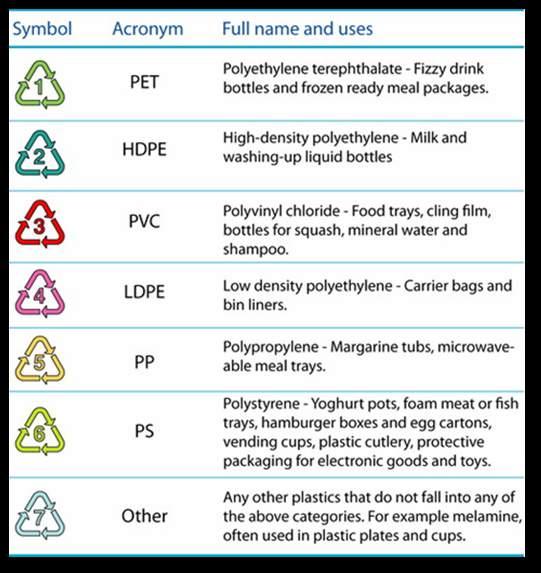
29 minute read
Polyester Recycling Strategies
SWARANJALI SUHAS JOSHI DR. RAVINDRA KALE
M.Tech student Guide Fibres and Processing Technology Department, Institute of Chemical Technology, Mumbai
Advertisement
Introduction Polymeric materials can be classified as thermosets and thermoplastics. Thermoset polymers refer to the irreversible polymerization and this type of polymer is cured by chemical reaction or heat and becomes infusible and insoluble material. Thermoplastics are made up of linear molecular chains and this polymer softens on heating and hardens when cooled [1–6]. Thermoplastic polymers are represented by a large range of plastic materials. There are three types of thermoplastic polymers. The crystalline thermoplastics, usually translucent with molecular chains which present a regular arrangement. Compared to other types, these polymers have more mechanical impact resistance. These polymers present unique properties (physical, thermal and electrical) that make them suitable for many applications. The injection moulding process is the main technique of polymer processing which allows the fabrication of different kinds of parts, such as the computer mouse [11–15]. These plastic materials can be modelled into a variety of products for a wide range of applications due to the fact that thermoplastic polymers are inexpensive, lightweight and durable. In the last decades, the production of plastics has increased significantly causing a big problem in the whole world regarding the discarded end-of-life plastics which are accumulated as debris in landfills and in natural habitats worldwide and by the management methods related to constantly growing resources of plastics. In the last years, the problem of recycled plastics was attempted to be solved by several methods (such as mechanical recycling or chemical recycling) leading to products ready to be used in determined conditions, in the most economic, ecological and rational way [16–23]. The purpose of this review is to present the advantages and disadvantages of thermoplastic polymers used in industrial applications, the processes used in the recycling and perspectives for a green bioindustry. Thermoplastic Polymers Due to the ideal properties of the thermoplastic polymers such as corrosion resistance, low density,
14 COVER STORY high strength, and user-friendly design, plastic usage has become much higher than the usage of aluminium or other metals. For example, density is a very important parameter because it reveals information about the intrinsic strength of the construction that is supposed to be created, as in the case of flax reinforcement when PP and LDPE are the best choices (because of their low density), since its purpose is to produce a composite that is as light as possible. The glass transition temperature (Tg) is another characteristic that is very important when studying polymer mechanical properties, because the flexibility of amorphous polymers is reduced drastically when they are cooled below Tg. At these temperatures, there are no dimensional changes or segmental motion in the polymer. Also, the mechanical properties are very important in the case of thermoplastic polymers, mostly the tensile strength (important for their performance under stress) and tensile modulus (the resistance of polymers to elastic deformation) [12, 24,31]. The main reasons which make the thermoplastic polymers used in various applications are: - The thermoplastic polymers can be processed by several methods leading to various kinds of plastic products; - They are used for a specific application several compounding, operating condition, additives, fillers, and reinforcements; - Several manufacturing systems are used at this moment to produce plastic items with the lowest cost range [33–35]. The recycling and incineration are the usual aspects of recovery methods in the case of thermoplastic polymers. The incineration presents some problems like the production of toxic gases and the residue ash which contains lead and cadmium. The recycling presents advantages such as reduction of environmental problems and saving both material and energy [33, 35, and 36]. Advantages and Key Properties of PET Resin • It is very strong and lightweight and hence easy and efficient to transport • It is known for its good gas (oxygen, carbon dioxide) and moisture barrier properties • It exhibits excellent electrical insulating properties • PET has broad range of use temperature, from -60 to 130°C • It has higher strength and stiffness than PBT (Polybutylene Terephthalate) • As compared to PBT, it also has higher heat distortion temperature (HDT) • It has low gas permeability, in particularly with carbon dioxide • PET is suitable for transparent applications, when quenching during processing • PET does not break or fracture. It is practically shatter-resistant and hence, a suitable glass-replacement in some applications • It is recyclable and transparent to microwave radiation • PET is approved as safe for contact with foods and beverages by the FDA, Health Canada, EFSA & other health agencies [38]. • Excellent resistance to alcohols, aliphatic hydrocarbons, oils, greases and diluted acids Moderate resistance to diluted alkalis, aromatic and halogenated hydrocarbons [40]. Disadvantages of PET • Flammable– This is definitely an advantage in