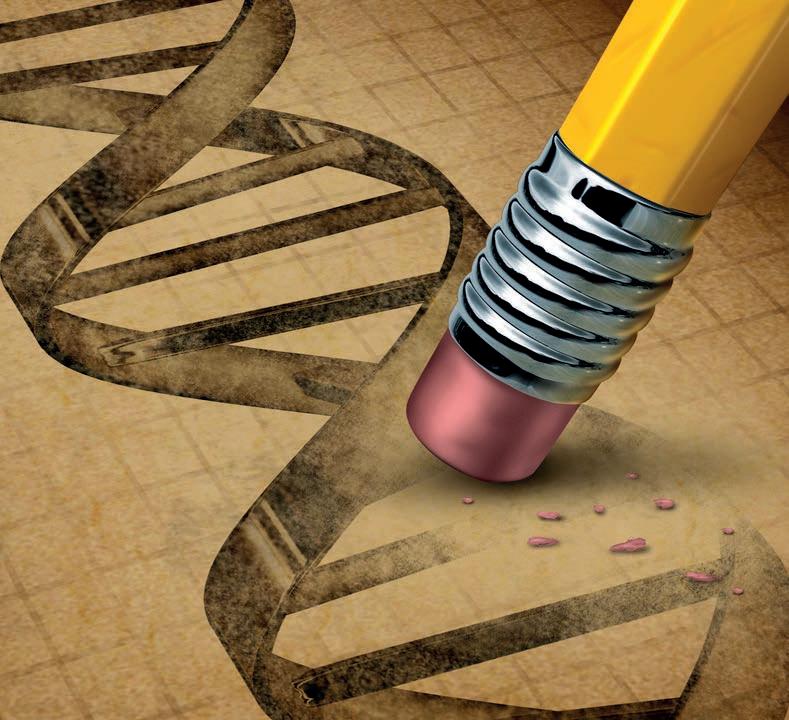
13 minute read
Genomics and biotechnology in poultry breeding
Genomics in poultry breeding
Since the release of the chicken and turkey DNA sequences in 2004 and 2010, respectively, poultry breeders have access to genomic information to increase accuracy of selection. The term genomic selection refers to the use of the naturally occurring DNA variation in the genome to predict breeding values. Genomic selection is routinely used in broiler, turkey and layer commercial breeding programmes. Aviagen incorporated genomic selection in broiler and turkey breeding programmes in 2013 and 2017, respectively. The company predicts that genomic selection will contribute with an increase in selection accuracy ranging from 5% to 50% over conventional selection depending on the trait. For turkeys, the impact of genomic selection means an additional rate of genetic gain of about +20grs of live weight and -0.01 FCR per year.
The use of DNA for predicting breeding values constitutes a recent breakthrough that allows combining traditional sources of information (e.g. pedigrees and phenotypic records) with the naturally occurring variation in the genome. It is key to emphasise that this process does not involve manipulating or artificially altering the genomic information in any way, but rather enhances the selective breeding process.
The following sections focus on techniques which are not currently used in commercial breeding programmes. These techniques, collectively called New Breeding Technologies (NBT’s), rely on biotechnology applications to achieve specific novel changes in the genome.
Aviagen will focus on a specific NBT technique called Gene Editing (GE) providing recent examples of its potential application in plants, livestock and poultry breeding and human medicine and discuss the current regulatory framework.
Biotechnology in agriculture and the emergence of new breeding techniques
In 1973 Boyer and Cohen create the first genetically engineered organism – a bacteria with an added gene to confer antibiotic resistance. This began the pursuit of modifying organisms for the improvement of species and their modification for research purposes. In 1982 the United States Food and Drug Administration approved synthetic insulin produced from genetically engineered bacteria for use in humans. In 1994 the California based company Calgene (purchased by Monsanto in 1997) introduced the first genetically engineered food, the Flavr Savr tomato, which was engineered to stay firm when ripe. In 1996 the multinational Monsanto (now owned by Bayer), released its first genetically modified crops and within a few years, Roundup Ready corn, soybeans, cotton, sugar beets, and canola dominated the market.
