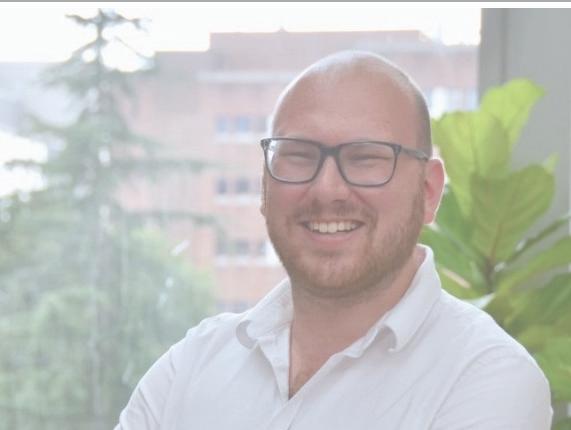
11 minute read
Modeling Innovation Patterns in Solar Technology
By Sameer Rao
Complete darkness. The year is 2030 and the United States power grid is no better than it was in 2020. However, there are now Perovskite solar panels on the roof. In a flash, the energy stored from the panels kicks in and the day is saved. Perovskites are a new generation of solar panels which have garnered much attention for their high efficiency. Understanding what drives innovation for solar technologies is essential to determining technological improvement in time to halt climate change. Noah Kittner, an assistant professor in UNC-Chapel Hill’s Department of Environmental Sciences and acclaimed researcher for his work in sustainability, has recently published research on the innovation of emerging solar technologies, where he and his team study learning curves to understand if perovskites demonstrate typical innovation patterns found in solar technology. 2 Dr. Kittner and his team embarked on their research with the intention of comparing Perovskite to two other solar technologies: dye-sensitized solar cells and quantum dot solar cells. Dye-sensitized solar cells and quantum dot solar cells are two well-established solar technologies which have long been the subjects of clean energy research. Perovskite solar cells, on the other hand, have only been in studies since 2009. The team analyzed power conversion efficiency (PCE) values, the efficiency at which a solar cell converts sunlight into usable energy, reported in recognized test labs and compared them to the values reported in scientific literature. 1 By comparing the PCE values in test labs to those documented by literature, Kittner and his team could confirm the accuracy of the PCE values and utilize them to construct innovation rate models called “learning curves.” Dr. Kittner explains that learning curves are graphic models that investigate the root causes of a phenomenon – solar technology in this case. 1 Learning curves illustrate the why: what is the impetus behind solar efficiency innovation? Dr. Kittner and his team investigated three factors that affect PCE learning curves - time since entering the industry, number of publications on that solar technology, and the number of patents on the technology. 2 To put their ideas to the test, Dr. Kittner and his team first needed to confirm the values reported by the test labs. His team first scraped a database with keywords on the technologies such as “perovskites” or “dye-sensitized” to find the proper articles. They then used text data mining to locate PCE values within literature, searching mainly the abstract sections of the literature. 2 After compiling a list of the PCE values, Dr. Kittner and his team compared the values reported in scientific literature to those reported by test labs. They found that the PCE values were nearly identical. 2 Kittner and his team now had accurate enough dates and PCE values to construct a learning curve. They modeled two equations
Advertisement
“Understanding what drives innovation for solar technologies is essential to determining whether we can improve our technology in time to halt climate change. “
Dr. Noah Kittner
Figure 1: Uptrend of PCE values in solar photovoltaics as number of research patents filed increases. Image courtesy of Kittner et al.

