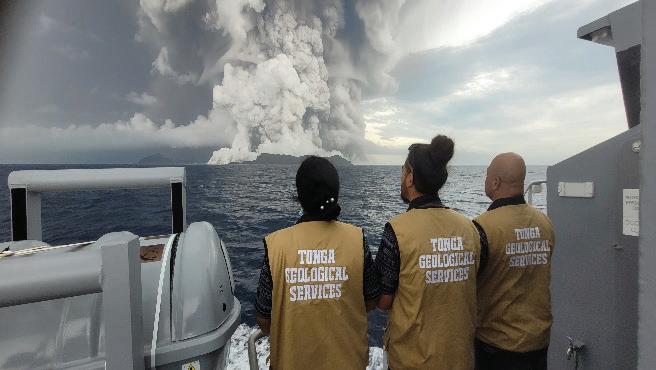
17 minute read
WHAT CAN WE LEARN ABOUT VOLCANIC HAZARDS FOR SUBSEA CABLES FROM THE 2022 HUNGA TONGA-HUNGA HA’APAI ERUPTION?
By
Michael
Clare,
Isobel Yeo, James Hunt (National Oceanography Centre, UK), Sally Watson Richard Wysoczanski, Sarah Seabrook, Michael Williams, Emily Lane, Kevin Mackay (National Institute of Water and Atmospheric Research, New Zealand), Peter Talling, Edward Pope (Durham University, UK), Shane Cronin (University of Auckland, New Zealand), Marta Ribó (Auckland University of Technology, New Zealand), Taaniela Kula (Tonga Geological Services, Kingdom of Tonga), David Tappin (British Geological Survey, UK), Samiuela Fonua, James Panuve (Tonga Cable Ltd), Dean Veverka (Southern Cross), Ronald Rapp (SubCom), Valey Kamalov (Google)
Abstract
The 15th January 2022 Hunga Tonga-Hunga Ha’apai volcanic eruption was the most explosive on Earth in more than a century. Here, we present findings from surveys performed only months after the large eruption to show how powerful pyroclastic flows plunged into the ocean, scouring deep gullies, and causing extensive damage to international and domestic cables, cutting communication links to the Kingdom of Tonga. These powerful flows travelled at high speeds (up to 122 km/hour) over >100 km. We share lessons learned from this event and discuss how to better understand the hazards posed by other volcanoes to subsea cables.
Introduction
Subsea cables provide critical communications links, carrying >99% of all intercontinental digital data traffic worldwide[1]. While geological hazards only account for <15% of reported subsea cable faults globally[2], such events can be particularly significant as they can affect very large areas of seafloor, damaging multiple cable systems synchronously[3]. Remote island nations are particularly reliant on submarine cables; however, they are often located in regions that are prone to natural hazards, such as earthquakes, tropical storms, underwater landslides and volcanic activity[1]. Diversity in cable routes and landing station locations ensures that most regions are sufficiently resilient to the impacts of both human and natural hazard-related cable damage[4]; however, islands that have few connections will be more vulnerable[5]. In this paper, we discuss the case of the January 2022 Hunga Tonga-Hunga Ha’apai volcanic eruption that led to extensive damage to the Kingdom of Tonga’s only subsea international telecommunications cable, severed it from the global network at a critical time for disaster response.
Aim And Objectives
Our overarching aim is to share results of recent surveys that reveal the seafloor impacts of the largest volcanic eruption in the last century and provide new insights for cable routing and resilience. We address the following questions.
1) What explains the unusually exten- sive damage on the international and domestic cables that serve the islands of Tonga? 2) What can we learn about the series of events that followed the major eruption on 15th January 2022 and hazards for cables along the Tonga-Tofua Arc in the South Pacific? 3) What are the broader implications for assessing threats to cables in other volcanically-active regions?
