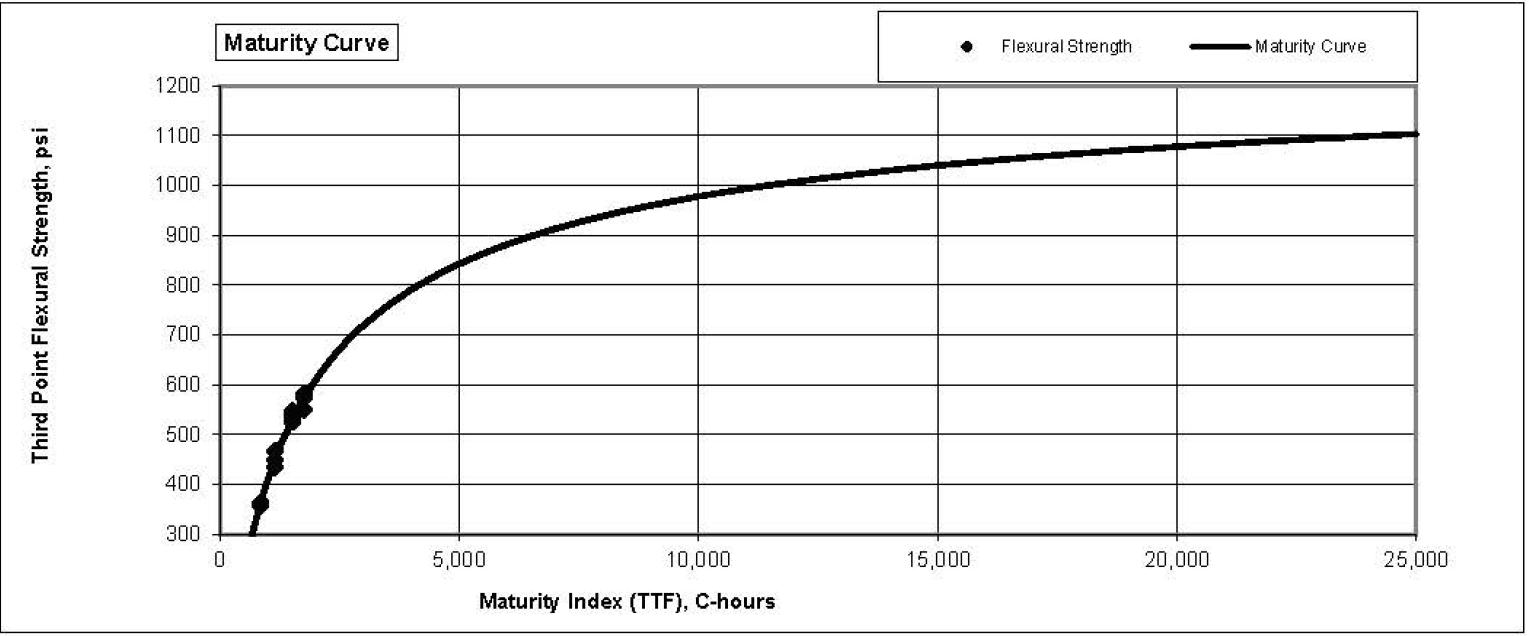
16 minute read
Table 1: Strength criteria from PennDOT 408 (2021
Traffic opening criteria can be based on age, compressive strength, flexural strength, or some combination. Compressive strength is the most common test for strength in the field, however, the measurement of cracking resistance is indirect. Flexural testing is more representative of the stresses a pavement experiences and is therefore a better indicator of cracking resistance. However, flexural strength testing is more complex when compared to the simplicity of compressive strength testing. Regardless of the strength test chosen, testing needs to be done after 1, 3, 7, and 28 days to properly establish the strength gain for conventional concrete (ASTM C39).
Most states have at least an age and compressive strength requirement, although, the age requirement is often secondary so the pavement can be opened whenever the strength criteria is met even if it is before the age criterion. For conventional concrete, the age, compressive strength, and flexural strength criteria ranges between 7 to 14 days, 2600 to 3500 psi, and 400 to 750 psi, respectively. PennDOT 408 requires various compressive or flexural strengths depending on the slab thickness and length (Table 1). However, often with the desire to open a pavement to traffic early, high early strength concrete is used instead of conventional concrete. High early strength concrete has its own set of criteria differing between states as well. For high early strength concrete, the age, compressive strength, and flexural strength criteria ranges between 4 to 24 hours, 1200 to 3500 psi, and 290 to 420 psi, respectively (Zayed, 2018). PennDOT 408 requires a minimum of 1,200 psi compressive strength to open to traffic for high early strength concrete. PennDOT also allows compressive strength to be measured using the standard destructive method or using the non-destructive maturity method.