that they can be melted down, however smouldering plastics can release toxic fumes into the environment. • Cost of Recycling – While recycling is a plus, recycling is a very costly endeavour. • Volume – In the United States 20% of our landfill is made up of plastics. As more products are being made of plastics, where will this lead us in the future • Durability – This is an advantage as well as a disadvantage. Plastics are extremely durable, which means that they last a long time. Those plastics in the landfill will be there for years. Plastics make our lives easier, however is their cost on the environment worth it? We can only hope that soon someone will invent a way to safely and cheaply melt and reuse plastics [39]. Recycling of PET The huge amounts of PET products bottles, spinning, and packaging films cause serious environmental pollution. Commonly, PET content reaches about 12 % in municipal plastic waste. In fact, the separation of PET bottles from municipal waste represents one of the most successful examples of polymer recycling. Numerous ways of recycling disposable beverage bottles are available, including methods of chemical recycling, such as hydrolysis, amylolysis, glycolysis, etc., or physical recycling by remelting. The plastic can be degraded in the environment by four mechanisms: photo-degradation, thermo-oxidative degradation, hydrolytic degradation, and biodegradation by microorganisms. The natural degradation of plastic begins with photo-degradation due to the UV light from the sun which provides the activation energy required to initiate the incorporation of oxygen atoms into the polymer, leading to thermo-oxidative degradation. In this step, the plastic becomes brittle and it’s fracturing into smaller pieces until the polymer chains reach sufficiently low molecular weight to be metabolized by microorganisms. The microorganisms convert the carbon of the polymer chains to carbon dioxide or incorporate it into biomolecules, but this process will take at least 50 years [36]. So, a solution to these problems will be the recycling, because most
commodity plastics are relatively stable, making monomer recovery poor. A driving force for PET recycling is that PET products have a slow rate of natural decomposition [12]. PET is a non-degradable plastic in normal conditions as there is no known organism that can consume its relatively large molecules. Complicated and expensive procedures need to be operated in order for PET to degrade biologically [13]. Many researchers reported that in order to achieve successful PET recycling, PET flakes should meet certain minimum requirements [13 – 16]. Description of recycling process Primary recycling (pre-consumer industrial scrap) • It is the oldest way of recycling PET and is also known as re-extrusion • Low cost, requites uncontaminated scraps and deals with only single type waste • The recycled scrap or waste is mixed with virgin material. Second grade material (Mechanical recycling) • Secondary recycling includes sorting arid separation of waste, removal of contaminates and is also known as mechanical recycling steps • Reduction of size by crushing and grinding, extrusion by heat and reforming Tertiary recycling (Chemical recycling) • Chemical recycling is done usually by means of solvolytic chain cleavage • Tins process can either be a total de-polymerization back to its monomers or a partial De-polymerization on to its oligomers. Quaternary Recycling (Energy Recovery) • Energy recovery is defined as the process how companies convert post-use, non-recyclable plastics into a range of useful products such as fuels and electricity and energy recovery is turning landfill-bound plastics and waste into a reliable and renewable energy source. In-plant recycling In-plant recycling, otherwise known as re-expulsion, is the most established method for reusing polyester family. It alludes to the in-lodge reusing of the scrap materials that have comparative highlights to the first items. The recycled scrap or waste is either mixed with virgin material to assure product quality, or used as a second grade material. This methodology guarantees effortlessness and ease, yet obliges uncontaminated scrap, and just manages single-sort wastes. It is the recycling of perfect, uncontaminated Industrial discard materials [41]. Mechanical recycling In this approach, the polymer is separated from its associated contaminants and it can be readily reprocessed into granules by conventional melt extrusion. Mechanical recycling includes the sorting and separation of the wastes, size reduction; melt filtration and reforming of the plastic material. The basic polymer is not altered during the process. The main disadvantage of this type of recycling is the deterioration of product properties in every cycle. This occurs since the molecular weight of the recycled resin is reduced due to chain-scission reactions caused by the presence of water and trace acidic impurities. A secondary recycling process presents some unique problems that may cause it to be inappropriate for the production of food-contact articles, particularly if the recycler had little or no control over the waste stream entering the recycling facility [41]. A secondary recycling methodology introduces some special issues that might make it to be designed for the generation of nourishment, especially if recycler had