based on the previous hypothesized factors and constructed graphs for each technology. Kittner and his team found some eye-opening information. Perovskites’s PCE advancement was discovered to be greater than that of dye-sensitized solar. However, the Perovskite advancement was much less than quantum solar dots. 1 This could be explained by a number of factors. Although many people believed that perovskites were the fastest growing emerging solar technology, quantum solar dots have been around for quite some time. There are thousands of publications on quantum solar dots which could serve as proof that the cumulative number of publications may correlate to a greater performance improvement for the technology. Kittner also explains that quantum solar dots had a number of patents early on as the technology has applications in LED lighting technology, consumer electronics, and medicine which have caused them to make more PCE growth progress in a shorter period of time than that of perovskites and dye-sensitized technology. 1 Although perovskites have received wide attention in their infancy, their learning rate is comparable to dye-sensitized technology. Quantum solar dots had unprecedented innovation that surpassed its competitors. Dr. Kittner and his team can conclude that research from patents to publications on the technology play a significant role in the learning rate of a solar technology. Kittner says that a combination of continued research as well as government policy is necessary to advance solar technologies. “We need a synergy between both investing in the R&D side and taking the lessons learned from past policy mistakes to create better energy technology and a more livable planet.” 1 Kittner and his team are optimistic about the future of solar technologies and believe that although they are on a short time limit, if these technologies continue to be researched and developed, they can catch up to the necessary efficiency rates. With continued drive for innovation, society could move closer to abandoning fossil fuels and promotes future wellbeing. Hopefully, the clean energy problem is a learning curve for mankind, one which will be overcome with continued effort.
References
1. Interview with Noah Kittner, Ph. D. 2. Sunter, D.; Ferrall I.; Knapstein, J.; Garfield, D.; Kittner, N.; Kammen, D. IEEE 7th World Conference on Photovoltaic Energy Conversion. 2018, 7, 1147- 1151.
CLOSING THE GAP: USING
CONDUCTIVE POLYMERS TO MAKE
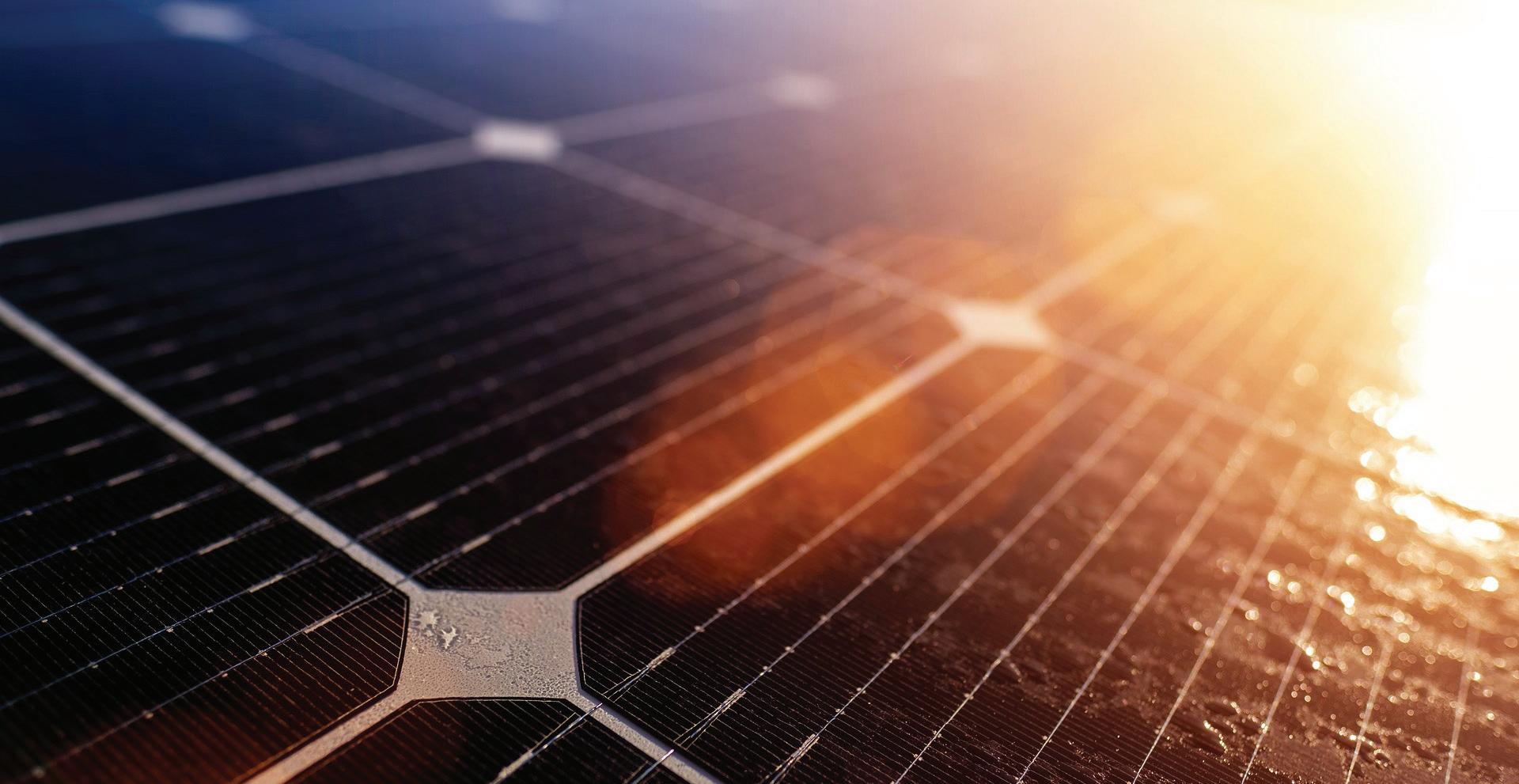
MORE EFFICIENT SOLARCELLS
HARRIS DAVIS