THE HUNGA TONGA - HUNGA HA’APAI ERUPTION
The most recent eruption of Hunga Tonga – Hunga Ha’apai began on 20th December 2021. The initial phases of the eruption were low explosivity, producing predominantly steam plumes with minor ash components, reaching heights of ~16 km. Relatively low explosivity activity, predominantly from the explosive interaction of hot volcanic material and seawater, continued from the start of the eruption to 5th January, producing small pumice rafts (floating volcanic rocks), ash and steam plumes[6]. During this phase, the small subaerial island that connected the islands of Hunga Tonga and Hunga Ha’apai almost doubled in area from ~3 km2 to 5 km2, and the vent, which was the same as that active during a 2014/15 eruption, remained subaerial[7]. Following a short hiatus, activity recommenced at the same vent on 14th January 2022[8]. The subaerial eruption generated a plume that reached a height of around 20 km (Fig. 1). Low explosivity volcanic activity was observed into the early hours of the 15th when satellite imagery showed that the island (that grew during previous phases of the eruption) had been removed. At 17:15 local time (04:15 UTC) on 15th January, the volcano entered a different eruptive phase. Two large explosions were detected from a submarine vent slightly to the east of the previous vent, producing an ash plume that reached 57 km in height and generating tsunamis that were detected around the world, with run up heights of 18-20 m on several Tongan islands[7-12]. Pyroclastic density currents formed during the eruption and plunged into the ocean, contributing to the generation of tsunami waves[13].
Two subsea cables connect to Tongatapu (the main island of Tonga), which include an international cable that runs towards the west and a domestic cable (Fig 2). These cables were both broken on 15th January, but not synchronous with the main eruption. Instead there was a lag of 14 and 88 minutes after the eruption for the domestic and international cable breaks respectively. Repair of the international cable took five weeks due to the exceptional extent and remoteness of the damage. The closest repair ship was in Papua New Guinea (2500 km away) and significant additional lengths of cable were needed. The delay of the cable faults after the eruption prompted initial speculation as to whether they were caused by seismicity associated with the eruption, local failures from the steep slopes adjacent to the cable routes, or density flows created by the eruption itself. If the latter, then this would be an unprecedented observation of such powerful and long runout flows. Unusually for this region, high-resolution bathymetric surveys had been performed around Hunga Tonga-Hunga Ha’apai prior to the eruption[14,15]. To answer the question of what caused the cable damage and inform the routing of repaired cables, post-eruption seafloor surveys were conducted, providing a unique insight into this globally-significant event.
Rapid Post Eruption Surveys Provide Evidence Of Powerful Seafloor Flows
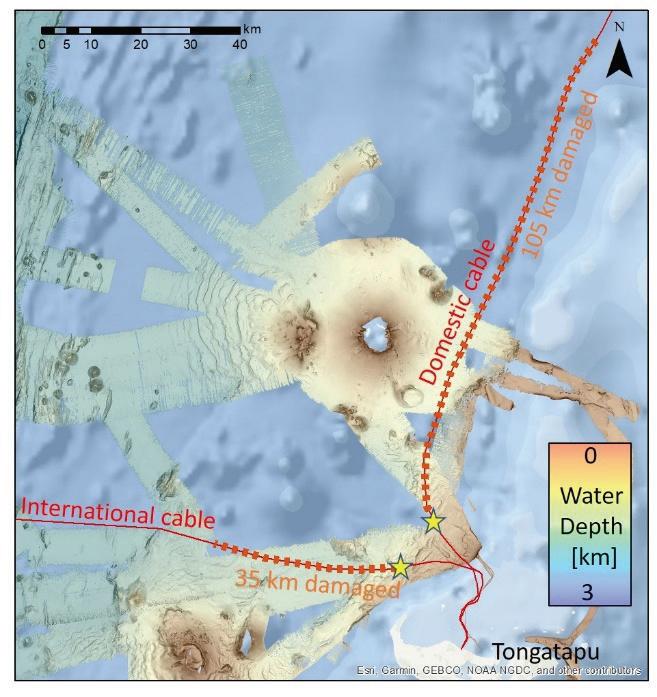
The data we analyse here come from two offshore surveys, performed just a few months after the eruption, including by the RV Tangaroa in April/May 2022 and the Uncrewed Surface Vehicle (USV) Maxlimer in July/August
2022. These surveys acquired: i) multibeam bathymetric data to compare with pre-eruption surveys performed in 2016 and 2017 [14,15] to document any changes to the seafloor; ii) seafloor photography to observe impacts on benthic biological communities; and iii) multi-coring to sample seafloor deposits.