diminutive or no power under the waste stream toward the inside reusing competence. The more unpredictable and sullied the waste is, the more troublesome it is to reuse it mechanically. Among the primary issues of optional reusing is the heterogeneity of the strong waste, and the debasement of the item properties, every time it is reused. The same predicament is the misuse of items made of the same pitch yet with different colour which usually impart undesirable grey colour. It is the recycling of clean, uncontaminated single-type waste which remains the most popular, as it ensures simplicity and low cost, especially when done ‘‘in-plant’’ and feeding with scrap of controlled history. The recycled scrap or waste is either mixed with virgin material to assure product quality or used as a second-grade material. Primary recycling of industrial scrap produced during the manufacture of food-contact articles is not expected to pose a hazard to the consumer [44]. Chemical recycling Unlike physical recycling, chemical recycling involves transformation of polymer chain. The polymer backbone under the recycling process is degraded into monomer units (i.e. depolymerisation) or randomly ruptured into larger chain fragments (i.e. random chain scission) with associated formation of gaseous products. The chemical recycling is carried out either by solvolysis or by pyrolysis; the former through degradation by solvents including water, and the latter through degradation by heat in absence of oxygen or air, or vacuum. Chemical recycling yields monomers, petroleum liquids and gases. Monomers are purified by distillation and drying, and used for manufacture of polymers. Chemical recycling, generally recognized as compound reusing, includes change of the PET polyester linear chains. Normally via
16 COVER STORY method for solvolytic chain cleavage, this methodology is able to reverse the depolymerisation in the direction of its monomers, or a fractional de-polymerization in the direction of its oligomers and other chemicals. Distinctive solvolysis systems included in PET depolymerisation is depicted in. Since PET is a polyester containing ester bunches, it can be effectively severed by a few reagents, for example like amine groups, acid, alcohols, water, and glycols. Additionally, PET can be shaped through a reversible poly-condensation response; subsequently, it can be changed back to its monomer or oligomer units by switching the response to the other way through the expansion of a build-up item. These low sub-atomic weight items can then be cleansed and reused as crude materials to create top notch compound items [41][43]. Among the reusing techniques, substance reusing is the most settled and the stand out as per the standards of ‘economic advancement’ which is characterized as the improvement that addresses the issues of present era without trading off the capacity of future eras to address their issues; World Commission on Environment and Improvement 1987) . This is on the grounds that concoction reusing prompts arrangement of the crude materials (monomers) from which the polymer was initially integrated. The environment is not surcharged, and there is no requirement for additional assets for the proliferation of PET. The response component for PET de-polymerization comprises of three reversible responses: (a) the carbonyl carbon in the polymer chain experiences quick protonation, whereby the carbonyl oxygen gets changed over to a second hydroxyl bunch; (b) the hydroxyl oxygen of the included hydroxyl-bearing atom gradually assaults the prorogued carboxyl carbon atom; and (c) the carbonyl oxygen (which was changed over to hydroxyl amass in the first step) and a proton. There are three primary techniques in PET synthetic reusing relying upon the included hydroxyl bearing particle: (1) glycol for Glycolysis, (2) methanol for Methanolysis, and (3) water for hydrolysis. Other strategies may incorporate amylolysis. From the point forward, various examination works have been done with a specific end goal to completely comprehend the synthetic pathways included in the depolymerisation routines, and along these lines, progress the yield of the desired products obtained by these methods. This process can be used with mechanical recycling as a complementation. Chemical recycling is defined as the process in which polymers are chemically converted to monomers or partially depolymerized to oligomers through a chemical reaction (a change occurs to the chemical structure of the polymer). The resulted monomers can be used for new polymerizations to reproduce the original or a related polymeric product. This method is able to transform the plastic material into smaller molecules, suitable for use as feedstock material starting with monomers, oligomers, or mixtures of other hydrocarbon compounds [41]. The chemical reactions used for decomposition of polymers into monomers are: • Hydrogenation • Glycolysis • Gasification • Hydrolysis • Pyrolysis • Methanolysis • Chemical depolymerization • Thermal cracking • Catalytic cracking and reforming • Photo-degradation • Ultrasound degradation • Degradation in microwave reactor According to the reagent used, different products are obtained. The main depolymerisation processes that have reached commercial maturity up to now are Glycolysis and Methanolysis. Nowadays there is growing interest in hydrolysis