben keet gemaakt aan de Schie.”
En ze vertellen hun maar half gelukte grap met den pontjesbaas.
Puckie vindt het „een flauwen bak”.
„Omdat jij ’m niet verzonnen hebt,” bijt Ambro hem toe.
„Neen, dan hebben wij een beteren voor vanavond,” zegt Chris. „Hè, Puckie?”
„Nou, ’n fijne!” stemt Puckie toe.
„’t Zal wat zijn,” smaalt Ambro.
„Als jij ’t niet verzint, kan ’t niet deugen,” zegt Chris.
„Wat zijn jullie strijdlustig vandaag,” zegt Karel, die zich behagelijk heeft neergevleid op den zachten grond en naar de plekjes blauw van den hemel kijkt, die door de boomen zichtbaar zijn.
„A la routschkia de Baviani, met den kikvorsch op den schouder, van de boesa van de bombernades!” zegt Ambro met een onweerstaanbaar mal gezicht.
„Wàt zeg je nou?” vragen de jongens vol verbazing.
„Dat is Russisch,” zegt Ambro gewichtig.
„Wat beteekent het?” vraagt Chris geamuseerd.
„Juffrouw, haal je katje naar binnen, want het begint te regenen.” [113]
„Och, malle, hou je snater,” giert Puckie.
„Toe, zeg ’t nog eens,” vraagt Chris.
„Neen, ik zing me liedje geen twee keer. Kom op met je plan, Chris, wat is ’t?”
En ze komen er mee voor den dag.
Chris neemt het woord en zegt met het gezicht van een toovenaar:
„Ik zie kans om in een heele straat belletje te trekken, door maar één bel aan te raken.”
„Da’s onmogelijk,” zegt Karel.
„Neen, dàt kan niet,” bevestigt Ambro.
„En òf ’t mogelijk is,” zegt Chris. „Vanavond, als ’t donker genoeg is, moeten jullie komen kijken en dan zal ik je bewijzen, dat ik waarheid spreek.”
„Top,” zegt Ambro. „Hoe laat moeten we komen, en waar?”
„Ik heb vioolles,” zegt Karel bedrukt.
„Tot hoe laat?”
„Tot half acht.”
„Nou, dat is net mooi, want dan is ’t donker genoeg om te beginnen.”
„Zeg nou es hoe je dat doet?” vraagt Ambro nieuwsgierig.
„Je zal ’t vanavond zien,” zegt Chris op vastberaden toon. „Komen jullie nou om kwart voor achten op de Heulbrug.”
„Wat een eind weg,” klaagt Karel.
„Ja, in een drukke straat gaat ’t niet.”
„Nou, vooruit dan. Ik zal er zijn. En we zullen Piet en Paul ook nog even waarschuwen.”
„Ja, da’s best.” [114]
De klok slaat half acht.
Op de Heulbrug wachten Chris en Puckie en kijken in donker uit, of ze de anderen nog niet zien verschijnen.
En jawel! in de verte hooren ze een vroolijken deun fluiten en even daarna komen hun makkers, in marsch-tempo, aangestapt.
„Hallo!” schreeuwt Ambro. „Daar waren we.”
„Het spul kan beginnen,” lacht Chris.
Op een drafje gaat ’t nu naar de bewuste zijstraat van den Bergweg waar het wonder vertoond zal worden.
Het is intusschen al goed donker geworden.
De straat, die Chris uitgezocht heeft tot het terrein zijner operatiën, is wel een der stilste van deze wijk, hetgeen voornamelijk komt, doordat ze doodloopt en begrensd wordt door een hooge houten schutting.
Geen enkel levend wezen vertoont zich in de straat, uitgezonderd een zwarte poes, die, door een krijgskreet van Ambro opgeschrikt, in dwaze vlucht wegijlt.
„Hou je bakkes,” zegt Chris met ingehouden woede. „Ze mogen ons niet hooren!”
Met „ze” bedoelde hij de argelooze bewoners der straat.
„Nou jongens,” zegt Chris. „Ik zal aan ’t laatste huis beginnen, mot je kijken.”
Hij haalt uit z’n zak een groot kluwen stevig touw. Het einde daarvan maakt hij vast aan den deurknop van het huis en loopt dan, het touw [115]flink strak houdend, naar den overkant, waar hij het touw aan de trekbel bevestigt.
Dan snijdt hij het touw af en bindt aan den deurknop van hetzelfde huis weer een eind vast, om vervolgens de bel van het huis aan den overkant daarmee te verbinden.
En zoo gaat hij voort, tot hij bij het zesde huis gekomen, bemerkt geen touw meer te hebben en den arbeid dus moet staken.
„Nou, opgelet, jongens,” zegt Chris. „Nou zal je een lol beleven.”
Mèt begeeft hij zich naar het eerste huis, waar hij het touw aan den deurknop vastbond en trekt hard aan de bel.
Het resultaat is schitterend!
In een portiek staan de jongens te kijken naar het effect.
De eerste deur wordt geopend, maar diezelfde deur brengt de bel van den overkant in beweging. Ook daar wordt geopend en met hetzelfde resultaat. En warempel, geen enkele deur die zijn plicht niet doet, geen enkele bel, die weigert.
Dan verschijnen zes hoofden aan beide zijden van de straat, en nijdige uitroepen weerklinken van „wie daar!!!” „Wie belt daar toch zoo!!”
Een juffrouw ontdekt het touw dat aan haar deurknop is bevestigd en in haar verwoede pogingen om het touw te verwijderen, klingelt, door
die beweging, de bel bij haar overbuurman, als bezeten door het huis, met het gevolg, dat een man in hemdsmouwen naar buiten komt rennen, die eveneens [116]het touw ontdekt en de verschrikte juffrouw aan den overkant vraagt, of ze gek is geworden en wat dat bellen van haar te beduiden heeft.
„Ik bel niet,” zegt de juffrouw nijdig.
„U belt wèl,” houdt de man vol.
„Nou kom dan kijken,” zegt de juffrouw met een hoofd als een kalkoenschen haan.
Intusschen zijn nog meer bewoners naar buiten gekomen, die geen van allen begrijpen hoe dit fopschellen zonder de aanwezigheid van kwajongens kan plaats hebben.
De jongens in het portiek rollen over elkaar van het lachen en besluiten, alvorens ontdekt te worden, het hazenpad te kiezen en in volle vaart hollen ze naar de Heulbrug terug. Ze worden niet achtervolgd, want de menschen hebben te veel werk om de touwen van hun knoppen en bellen te verwijderen, maar de liefelijkste verwenschingen worden ze achterna geroepen.
Geheel buiten adem door het harde loopen, komt het stel op de Heulbrug aan.
„Hè, hè!” zegt Ambro voldaan. „Hij was van den bakker! Je hebt er alle eer van, Chris! Hoe ben je op ’t idee gekomen?”
„Dat weet ik zelf niet. Maar we zullen ’t meer doen! ’t Is alleen jammer, dat je je touw kwijt raakt bij zoo’n grap. ’t Was vast een eind van 100 Meter.”
„Nou, dan leggen we botje bij botje en we koopen weer eens een kluw.”
Dit vonden ze allen best. [117]
Het was intusschen kwart over acht geworden en hoog tijd om naar huis te gaan.
Dus keerde de vroolijke troep lachend en joelend huiswaarts en Ambro droomde dien nacht dat hij stond in een grooten kring van juffrouwen en vloekende kerels die groote schellen in ’t rond zwaaiden en hem steeds dichter naderden.
[Inhoud]