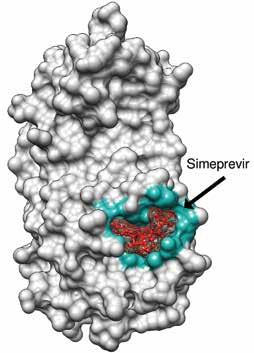
14 minute read
Anti-fungal Resistance Magnitude of the problem and impact
Three key protein targets essential for the function of SARS-CoV-2 were used to screen a large library of ~12,000 small molecule drug candidates. The targets were the main protease of the virus, Mpro or 3CLpro, the RNA -dependent RNA polymerase (RdRp, essential for viral replication), and the helicase (also part of the virus’ replicative machinery). The docking calculations were validated by redocking drugs into the relevant protein binding sites to recapitulate the binding poses in the experimental structure. The calculations were run on large GPU cluster machines generously made available during the pandemic by Oracle Cloud Systems. The top 80-100 drug candidates identified by the docking calculations were subjected to molecular dynamics calculations to improve the estimates of the relative binding energies of drugs to proteins, and their binding poses in the protein active sites. Subsequent literature searches disclosed that >30 per cent of our predicted repurposing candidates for all three SARS-CoV-2 protein targets have experimental validation data, a very impressive validation rate for the computational methods employed.
Red color is the drug inside the binding pocket Blue color highlights the interacting residues in the binding pocket. Grey color is the protein
Fig3: Surface binding representation of Simeprevir with MPro.
In silico approaches to high-throughput drug screening are set to revolutionise pandemic drug discovery efforts.
SARS-CoV-2 Mpro inhibitors
Mpro is a key protease essential to SARS-CoV-2 function. We therefore screened drug libraries for compounds that might bind and inhibit Mpro . Several of the top ten best binding drug candidates were antiviral agents simeprevir, sofosbuvir, lopinavir, ritonavir and remdesivir. The more interesting candidates had similar binding affinities and were of diverse activity classes. These were bemcentinib (AXL receptor kinase inhibitor), montelukast (leukotriene receptor antagonist), ergotamine and mergocriptine (ergot alkaloids with adrenergic and dopaminergic receptor agonist activities). Bemcentinib shows in vitro activity against SARS-CoV-2 with 10-40 per cent protection at 50µM in Vero cells and an IC50 of 100nM and CC50 of 4.7µM in Huh cells. Indeed, it is already an investigational treatment for COVID-19 , with a phase 2 trial underway. Montelukast another interesting hit has been shown to produce a significant reduction in SARS-CoV-2 infection in elderly asthmatic patients and has a reported in vitro SARS-CoV-2 IC50 of 18.8 µM, and CC50 >20 µM.
SARS-CoV-2 RdRp inhibitors
Given the function of SARS-CoV-2 RdRp to bind RNA, its binding site is large. Most of the repurposed drugs in the list of 20 best binders we predicted had relatively large complex structures and substantial ligand flexibility. Antiviral drugs accounted for half of this list, the remaining compounds in being mostly natural products or their derivatives. These were of particular interest given their relative novelty and molecular diversity. Interestingly, our computational screen identified ivermectin as fitting snugly into the RdRp binding pocket. Ivermectin and other avermectins and milbemycins are broad-spectrum antiparasitic macrocyclic lactones that have shown inconsistent activity against SARS-CoV-2. Ivermectin has been widely touted as a drug against COVID-19, although the debate around its use has become highly politicized. It is interesting, therefore, that our unbiased screening methods identified Ivermectin as one of its top hits. Digoxin is a widely used cardiac drug used to treat heart arrythmias and cardiac failure and has a large complex structure that fitted well into the RdRp binding pocket. It exhibited potent in vitro antiviral effects against SARS-CoV-2 in Vero cells (IC50 37 nM). Another tightly binding natural product, silibinin, is a flavonolignan that is the major active constituent of silymarin, a standardised extract of the milk thistle seeds. It can function as an antioxidant, antineoplastic drug, and hepatoprotectant. Rapamycin, a macrolide antifungal metabolite with immunosuppressant activity that also bound tightly to RdRp, is currently in COVID-19 clinical trials. Other highly ranked natural products from our in silico screens included: carbetocin, a synthetic analogue of oxytocin;eribulin, a fully synthetic macrocyclic ketone analogue of the marine natural product halichondrin B, a potent anti-mitotic anticancer agent; novobiocin, a DNA gyrase and bacterial type IIA topoisomerase inhibitor; and
ergotamine and related ergot alkaloids also predicted by us and others to bind several SARS-CoV-2 molecular targets, including the main protease, Mpro.