Slab Thickness, in
6.0 7.0 8.0 9.0 10.0 + Table 1: Strength criteria from PennDOT 408 (2021)
f’c Strength for Opening to Traffic, psi Slab Length < 10 ft Slab Length ≥ 10 ft MR (3rd point loading) f’c MR (3rd point loading)
3000 490 3600 540
2400 370 2700 410
2150 340 2150 340
2000 2000 275 250 2000 2000 300 300
The existing criteria, regardless of the state, do not account for time of construction, design features, early age traffic load conditions, climate conditions, edge support conditions, and other factors that affect early age pavement performance (ACPA, 1994). The current methods also ignore the effect early opening has on the long-term performance. This limits the accuracy of cost-benefit decisions for the life of the pavement when considering when to open to traffic. Another restriction of the current criteria is that there is no separation between traffic levels. The stresses applied by lightweight, or passenger vehicles will be lower than heavy load vehicles. Therefore, smaller vehicles could be allowed on the pavement before the strength
criteria is reached for full traffic allowance without compromising long-term performance. This would limit the short-term traffic disruption caused by the construction.
2 Nondestructive Testing
Destructive testing is the most common method of strength testing. Compressive or flexural strength is used as the opening criteria for every state. However, there are significant drawbacks to these methods. Large number of samples are necessary for testing because to establish strength gain, multiple rounds of testing must be performed at specific times along with the curing process. This introduces variability into the test because each sample is created by hand versus the consistent machinery used to pave the in-situ pavement. Another issue is that the separate samples have different environmental and boundary conditions than the pavement. Environmental chambers are used to replicate the average outdoor climate condition for the location and date, however, common fluctuations of climate conditions, such as temperature and moisture, cannot be replicated in a laboratory. There is also variability within the same slab that can be due to small environmental, mixture, or placement differences. The limited number and size of the samples makes it more challenging to consider such effects. The limitations have led to other testing methods being used alongside destructive methods to improve concrete pavement strength gain monitoring.
Although destructive testing is still wildly used by Departments of Transportation, nondestructive testing is gaining popularity. Many departments, including Pennsylvania, have begun accepting nondestructive methods as acceptable measurements of compressive strength to be used for strength opening criteria. Nondestructive testing allows the user to inspect, monitor, or test systems without removing materials from the structure (Helal, 2015). The key aspect of this testing method is that it leaves minimal to no impact on the structure while providing material property estimations that account for the environmental factors in-situ pavements experience. Specifically, within concrete pavements, nondestructive testing has been performed for years to measure various concrete properties.
There are various test methods that can measure or estimate a variety of concrete properties including moisture content, density, thickness, and elastic modulus (Crawford, 1997). Each nondestructive method involves different technologies with some being imbedded within the pavement, attached to the surface, or a portable machine. Nondestructive testing, despite the method used, can be performed directly on the concrete that will be in service. This allows the test to account for the effect of environmental, boundary, or curing conditions that may not be repeatable in a laboratory setting. In addition, separate samples are unnecessary in the field in comparison to destructive tests like compressive or flexural strength testing which require many samples. Many methods are also able to gather data continuously or are quick enough to be done often so that more data can be obtained. Another advantage for many methods of nondestructive
testing is the portability. Multiple locations on a slab can be monitored to account for variability within a single slab, monitor the strength of critical stress locations, or check areas flagged for potential errors.
This project is specifically interested in those that can estimate the elastic modulus as it can be related to concrete strength. A few popular methods include maturity, ultrasonic tomography, ground penetrating radar, electromechanical impedance, and sounding methods (Freeseman, 2016). Maturity is a method for concrete strength estimation that involves temperature sensors previously embedded within the concrete and is accepted for use by PennDOT. The other listed involve emitting different types of waves into the concrete and analyzing the returning signal to estimate various concrete material properties or locate damage or internal boundaries. For this project, maturity and ultrasonic tomography were chosen to estimate early age concrete strength to determine improved opening times.