Despite its recent focus, GE in its current form has been used since the 1990’s. The ability to make deletions in the genome has allowed researchers to adapt and/or manipulate functions within the cell for research and commercial purposes. As new technologies have been discovered and developed, the ease and the reduced cost of application have made GE available to universities and small and large biotechnology companies. The latest technology, called CRISPR-Cas9, is cheap, easy to apply and has been widely taken up as the technique of choice for GE.
In recent years, GE has captured enormous attention given its potential application in a wide range of fields ranging from plant and livestock breeding to human medicine. In contrast with Genomic Selection, GE involves altering the naturally occurring DNA configuration of the genome to add and or delete individual bases or DNA segments to achieve a specific biological response in the phenotype. NBT’s have brought about new fields of research allowing the examination of biological processes and the production of animals, plants and crops with enhanced commercial traits.
The CRISPR-Cas9 system is part of the naturally occurring adaptive immune response of bacteria. When a bacteria is exposed to an invading virus, the bacteria maintains its protection by keeping a small piece of viral DNA (guide DNA) in its own genome and uses this piece to patrol the cell looking for an identical copy. If it finds it, this is an indication of infection and it will cut that DNA to interfere with virus replication.
By providing the CRISPR-Cas9 protein with an artificial guide sequence, the protein can be directed to nearly any DNA sequence and produce a cut in that specific location in the genome. This cut in the genomic DNA can be repaired randomly by a normal DNA repair mechanism of the cell known as non-homologous end-joining (NHEJ). This repair can result in a perfect repair or the insertion and/or deletion of random amounts of DNA. To make very specific known changes in the genome, a piece of DNA can be included in the process that is used to repair the cut. This method, known as homology-directed repair (HDR), has the same results as NHEJ except the genomic DNA sequence is repaired exactly as is provided in the HDR DNA.
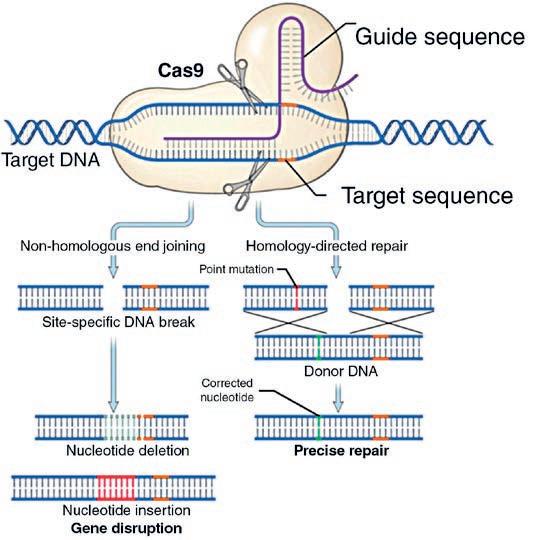
Figure 1
In Figure 1 CRISPR-Cas9 finds the target sequence by comparing to a guide sequence. In non-homologous end joining (NHEJ) the DNA is repaired randomly by the normal cell machinery, resulting in a perfect repair – which will be cut again – or imperfectly resulting in DNA changes, insertion and/or deletion of random DNA sequences. By including a piece of DNA that precisely repairs the target region, the homology directed repair (HDR) process can make specific changes to the target DNA.
The CRISPR-Cas9 technology has been used to delete or add small specific pieces of genomic DNA from and to the genome. Through changes of the enzyme, this technology is also able to mutate bases within the genome, making subtle changes that can increase or decrease the amount of protein produced within the cell. In a similar fashion, small, or larger, parts of proteins can be replaced with new sequences that change functions of that protein.
Applications in plants
There have been a wide range of GE applications in plants. Perhaps the most widely known is the production of a ‘waxy’ corn variety that has had a deletion made in its genome causing the composition of starch in the corn kernel to contain very high levels of Amylopectin allowing a much broader range of commercial uses. This gene variant was already present naturally, so CRISPR-Cas9 GE was used to increase its frequency in breeding populations. This new generation ‘waxy’ corn is due for commercial release in 2020. CRISPR-Cas9 has been used in rice, wheat, tomato, cucumber and other plant crops to increase their resistance to disease gene deletions. Improvements in the storage and longevity of harvested produce have been seen in apples and mushrooms where some of the enzymes that cause browning have been removed. These apples and mushrooms will still brown but at a slower rate, helping counter the waste in food supply when food spoils. Similarly, in soybeans, a genome deletion results in improved shelf life and heat stability of soybean oil. In the paper production business, GE has been used in eucalyptus to produce a tree that can survive freezing temperatures, opening up new geographic regions for tree growth while at the same time introducing sexual sterility to achieve genetic containment and avoid genetic migration into non-GE varieties.
