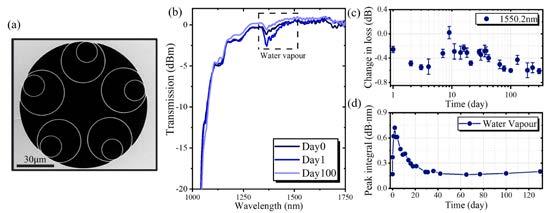
6 minute read
Research income
| High Performance Silica Optical Fibres
Challenge
To assess the long-term optical and mechanical performance of hollow-core fibres (HCFs) in environments and configurations relevant to future deployment in various applications. To develop techniques and fabrication processes to mitigate any reliability or aging effects observed.
Progress
HCFs are a specialty optical fibre where light is guided in an air core, surrounded by a carefully designed cladding structure, consisting of thin glass (usually silica) membranes (see Figure 1a). In recent years, advanced fibre design and improved fabrication methods have significantly reduced the minimum loss achievable in a HCF [1] and these fibres are now being deployed in various applications, including telecommunications [2]. Beyond low loss, HCFs are particularly attractive for telecommunications as light transmission in an air-filled core significantly reduces non-linearity and latency (transmission time) as compared to conventional fibres where light is transmitted in glass. However, the long-term performance of these fibres is as yet largely unexplored and is a critical factor for future success. The impact of the gas composition and pressure inside the holes which run along the length of these novel fibres could be important in this long-term performance.
This year, we have focused on investigating the impact of the gas inside the core on the consistency of a HCF’s optical properties. We have previously shown that the gas pressure inside the holes of a HCF immediately post-fabrication is much lower than atmospheric [3]. Now, we have been studying the consistency of the transmission properties of different HCF designs when the fibres are exposed to atmospheric air over long time periods.
Here we show initial results from a nested antiresonant nodeless fibre (155m, NANF, Figure 1a); this type of HCF which was chosen as this fibre design is very similar to that recently used to demonstrate record low loss HCF transmission [1]. The fibre under test was sealed immediately after fabrication (to avoid atmospheric gas ingress until the experiment started). The fibre was then spliced at the optical input end to allsolid fibre (to provide a consistent coupling of light into the fibre) and the output end was opened up to atmospheric gas species and the fibre was kept in a laboratory (22, 40%RH (relative humidity)). The transmission of the fibre was then carefully recorded periodically over ~ 1 year.
Co-Investigator contact:
Dr Natalie Wheeler nvw1v10@orc.soton.ac.uk | High Performance Silica Optical Fibres
Figure 1: (a) A SEM image of a HCF (NANF design). (b) The evolution of the transmission spectra through the NANF for different measurement days after opening the fibre end to atmospheric gas. (c) The change in the loss of the NANF at 1550.2 nm. (d) The evolution of the water vapour absorption within the NANF based on the water vapour absorption feature at 1441.4 nm. The transmission spectra through the NANF recorded for different days after opening up to atmospheric gas are compared in Figure 1b and overall shows no significant changes (considering the measurement uncertainty) over the time period measured up until this point. This reliability of the NANF transmission is highlighted in Figure 1c which shows the fibre transmission over time at a wavelength of 1550.2 nm. Degradation of the optical performance was not observed even after 304 days of atmospheric exposure. During this measurement, the evolution of water vapour absorption within the NANF was also observed in the wavelength range between ~1300 and 1450 nm. As an example, the evolution of the water vapour absorption line at a wavelength of 1441.4 nm is shown in Figure 1d. The dramatic increase for the first ~8 days was due to the initial sub-atmospheric pressure of the post-fabrication HCF [3] and the following reduction was attributed to absorption of water vapour onto the silica surface within the fibre’s core [4]. The results shown here indicate that this water vapour absorption has negligible impact on the transmission of this type of HCF and this may be because of the very small fraction of power overlapping with the glass surface defining the hollow core, further work is ongoing to study this in more detail.
Future plans
The work described is part of a larger ongoing study into the performance of different HCF designs in a variety of different environmental conditions which are relevant to their deployment in a range of applications, including telecommunications.
