THE INNOVATION PIPELINE
The value chain of innovation in Australian universities
Quantum tech comes of age, p3
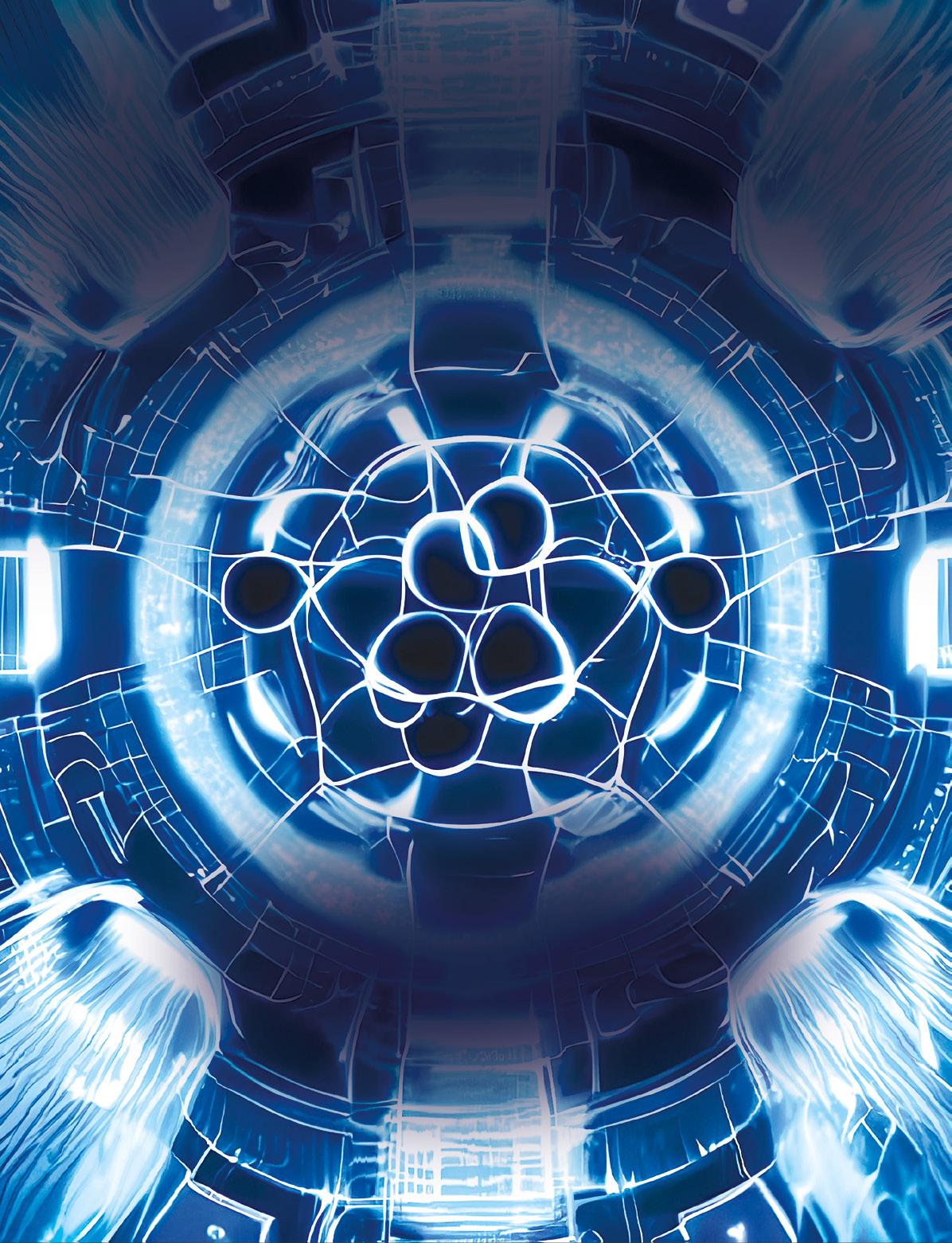
Runways to innovation, p6
Four areas of science set to boom, p8
The value chain of innovation in Australian universities
Quantum tech comes of age, p3
Runways to innovation, p6
Four areas of science set to boom, p8
University research is never just basic, or just commercialisable: it’s a complex value chain that drives innovation. I moved from science to business, and as such I’m deeply passionate about the research that delivers impact.