After well over a century of dependence on coal and oil, energy infrastructure in the modern world is devoted to combustion systems. Now, as we look for ways to supply our energy demand from more sustainable sources, we face economic barriers. Solar power is one of the more popular green systems among scientists, but current processes for refining the semiconductors found in most commercial solar cells are expensive. Consider silicon, which covers the planet’s coasts as sand (silicon dioxide). While the material is abundant, using it to build efficient photovoltaics requires an extremely expensive purification process. 2 The question becomes: what if there was a material that offered silicon’s conductive properties, but did not require such costly refinement? The short answer is that it already exists—in the form of a group of materials called organic polymers (think plastics). However, engineering polymers that will conduct electricity the same way as semiconductors do requires meticulous chemical design, which researchers at UNC-Chapel Hill are currently developing. The common denominator for all solar cells is that they take advantage of a principle known as the photovoltaic effect. Electrons in a valence band become excited and enter the “conduction band” in which they can flow freely; the energy which allows the electron to become excited comes from the absorption of sunlight in the form of a photon. 9 This excitation lets electrons move freely and produce a current. The energy required to excite an electron from the valence shell to the conduction band is known as the “band gap,” and this requirement determines the colors—or wavelengths of light—a photovoltaic material can absorb. To even consider conduction and band gaps in organic polymers, the compounds must be conjugated—a property which can be synthetically designed by a chemist. A conjugated material has delocalized electrons, similar to those which exist naturally in metals. Scientists engineering organic polymer-based photovoltaics (OPVs) spend much of their time experimenting with energy levels and designing ideal band gaps, as this principle is critical in generating voltage. Generally speaking, deeper band gaps, which involve low-energy valence shells, are associated with higher voltages in photovoltaic materials. More electronegative materials—such as fluorine—offer a way to achieve those deeper band gaps, and thus greater solar cell efficiency. Since 2006, Dr. Wei You and his group of researchers in UNC’s chemistry department have been working to enhance the photovoltaic effect in OPVs. A highlight of their work has been the development of a methodology to add fluorine— the most electronegative element—to polymers to deepen their band gaps. In 2009, the You group had the idea to replace specific hydrogen atoms in conjugated organic polymers with fluorine, as this element is not much larger than hydrogen and can
PRIORTO THE 1980S, EVERYBODY THOUGHT THAT PLASTICS WERE
INSULATORS. BUT WITH HEEGER’S, SHIWA’S, MACDIARMID’S RESULTS, WE ARE NOW SEEING THAT PLASTICS ACTUALLY CAN CONDUCT ELECTRICITY.