for the chemical recycling of PET, since it is the only method with the reaction products terephthalic acid (TPA) and ethylene glycol (EG), i.e. the monomer from which PET is produced. This is associated with the trend in the new factories for PET synthesis to produce it directly from TPA and EG, thus replacing dimethyl terephthalate (the traditional monomer) from the technological process [50]. Disadvantage of this method is the use of high temperature (200–250 ⁰C) and pressure (1.4–2 MPa) as well as long time needed for complete depolymerisation. Commercially, hydrolysis is not widely used to produce food-grade recycled PET, because of the cost associated with purification of the recycled TPA. Hydrolysis of PET can be carried out as (a) alkaline hydrolysis, (b) acid hydrolysis and (c) neutral hydrolysis [41]. Alkaline Hydrolysis Alkaline hydrolysis of PET is usually carried out with the use of an aqueous alkaline solutionof NaOH or KOH, of a concentration of 4–20 wt. %. The reaction products are EG and the disodium or di- potassium terephthalate salt, according to the chemical reaction shown below
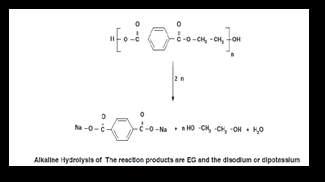

PET flakes were hydrolysed to sodium terephthalate and ethylene glycol (EG) in NaOH solutions before oxygen introduction. Because sparingly soluble sodium terephthalate in concentrated NaOH solutions was stable to the oxidation, the TPA yield was approximately 100 mol% under all conditions. In contrast, EG was oxidized to oxalate and CO2, and the maximum oxalic acid yields Thus, apart from the monomer (TPA) a valuable by product (oxalic acid) was obtained. Factors such as temperature, time and alkali concentration influencing the kinetics of the alkaline de-polymerization of PET in NaOH solution. Also the choice of solvent plays an important role in the alkaline depolymerisation of PET. The main advantage of alkaline hydrolysis is that it can tolerate highly contaminated, post-consumer PET such as magnetic recording tape, metallized PET film, or photographic film (X-ray film) [14]. The process is relatively simple and less costly than Methanolysis. PET hydrolysis in aqueous alkaline solute ion was investigated by Karayiannis. PET decomposition was conducted in a 2 L stainless-steel autoclave reactor equipped with a digital temperature-control system, an agitator, and a manometer as a pressure indicator. The reaction took place with a constant NaOH concentration and different reaction-time intervals and temperatures. At the appropriate time the reactor was cooled and the reaction mixture was neutralized to pH 6.5 with H2SO4 and filtered to remove unreacted PET solids. The TPA in the mixture was precipitated by acidification with H2SO4 to pH 2.5 and the mixture was filtered and washed with methanol. The solid TPA produced was dried in an oven at 80 ⁰C and weighed. A great increase in the TPA yield on increasing the reaction temperature was observed. This is expected if the chemical reaction is the rate-determining step. At the highest-studied temperature of 200⁰C a TPA yield of 98% was obtained in only 1 h [41]. PET Hydrolysis in a Non-Aqueous Alkaline Solution: It has been observed that addition of an ether (such as dioxin or THF) in nonaqueous alkali solutions accelerates the rate of chemical degradation of PET [21]. One possible explanation is that ethers accelerate the percolation of hydroxide ions and increase the ionic strength of the hydroxide ion and, therefore, the decomposition of PET is increased. Methyl Cellulose is a chemical compound combining the properties of ether together with those of an alcohol. It is for this reason that this substance was selected for this study. The ether part will lead to swelling of the PET solid and the alcoholic part will support the action of KOH in destroying the chemical structure of PET during depolymerization (PET surface is easily attacked by alcohols). The addition of an ether (such as dioxane, or tetrahydrofuran (THF)) as a mixed solvent with an alcohol (methanol, or ethanol) accelerated the chemical degradation of PET. It is for this reason that the alkaline hydrolysis of PET at 110–120 ⁰C with a nonaqueous solution of KOH in methyl cellulose was selected to be studied by Karayannidis. Pellets of potassium hydroxide were dissolved in methyl cellulose and the alkali hydroxide solution was added to a glass reaction vessel equipped with a reflux condenser, an inertgas flow, a stirrer and a heating device. The reactor was immersed in a silicon-oil bath in order to obtain high enough temperatures. PET flakes were added intothe reactor and the inert-gas flow was started, together with the agitation. After a certain period the inertgas flow stopped and the temperature was set to the desired point. After reaching the desired temperature, the reaction time started and the PET decomposition was followed for a specified time period. After that time, the reaction mixture was cooled rapidly by immersing the flask in cold water. The mixture was then filtered to remove the un-decomposed PET solids and dried in an oven at 110 ⁰C. In the final product, 500 mL of distilled water were added, in order to dissolve all of the potassium terephthalate. The solution was filtered again and the procedure described previously for the isolation of TPA was followed [43]. Treatment processes based on partial PET alkaline hydrolysis are widely used in the polyester fiber industry. The effect of such processes on the mechanical properties of fibers, oligomer content and change of the molecular weight distribution, or loss of fiber mass has been investigated. From the point of view of research on PET chemical recycling, an interesting relationship between PET mass loss, reaction time, and the concentration of the NaOH aqueous solution used, as well as between oligomer contents and the molecular weight distribution of degraded PET has been observed. NaOH solutions in methanol react with PET significantly faster than analogous aqueous solutions. Namboori and Haith have compared the reactivity of NaOH aqueous solutions, as well as solutions of sodium tert-butoxide in tertbutanol, sodium isopropoxide in