Applications in animals
While more difficult to implement in animals than in plants, animals have had numerous successes through GE in recent years. Two genome edits resulting in disease resistance have been achieved in pigs. In the first case, a porcine reproductive and respiratory syndrome virus (PRRSV) resistant pig was produced by deleting part of the virus receptor, the protein used by the virus to enter the cell. The second case involves exchange of the receptor protein between two closely related porcine species. Domestic pigs are susceptible to African Swine Fever Virus (ASFV) while warthogs are resistant. By moving the warthog gene to the domestic pig genome, researchers have made an ASFV immune pig.
Also in pigs, researchers have repaired a gene that is non-functional in pigs but functional in other mammals. The gene is involved in energy usage and when repaired, the edited pigs were able to maintain their body temperature thereby reducing the need to provide strict temperature-controlled environments. The repaired protein is able to breakdown fat to provide the energy needed by the pig and the modified pigs had 24% less body fat than non-modified pen mates.
In dairy cattle, GE was used to produce hornless calves, by bringing the polled mutation from a beef cattle population in which it is naturally present, into the same location in the genome of a Holstein dairy cow. The polled mutation is found in some cattle that prevents the growth of horns. This edit brought a lot of media attention due to its potential practical application in eliminating manual de-horning hence its potential welfare benefits as well as safer herd management by farmers. While the introgression of the polled mutation into the Holstein elite dairy cattle could take place through natural breeding, the loss of superior dairy-related traits would require many generations of back-crossing to return the Holstein dairy line back to be a high performing dairy cow. Similar to the waxy corn example, GE was used to introduce a naturally occurring variation from one breed type to another.
In chickens, researchers have used CRISPR-Cas9 to generate cells resistant to Avian Leukosis subgroup J (ALV-J) by editing the virus cell surface receptor. Only one amino-acid change in the protein structure confers resistance. This work was done in cells and there is no evidence so far that the same effects would be seen in live birds. Work on Avian Influenza resistance using GE is progressing for a number of candidate regions in the chicken genome reported to have an impact on virus replication. So far, all the work is exploratory, and no evidence is available that those edits would confer resistance or resilience in birds either experimentally or under a field challenge. Clearly, the greatest potential for GE in poultry is for disease resistance.
In an effort towards producing safer egg derived food products researchers have deleted two of the major allergens from chicken eggs. While not removing all allergens, these eggs may allow people with egg allergies to eat cooked egg products. Another potential application is the editing of the chicken genome for vaccine manufacture. Human influenza vaccines are produced using embryonated eggs and researchers have targeted the deletion of genes in the chicken genome which restrict virus growth to produce eggs that give higher yields of virus for vaccine production. The ability to produce eggs with high vaccine yield could have implications for the whole vaccine production chain (reducing the number of eggs needed per dose and reducing transport cost and waste).
The use of chickens as bio-reactors for protein-based medication has also been a focus of attention. Recently, researchers used CRISPR-Cas9 to edit chickens to produce human proteins in their eggs. Researchers have focused on two proteins with therapeutic potential, Interferon Alpha 2a which has powerful antiviral and anti-cancer effects and the macrophage CSF which is being developed as a therapy that stimulates damaged tissues to repair themselves.
Applications in humans
GE of human reproductive tissue is prohibited in many countries and strongly restricted in others. In other cell types, GE technologies have already reached clinical trials. The pace at which GE enters human clinical trials is bound to accelerate in coming years as successful pre-clinical studies move into clinical trials for genetic diseases that are difficult to manage or even incurable. In a research environment, genome editing has been used to correct defects in immune cells in humans. People with rare genetic defects in their immune system can have cells removed, repaired with genome editing and replaced back in the patient.