Based on these rapid post-eruption surveys, Seabrook et al.[15] showed that major seafloor change occurred within the caldera, where up to 900 m thickness of material was removed by the eruption; accounting for a missing volume of ~6-7 km3. The gross morphology of the volcano flanks did not change fundamentally, in contrast to other volcanic eruptions such as in 2018 at Anak Krakatau, Indonesia, where half of the volcanic island collapsed into the ocean[16]. No evidence of slope failures was observed on the volcano flanks, nor the surrounding terrain. Instead, localised erosion was observed on the steep volcano flanks, where up to 100 m-deep gullies and trains of upslope-migrating scours were incised on the steepest parts of the volcano flank (Fig. 3), accounting for an additional eroded volume of 3.5 km[3,15]. The dominant picture over the remaining area is of deposition; an average of 2.8 m elevation gain across the surveyed area, with localised accumulations of >20 m in thickness[15]. Seafloor photographs reveal exposed bedrock where sediment has been stripped on the steep volcano flanks and widespread blanketing of volcanic sediment, with ripples locally observed, several tens of km away from the volcano, leading to loss of almost all seafloor life[15]. Coring recovered granular volcanic deposits with sharp bases that fine upwards, which are overlain by finer ash fall-out deposits. These locally erosional and more widely depositional patterns were created by fast and dense seafloor flows of sediment that initiated when pyroclastic flows entered the ocean.
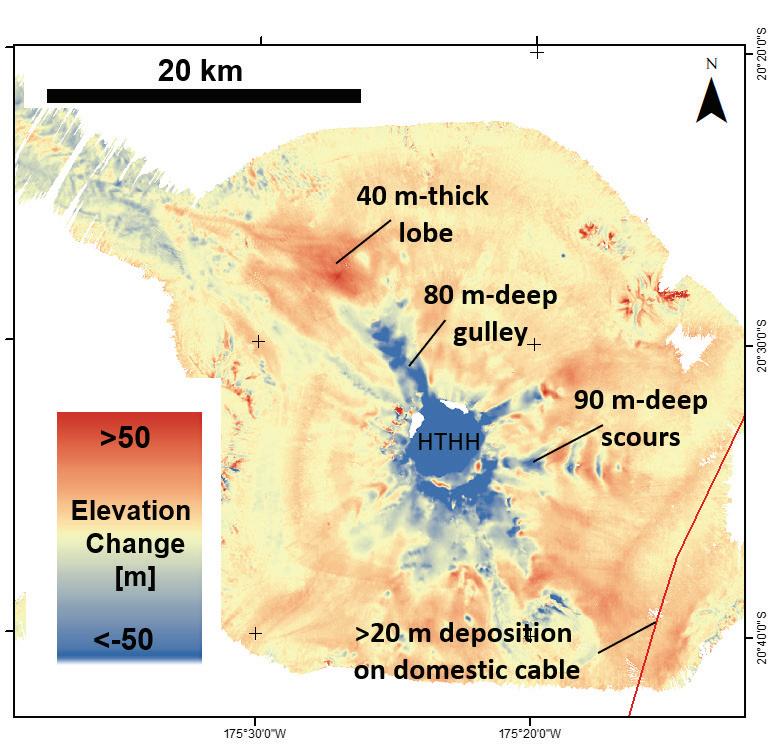
The seafloor flows were funnelled along gullies that radiate from the steep flanks of the volcano; three of which are effectively aimed at the domestic cable that lies within a valley 15 km downslope to the southeast of the caldera rim (Fig. 4). Sediment density flows triggered by pyroclastic flows impacted the domestic cable orthogonally, and were then deflected to the north and south (i.e. parallel to the cable) by the seafloor relief. As the flow transitioned to lower gradients (i.e. within the valley where the domestic cable lies), it deposited up to 22 m of volcanic sediment on top of the cable (Fig. 3). Based on the time between the collapse of the eruptive column and the damage to the domestic cable and the identified flow pathways, we calculate an averaged velocity of 68-122 km/hour for these flows; the fastest velocity documented for any underwater density current. The remarkably fast and voluminous nature of these flows explain the extensive damage to 105 km of the domestic cable. For context, the fastest recorded velocities for sediment density flows to date were 72 km/hour (based on sequential cable breaks due to the Grand Banks landslide offshore Newfoundland in 1929, the 1954 Orleansville earthquake offshore Algeria, and the 2006 Pingtung earthquake offshore Taiwan[3,17]).