isopropyl alcohol, sodium methoxide in methanol, and sodium ethoxide in ethanol with PET. They have demonstrated that, of the above-mentioned solutions, sodium ethoxide in ethanol is the most reactive and an aqueous solution of sodium hydroxide is the least reactive. In the recycling of PET to terephthalates of alkali metals or alkaline-earth metals, a process described by Benzaria may be crucial. The de-polymerization is carried out in a mixer-extruder with the use of solid NaOH at temperatures of 100–200 ⁰C. After the distillation of EG from the post reaction mixture under reduced pressure, a corresponding salt of terephthalic acid in the form of a powder is obtained. In this method the necessity of separating the glycol and water mixture is eliminated, which is undoubtedly its essential advantage. The degree of polyester saponification achieved a level of about 97% [41]. Alkaline Hydrolysis in the Presence of a Phase Transfer Catalyst Phase transfer catalysed alkali decomposition of PET taken from post-consumer soft-drink bottles, was revealed to be an efficient method for the reproduction of pure terephthalic acid. The kinetics of the depolymerisation reaction was extensively studied. The effects of temperature, alkali concentration, PET particle size, PET concentration and catalyst to PET ratio on the TPA yield were investigated. This method had been applied in PET fibres as well as Nylon-46 and Nylon-66 fibers. Very good results were obtained for the de-polymerization of PET and the yield of TPA was as high as 93%. Kosmidis extended the use of the phase-transfer catalyst in the depolymerization of PET flakes taken from waste soft-drink bottles and the reaction kinetics was extensively studied [32]. Acid Hydrolysis Acid hydrolysis is performed most frequently using concentrated sulphuric acid, although other mineral acids such as nitric or phosphoric acid have also been employed. In order to avoid high pressures and temperatures in the reaction vessel, a concentrated sulphuric acid (14.5 M) has been proposed by Pusztaszeri, Brown, O’Brien and Sharma. However, the process becomes very costly due to the need to recycle large amounts of concentrated H2SO4 and the purification of EG from the sulphuric acid. TPA recovery from PET scrap material in concentrated sulphuric acid at 60– 93 ⁰C has been also described (acid concentration of, at least, 87 wt.%). EG was recovered from the final filtrate through extraction with organic solvents such as trichloroethylene. In another patent, the production of pure TPA was described by acid hydrolysis of PET in a 90 wt.% H2SO4 solution at 85–90 ⁰C. A substantial drawback of PET hydrolysis by concentrated sulphuric acid is the high corrosiveness of the reaction system and the generation of large quantities of inorganic salts and aqueous wastes. Different parameters, such as acid concentration, time, temperature and PET particle size, on the decomposition and reaction yield was investigated there. Acid hydrolysis of PET in sulphuric acid at different temperatures and solution concentrations was reported. The depolymerisation reaction was carried out in a 0.5 L reactor equipped with a reflux condenser and a magnetic agitator. The required amount of the sulphuric-acid solution (70–83 wt.%), together with the PET flakes, was added into the reactor and heated to the desired reaction temperature (between 30 and 90 ⁰C). The agitation started in order to keep the mixture homogeneous and the reflux condenser set. The reaction time started and the mixture was allowed to react for 3–5 h. Afterwards, the mixture was filtered to separate the TPA produced and the unreacted PET. A solution of KOH was added to the solid product. In this way, the TPA reacted to form the dipotassium salt, while PET remained unreacted. Finally, the mixture was filtered again, dried in an oven until it had a constant weight and weighed in order to calculate the percentage of unreacted PET. The depolymerisation reaction of PET with water in an acid (H2SO4) environment proceeds according to the reaction in below. The detailed mechanism of acid depolymerisation is as under [41][43].
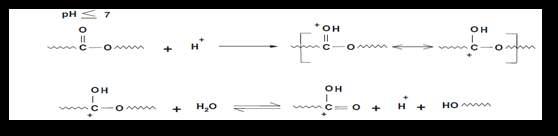
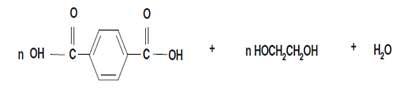
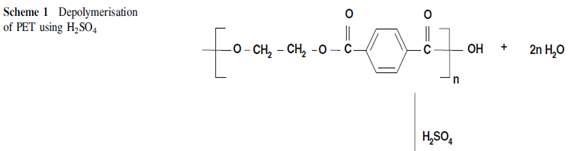
Glycolysis Another most important method in chemical processing of PET is glycolysis. This process is used widely on a commercial scale. The glycolysis reaction is the molecular degradation of PET polymer by glycols, in the presence of transesterification catalysts, mainly metal acetates, where ester linkages are broken and replaced with hydroxyl terminals [41].