largely maintain other structural features of the molecule. Since then, they have been working to make fluorination viable as a means of band gap deepening. In early 2011, Dr. You’s team achieved the “first successful application of fluorine to a donor–acceptor conjugated polymer.” 4,5 While 7% efficiency does not sound like much when compared to some semiconductor-based solar cells, 6 this was a record for OPVs at the time, and the team’s work was praised by groups such as Thomson Reuters upon publication. 2
of interest for dFT-HTAZ: a higher open-circuit voltage and, as a result, greater efficiency. Further experimentation found that blending dFT-HTAZ with the ITIC-Th1 fluorinated electron acceptor brought efficiency to nearly 10%, which constituted a nearly 300% improvement from the non-fluorinated HTAZ polymer. 7 The success of this process has broad implications for the field of OPVs. Making use of fluorine’s high electronegativity to deepen the energy levels of these polymers has a massive ability to make these materials more efficient. Although the idea of building OPVs has been around since the 1980s, pioneered by researchers such as Alan Heeger and Richard Friend, 1 it was only after the principle of band gap engineering was introduced to the field that the use of these polymers became viable. We now know OPVs can be extremely efficient: the record efficiency achieved by Dr. You and his colleagues was over 13.5%,8 and the most efficient organic polymer solar cell to date was built in January 2020, with an efficiency over 18%. 9 However, barriers to OPVs still exist in the mainstream. The next logical step to bring down the cost of solar power is to find a way for these materials to compete with semiconductors such as silicon in the energy market, and conjugated polymers face a severe disadvantage in terms of stability. While 3–-5 years of use has been demonstrated for some OPVs, silicon cells can last for 30. But researchers, including those at the You Group, are working to close this gap, and as they make progress they are earning national attention for the potential of their work. The affordability—as well as the physical flexibility— of OPVs has caught the eye from the United States military as potential lightweight power sources to replace the heavy batteries currently in use overseas. It is difficult to predict the length of time between now and widespread implementation of OPVs. According to Dr. You, however, the outlook on this new frontier is promising. The specific nature of the You Group’s work, which achieves higher efficiency by fluorinating a polymer linker (as opposed to the entire molecule), offers experimental freedom to researchers looking to build off of these results. This freedom is also attractive to researchers who are exploring new polymerization methods. With a growing interest in inexpensive OPVs within the scientific community, we can expect to see shifts towards economic soundness on the front of sustainable energy.
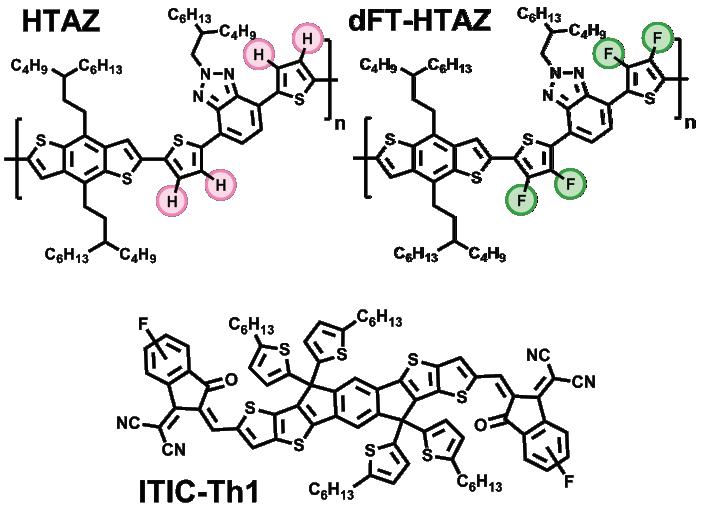
Flash forward ten years, and the You Group has honed the fluorination process extensively. They recently experimented with a dual fluorinated linker unit (dFT) 7 —which is a chemical that is used to join monomers into polymers—to test its efficiency against similar non-fluorinated conjugated polymers. Using a polymer lacking functional groups as a model (HTAZ), members of the You Group led by Jeromy Rech found that, with dual fluorinated linkers (dFT-HTAZ), the polymer operated at a much higher efficiency than its non-fluorinated counterpart. 7 After synthesizing HTAZ and dFT-HTAZ, the You group examined the chemical properties of both for comparison. While the polymers had band gaps of similar energy (which allowed them to absorb similar wavelengths of light to generate an electric current), lower orbital occupation was found for dFT-HTAZ (i.e., the band gap was deeper within the molecule). This contributed to the key photovoltaic property
Left: Dr. Wei You; Right: Jeromy Rech
More information about conjugated polymer photovoltaics is available at the You Group’s website: https://www.weiyougroup.org/. Figure 1: Models of non-fluorinated vs. difluorinated polymers
Image courtesy of reference 6 (refer to publication).