The flows were then steered along tortuous paths created by the irregular volcanic topography into deeper water, where they impacted the international cable 70 km from the volcano. Multibeam bathymetric data reveal the presence of bedforms along the flow pathway within a valley flanked by seamounts. The averaged velocity of the flows that reached the international cable is estimated at 34-51 km/hour, and while flows were decelerating, this is still remarkably fast and explains the damage along 35 km of the cable length. For example, the maximum velocity of sediment density flows that damaged multiple cables in 2020 in the deep-sea Congo Canyon, West Africa was 29 km/hour[18].
IMPLICATIONS FOR EXISTING AND FUTURE CABLE ROUTES ALONG THE TONGA-TOFUA ARC
Based on the evidence of damage to the two subsea cables and data gathered from rapid response surveys, we have shown that:
• Individual volcanic eruptions can create multiple pyroclastic flows that radiate from all sides of the volcano flanks.
• The resultant density flows can travel at fast velocities (up to 122 km/hour) and erode the seafloor to >100 m depth on steep slopes.
• At breaks in slope, these flows may suddenly deposit thick accumulations, and can even flow upslope where they have sufficient inertia.
• These dense flows preferentially exploit, and are steered by, pre-existing topography and may maintain high densities over distances of >150 km.
• The volume of material that blanketed the seafloor following the eruption (due to ash fall as well as density flows) has effectively smoothed some of the pre-existing volcanic relief, which may provide more preferable conditions for a surface laid cable.
The 2022 eruption is estimated to be on the order of a 1:1000-year event for this volcano. As a result, it is unlikely that an equivalent eruption of Hunga Tonga-Hunga Ha’apai will occur within the design life of the repaired cables, although there are many similar volcanoes in the region. Little is known about why this eruption escalated so rapidly to become so explosive and there is a need to better understand the controls on such a hazardous event. This eruption also raises questions about hazards posed by the many other active volcanoes along the Tonga-Tofua Arc[15]. Recent mapping around nearby Fonuafo’ou (formerly known as Falcon Island, which is presently submerged) reveals it has a similar dimension caldera, has likely experienced similar eruptions in its past, and is more charged with magma than Hunga Tonga-Hunga Ha’apai is currently. However, most of the volcanoes in the region are poorly or un-surveyed, particularly in the case of those that are entirely submerged and unmonitored. This creates a blind spot with regards to low probability but high impact hazards[19,20].
We now discuss some recommendations. More extensive multibeam bathymetric coverage is required to identify the locations and nature of different volcanoes (especially sub- merged edifices, which are the least well understood), better characterise the terrain for cable routing, and improve the assessment of associated volcanic hazards. Repeated seafloor surveys can provide an indication of volcanoes that have recent or are undergoing phases of activity and can aid in monitoring their development[21]. Regional seismo-acoustic monitoring of volcanic and associated hazards (e.g. seismicity, slope failure) could enable a greater forewarning of events, such as the 2022 eruption. In addition, monitoring could be improved by the use of land-based seismic networks and ocean bottom node arrays. This could include the installation of bespoke instrumented cables[22] or fibre-optic sensing such as distributed acoustic sensing, State of Polarisation or interferometry along standard telecommunications cables[23,24].
