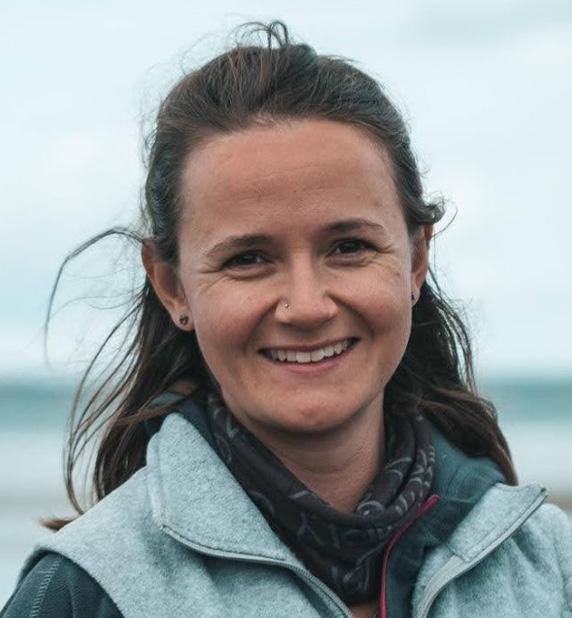
8 minute read
Refurbishing the Besse Building
Ancient magnetic fields and life on Earth
Dr Claire Nichols joined the Department of Earth Sciences in Oxford in the summer of 2020 and became a Tutorial Fellow at Teddy Hall at the end of 2021.
After initially aspiring to be a theoretical physicist, Claire quickly discovered the practical side of Earth Sciences was a much better fit and particularly enjoyed field trips and the opportunities to travel to remote places. After completing an MSci in Earth Sciences, Claire remained at Cambridge for a PhD studying ‘tiny space magnets’, a type of nanoscale iron-nickel microstructure in meteorites which allows records of early solar system magnetic fields to be recovered.
All of us have pondered whether there are little green men living elsewhere in the Universe, and how and why life first emerged on Earth. These are fundamental questions to which we may never have concrete answers. However, we are beginning to have an idea of the ‘must have’ qualities for a habitable planet. Liquid water and the correct assortment of chemical elements are certainly key, but what controls whether a planet will host such conditions? And for how long can these favourable conditions be sustained? My research focusses on one very specific question: whether a magnetic field is an essential criterion on the habitability checklist.
First, we need to consider how planets generate magnetic fields in the first place, how common they are, and how long-lived. When planets first form, dense metallic iron sinks to the planetary centre to form a core. While these cores are at least partially molten, the liquid part can be vigorously stirred. Since metallic iron is electrically conductive, this stirring is a little like applying a current through a coil of wire; it will generate a magnetic field. Earth’s core has generated a magnetic field, known as the geodynamo, for at least 3.5 billion years. Mars’s core, on the other hand, stopped generating a magnetic field more than 4 billion years ago. This has led scientists to ponder whether the cessation of the Martian magnetic field led the planet to evolve to its present cold, arid and hostile state while Earth remained warm, wet and habitable.
A perhaps even larger conundrum is the Moon’s magnetic field. The Moon has a tiny metallic core, which is just one seventh of its radius (Earth’s core is half its radius), suggesting it would only ever have generated a weak, short-lived magnetic field.
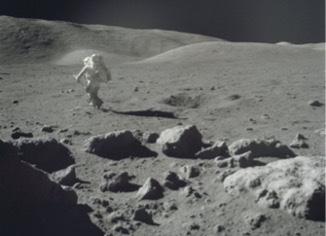
Astronaut Harrison ‘Jack’ Schmitt collecting samples from the Moon during the Apollo 17 mission (Credit NASA). Collecting samples in Isua, Greenland to investigate Earth’s magentic field 3.7 billion years ago.