The use of GE to modify the patient’s immune system cells to specifically target cancerous cells is a reality in the clinical treatment of cancer. The use of modified immune T cells to treat children with B-cell acute lymphoblastic leukaemia has been granted an EU license and the treatment is available in England through the National Health Service (NHS). This treatment is now being expanded to adults with lymphoma. This constitutes a breakthrough in the treatment of cancer and it is expected that similar applications using GE will become increasingly available.
In contrast to the cell therapies, late in 2018, a researcher from China announced production of the first GE human twins. The twins had been modified to be immune to HIV. This work has raised a number of ethical concerns and there is wide international discussion amongst researchers and regulators about assessing the evolving scientific landscape, possible clinical applications, and attendant societal reactions to human GE.
The widely reported successes of GE applications in humans for clinical use and the current ethical debate of certain applications undoubtedly puts GE under the public opinion light. This will no doubt allow the general public to be more aware of the technique and its potential, but it is not clear whether its acceptability for food production will follow the same direction of its acceptability as a therapeutic tool.
Regulatory framework
Regulation on GE plants, animals and organisms is far from universal and far from finalized. In some areas, regulatory bodies have proposed to continue to regulate GE plants in the same way as Genetically Modified Organisms (GMO). However, GE has raised the question about what qualifies as a GMO and whether distinction should be made between changes within a species, putting naturally occurring DNA segments in different locations of the genome, and modifying a genome with elements from other species? Further, how should a NBT not classified as a GMO be regulated? The United States Department of Agriculture (USDA) has recently suggested criteria for excluding a GE from the GMO regulatory process. In short, changes that could happen during the normal reproduction process should not be regulated as a GMO.
A GE product that the USDA does not regulate will still require Environmental Protection Agency (EPA) regulation to ensure that the product is not a plant pest and Food and Drug Administration (FDA) regulation to ensure the food is safe to eat – if the product is planned for consumption. Some South American countries have adopted a similar logic with a case-by-case regulatory framework for NBT-derived products. This allows for informed regulation of a product rather than relying solely on the method used to create the new product. In Europe, the European Court of Justice (ECJ) has recently issued the judgment that organisms obtained using NBT’s of directed mutagenesis (including GE) are GMOs and subject to the obligations of the GMO Directive legislation. This could potentially have profound effects on the future research and commercial application of GE in Europe. There is an active dialogue across stakeholders and the general public including calls for a revision of the GMO Directive to reflect current knowledge and scientific evidence and assess if GE should be covered under the GMO legislation.
The regulation of NBT-derived animals is less clear as regulators have produced less conclusive documentation regarding animals. In the USA, the FDA has proposed to regulate all animals whose genomes have been “intentionally altered” as GMO’s and put them through the “New Animal Drug” regulatory process. Under this regulation, each modification undertaken in an animal would be regulated as a separate new animal drug and would require the expensive and onerous process of going through the Federal Food, Drug, and Cosmetic (FD&C) Act for commercialization. It doesn't help the regulatory process in the USA where agencies are still arguing over who should regulate NBT-derived products.
Final remarks
GE is a technique that is currently readily available to researchers and examples of its potential application in plants and animal breeding, and human medicine is growing exponentially.
In humans GE based therapies are already available and in plant breeding there are already varieties getting close to commercialization. On the other hand, in livestock GE has not yet gone beyond the proof of concept stages and it is not clear whether this technique will crystalize as a breeding technique in plants and animals.
The regulatory framework is still evolving, and public acceptability and product safety of the products derived from GE (and NTBs in general) must be carefully considered before evaluating the use of this technique in commercial animal breeding programmes.
For the foreseeable future poultry breeders will use traditional breeding technologies and techniques including genomics selection to exploit the naturally occurring variation in the DNA as an additional tool to increase selection accuracy.
Modern breeding goals are balanced and include traits related to a range of biological functions (e.g., growth, yield feed efficiency, reproductive fitness, environmental adaptability and welfare) so while a specific change in the DNA could lead to a beneficial change in one trait, it is critical to consider its impact on other traits.
References are available on request
From the Proceedings of the 13 th Turkey Science and Production Conference
S. Avendano and S. Tyack - Aviagen Ltd, Newbridge, Edinburgh, UK