2.1 Maturity
Maturity is a well-established, nondestructive method for concrete strength estimation and has been accepted by many Departments of Transportation. This method has been used for many years in research to reliably estimate concrete strength (Crovetti and Khazanovich, 2005; Helal, 2015; Soutsos, 2020; Soutsos, 2021; Freeseman, 2016; Hong, 2020; Olek, 2002; Hsu, 2002; Mancio, 2004; Mynarcik, 2013; Nazarian, 2003; Wilson, 2020) and has an ASTM standard for consistent use (ASTM C1074).
The maturity technique is a non-destructive method that is used to evaluate the strength development of cementitious materials using the combined effects of time and temperature (Carino and Lew, 2001). The hydration reactions between cement and water are exothermic. The amount of heat released by the mixture during the hydration process is an indicator of the amount of produced hydration products. Therefore, there is a correlation between the heat generated by the mixture and the developed strength. Maturity testing involves imbedding temperature sensors in the concrete, as it is poured, at specific depths. Temperature data is continuously gathered and is used in a strength-maturity relationship previously calculated in laboratory work. This allows immediate evaluation of concrete strength at any given time. Maturity is commonly used to determine when a concrete pavement reaches the required strength to open to traffic. Furthermore, the strength-maturity relationship allows for future strength gain to be estimated (Crovetti and Khazanovich, 2005; Van Dam et al., 2005).
The relation between temperature and strength is unique for each concrete mixture, therefore, before use in the field, pre-construction laboratory work is required. ASTM C1074 is the standard for estimating concrete strength using the maturity method. This method has been proven to provide accurate strength estimations and maturity has been accepted by many Departments of Transportation as an acceptable strength estimation. Minnesota Department of Transportation (MnDOT) has been using maturity method in their pavement procedures with
success for some time and have a well-established procedure for creating the strength-maturity curve. The development of a maturity-strength relationship is performed in three broad steps:
1. Determining the maturity-strength curve in a lab or in the field. 2. Estimating the in-situ strength in the field. 3. Verifying the maturity-strength relationship in the field.
To use the maturity method, a maturity meter (or temperature sensors) and a data logger are needed along with the apparatuses to test either flexural or compressive strength. Calculating maturity in Celsius is preferred over Fahrenheit for easier computation. In the laboratory, the concrete strength test method can be either flexural or compressive strength. If flexural is chosen, 15 beam specimens are needed for testing with at least two containing temperature sensors. If compressive is chosen, 17 cylinder specimens are needed, again with two containing temperature sensors. MnDOT suggests different testing ages depending on the type of concrete used. For example, normal strength concrete is tested at 1, 2, 3, 7, and 28 days. Three specimens are tested at each age with the specimens containing the temperature sensors being tested on the last day.
The Nurse-Saul method, described in the ASTM C1074 and MnDOT procedures, is commonly used to develop the maturity-strength curve by computing the concrete maturity using laboratory temperature data at each testing age using Equation (1). This equation calculates the time-temperature factor (TTF) which will be related to the measured concrete strength at that particular TTF value. TTF is also known as the maturity index (M(t)).
(1)
where:
TTF is the temperature-time factor at age t, degree-days or degree-hours
is a time interval, days or hours
is the mean concrete temperature during the time interval , °C
is the datum temperature set to be equal to 0 °C in this study,
An example of the developed maturity-strength curve can be seen in Figure 1. Note the points represent the measured strength and the respective maturities indices. The complete maturity curve is then developed using these points.