Growing up, I knew that the only thing I wanted to be was a scientist. I did a PhD in chemistry before moving into the commercial world as director and chair of multiple high-profile organisations with a basis in science. I was on the board of several Co-operative Research Centres (CRCs) as well as Sirtex, a listed ASX100 company making a liver cancer treatment, developed with the ongoing research contributions of the University of Western Australia. Sirtex was ultimately sold offshore for A$1.9 billion.
While this commercialisation story is one measurement of innovation involving university research, it’s by no means the only one. The university-science research value chain is complex, iterative, and has value at all stages. There are many steps along the ‘runway’ of innovation and all of us — the researchers, the end users, those of us in leadership, and those just starting out — play a role in its ultimate course.
Universities are fertile ground for innovative growth. At every point of the research value chain, the uncovering of new knowledge, driving of new processes, and a broad network of highly skilled people can create different outcomes. Today’s biodiversity research is tomorrow’s global virus response, and today’s fundamental space studies are tomorrow’s data-driven insights (which is how Wi-Fi originated in radiophysics research).
This is partly why the Australian
Academy of Science recently called for Australian Research Council (ARC) grants to restore and safeguard support for fundamental research. The networking, technical capacity, collaboration and ideation within university science supports new insights and drives whole new industries.
It is also important to note that many university-led centres and industry collaborations deliver pure public good research. The Bushfire and Natural Hazards CRC, (now Natural Hazards Research Australia), which I chaired, is a partnership of 29 university partners and multiple state, local, and national associations, governments, and agencies. It has saved countless lives through communication of bushfire risk.
Another organisation I had a long involvement with was the Antarctic Climate and Ecosystems (ACE) CRC, a multidisciplinary partnership of 23 national and international organisations of which the University of Tasmania was a valuable partner. The ACE CRC informed governments to drive policy change in protecting Antarctic waters and land, as well as developing a greater understanding of the role of Antarctica in the global climate engine.
The scope of university science outcomes is huge. It includes high-profile commercialisable research, such as green hydrogen or quantum information systems technology, “blue sky” research and its new directions:
Exploring the achievements of university science in building Australia’s sovereign capability
Australia’s strong science research and training is integral to driving new economies. Universities have a critical role as partners in establishing innovation and technological change in industry. As science delivers new insights and tools, new industries are emerging, and people with science skills will be essential to these new industries.
Australian University Science magazine highlights these stories, showcasing exceptional science teams and Australian science graduates working in industry. To provide feedback or suggestions, subscribe or order additional copies, visit acds.edu.au/AustUniScience
it also drives policy and understanding that is essential as we face a challenging future. This is the bedrock upon which we must build to deliver further impact down the line. Without these ‘runways’ of research, we won’t have the innovation to deliver future commercial and public good benefits for Australia and the world.
Dr Katherine Woodthorpe, President Australian Academy of Technological Sciences and EngineeringCover Image: Graphic illustration of quantum computing created by OpenAI. Image: Open AI/Heather Catchpole. Published 5 April 2023 by Refraction Media on behalf of the Australian Council of Deans of Science. Designed by Jon Wolfgang Miller. Printed in Australia by IVE. ISSN: 2652-2403.
© 2023 Australian Council of Deans of Science, all rights reserved. No part of this publication may be reproduced in any manner or form without written permission. If you would like to reproduce anything from this issue, email info@refractionmedia.com.au.
University science research falls into two categories when it comes to funding. On one side: fundamental research, generally supported directly by universities or by Australian Research Council (ARC) grants. On the other side is funding directed at commercial outcomes. For this, researchers often look to industry partners, and apply for National Health and Medical Research Council grants, ARC Linkage projects, Co-operative Research Centre programs and others. This duality, however, oversimplifies the complex, iterative process of university science. Researchers work across every part of the spectrum, from seeking fundamental knowledge to developing participatory outcomes that include benefits to end users, influence on policy, commercial partnerships and outcomes.
