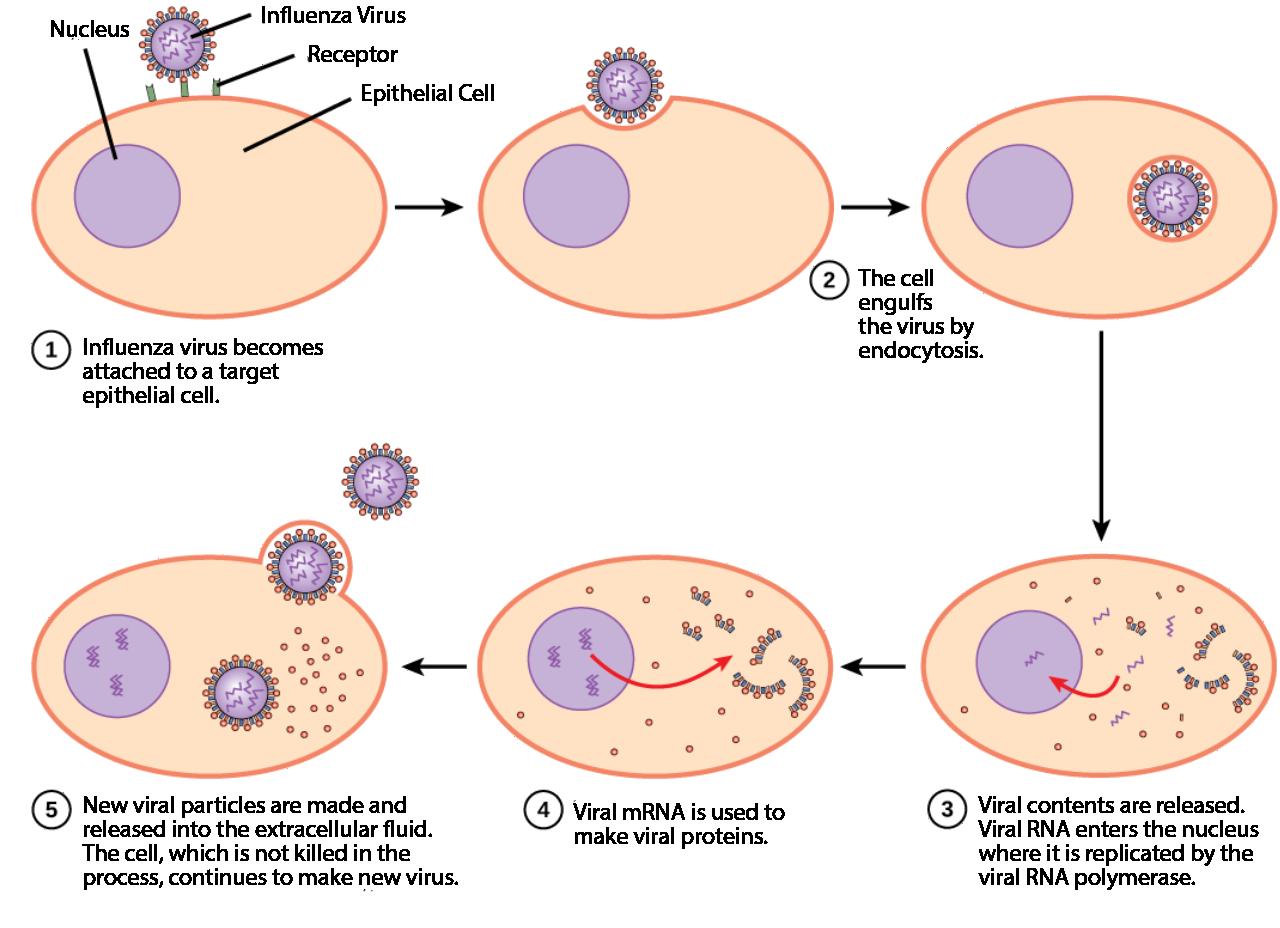
3 minute read
Engineering the Future with CRISPR/Cas9 Brian Lee
Engineering Engineering the Future with the Future with CRISPR/Cas9 CRISPR/Cas9
Chinese scientist He Jiankui made international headlines when he claimed to have created the world’s fi rst two genetically-modifi ed human babies. He applied CRIPSR/Cas9 to change their DNA so that they would be immune to HIV, a disease which attacks the body’s own immune system. Naturally, his unsanctioned experiments have stirred much controversy: where do scientists draw the line with genetic editing? Are genetic modifi cations safe in the real world? Can they potentially save lives?
Advertisement
What is genome editing? What is CRISPR/Cas9?
Genome editing involves tools that allow scientists to change DNA inside a cell. DNA is a large polymer made out of individual units called nucleotides. The cell has internal machinery which “reads through” the sequence of nucleotides in DNA to make proteins. DNA is the instruction set for the cell, dictating all of its functions. Genome editing
Written By Brian Lee Designed By Caitlyn Prabowo
is used to understand the functions of genes or to engineer a new diagnostic or therapeutic. Editing technologies are capable of removing or adding genes at very specifi c locations in the genome.
CRISPR/Cas9, short for Clustered Regularly Interspaced Short Palindromic Repeats and CRISPRassociated protein 9, is the most recent advancement in genome editing.1 It won the 2020 Nobel Prize in Chemistry for being a more accurate and more effi cient tool than a majority of other gene-editing methods available to scientists.2
Where did CRISPR/Cas9 come from? How does it work?
CRISPR/Cas9 actually comes from nature (with some modifi cations in the lab). Bacteria use CRISPR complexes to defend themselves very effectively from viral infections.1, 3
Since viruses are not ‘alive,’ they must rely on a host (i.e. cells in our bodies) to replicate the proteins they need for replication. Figure 1 summarizes this process. Just like our cells, viruses rely on DNA to encode the instructions to build their components; therefore, if a bacterial cell had a method to destroy a virus’s DNA, it would stop the viral infection in its tracks. This is where the genius of CRISPR/Cas9 comes in.
After a viral infection, the bacteria will capture segments of the virus’s DNA to create a “spacer” CRISPR sequence. When the bacterium is invaded again, this spacer sequence is the key to the bacterium’s self-defense: it can be used to search out viral DNA in the cell. If a match is found, the Cas9 enzyme would bind to the identifi ed viral DNA and then (physically) cut it, effectively disabling the virus by halting replication.3 This process is depicted below in Figure 2.
CRISPR/Cas9 is revolutionary because of this ability to shut off specifi c portions of the genome: potentially, if a scientist has a specifi c stretch of DNA in mind, they can use its sequence to fi nd a unique spacer sequence. Then, they can proceed to engineer a CRISPR/Cas9 complex that can be used to specifi cally turn off that portion of a transcript within a model organism (i.e. bacteria), allowing for very precise alterations in model organism genomes.1
How can CRISPR/Cas9 transform medicine?
Given its ability to specifi cally alter unique genes, CRISPR/Cas9 has the potential to cure a range of conditions with a signifi cant genetic/ heritable component, such as genetic/congenital neurodegenerative diseases, blood disorders, cancers, or ocular disorders.
CRISPR/Cas9 technology is already being used to treat genetic diseases. For example, Sickle cell disease is caused by a mutation in the HBB gene that leads to malformations in hemoglobin, the essential oxygen-carrying protein in red blood cells. These malformations may lead to red blood cells collapsing into a dysfunctional ‘sickle’ shape, giving the disease its name. Since sickle cell disease currently has no cure, scientists have investigated using CRISPR/ Cas9 to undo the genetic mutation in HBB. Using CRISPR/Cas9, scientists targeted enhancers key in