SARS-CoV-2 Helicase inhibitors
The twenty molecules with the best predicted helicase binding affinity can be broadly characterized as containing one or more hydrophobic aromatic moieties linked to another polycyclic moiety containing hydrogen bond donors or acceptors. These molecules come from diverse drug classes, with antiviral agents making up 25 per cent of the hits and antihistamines and antipsychotics also being well represented. The most interesting non-antiviral agents identified by the study were hesperidin(citrus flavanone glycoside), rutin (flavonol glycoside), conivaptan (vasopressin inhibitor), aprepitant (NK1 antagonist), manidipine (Ca channel blocker, anti-hypertensive), aminoquinuride (trypanocidal agent), antrafenine (analgesic anti-inflammatory), epirubicin (anticancer intercalator), dicoumarol (anticoagulant), fluspirilene (antipsychotic), fexofenadine (antihistamine), astemizole (antihistamine), sertindole and pimozide (antipsychotics). Of these manidipine exhibits broad-spectrum antiviral activity, with SARS-CoV-2 IC50=2µM in HUH7 cells and 7.5µM in Vero cells. Tipranavir also exhibits a relatively broad spectrum of antiviral activity, inhibiting replication of SARS-CoV-2 in VeroE6 cells. Fluspirilene activity against SARSCoV-2 was reported to be 3.1µM in Vero E6 cells, mirroring the activity of fluspirilene against MERS-CoV and SARS-CoV in Vero E6 cells of 7.5µM and 6.0µM, respectively. Astemizole had an EC50 of 1µM against SARSCoV-2 in Vero E6 cells and 4.9µM and 5.5µM against MERS-CoV and SARS respectively.
Conclusions
Computational screening methods promise fast and reliable identification from libraries of existing drugs, clinical trials candidates, and approved natural products of compounds that may have useful activity against SARS-CoV-2. The three studies summarised here show that docking methods are capable of reliably predicting the binding pose of drugs to structures of virus target proteins. Complementary MD simulations performed on the repurposing candidates with the highest docking scores provide more reliable relative binding affinities of these drugs for the targets, allowing the docking hits to be ranked for activity. This is a very rapid and efficient way to screen for potential anti-viral drug activities.
The fact that >30 per cent of the top 80-100 repurposing candidates we identified have experimental activity against the target and/or the virus, with several already in clinical trials for treating COVID-19 shows the computational predictions were highly reliable. Therefore, the 70 per cent of repurposing candidates identified by these studies that have not yet been assessed for in vitro or in vivo activity could provide a rich resource of potentially useful drugs for treating COVID-19. As these are generally applicable, platform methods, they are equally suitable for identifying repurposed drugs to treat neglected tropical diseases, or viral infections and future pandemics.[5]We are seeing the beginning of an era where experimental high throughput drug screens will complement structural biology-based and machine learning methods to provide faster, more efficient, cheaper, and more robust methods for repurposing existing drugs or discovering new ones. References are available at www.pharmafocusasia.com
AUTHOR BIO
Nikolai Petrovsky is Professor of Medicine at Flinders University, Director of Endocrinology at Flinders Medical Centre and Research Director of Vaxine, an Adelaide-based biotechnology company focused on vaccine development. He has been awarded over 50 million dollars in vaccine grants including from the US National Institutes of Health. In addition to authoring over 200 peer-reviewed research papers he is an inventor on multiple vaccine patents, and most recently developed the Covax-19/SpikoGen® vaccine against COVID-19 that in October 2021 received emergency use authorization in Iran, making it the first recombinant protein COVID-19 vaccine in the world to receive approval, and the first successful human vaccine developed in Australia in the last 40 years.
David A Winkler is Professor of Biochemistry &Chemistry at La Trobe University. He applies computational chemistry, AI, and machine learning to design drugs, agrochemicals, nanomaterials, and biomaterials. He is ranked 227th of 81,000 medicinal chemists worldwide and has written >250 journal articles and book chapters and his H index is 54.
Sakshi Piplani is a Senior Bioinformatics Scientist skilled in Structural Bioinformatics, Data Analytics, Machine learning. She uses computational modelling of immuogenic receptors, discovery of potential new drugs/ lead compounds through machine learning and virtual screening. She also uses computers to simulate protein structures, and identify their functions.
ANTI-FUNGAL RESISTANCE
Magnitude of the problem and impact
Anti-fungal resistance is increasing, with the global spread of the multi-drug resistant Candida auris since 2008, increasing rates (up to 95 per cent) of azole resistance in Aspergillus fumigatus and the emergence of a new species of Trichophyton in India which is terbinafine resistant. Accurate and rapid diagnosis of most fungal infections is now possible with nonculture based tests, allowing tailoring of both antibacterial and anti-fungal therapy, but culture and susceptibility testing are required to detect resistance. New anti-fungal agents are being developed, but not fast enough to address this problem without minimising empirical anti-fungal therapy in patients and reduction in azole fungicides in crops.