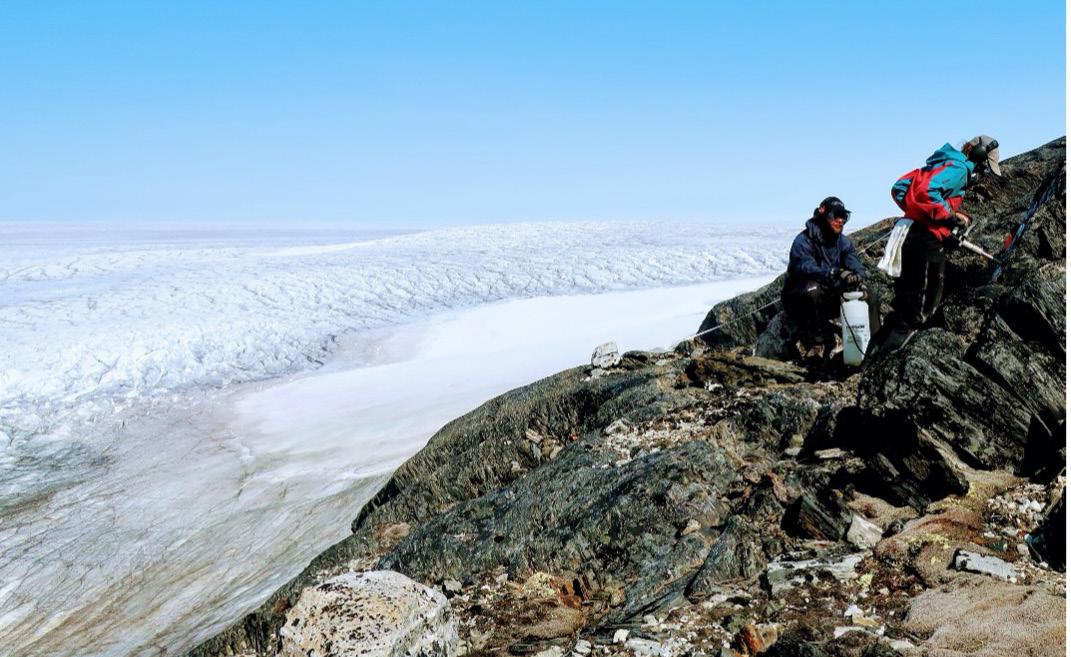
However, laboratory studies on returned samples from the Apollo missions have revealed the Moon had an intense magnetic field for at least 2 billion years. In my recent research with collaborators at MIT and Apollo 17 astronaut Harrison ‘Jack’ Schmitt, we have investigated the shape of the ancient lunar magnetic field to see if this provides clues to how the ancient field was so strong. We found that the magnetic field around the Moon looked much like that around Earth today, with North and South magnetic poles aligned along the spin axis. This was unexpected – we know that the lunar magnetic field couldn’t be generated by the same processes driving Earth’s magnetic field today – this highlights the huge body of work ahead of us to understand even the basics of how planetary magnetic fields are generated and sustained. Similarly, there is debate as to how Earth’s early magnetic field was generated – particularly at the time when liquid water and life first emerged on the planet almost 4 billion years ago. Today, Earth has a solid inner core. As the inner core grows, it releases light elements such as sulfur into the overlying liquid outer core. These light elements are buoyant and rise up to the top of the core, driving vigorous compositional convection. However, prior to inner core solidification (the timing of which is still debated) it is unclear how convection was sustained. For the last few years I have been working in Isua, a rocky region of southwest Greenland nestled up against the ice sheet. These rocks are some of the oldest anywhere in the world, with a staggering age of 3.7 billion years. We have been trying to untangle their complex geological history to see if these rocks may hold the oldest record of Earth’s magnetic field to-date. Using the approaches developed in this research, we hope to be able to ‘fill in the gaps’ in Earth’s magnetic field history to try and spot trends which may be related to events such as the solidification of the inner core.