References
[1] H. Sakr et al. “Hollow Core NANFs with Five Nested Tubes and Record Low Loss at 850, 1060, 1300 and 1625 nm,” in Proc. 2021 Optical Fiber Communications Conference (OFC) 2021, F3A.4 1-3. [2] euNetworks Fiber UK Limited, “euNetworks deploys Lumenisity Limited CoreSmart hollow core fibre cable in London,” Apr. 14 2021. [Online]: https://eunetworks.com/app/uploads/2021/04/euNetworks- deploys-Lumenisity-Hollowcore-Fibre-in-London FINAL.pdf [3] S. Rikimi et al. “Pressure in As-drawn Hollow Core Fibers,” in OSA Advanced Photonics Congress (AP) 2020, paper SoW1H.4 [4] S. Rikimi et al. “Comparison between the Optical Performance of Photonic Bandgap and Antiresonant Hollow Core Fibers after Long-Term Exposure to the Atmosphere,” accepted for presentation at OSA Optical Fiber Communication Conference (OFC) 2022.
| High Performance Silica Optical Fibres
Short-wavelength operation (<1950 nm) of Cladding-pumped Thulium Fibre Laser in a Power Scaleable Fibre Design
Co-Investigator contact:
Professor Jayanta Sahu jks@orc.soton.ac.uk
Challenge
Two-micron lasers are of great interest for a range of applications in laser surgery, plastics processing, spectroscopy, and pumping of mid-IR sources. Tm-doped silica fibres (TDFs) are the preferred gain medium for power scaling of 2 μm sources as well as they are capable of operating in the wavelength range from < 1700 nm to 2100 nm. By optimising the glass composition within the TDF core, a two-for-one cross-relaxation process can be exploited, that enables Tm-doped fibre laser (TDFL) efficiency to reach well above its quantum limit with 79xnm pumping.
An efficient cross-relaxation process requires a high Tm concentration resulting in a high core numerical aperture (NA) that degrades the beam quality in a large core fibre. Furthermore, the strong three-level nature at the short wavelength side of Tm emission band poses significant challenges for 79xnm cladding-pumped TDFLs to operate below 1950 nm with high efficiency that can be achieved at long wavelengths.
Progress
Our research has focused on the development of TDF through engineering the core glass composition and tailoring the rare-earth doping profile that enables TDFL to operate at short wavelengths with a good output beam quality. Important fibre design features include a high Tm-concentration of >3 wt% to promote crossrelaxation process when cladding-pumped at 79xnm and a large core with a confined Tm-doping region to reduce signal reabsorption while maintaining an adequate pump absorption in a double-clad TDF. | High Performance Silica Optical Fibres
Figure 1: (a) Refractive index profile of confined Tm-doped fibre and (b) Optical spectra of tunable TDFL. Figure 1a Figure 1b
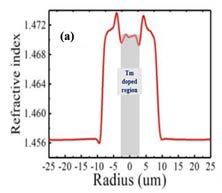
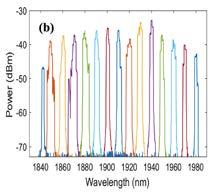
Figure 1 (a) shows the refractive-index profile of confined TDF. A 19 μm diameter core, with NA 0.19, has a central 6 μm confined Tm-doped region. The octagonal inner cladding has a diameter of 80 μm and is coated with a low-index polymer providing the cladding NA of 0.46. The small-signal pump absorption is 7 dB/m. The TDF with confined doping has been tested for tunable laser operation in a Littrow-configuration using a diffraction grating (300 lines/mm). We obtained a continuous tuning and stable operation of the laser from 1850 nm to 1980 mm, as shown in Figure 1b. Throughout the tuning range, the output power of the laser was marinated above 5W, which was primarily limited by the optics and launched pump power. We measured the beam quality factor (M2) as 1.2. The laser slope efficiency has reached >50% across this tuning range with a maximum efficiency of 60% at 1880nm has been obtained. We also achieved 21W of output power at 1880 nm and 15W at 1850 nm by placing the TDF in a water bath. Further optimisation of the laser design would offer the prospect of power scaling of TDFL in the short-wavelength side of the Tm transmission band with a good output beam quality using the confined-doped fibre design.