L Shamithra M Sigera, Manchester Fungal Infection Group, Core Technology Facility, Grafton Street, University of Manchester; Department of Mycology, Medical Research Institute
David W Denning, Manchester Fungal Infection Group, Core Technology Facility, Grafton Street, University of Manchester; Global Action for Fungal Infections
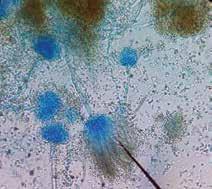
Microscopic view of Aspergillus terreus
Fungal disease of humans, animals, and plants
Fungi are ubiquitous in nature and cause disease in humans, animals, and, especially, plants. The range of human fungal infection varies from superficial infections (pityriasis, athlete’s foot, ringworm, nappy rash, and many others), allergic disease (chronic sinusitis and fungal asthma), chronic infections (chronic pulmonary aspergillosis and the NTD mycetoma as examples) to severe life-threatening invasive infections (cryptococcal meningitis, invasive aspergillosis, candidaemia, mucormycosis, etc). Some of these are recurrent infections (vaginal candidiasis) and some fungal infections are contagious. Globally, over 300 million people are threatened by serious fungal infections. Invasive fungal infections are associated with high mortality even with effective anti-fungal management: Cryptococcal meningitis; >50 per cent in the developing world and 15-20 per cent in the USA, invasive aspergillosis;~30 per cent mortality in leukaemia and >90 per cent in liver failure, invasive candidiasis; ~40 per cent mortality even with treatment [GAFFI].
Fungal infections go beyond humans — invading plants, insects, and animals. Fungi infect and destroy food crops reducing food production. It is estimated that one-third of all food crops are destroyed by fungi annually (Fisher et al., 2012). They have a high impact on global nutrition and the economy by affecting the most widely consumed crops such as rice, wheat, maize, potatoes, and soybeans. The list includes most high-value crops (coffee, bananas, cacao, mangos, and spices). Moreover, food becomes non-edible when fungi produce mycotoxins, commonly in if poorly stored. Fungi are major pathogens of trees, such as chestnut blight, ash dieback, and many others, impacting attempts to ameliorate global warming and incurring major economic loss. More species extinctions are attributable to fungi than any other infectious group, notably many frog species in recent years. Fungal disease leads to decreased biodiversity and indirectly contributes to global warming.
Anti-fungals and resistance
Anti-fungals are used to prevent and treat fungal infections in humans, animals, and plants. Moreover, they play an important role in material preservation and food safety [Verweij, P.E., et al. 2020]. Different classes of anti-fungals act on different targets, inhibiting fungal growth or killing them. A limited number of
anti-fungals are currently available for use (Table 1) and new anti-fungals come onto the market at a snail’s pace. Fungi have large genomes and are very adaptable. Both intrinsic (primary) resistance and secondary (acquired) resistance are well-known. Multiple strategies have been described in different fungi to resist the effect of anti-fungals, including modification of drug-acting sites or voiding the drug out of the fungal cell by efflux as 2 common examples. Many researchers continue to identify new mechanisms of anti-fungal resistance, and in many fungi, several mechanisms coexist in individual cells. Resistance in fungi is both increasing in incidence and increasingly detected. Certain resistant fungi are already widespread across the globe [Denning, D.W., 2022].
Drug resistance fungi
Anti-fungal resistance might be either intrinsic or acquired. Naturally, some fungi are not killed by certain anti-fungals, and they are called intrinsically resistant to that anti-fungal. The second group is usually susceptible but alters their susceptibility over time with the presence of anti-fungals and becomes non-responders; Is called acquired resistance. Most of the antifungal resistance is acquired [Denning, D.W., 2022].Interestingly,
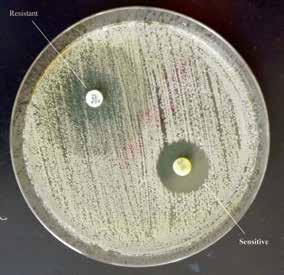
An antifungal susceptibility plate of Candida species. The strain is susceptible (S) to amphotericin B (AP, seen at 5:00 with a large zone of inhibition) compared to fluconazole (FCA, seen at 11: 00 with a smaller zone of inhibition).