“At WEHI (Walter and Eliza Hall Institute), our successes, particularly in cancer and malaria drugs, have involved over 20 years of basic science,” said Dr Anna Coussens, laboratory head of Infectious Diseases and Immune Defence.
“Understanding the fundamental processes of cells and their interaction with pathogens allows us to identify potential targets for drug development. Everyone is working on fundamental questions, and together we can come up with ideas that create real clinical outcomes.”
Environmental scientist Professor Peter Macreadie, head of Deakin University’s Blue Carbon Lab, says their work is rooted in both fundamental research and practical outcomes. His lab collaborates with end users, including community and industry, to ensure “realworld impact for the communities we serve, from governments and industry to everyday people — and the planet as well.
“We’re on site with these companies and on field, we’re making sure our Indigenous partners are on board as well,” he said. “Without universities we wouldn’t have the data to back up the concept of the wealth of natural capital.”
Collaboration is an integral part of university science, resulting in exchanges of ideas that lead to innovation and progress. For example, innovations in tuberculosis (TB) diagnosis and management helped countries with robust TB public health systems to respond more quickly and appropriately to COVID-19, said Coussens.
This research continuum in science can be compared to a value chain in business: a progression of activities that allows us to build and develop solutions — even when we don’t know what they will turn out to be. Unlike in business, however, university
science has an intrinsic value at the start: changing the very way we see the world.
It’s impossible to know what direction fundamental research will go: and questioning its value is like asking what use a newborn baby is. It might grow up to be a nurse who looks after you. But their worth goes far beyond that.
Today, science is delivering innovation from previous decades faster than ever before. Quantum technologies took 100 years to mature, molecular biology 70 years, AI just 50. Expedited development of COVID-19 vaccines is expected to benefit the development of other vaccines as well (see p6–7 for examples).
To better reflect how researchers work, collaborative spaces like universities need funding at all stages of research, from the first seed of an idea to its outcome. The massive philanthropic investment of funding during COVID-19 is a case in point: a huge network of people came together to create a strong ‘pull’ on the research sector. The science research ecosystem was able to quickly respond, leveraging existing networks, broad knowledge and the innovative applications of this knowledge. “Philanthropy created a quick turnaround on research funding. It shows what you can do quickly if you try,” said Coussens.
To foster industry growth, it’s crucial to understand the intrinsic value of university science, how good our value chains are and which are our important ones — including their limitations and what the ultimate applications might be.
There are 19 quantum-related companies in Australia that have received funding and investment of over $400 million in recent years. Speaking at the CSIRO Quantum Commercialisation Forum recently, Minister for Industry and Science Ed Husic said quantum is a good example of how Australia can be world leaders, and not just focus on “small parts of the value chain”.
“Investment in innovation, science and research lays the foundation for groundbreaking technologies and improved products and processes,” he said.
The case for this surely begins with university science. — Heather Catchpole
For innovation, overnight success is often decades in the making: a keystone of which almost always comes from basic science at universities.
Just about every technology that becomes a global success, triggering disruptive changes in industry and society, began in a university and took years to ripen. Often, the bigger the success, the longer it may have gestated.
Take the internet: first established between universities in the late 1960s, but not widely adopted by industry and consumers until the 1990s. Artificial intelligence is today a seemingly unstoppable juggernaut burrowing into every nook and cranny of modern life, and it began with basic research at universities in the 1950s.
“Most of the big Australian science
blockbusters — like the HPV vaccine or the Cochlear implant — are firmly rooted in university research,” said Tony Peacock, former long-time CEO of the Cooperative Research Centres Association. An Adjunct Professor at the University of Canberra, he now chairs the boards of two biomedical companies.
“And the big CSIRO winners, like Wi-Fi and the polymer contact lens, relied on deep collaboration with Macquarie University and UNSW.”
It’s not just in Australia. “History is filled with examples of fundamental research undertaken in universities, driven by the curiosity of researchers, finding significant applications that could never have been imagined,” said Prof Kate Smith-Miles,
a professor of mathematics and statistics, and Associate Dean (Enterprise & Innovation) for the Faculty of Science at the University of Melbourne. It took mathematical breakthroughs to make the internet and MRI scans possible, and “fundamental understanding of DNA led to modern techniques of DNA sequencing and genetic engineering.”