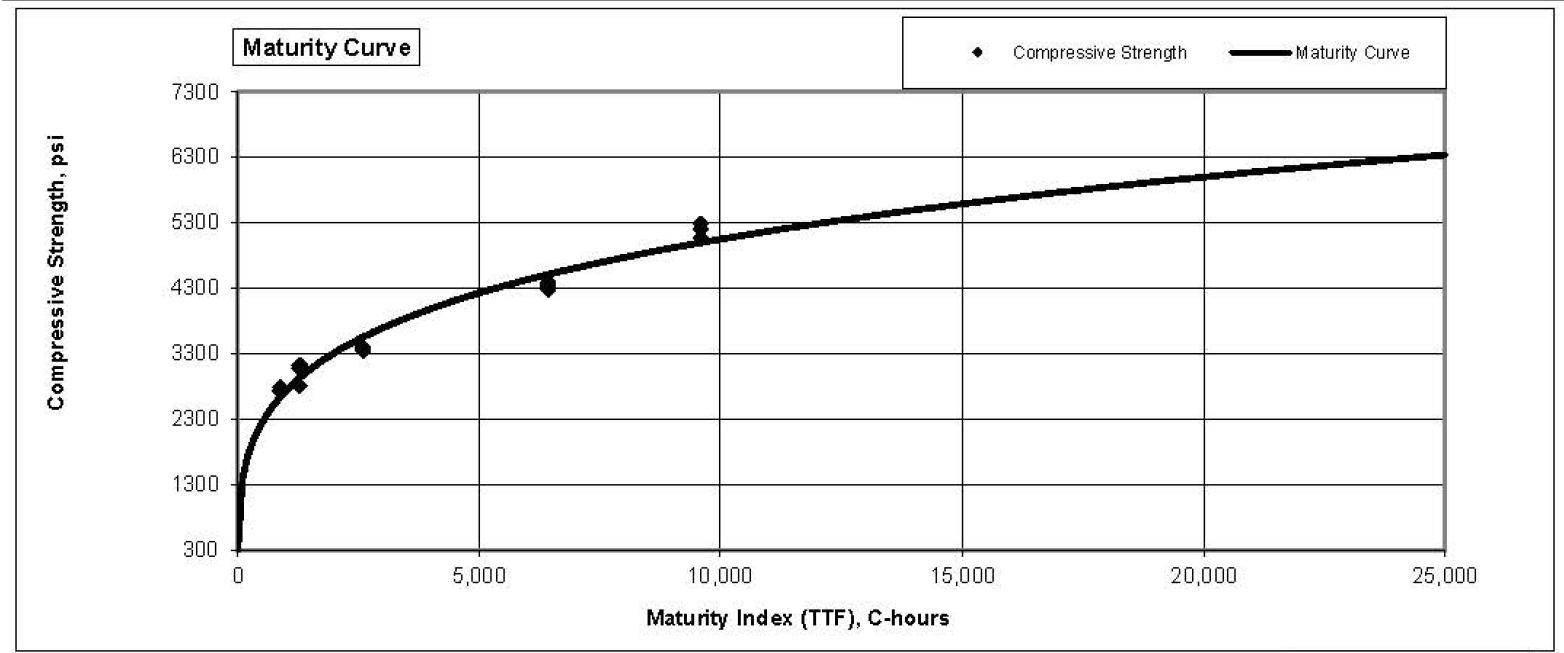
Figure 1: Example of developed maturity curves (MnDOT Specifications 2461.3.G.6, 2020)
The primary benefit of maturity method is the continuous strength estimations which can be used to better time critical construction scheduling such as joint cutting, form removal, and opening to traffic. Another major advantage is the predictive ability of the strength-maturity relationship which can be used to improve construction scheduling and guide future activities. Maturity also removes the variability destructive testing has from different environmental and curing conditions in the field as compared to in a laboratory.
However, there are also limitations to this method. If the concrete mixture in the field is changed, which is possible under the contractors’ discretion, the strength-maturity relationship is no longer valid, and the project must return to destructive testing for strength values. Maturity is also unable to evaluate the effect of curing on moisture retention or identify errors in placement consolidation or curing (Freeseman et al, 2016). Set temperature sensor locations are another limitation because the area chosen may not be representative of the entire pavement, or it may
miss discontinuities elsewhere in the slab. Despite the increased prerequisites and other potential limitations, maturity is a common choice to reliably monitor strength gain in concrete.
2.2 Ultrasonic Tomography
Ultrasonic tomography is an emerging technology that directly evaluates the condition of concrete using sound waves. While it is not as well-known as the maturity method, ultrasonic tomography has proven to estimated concrete strength more reliably and is used for many more applications. Ultrasonic tomography is unaffected by moisture or constraint conditions and therefore has a lower variability compared to other nondestructive tests (Cho et al., 2007; An et al., 2009).