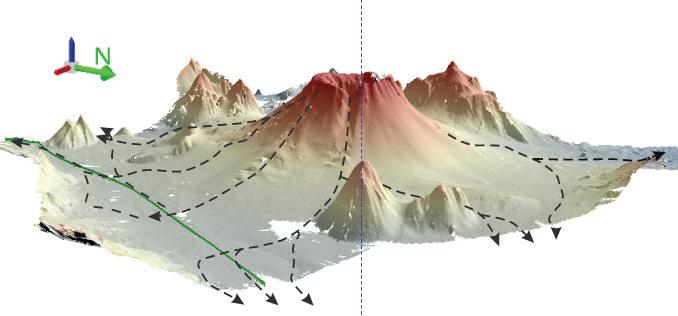
Additional and more geographically-diverse cables routes and landing stations would provide greater resilience; however, identifying alternative appropriate routes is challenging in regions where there is sparse, detailed bathymetric data and as geologically complex as the Tonga-Tofua Arc. Steep slopes lie to the east and north of the islands of Tonga where they transition to a deep-sea trench. This is the focal point for seismogenic earthquakes. The steep slopes can also be prone to slope failure and are incised by submarine canyons, and hence are sub-optimal locations for cable routing[3]. One of the biggest challenges facing the repair operation offshore Tonga was the unprecedented extent of damage, requiring significant lengths of new cable. Holding a more local stock of replacement cable could mitigate this in future; however, the damage caused by the 2022 eruption was unprecedented and this would need to be assessed on a cost-benefit basis.
Broader Implications Of Volcanic Hazards Worldwide
Cable faults related to volcanic hazards are relatively rare, largely because cable routing aims to avoid active volcanic centres. Most natural hazard-related faults are instead attributed to earthquakes, storms and submarine landslides or turbidity currents. However, there have been a small number of faults linked to volcanic hazards, which are not always associated with eruptions. These generally affected small islands, including those in the Pacific and the Caribbean. There is a socioeconomic push to connect these regions; hence, there will be a growth in cable routes that traverse volcanically active areas, meaning that associated hazards cannot necessarily be fully avoided in future.
• We now discuss some of these previously-documented instances of cable damage associated with volcanic hazards. In 1902, eruptions of Mount Pelee, Martinique and La Soufriere, Saint Vincent were accompanied by a loss of submarine telegraphic cable contact[25,26]. Eruptions on the south-west flank of Montserrat caused major disruptions to the regional telecommunications network in the Caribbean in 1997 following pyroclastic flows and dome collapse[27]. A 2015 eruption of the submarine Kick ‘em Jenny Volcano led to damage of the ECFS and SCF cables tens of kilometres away, caused by a landslide and debris flow that originated from a collapse of the crater rim and flank[28,29].
• The aftermath of volcanic eruptions may also pose additional challenges to cable operations for survey, installation and repair.
• It is hard to say with any certainty when a volcanic eruption has ended, particularly in remote regions where there is little or no geophysical monitoring, so establishing at what point it is safe to deploy repair vessels requires a judgement call.
• Volcanic eruptions can cause substantial bathymetric changes, that may pose navigational hazards to vessels. Eruptions may both directly (as a result of ash fall, pyroclastic flows, lava flows or lahars) or indirectly (from the generation of tsunamis) damage nearby ports, coastal infrastructure and ships, meaning repair vessels may need to travel longer distances to reach the site, or to mobilise for repairs[30].
• Pumice rafts (islands of floating volcanic rock produced by some eruptions) can block vessel water intakes, abrade hulls and endanger operations[31]. These rafts may cover thousands of square kilometres of the ocean, hampering efforts to reach repair sites or carry out repairs once there[32].
• Exclusion zones are often imposed around volcanoes during and after activity to protect vessels from potential hazards. This may also make reaching damaged sites logistically challenging. Access can be further complicated where damage to cables has resulted in loss of communications.