References (cont. from pg 23)
1. Interview with Wei You, Ph.D. 2/5/2020 2. Email with Wei You, Ph.D. 2/19/2020 3. Gokul Dharan, Jordan Hanania, Kailyn Stenhouse, Jason Donev. Energy Education - Conduction band. https://energyeducation.ca/encyclopedia/Conduction_band (accessed February 17th, 2020). 4. Zhou H, Yang L, Stuart AC, Price SC, Liu S, You W. Development of Fluorinated Benzothiadiazole as a Structural Unit for a Polymer Solar Cell of 7% Efficiency. Angewandte Chemie International Ed. 2011, 50(13), 2995–2998. doi:10.1002/anie.201005451 5. Price SC, Stuart AC, Yang L, Zhou H, You W. Fluorine Substituted Conjugated Polymer of Medium Band Gap Yields 7% Efficiency in Polymer−Fullerene Solar Cells, Journal of the American Chemical Society 2011, 133(12), 4625-4631. doi:10.1021/ja1112595 6. Green MA. High efficiency silicon solar cells. In Seventh EC Photovoltaic Solar Energy Conference; Adolf Goetzberger, Willeke Palz, G. Willeke, Eds; Springer: Dordrecht, 1987; pp 681-687. 7. Rech J, Yan L, Peng Z, Dai S, Zhan X, Ade H, You W. Utilizing Difluorinated Thiophene Units to Improve the Performance of Polymer Solar Cells. Macromolecules 2019, 52(17), 6523-6532. doi:10.1021/acs. macromol.9b01168 8. He D, Zhao F, Xin J, Rech J, Wei Z, Ma W, You W, Li B, Jiang L, Li Y, Wang C. A Fused Ring Electron Acceptor with Decacyclic Core Enables over 13.5% Efficiency for Organic Solar Cells. Advanced Energy Materials. 2018, 8(30), 1802050. doi:10.1002/aenm.201802050 9. Liu Q, Jiang Y, Jin K, Qin J, Xu J, Li W, Xiong J, Liu J, Xiao Z, Sun K, et al. 18% Efficiency organic solar cells. Science Bulletin 2020. doi:10.1016/j.scib.2020.01.001.