Ultrasonic tomography involves a portable device that is used externally on the concrete surface. The ultrasonic tomography device uses dry point transducers that emit and receive shear waves as they travel through the pavement. This high frequency shear wave, greater than 20,000 Hz, travels through the concrete and then returns to the device where the shear wave velocity is recorded and a 3D reconstruction of the pavement directly below the scan is created (Hoegh 2011; Haza 2013). Each scan only takes a few seconds. Data is internally recorded automatically or can be manually recorded. The shear wave velocity can then be related to concrete properties, including elastic modulus, Poisson’s ratio, and density. (Cho et al., 2007).
This method has been used for a wide variety of testing. Quality control tests are common tests performed using shear waves. The 3D reconstruction allows the user to check pavement thickness, dowel bar location, or locate damage or delamination. This variety of uses is an appealing aspect in pavement construction. Hoegh et al. (2011) used linear-array ultrasonic tomography to determine slab thickness, reinforcement location, and internal distresses. Hoegh et al (2013) used ultrasonic tomography to classify joint damage and found this method to be more accurate that traditional methods. Vancura et al. (2013) used ultrasonic tomography to check pavement thickness in multiple locations over a project with accuracy. Choi et al. (2016) used ultrasonic shear-wave tomography to identify cracks and delamination in pavements. Salles et al. (2019) used ultrasonic tomography to identify incipient cracks in concrete slabs. Additional testing includes determining the extent of damage, boundaries of damaged concrete, and delamination between layers for improved maintenance (Hoegh et al., 2011; Choi 2016). This study will focus on the ability of ultrasonic tomography to measure material parameters, specifically, elastic modulus (Cho et al., 2007).
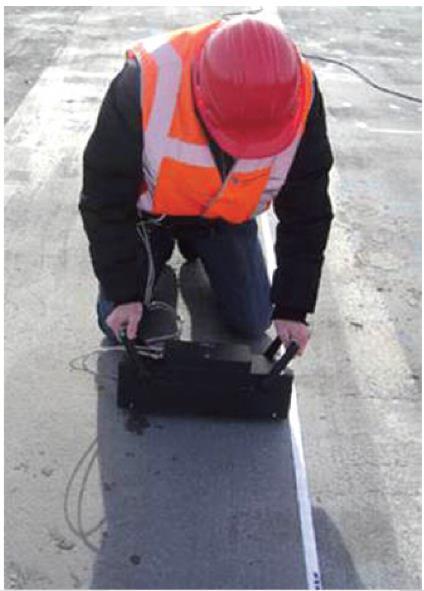
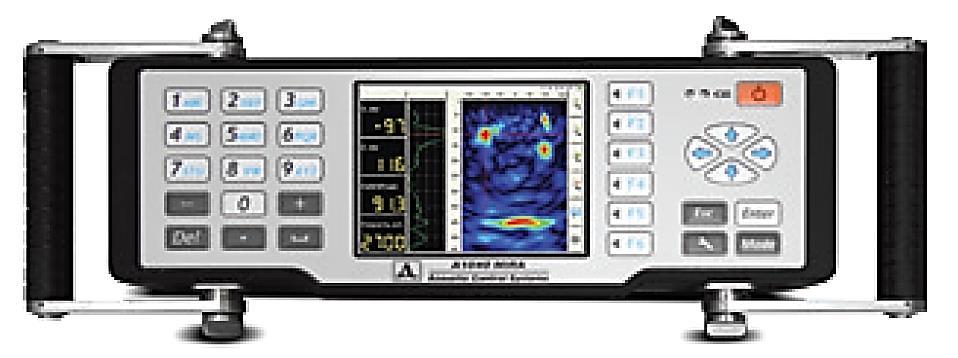
Figure 2: Ultrasonic tomography device (MIRA, 2021)
Shear wave velocity is closely related to the elastic modulus of concrete and can therefore be related to concrete strength (Cho et al., 2007). The aggregate source must be consistent through calibration and monitoring for accurate relations. To determine the shear wav velocity strength curve, there must be flexural testing alongside. In a laboratory, the beam specimens are measured on all four sides and then the average shear wave velocity is adopted as the representative shear wave velocity for that testing age. The relationship can then be developed between the average shear wave velocity and the average concrete strength.
The use of the shear wave velocity method for estimating the in-place concrete strength has the following advantages over the maturity methods:
It has been shown to have more accuracy than maturity for in-situ strength estimation. It does not require to pre-installed sensors into the pavement so testing can be conducted at multiple locations that do not have to be pre-defined.
However, the major drawback of this testing method is that it can only provide estimations at the time of scanning. There is no predictive relationship like that of maturity.