• Active and dormant seamounts and other volcanic terrain create a rough seabed topography. Such rough terrain can exacerbate abrasion and suspension fatigue, especially in an energetic ocean.
• Volcanic eruptions may also exert an influence on weather and climatic conditions, resulting in unexpected conditions[33].
Conclusions
The exceptional damage to the cables offshore Tonga following the Hunga Tonga-Hunga Ha’apai eruption was related to pyroclastic flows that entered the ocean and created long run out density flows. This demonstrates that any standoff distances may need to be >100s of kilometres, which is generally impractical for islands along volcanic arcs, particularly given the low probability of such events. However, when they do occur their impacts can be wide-reaching as clearly demonstrated here. Enhanced seafloor mapping and improved volcanic monitoring is required to improve our understanding of volcanic activity and associated hazards, while increased diversity of routes and landing points, backup stocks of cable for repairs, and complementary communications (e.g. low-level satellites) will increase resilience. STF
Acknowledgements
We thank the Kingdom of Tonga for allowing us to undertake this research, and in particular acknowledge the support of Permanent Secretary for Natural Resources; the Nippon Foundation through the NIWA-Nippon Foundation Tonga Eruption Seabed Mapping Project for providing financial support; and The Nippon Foundation-GEBCO Seabed 2030 project and their alumni for their support. This work would not have been possible without the captain, crew, and scientists aboard RV Tangaroa (Voyage TAN2206) and the use of SEA-KIT International’s Uncrewed Surface Vessel Maxlimer for mapping the caldera. We extend our gratitude to everyone involved in these voyages and for assistance in processing the results. We acknowledge funding from the Natural Environment Research Council (NE/X00239X/1 and NE/X003272/1), University of Auckland and support from the International Cable Protection Committee.
References
Burnett, D.R. and Carter, L., 2017. International submarine cables and biodiversity of areas beyond national jurisdiction: the cloud beneath the sea (p. 80). Brill.
Kordahi, E., Rapp, R.J., Stiz, R.K., Sheridan, S., Irish, O.B., Wall, D., Waterworth, G., Perratt, B., Wilson, S., Holden, S. (2019). Global trends in subsea cable system faults, SubOptic, 2019, New Orleans, Session OP8-1
Carter, L., Gavey, R., Talling, P.J. and Liu, J.T., 2014. Insights into submarine geohazards from breaks in subsea telecommunication cables. Oceanography, 27(2), pp.58-67.
Palmer-Felgate A, ‘A Global Comparison of Repair Commencement Times: Update on the Analysis of Cable Repair Data’, ICPC 2018 Plenary Meeting, 10-12 April 2018, Cape Town, South Africa.
Franken, J., Reinhold, T., Reichert, L. and Reuter, C., 2022. The digital divide in state vulnerability to submarine communications cable failure. International Journal of Critical Infrastructure Protection, 38, p.100522.
Global Volcanism Program, 2015. Report on Hunga Tonga-Hunga Ha’apai (Tonga) (Wunderman, R., ed.). Bulletin of the Global Volcanism Network, 40:1. Smithsonian Institution. https://doi. org/10.5479/si.GVP.BGVN201501-243040
Lynett, P., McCann, M., Zhou, Z., Renteria, W., Borrero, J., Greer, D., Fa’anunu, O., Bosserelle, C., Jaffe, B., La Selle, S. and Ritchie, A., 2022. Diverse tsunamigenesis triggered by the Hunga Tonga-Hunga Ha’apai eruption. Nature, 609(7928), pp.728-733.
Global Volcanism Program, 2022. Report on Hunga Tonga-Hunga Ha’apai (Tonga) (Crafford, A.E., and Venzke, E., eds.). Bulletin of the Global Volcanism Network, 47:2. Smithsonian Institution.
Global Volcanism Program, 2022. Report on Hunga Tonga-Hunga Ha’apai (Tonga) (Bennis, K.L., and Venzke, E., eds.). Bulletin of the Global Volcanism Network, 47:3. Smithsonian Institution.