ANTI-FUNGAL ROUTE PRIMARY INDICATIONS
Fluconazole
Itraconazole
Voriconazole Oral, IV Treatment for vaginal candidiasis Oropharyngeal and esophageal candidiasis (mucosal candidiasis), Treatment and maintenance therapy for cryptococcosis Prophylaxis in leukaemia, HSCT, and intensive care
Oral, IV* Treatment for vaginal candidiasis Oropharyngeal and esophageal candidiasis (mucosal candidiasis. Fungal skin and nail infections All forms of aspergillosis and endemic fungal infections Prophylaxis in leukaemia
Oral, IV Invasive and chronic aspergillosis, some rare moulds
Posaconazole
Griseofulvin Oral, IV Prophylaxis of fungal infections in haemat opoietic stem cell transplant (HSCT) recipients and acute leukaemia Refractory oropharyngeal and oesophageal candidiasis Salvage therapy for the treatment of invasive and chronic aspergillosis and mucormycosis
Oral Tinea corporis and capitis
Terbinafine Oral Tinea corporis and capitis Onychomycosis (fungal nail infection) due to dermatophytes
Amphotericin B IV and topical Invasive candidiasis and Induction therapy of cryptococcal meningitis, endemic fungal infections Empiric therapy in febrile neutropenia Mucormycosis Second-line for invasive aspergillosis
Flucytosine Oral, IV Cryptococcal meningitis induction therapy, Neonatal candidiasis, Candida endocarditis, and endophthalmitis, other rare refractory fungal infections
Echinocandins (micafungin, caspofungin, anidulafungin) IV Invasive candidiasis and candidaemia Second-line invasive and chronic pulmonary aspergillosis Empirical therapy in febrile neutropenia Prophylaxis in acute lymphoblastic leukaemia
NO. RESISTANT FUNGI COMMENT
01 Candida auris Multidrug resistance /pan anti-fungal resistant Identified since 2008 in all continents of the world. Can persist in the environment irrespective of common cleaning and disinfection methods. Causes severe fungal infections including bloodstream infections A cause of hospital-acquired infections and difficult to control outbreaks. Outbreaks have been reported in India, Italy, Florida, and California
02 Candida tropicalis Resistant to the azoles have been reported Present on the skin, gut, genitourinary and respiratory tracts of humans. Harmless in healthy people Causes oral candidiasis, VVC, blood, and deep-seated organsin people with a suppressed immune system Very strong biofilm producer
03 Candida parapsilosis
Resistance to the azoles and echinocandins reported (China, South Africa) Present on the skin as a commensal, hospitals spread disease through the hands of health staff Causes blood and deep-seated organs and infections associated with prosthetic devices and indwelling catheters This species can form biofilm
04 Candida glabrata Presents in the GI tract, mouth, and genital area. Usually resistant to azole anti-fungal agents and resistance develops while on treatment; occasional echinocandin resistance A common problem in recurrent vaginal candidia sis Systemic Candida infections including bloodstream infections Systemic infections are often nosocomial, as fluconazole breakthrough
05 Aspergillus fumigates
Common mould and spores in the air are inhaled regularly Azole resistance driven by azole fungicide use in crops, increasing. Causes a wide range of infections (chronic lung disease, invasive aspergillosis, allergic disease). A major concern in transplantation, leukaemia, severe influenza and Covid-19, and high doses of corticosteroid.
06 Mucorales Common environmental fungi occurring on decaying organic matter Resistant to most anti-fungals, except amphotericin B, posaconazole and isavuconazole Uncontrolled diabetes and corticosteroids are major risks. Acute severe infections often of the nose, sinuses and penetrate the brain, and lungs.
07 Fusarium spp. Fungi occur on decaying organic matter and water supply Resistant to most anti-fungals. Causes nail infections, kerat,itis and bloodstream infection (in leukaemia). Important plant pathogen, causing root rot and stem canker
08 Scedosporium spp Fungi occur on decaying organic matter (sewage, contaminated water, and urban soil) Some species are highly resistant to most anti-fungal agents It has a neurotropic nature Causes subcutaneous infection infections, asymptomatic pulmonary colonization in cystic fibrosis patients, and severe invasive infections such as brain abscess and infective endocarditis. It is particularly involved in the near-drowning syndrome
09 Trichophyton indot ineae
Human skin - adapted fungus, newly described in 2020 in India Highly resistant to terbinafine and less susceptible to itraconazole Contaminates the environment and causing infection in household and other close contacts Common in India and now seen across the world Causes extensive ringworm, with strong inflammatory component Table 2: Common fungal species with increasingly documented anti-fungal resistance
some of these fungi exhibit resistance to multiple anti-fungals, and some of them are resistant to almost all the available anti-fungals or to a complete class, such as pan-azole resistance. Table 2 shows the most frequently observed resistant fungi, and Table 3 to which anti-fungal fungus is resistant to.
How anti-fungal resistance develops
Misuse and overuse of anti-fungals, inadequate infection control in the healthcare sector, poor medical facilities, and lack of awareness are blamed for the emergence and spreading of anti-fungal resistance.
Expansion of preventive and therapeutic use of anti-fungals contributes to the growth of anti-fungal resistance. Repeated drug exposure in the form of prophylaxis or long-term therapy is often associated with the emergence of resistance. When fungi are exposed to suboptimal concentrations, drug-resistant fungal strains are