Quaternary recycling The energy content of the plastics waste can be recovered by incineration. When the collection, sorting and separation of plastics waste are difficult or economically not viable, or the waste is toxic and hazardous to handle, the best waste management option is incineration to recover the chemical energy stored in plastics waste in the form of thermal energy. This is carried out in special type of reactors called incinerators, to burn wastes in the presence of air in a controlled manner to convert hydrocarbons of the plastic into carbon dioxide and water. The heat produced by burning plastics in the waste in the form of superheated steam can be utilized for generating electricity through turbine generators, and the residual heat from the waste stream for heating residential and industrial buildings. The melt residue from the incinerator is free from toxicity hazards and may be disposed of by landfill. Although
polymers are actually high yielding energy sources, this method has been widely accused of being ecologically unacceptable owing to the health risk from air borne toxic substances such as dioxins (in the case of chlorine containing polymers). It should admit that it is not possible to have zero emission in the incineration of waste plastic. Apart from the aforementioned methods, direct reuse of a plastic material (i.e., PET) could be considered as a ‘‘zero-order’’ recycling technique [8]. In a lot of countries, it is a common practice for PET bottles to be refilled and reused. However, this should be done with great care since plastic bottles are more likely than glass to absorb contaminants that could be released back into food when the bottle is refilled. Moreover, refilling of a PET bottle with a drink with high alcohol content may lead to degradation of the macromolecular chains with unexpected results. Worldwide, the main end-use of post-consumer PET is for the production of fibers (almost 70%), with only 4% of PET recycled with chemical methods. Among the above recycling techniques, the only one acceptable according to the principles of sustainable development (development that meets the needs of the present generation without compromising the ability of future generations to meet their needs) is chemical recycling; since it leads to the formation of the raw materials (monomers) from which the polymer is made. In this way the environment is not surcharged and there is no need for extra resources (monomers) for the production of PET. This method refers to the recovery of the plastic’s energy content. The most effective way to reduce the volume of organic materials which involves the recovery of energy is represented by incineration. This method is a good solution because it generates considerable energy from polymers, but it’s not ecologically acceptable because of the health risk from airborne toxic substances, for example dioxins (in the case of heavy metals, chlorine-containing polymers, toxic carbon, and oxygenbased free radicals). Among the above recycling techniques, the only one acceptable according to the principles of sustainable development is chemical recycling, because this method leads to the formation of the monomers from which the polymer is made [41] [50].
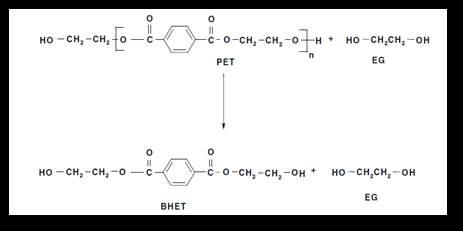
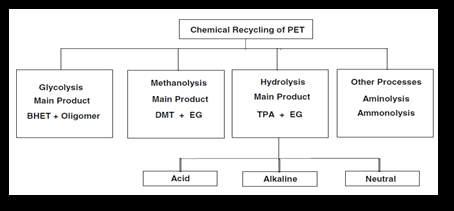
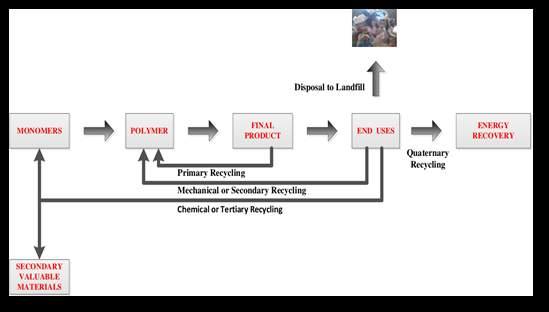