Successful innovation requires disparate players to become fellow travellers and find ways to connect and collaborate to create a value chain, said Prof Caroline McMillen, the Chief Scientist for South Australia, and former Vice Chancellor of the University of Newcastle.
She cited the example of ‘rust belt’ cities — such as Albany, New York, or Eindhoven in The Netherlands — where a combination of visionary thinkers, local universities, government initiatives and start-ups transformed old industries into smart new products by integrating information technology, sensors, big data, new materials, and automation.
“These communities went through remarkable economic and social transitions when traditional manufacturing moved offshore,” McMillen said. “That seismic shift also created a burning need for new ideas, and they focused on universities in ways they hadn’t before. New industries arose, based on some existing skills or the manufacturing base. But that needed active partnerships, especially between research and businesses.”
Take Akron, Ohio, once the tyre-making capital of the world — home of Goodyear, Firestone and others; “Polymers were their core expertise,” she said. “What else could they do with plastics and rubber?” From the depths of the 1990s doldrums, Akron is now one of the world’s leading polymer centres with more than 400 companies manufacturing polymer-based materials for everything from lipstick to medical devices.
Successes like these have been slower in Australia — partly because businesses are
hesitant and unfamiliar with universities, and partly because universities were in the past less entrepreneurial and more interested in advancing their research agenda. That’s changing.
“Things like intellectual property management at universities and contracting out are still slower here than overseas,” said Peacock. “But researchers are getting more skilled at working with business. Not seeing companies as simply a funding source for a project is a big change in mindset. And more companies are learning their way around universities.”
While universities are an important link in the value chain of innovation, that’s not how many Australian companies see them. “But I think there’s growing recognition of the value universities can offer. Very often, things might start with a simple job they need help with, and then a relationship develops,” he added.
“There’s no one way to do collaboration,” said McMillen. “But it all starts with a conversation.” — Wilson da
SilvaToday’s technologies are now emerging from decades of university science research.
Ultrafast data processing: The Square Kilometre Array radio astronomy project, borne out of fundamental physics studies of the universe, will generate colossal data streams that will drive major advances in fast data processing, storing, and analysis — generating benefits for all. Highspeed systems that can process mass amounts of data in real-time are already finding commercial applications, such as live analysis of stock market data to make rapid investment decisions; or in medicine to quickly process vast amounts of patient data to improve diagnosis and treatment.
mRNA: Messenger RNA technology prompts a body’s own cells to produce proteins for therapeutic purposes. First developed in the 1990s, it became a global lifesaver during COVID-19. Its ability to rapidly create mass quantities of tailored proteins bypasses the traditional time-consuming and complex process of creating drugs and promises to make whole new classes of previously inconceivable treatments possible.
Quantum sensing: This booming sector utilises the weird effects of quantum mechanics to detect and measure physical phenomena with startling precision and sensitivity. Experts anticipate dramatic improvements in imaging, navigation, and a more detailed understanding of how biological systems function (see p7).
Universities are the hubs of transformative innovation, where every year, countless new ideas take flight. The last 50 years have seen an explosion of technologies borne out of the complex interchange between basic university research and commercial partnerships that have gone on to transform the world. Here are two new totemic technologies that will disrupt the future, and how they came to be.
1953
DOUBLE HELIX STRUCTURE OF DNA DISCOVERED
University of Cambridge
James Watson, Francis Crick, Rosalind Franklin, Maurice Wilkins
Mixing biology, engineering, and computer science, synthetic biology is the design and assembly of artificial biological systems or the re-engineering of existing ones. It is accelerating biotechnology in industrial and medical fields and creating new sustainable methods of manufacturing and energy production. Applications include biofuels, novel vaccines and therapies, engineering agriculture to improve yields, and resistance to pests and climate change.