As we understand more about ancient planetary magnetic fields we can assess their influence on the evolution of a planetary surface. For example, models of atmospheric escape for charged particles, or ‘ions’, are highly sensitive to magnetic field strength and shape. Surprisingly, results suggest that a planetary magnetic field may enhance atmospheric escape rather than shield from it. Earth’s surface became oxidised around 2.5 billion years ago during the ‘Great Oxidation Event’, which may have been triggered by the loss of hydrogen along polar magnetic field lines. Our next target is to look for changes in Earth’s magnetic field strength leading up to this event, to truly determine whether Earth’s magnetic field protects or harms our planetary surface, and therefore its role in building a habitable planet.
An atmospheric journey in the Cradle of Humankind
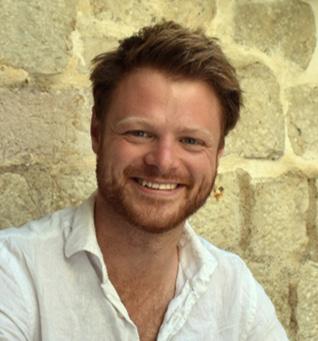
Dr Callum Munday completed his DPhil (2014-2019) at St Edmund Hall and was a college lecturer at Keble College and Teddy Hall from 2017-2021, before joining the Hall as a Fellow by Special Election in Geography.
Callum is a climate scientist specialising in African climate and climate change. He is currently working on a NERC- funded project in southern Africa and is a visiting scientist at the UK Met Office.
Our earliest ancestors roamed around Africa 6 million years ago. Much of what we know of these early humans comes from a remote part of northwest Kenya called the Turkana Basin. There, scientists, including the late great Richard Leakey, have uncovered a sequence of fossils, which tell the story of our evolutionary history.