A fourth recycling technique, called the quaternary recycling process for plastic waste administration is concerned with the recuperation of its vitality content. Incineration (ignition), pointed towards recuperation of vitality is as of now the most powerful approach to decrease the volume of natural material, inferable from an absence of other reusing potential outcomes. Plastics, either thermoplastic or thermosetting, are really highyielding vitality sources. One litre of warming oil has a net calorific estimation of 10,200 kcal, while 1 kg of plastics discharges 11,000 kcal worth of vitality. For examination reason it can be said that 1 kg of charcoal briquettes have a net calorific estimation of 4800 kcal. It was assessed that by smouldering 1 ton of waste, roughly 250 l of warming oil can be spared. Clean incineration of civil strong waste is broadly acknowledged in nations like Sweden and Germany (50 % of aggregate MSW), Denmark (65 %), Switzerland (80 %), and Japan (70 %). Albeit there exist extremely stringent discharge regulations, more than 50 reject units are working in Germany. As has been mentioned above, among the different reusing methods, the one and only satisfactory strategy as per the standards of supportable advancement is substance reusing, since it prompts the development of the crude materials (monomers) from which the polymer was originally produced [41]. References 1. Amin, S.; Amin, M. Thermoplastic elastomeric (TPE) materials and their use in outdoor electrical insulation. Rev. Adv. Mater. Sci. 2011, 29, 15–30. 2. Ribeiro, M.C.S.; Fiúza, A.; Ferreira, A.; Dinis, M.D.L.; Meira Castro, A.C.; Meixedo, J.P.; Alvim, M.R. Recycling Approach towards Sustainability Advance of Composite Materials’ Industry. Recycling 2016, 1, 178. [CrossRef] 3. Ashori, A. Wood-plastic composites as promising green-composites for automotive industries! Bioresour. Technol. 2008, 99, 4661–4667. [CrossRef] [PubMed] 4. Pascault, J.-P.; Sautereau, H.; Verdu, J.; Williams, R.J. Thermosetting Polymers; CRC Press: Roca Raton, FL, USA, 2002; Volume 64. 5. Brazel, C.S.; Rosen, S.L. Fundamental Principles of Polymeric Materials; John Wiley & Sons: Hoboken, NJ, USA, 2012. 6. Yang, Y.; Boom, R.; Irion, B.; van Heerden, D.-J.; Kuiper, P.; de Wit, H. Recycling of composite materials. Chem. Eng. Process. Process Intensif. 2012, 51, 53–68. [CrossRef] 7. Schlosser, E.; Nass, B.; Wanzke, W. Flame Retardant Combination for Thermoplastic Polymers L. U.S. Patent 6,547,992, 15 April 2003. 8. Bicerano, J. Prediction of Polymer Properties; CRC Press: Roca Raton, FL, USA, 2002. 9. Ultem, L.; STM, U.S.; Ultrablend, S. Polymer Science Dictionary; Springer: Dordrecht, The Netherlands, 2017; ISBN 978-94-0240893-5. 10. Nicholson, J. The Chemistry of Polymers; Royal Society of Chemistry: London, UK, 2017. 11. Giboz, J.; Copponnex, T.; Mélé, P. Microinjection molding of thermoplastic polymers: A review. J. Micromech. Microeng. 2007, 17, R96. [CrossRef] 12. Van Krevelen, D.W.; Te Nijenhuis, K. Properties of Polymers: Their Correlation with Chemical Structure; Their Numerical Estimation and Prediction from Additive Group Contributions; Elsevier: Amsterdam, The Netherlands, 2009. 13. Khan, M.I.; Zagho, M.; Shakoor, R. A Brief Overview of Shape Memory Effect in Thermoplastic Polymers. In Smart Polymer Nanocomposites; Springer: Berlin, Germany, 2017; pp. 281–301. 14. Wei, C.; Esposito, D.; Tauer, K. Thermal properties of thermoplastic polymers: Influence of polymer structure and procedure of radical polymerization. Polym. Degrad. Stab. 2016, 131, 157–168. [CrossRef] 15. Michler, G.H.; Balta-Calleja, F.J. Mechanical Properties of Polymers Based on Nanostructure and Morphology; CRC Press: Roca Raton, FL, USA, 2016; Volume 71. 16. Kolek, Z. Recycled polymers from food packaging in relation to environmental protection. Pol. J. Environ. Stud. 2001, 10, 73–76. 17. Jiun, Y.L.; Tze, C.T.; Moosa, U.; Mou’ad, A.T. Effects of Recycling Cycle on Used Thermoplastic Polymer and Thermoplastic Elastomer Polymer. Polym. Polym. Compos. 2016, 24, 735.