The global synthetic biology market was worth an estimated $14.15 billion in 2021, and growing rapidly: it is expected to reach $45.7 billion by 2026. Australia’s Synthetic Biology Roadmap estimates that, by 2040, synthetic biology could generate $27 billion in annual revenue for Australia and create 44,000 new jobs. Notable Australian companies are Samsara Eco, an Australian start-up that uses enzyme-based technology to break down plastic into its core molecules; and Starpharma, which uses nanoscale polymers for drug targeting. Leading universities are the University of Melbourne, Monash University, the University of Sydney, Macquarie University, the University of Queensland, and UNSW.
1977
FIRST COMPLETE GENOME SEQUENCED University of Cambridge Frederick Sanger
1972
RECOMBINANT DNA TECHNOLOGY DEVELOPED
Stanford University; University of California San Francisco
Stanley Cohen, Herbert Boyer (Boyer co-founds Genentech Inc in 1976; first gene patent issued 1980)
1972
SYNTHESIS OF THE FIRST COMPLETE ARTIFICIAL GENE University of Wisconsin Madison Har Gobind Khorana
2010
CREATION OF THE FIRST SYNTHETIC CELL
J. Craig Venter Institute; U.S. National Institute of Standards and Technology; Massachusetts Institute of Technology
Elizabeth Strychalski, John Glass, Lijie Sun, James Pelletier, Andreas Mershin, Neil Gershenfeld, Kim Wise, Nacyra Assad-Garcia, Bogumil Karas, Thomas Deerinck, Mark Ellisman, Ray-YuanChuang
2022
FIRST FULLY SYNTHETIC YEAST GENOME FOR WINE Macquarie University; University of Adelaide; Australian Wine Research Institute; NSW
Department of Primary Industries
Thomas Williams, Hugh Goold, Ian Paulsen, Isak S. Pretorius, Anthony Borneman, Dariusz Kutyna, Cristobal Onetto, Daniel Johnson
1980
PROPOSES USING SUPERPOSITION AND ENTANGLEMENT IN COMPUTATION
Moscow State University, Russia Yuri Manin
1980
DEVELOPS A THEORETICAL MATHEMATICAL MODEL FOR QUANTUM COMPUTATION
Argonne National Laboratory, USA
Paul Benioff
Creating computers that operate on the principles of quantum mechanics — the physics of how matter and energy behave at subatomic level — has long been recognised as potentially powerful, if impossibly complex. Unlike today’s ‘classical’ computers, which process information in binary bits (0s and 1s), quantum computers rely on ‘quantum bits’, or qubits, which can exist in multiple states at once. Known as superposition, this allows a multitude of computation strategies — some exponentially faster, some simultaneous — that are far beyond modern computers. Another key property, entanglement, allows quantum computers to process data in parallel. Although a nascent technology that is delicate and unstable, quantum computers are already used to simulate chemical and molecular interactions to help discover new drugs or novel materials. As they scale up over the next decade, quantum computers will revolutionise cryptography, financial modelling, and chemical engineering, solve complex optimisation problems such as scheduling and routing, and accelerate types of machine learning algorithms.
The global quantum computing market is valued at an estimated $15.3 billion, and growing fast, due to enormous expenditure by governments and commercial companies. The market for quantum computing is predicted to reach $186 billion by 2030. It’s predicted quantum computing will generate $2.2 billion in Australian revenue by 2030 and nearly $6 billion by 2045, creating 8700 new jobs by 2030 and 19,400 by 2045. Notable Australian companies are PsiQuantum, which is developing a quantum computer based on silicon photonics; Silicon Quantum Computing, focusing on single-atom qubits for information processing; and Diraq, which relies on spin qubits and existing technology used by today’s classical computers. Leading universities are UNSW, the University of Sydney, the University of Melbourne, Monash University, and the University of Queensland.