The emerging agreement is that the idiosyncrasies of African climate shaped our evolutionary trajectory. Scientists studying changing climate through ancient lake deposits, the diet of long extinct mammals and layers of mud on the floor of the oceans, describe important changes in environmental conditions coinciding with our divergence from apes. However, this climate record is incomplete: depending where you look, you can find different versions of how the climate of the deep past shimmied and shifted. So how can we set the story straight? One place to look for evidence is in the present day. The Turkana Basin is one of the driest places on the planet, and is home to the world’s lowest latitude desert. If we can find out what controls the climate there today, then we have a better chance of understanding the past.
Unfortunately, the cradle of humankind is one of the most data sparse regions of the world. We have more weather stations in Tyneside compared to Turkana. The biggest data void is in the layer of the atmosphere above the surface. The temperature, humidity and winds in this layer control the formation of clouds and rain but, up until recently, we had no data to guide us on what they might be.
In 2021, scientists from University of Oxford, University of Nairobi and the Kenya Meteorological Department
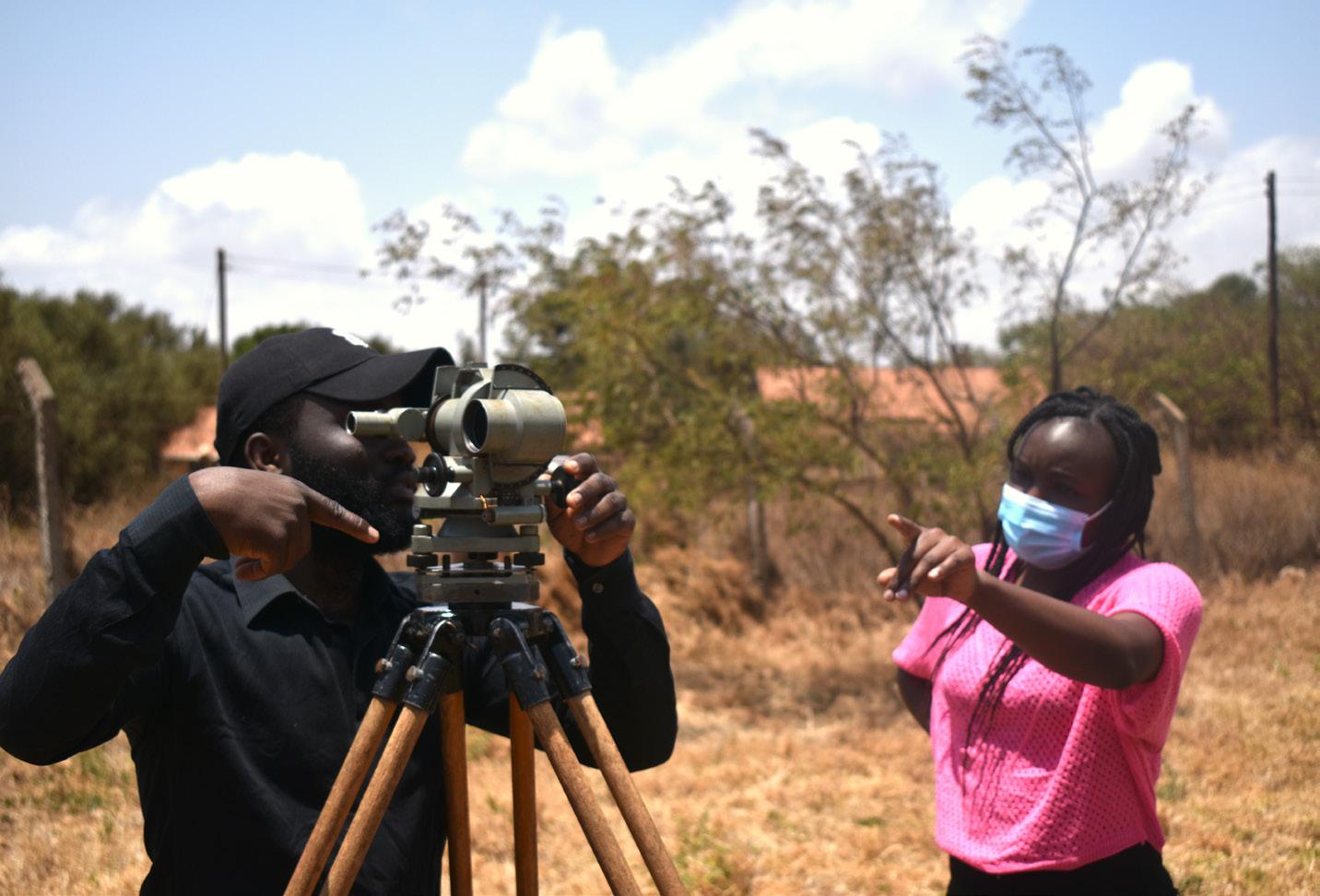
Masters students from University of Nairobi (Rose and Clinton) get to grips with tracking balloons. The whole team from Uni of Oxford, Uni of Nairobi and the Kenya Met Department gather for a final balloon.