18. Hopewell, J.; Dvorak, R.; Kosior, E. Plastics recycling: Challenges and opportunities. Philos. Trans. R. Soc. Lond. B Biol. Sci. 2009, 364, 2115–2126. [CrossRef] [PubMed] 19. Drobny, J.G. Handbook of Thermoplastic Elastomers; Elsevier: Amsterdam, The Netherlands, 2014. 20. Rodriguez, F.; Cohen, C.; Ober, C.K.; Archer, L. Principles of Polymer Systems; CRC Press: Roca Raton, FL, USA, 2014. 20. Lee, S.-T.; Park, C.B. Foam Extrusion: Principles and Practice; CRC Press: Roca Raton, FL, USA, 2014. 22. Fried, J.R. Polymer Science and Technology; Pearson Education: London, UK, 2014. 21. Myshkin, N.; Pesetskii, S.; Grigoriev, A.Y. Polymer Tribology: Current State and Applications. Tribol. Ind. 2015, 37, 284–290. 22. Fried, J.R. Polymer Science and Technology; Pearson Education: London, UK, 2014. 23. Myshkin, N.; Pesetskii, S.; Grigoriev, A.Y. Polymer Tribology: Current State and Applications. Tribol. Ind. 2015, 37, 284–290. 24. Park, H.M.; Li, X.; Jin, C.Z.; Park, C.Y.; Cho, W.J.; Ha, C.S. Preparation and properties of biodegradable thermoplastic starch/clay hybrids. Macromol. Mater. Eng. 2002, 287, 553–558. [CrossRef] 25. Park, H.M.; Li, X.; Jin, C.Z.; Park, C.Y.; Cho, W.J.; Ha, C.S. Preparation and properties of biodegradable thermoplastic starch/clay hybrids. Macromol. Mater. Eng. 2002, 287, 553–558. [CrossRef] 26. Koo, J.H. Polymer Nanocomposites: Processing, Characterization, and Applications; McGrawHill Professional Pub.: New York, NY, USA, 2006. 27. Schulz, U. Review of modern techniques to generate antireflective properties on thermoplastic polymers. Appl. Opt. 2006, 45, 1608–1618. [CrossRef] [PubMed] 28. Mishra, J.K.; Hwang, K.-J.; Ha, C.-S. Preparation, mechanical and rheological properties of a thermoplastic polyolefin (TPO)/ organoclay nanocomposite with reference to the effect of maleic anhydride modified polypropylene as a compatibilizer. Polymer 2005, 46, 1995–2002. [CrossRef] 29. Turi, E. Thermal Characterization of Polymeric Materials; Elsevier: Amsterdam, The Netherlands, 2012. 30. Mano, J.; Koniarova, D.; Reis, R. Thermal properties of thermoplastic starch/synthetic polymer blends with potential biomedical applicability. J. Mater. Sci. Mater. Med. 2003, 14, 127–135. [CrossRef] [PubMed] 31. Wollerdorfer, M.; Bader, H. Influence of natural fibres on the mechanical properties of biodegradable polymers. Ind. Crops Prod. 1998, 8, 105–112. [CrossRef] 32. 31.Nichols, C.; Moore, T.; Griffith, S. Thermoplastic Polymers with Improved Infrared Reheat Properties. U.S. Patent 09/973,436, 9 October 2001. 33. Van de Velde, K.; Kiekens, P. Thermoplastic polymers: Overview of several properties and their consequences in flax fibre reinforced composites. Polym. Test. 2001, 20, 885–893. [CrossRef] 34. Mohammadzadeh, M. Characterization of Recycled Thermoplastic Polymers. Master’s Thesis, University of Borås, Borås, Sweden, 2009. 35. Saleh, T.A.; Danmaliki, G.I. Polymer Consumption, Environmental Concerns, Possible Disposal Options, and Recycling for Water Treatment. In Advanced Nanomaterials for Water Engineering, Treatment, and Hydraulics; IGI Global: Hershey, PA, USA, 2017; pp. 200–222. 36. Favis, B.D.; Le Corroller, P. Polymeric material and process for recycling plasticblends. U.S. Patent 9,670,344, 6 July 2017. 37. Francis, R. Recycling of Polymers: Methods, Characterization and Applications; John Wiley & Sons: Hoboken, NJ, USA, 2016. 38. omnexus.specialchem.com 39. essaylead.com 40. www.ktu.lt 41. link.springer.com 42. Vijaykumar Sinha. "Pet Waste Management by Chemical Recycling: A Review", Journal of Polymers and the Environment, 09/03/2008 43. www.electrochemsci.org 44. David E. Nikles, Medhat S. Farahat. "New Motivation for the Depolymerization Products Derived from Poly(Ethylene Terephthalate) (PET) Waste: a Review", Macromolecular Materials and Engineering, 2005 45. Claudia Pudack, Manfred Stepanski, Peter Fässler. "PET Recycling – Contributions of Crystallization to Sustainability", Chemie Ingenieur Technik, 2020 46. Trinath Biswal, Pravin Kumar Kar. "chapter 1 Plastic Pollution and Its Effect on the Environment", IGI Global, 2020 47. thisisplastics.com 48. B. Naresh, Vinod Kumar Singh, V. K. Sharma. "Integration of RF rectenna with thin film solar cell to power wearable electronics", International Journal of Microwave and Wireless Technologies, 2020 49. George P. Karayannidis, Dimitris S. Achilias. "Chemical Recycling of Poly(ethylene terephthalate)", Macromolecular Materials and Engineering, 2007. 50. www.mdpi.com