3 Estimating Concrete Strength: Field Study
The main objective of this chapter was to present and discuss the laboratory and field evaluation of a concrete paving project with focus on the estimation of concrete strength. For this, two non-destructive tests (maturity and ultrasonic tomography) were used and correlated with conventional laboratory strength testing. Tests were performed in laboratory and field using two different concrete mixes: high-early strength concrete (7-hr accelerated mix design) and long-life concrete (conventional mixture). The field study was divided in three main parts:
Description of the paving project, concrete mixes, and tests performed. Results and discussion for the High-Early Strength Concrete Pavement. Results and discussion for the Long-Life Concrete Pavement.
Strength criteria for opening to traffic lacks a federal guideline creating a wide variety of requirements at the state level. These criteria lack quantifiable justifications for the chosen values and are designed to be a conservative, generalized value that can be used across an entire state. While the simplicity is convenient, strength gain relies on many factors that can vary significantly between projects even in the same region. Including these factors in decision making can decrease the construction time by trimming unnecessary curing time and allowing traffic at the earliest ability.
To measure concrete strength in the field and, in the end, predict strength gain, two popular nondestructive methods will be considered in this study: maturity and ultrasonic tomography. Nondestructive testing is an alternative to destructive testing used to reliably estimate concrete strength. Maturity and ultrasonic tomography are two common methods that both correlate to strength well, although each have their own limitations. A maturity – shear wave velocity relationship that relates these two methods will be developed in this project allowing the benefits of both methods to be combined to optimize concrete strength measurement.
3.1 Description of Field Study Paving Project
The testing site (parking lot), shown in Figure 3, consists of multiple lanes of 12 × 12 ft dowelled Jointed Plain Concrete Pavement (JPCP). Construction began at 7:00 A.M. on July 13, 2020. Figure 4 shows photos from construction. The project was an 8-inch-thick concrete pavement using high-early strength (HES) mixture for the first lane and Long-Life Concrete Pavement (LLCP) mixture for the remaining six lanes. On the first day, four lanes were paved. Due to the shape of the first lane (bottom to top in Figure 3), the HES lane was constructed using fixed forms. A slipform paver was used for the three LLCP lanes. A sprayed compound was used on each lane as the curing method. Joints were sawed approximately six to seven hours after paving.
To perform maturity measurements, thermocouples were installed at two locations shown in Figure 3: one at the slab center (TC) and another near the lane edge (TE). In addition to maturity data, concrete shear wave velocity was measured at several locations on the slab surface as shown in Figure 3: slab edge (TE), center (TC), corner right north (CRN), corner right south (CRS), corner left north (CLN), and corner left south (CLS).
C C P l n a o i n t e n v C o e f g L i L o n ) m r f o p i S l ( C3
13:40 p.m.
C2
12:00 p.m.
C1.1 C1.2 C1.3
10:20 a.m.
Facility HES1 HES2 HES3
07:35 a.m.
High Early Strength PCC (HES) (Fixed-Form)
CLN C1.1
CRN
TC
CLS TE CRS
Thermocouples
C L S C L N
T E
C R S T C
HES1
C R N
Figure 3: Testing site design with construction and instrumentation details.