Authentically Diverse Environment Authentically Diverse Environment +
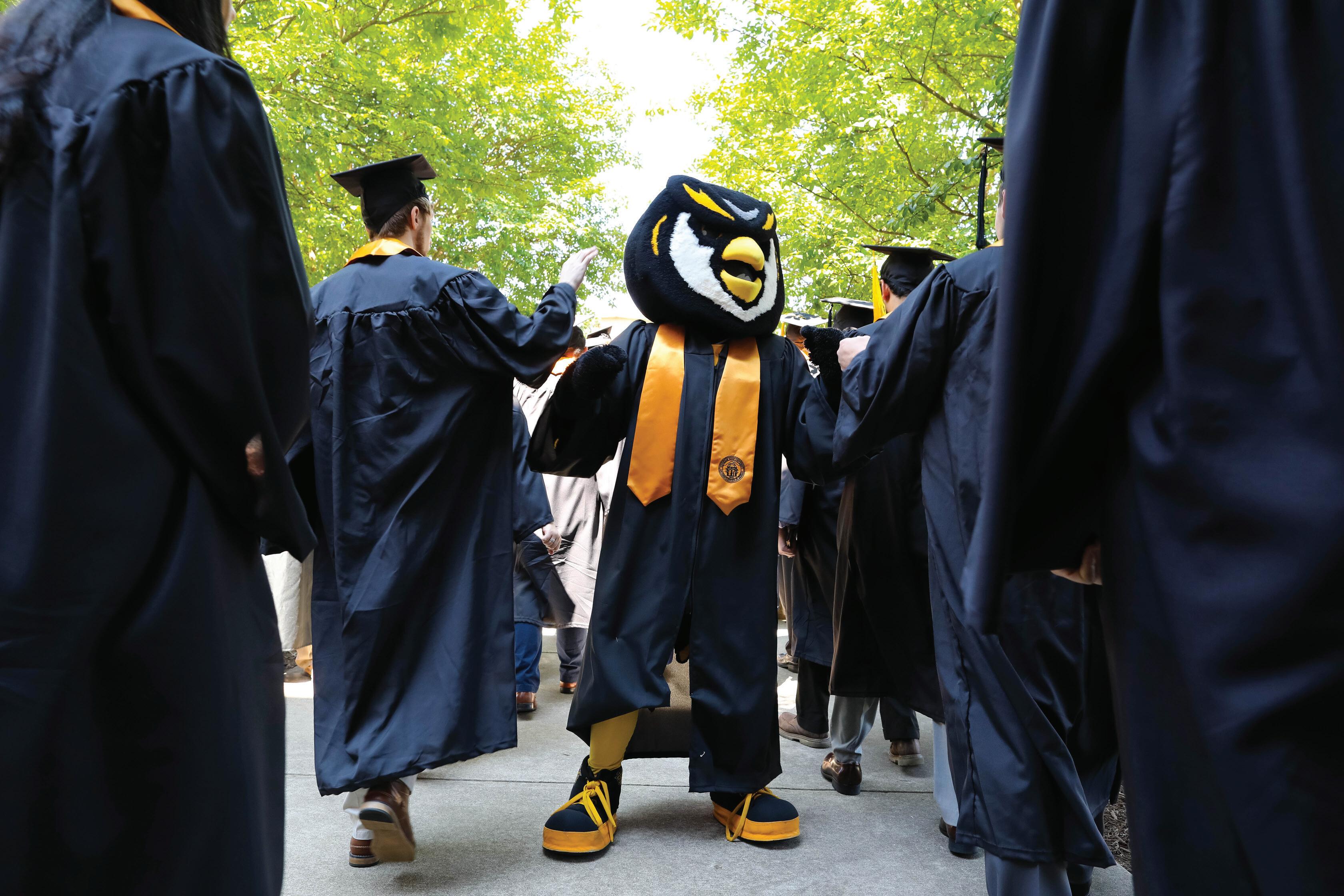
Research Driven Education Research Driven Education =
Future Ready Graduates
Take a virtual tour today at: kennesaw.university-tour.com Future Ready Graduates
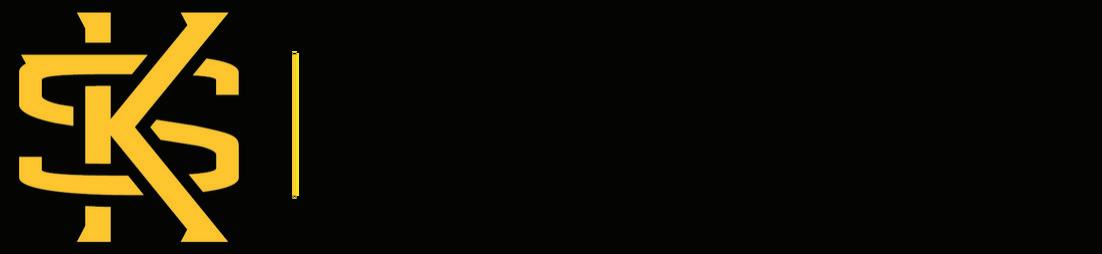