Poli, P. & Shapiro, N. M. Rapid characterization of large volcanic eruptions: measuring the impulse of the Hunga Tonga Ha’apai explosion from teleseismic waves. Geophys. Res. Lett. 49, e2022GL098123 (2022).
Gupta, A.K., Bennartz, R., Fauria, K.E. and Mittal, T., 2022. Eruption chronology of the December 2021 to January 2022 Hunga Tonga-Hunga Ha’apai eruption sequence. Communications Earth & Environment, 3(1), pp.1-10.
Proud, S.R., Prata, A.T. and Schmauß, S., 2022. The January 2022 eruption of Hunga TongaHunga Ha’apai volcano reached the mesosphere. Science, 378(6619), pp.554-557.
Pakoksung, K., Suppasri, A. and Imamura, F., 2022. The near-field tsunami generated by the 15 January 2022 eruption of the Hunga Tonga-Hunga Ha’apai volcano and its impact on Tongatapu, Tonga. Scientific reports, 12(1), pp.1-15.
Cronin, S., Brenna, M., Smith, I., Barker, S., Tost, M., Ford, M., Tonga’onevai, S., Kula, T. and Vaiomounga, R., New Volcanic Island Unveils Explosive Past. Eos http://eos.org/scienceupdates/new-volcanic-island-unveils-explosive-past (2017).
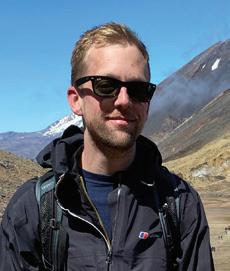
Seabrook, S., Mackay, K., Watson, S.J., Clare, M.A., Hunt, J.E., Yeo, I.A., Lane, E., Clark, M.R., Wysoczanski, R., Rowden, A.A., Hoffmann, L.J., Armstrong, E., Williams, M.J.M (2023, In Review). Pyroclastic density currents explain far-reaching and diverse seafloor impacts of the 2022 Hunga Tonga Hunga Ha’apai eruption. Nature Communications (Pre-print).
Hunt, J.E., Tappin, D.R., Watt, S.F.L., Susilohadi, S., Novellino, A., Ebmeier, S.K., Cassidy, M., Engwell, S.L., Grilli, S.T., Hanif, M. and Priyanto, W.S., 2021. Submarine landslide megablocks show half of Anak Krakatau island failed on December 22nd, 2018. Nature communications, 12(1), pp.1-15.
Talling, P.J., Paull, C.K. and Piper, D.J., 2013. How are subaqueous sediment density flows triggered, what is their internal structure and how does it evolve? Direct observations from monitoring of active flows. Earth-Science Reviews, 125, pp.244-287.
Talling, P.J., Baker, M.L., Pope, E.L., Ruffell, S.C., Jacinto, R.S., Heijnen, M.S., Hage, S., Simmons, S.M., Hasenhündl, M., Heerema, C.J. and McGhee, C., 2022. Longest sediment flows yet measured show how major rivers connect efficiently to deep sea. Nature communications, 13(1), pp.1-15.
Cassidy, M. and Mani, L., 2022. Prepare now for big eruptions. Nature, 608, pp.469-471.
Goff, J. and Terry, J.P., 2016. Tsunamigenic slope failures: the Pacific Islands ‘blind spot’?. Landslides, 13(6), pp.1535-1543.
Caress, D.W., Clague, D.A., Paduan, J.B., Martin, J.F., Dreyer, B.M., Chadwick, W.W., Denny, A. and Kelley, D.S., 2012. Repeat bathymetric surveys at 1-metre resolution of lava flows erupted at Axial Seamount in April 2011. Nature Geoscience, 5(7), pp.483-488.
Howe, B.M., Arbic, B.K., Aucan, J., Barnes, C.R., Bayliff, N., Becker, N., Butler, R., Doyle, L., Elipot, S., Johnson, G.C. and Landerer, F., 2019. SMART cables for observing the global ocean: science and implementation. Frontiers in Marine Science, 6, p.424.