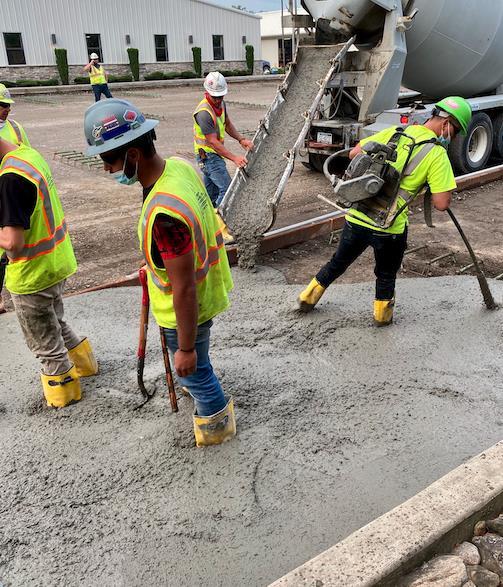
(a) HES
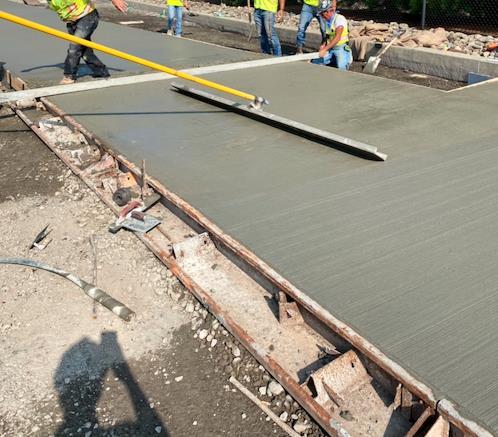
(c) HES
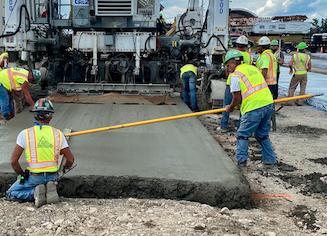
(e) LLCP (b) HES
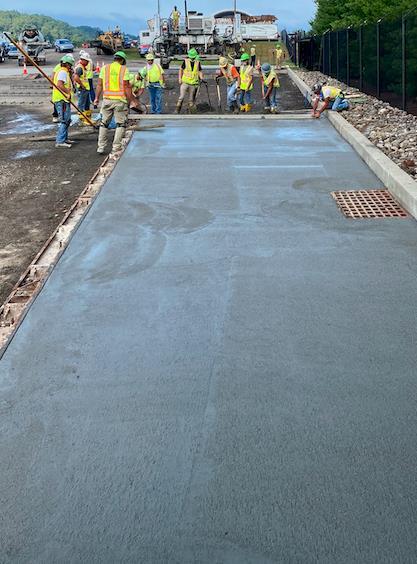
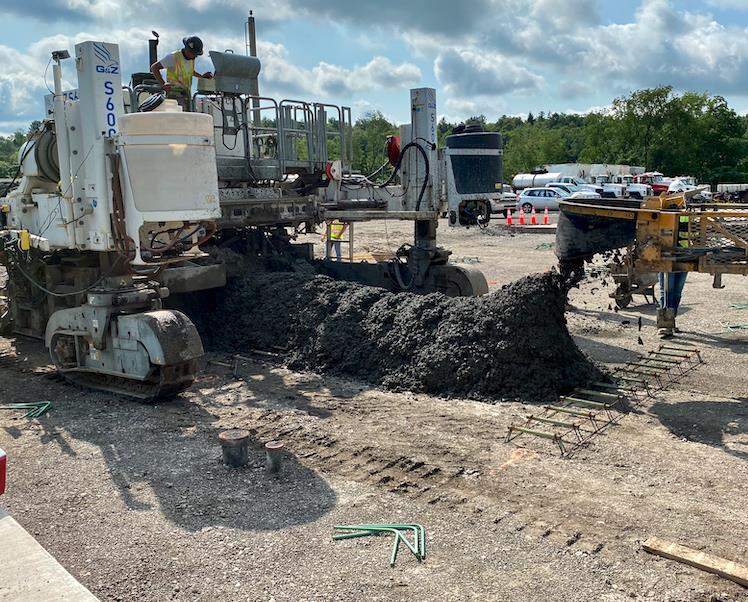
(d) LLCP
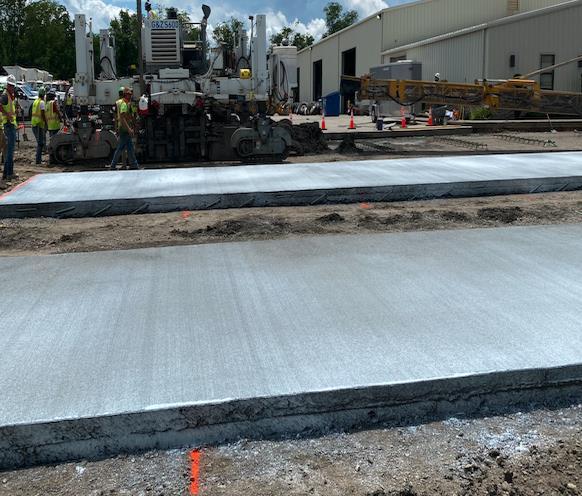
(f) LLCP
Figure 4: Construction of HES lane (a, b, and c) and of LLCP lanes (d, e, and f)