1982
DETAILS HOW QUANTUM MECHANICS COULD BE USED TO PERFORM CALCULATIONS IMPRACTICAL OR IMPOSSIBLE FOR CLASSICAL COMPUTERS
California Institute of Technology, USA
1994
DEVISES A QUANTUM ALGORITHM CAPABLE OF FACTORING LARGE NUMBERS QUICKLY, BREAKING SECURE CRYPTOGRAPHIC SYSTEMS
Massachusetts Institute of Technology, USA
Richard Feynman
1998
CONCEPT AND DEVELOPMENT OF A SCALABLE QUANTUM COMPUTER IN SILICON
University of New South Wales
Bruce Kane, Michelle Simmons
2001
FIRST USE OF SHOR’S FACTORING ALGORITHM IN A QUANTUM SYSTEM
Stanford University; IBM Almaden Research Centre, USA
Isaac Chuang, Lieven Vandersypen, Matthias Steffen, Gregory Breyta, Costantino Yannoni, Mark Sherwood
2012
FIRST QUANTUM ALGORITHMS RUN ON SILICON QUANTUM COMPUTER
University of New South Wales
Jarryd Pla, Andrew Dzurak, Andrea Morello, Kuan Tan, Juan Dehollain, Wee Lim, John Morton, Floris Zwanenburg, David Jamieson,
Peter ShorFour areas where fundamental research is set to bring benefits to society and the economy.
Oceans of ecosystem data from decades of environmental science at Australian universities provide a wealth of information on the value — natural beauty aside — of the Great Barrier Reef. Researchers led by Deakin University’s Blue Carbon Lab (including director Prof Peter Macreadie, left) have identified that — through conserving and restoring coastal and marine ecosystems — the reef has enormous capacity to store carbon dioxide. Seagrass meadows and mangrove forests within the reef’s coastal catchment areas already sequester an astonishing 111 million tonnes of carbon. Damage to catchments could result in greater carbon emissions, boosting the argument for reef protection. Restoring 90,000 hectares of land throughout the reef’s catchments could enable an extra five million tonnes of carbon to be captured by 2100. In fact, coastal wetlands can capture and store carbon in the ground 10 times faster than nearby rainforests.
Western plant science traces its roots to Ancient Greece, and has long provided inspiration across different disciplines. Drawing on a network of expertise across plant science, energy research and engineering, RMIT University researchers are looking at the leaf structure of the western sword fern as inspiration for the development of a graphene-based capture and storage of solar energy. Their research revealed that sword fern leaves, packed with veins, are extremely efficient at storing energy and moving water within the plant. This led to the creation of a prototype electrode based on this leaf design. By combining it with supercapacitors, the researchers were able to dramatically improve the solar energy storage capacity. Elements of the prototype are based on flexible thin-film technology, potentially allowing a solar storage solution that is less bulky and rigid than current solar cells, and which could have many uses: including car panels, smartphones, and watches.
Our insatiable appetite for electronic devices has led to a rapid increase in energy use, with information technology now responsible for 8%
of the world’s electricity consumption. Experts predict the technology currently powering these devices simply can’t keep up. The materials of the future that will cater for rapid advances in technology are ideated in the hallways of university science — but it takes a village. FLEET, the ARC Centre of Excellence in Future LowEnergy Electronics Technologies, is a collaboration of Monash, ANU, RMIT, UNSW, the University of Queensland, Swinburne, and the University of Wollongong, with 18 other Australian and international research institutions. Combining the expertise of 200 researchers, FLEET aims to create a new generation of ultra-low energy electronics based on novel materials, that would allow devices to ‘switch’ at much lower voltages, resulting in far less energy use. The physicists, materials scientists and engineers of FLEET
are reinventing solid state physics to develop new materials that harness the strange effects of quantum mechanics to attain the crowning ambition of room temperature, zero-resistance electrical pathways.
Future outposts on the Moon or Mars will be unable to bring all they need from Earth, so they will need to grow their own food, and create medicines and materials. That’s the mission of the ARC Centre of Excellence in Plants for Space, based at the University of Adelaide and involving Flinders, the University of Melbourne, UWA and La Trobe, as well as eight companies and 20 research institutions in Australia and overseas, including NASA. Known as P4S, the centre plans to cultivate new varieties of plants that can grow in controlled environments. This expertise relies on years of Australian science advances in agriculture in some of the most challenging climates on Earth. Breakthroughs from the new centre will be immediately applicable on Earth, since we also face sustainability challenges in food and biomaterial production — such as lack of water, the need to recycle nutrients and avoid waste — and will fast-track current research on controlled environment agriculture and vertical farming.