Jousset, P., Currenti, G., Schwarz, B., Chalari, A., Tilmann, F., Reinsch, T., Zuccarello, L., Privitera, E. and Krawczyk, C.M., 2022. Fibre optic distributed acoustic sensing of volcanic events. Nature communications, 13(1), pp.1-16.
Nishimura, T., Emoto, K., Nakahara, H., Miura, S., Yamamoto, M., Sugimura, S., Ishikawa, A. and Kimura, T., 2021. Source location of volcanic earthquakes and subsurface characterization using fiber-optic cable and distributed acoustic sensing system. Scientific reports, 11(1), pp.1-12.
Anderson, T., 1908. VII. Report on the eruptions of the Soufrière in St. Vincent in 1902, and on a visit to Montagne Pelée in Martinique -Part II. The changes in the districts and the subsequent history of the volcanoes. Philosophical Transactions of the Royal Society of London. Series A, Containing Papers of a Mathematical or Physical Character, 208(427-440), pp.275-303.
Pyle, D.M., Barclay, J. and Armijos, M.T., 2018. The 1902–3 eruptions of the Soufrière, St Vincent: Impacts, relief and response. Journal of Volcanology and Geothermal Research, 356, pp.183-199.
Global Volcanism Program, 1997. Report on Soufriere Hills (United Kingdom) (Wunderman, R., ed.). Bulletin of the Global Volcanism Network, 22:5. Smithsonian Institution. https://doi. org/10.5479/si.GVP.BGVN199705-360050.
Global Volcanism Program, 2015. Report on Kick ‘em Jenny (Grenada). In: Sennert, S K (ed.), Weekly Volcanic Activity Report, 22 July-28 July 2015. Smithsonian Institution and US Geological Survey.
Global Volcanism Program, 2017. Report on Kick ‘em Jenny (Grenada). In: Sennert, S K (ed.), Weekly Volcanic Activity Report, 26 April-2 May 2017. Smithsonian Institution and US Geological Survey.
Paris, R. 2015, Source mechanisms of volcanic tsunamis. Philosophical Transactions of the Royal Society A, 373, 1–15, doi:10.1098/rsta.2014.0380.
Carey, R., Soule, A.S., Manga, M., White, J. D. L., McPhie, J., Wysoczanski, R., Jutzeler, M., Tani, K., Yoerger, D., Fornari, D., Caratori-Tontini, F., Houghton, B., Mitchell, S., Ikegami, F., Conway, C.; Murch, A., Fauria, K., Jones, M., Cahalan, R., McKenzie, W. 2018, The largest deepocean silicic volcanic eruption of the past century. Science Advances, 4, 1–7, doi:10.1126/ sciadv.1701121.
Jutzeler, M., Marsh, R., Sebille, E., Mittal, T., Carey, R. J., Fauria, K. E., Manga, M., McPhie, J. (2020). Ongoing Dispersal of the 7 August 2019 Pumice Raft from the Tonga Arc in the Southwestern Pacific Ocean. Geophysical Research Letters, 47, 0–3, doi:10.1029/2019gl086768 Marshall, L.R., Maters, E.C., Schmidt, A., Timmreck, C., Robock, A. and Toohey, M., 2022. Volcanic effects on climate: recent advances and future avenues. Bulletin of Volcanology, 84(5), pp.1-14.
DR MIKE CLARE is a Principal Researcher in Ocean Biogeoscience at the National Oceanography Centre, UK. His research focuses on deep sea processes, the influence of climate change and human activities on the seafloor, and assessing risks to seafloor infrastructure. He has published >70 papers in international peer-reviewed scientific journals. Since 2019, he has been the Marine Environmental Advisor to the International Cable Protection Committee, and provides evidence for policy to various stakeholders. He currently leads cutting-edge research into monitoring deep sea hazards and recent cable-damaging natural hazards in the deep-sea Congo Canyon and offshore Tonga.