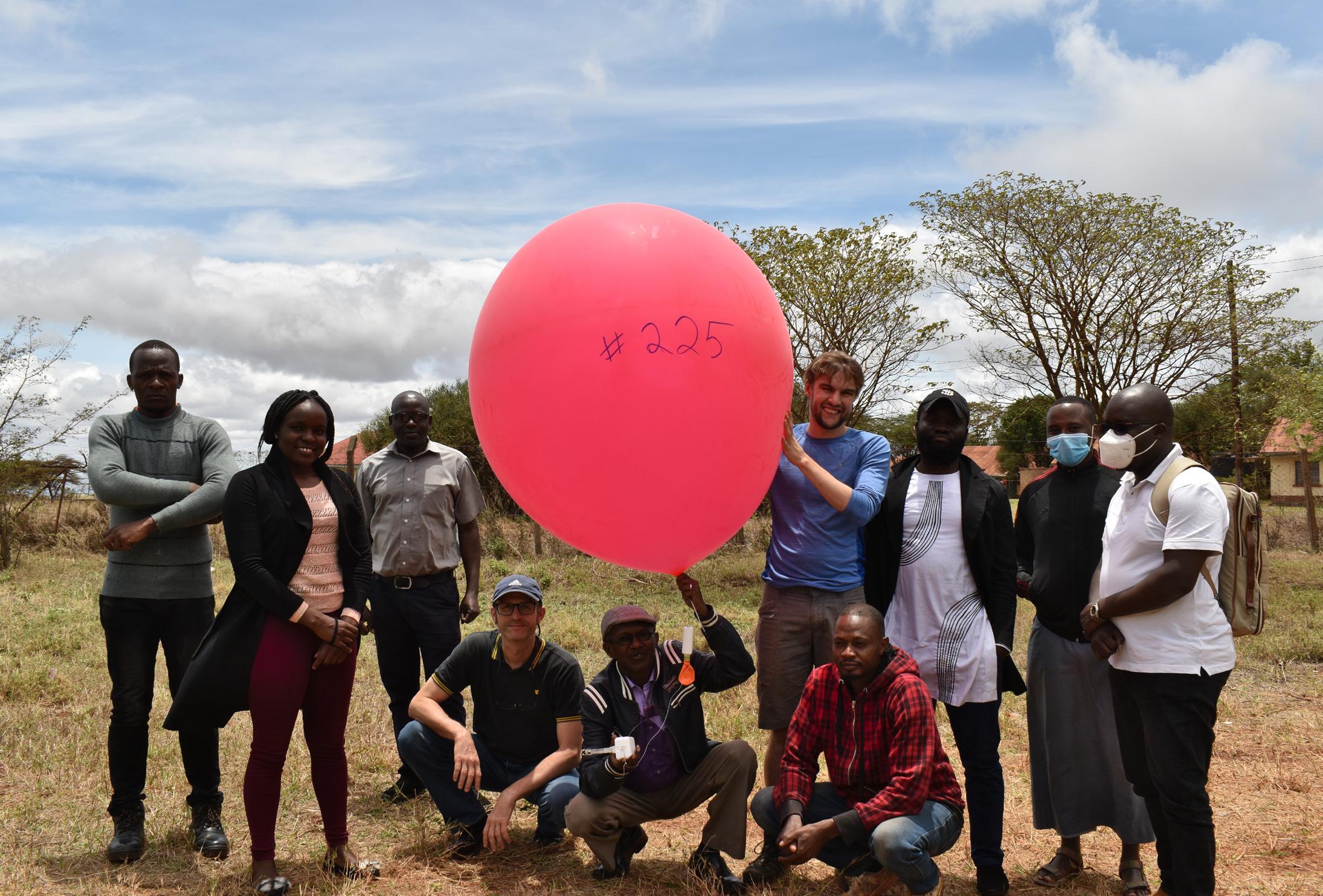
travelled to northwest Kenya to embark on a programme of scientific observations. Over a month-long expedition, the team released over 200 weather balloons to tune into the rhythm of the atmosphere through the day and night.
The team found remarkable climate processes at play. A few hundred metres above our heads, a massive stream of water vapour snakes its way from the Indian Ocean through the Chalbi Desert. The amount of water shifted is equivalent to two times the discharge of the Amazon River. But this water vapour does not fall as rain. Instead, the skyborne river passes silently through the desert, like a train past a defunct station, providing the moisture for its final destination in the Congo rainforest.
Why this happens is a puzzle the team are still figuring out. The rugged geography of the Great Rift Valley is one possible cause: steep mountains may act as a funnel, ensuring no water spills as rain on its journey inland through Turkana. If this is so, then details of the moving mountains over millions of years could hold the key to understanding the region’s climate and, possibly, our history.
What we do know, is that the climate in the place where it all started for humans is truly unique. With more observations, we can build a better picture of how the climate works now, to inform how it worked then and to find out how it will change in the future. “Why this happens is a puzzle the team are still figuring out.”