Biodesign Portfolio
Carlos Balaguer García Spring Semester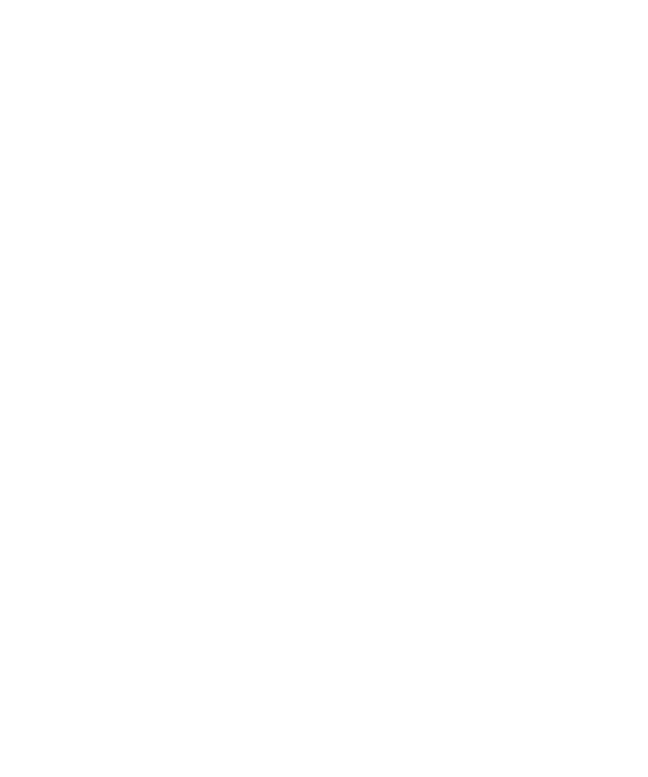
Biodesign: definition and entanglements _ Page 1
Algae futures _ Page 4
Designing with algae _ Page 8
The bioeconomy _ Page 11
Designing with living organisms _ Page 16
Glossary _ Page 19
References _ Page 21
Annex: Field research _ Page 23
The term biodesign commonly to the subfield of design studies that comprises the design “of, with or from biology” (Ginsberg & Chieza, 2018). Paradoxically, this seemingly precise definition also frames biodesign as an umbrella term for all disciplines that develop solutions within the overlaps of biology, art, and technology, such as biotechnology, synthetic biology, biomedical engineering, etc., as they “integrate organic processes and materials into the creation of (…) products” by “incorporating or imitating living organisms into design as material sources” (Omuus, 2018)
Fundamentally, biodesign envisions a historical change of paradigm in our relation as a species with the fabrication of products and goods to meet material demand (Benjamin, 2011), thus embodying a transition from industrial manufacturing techniques towards a horizon of biofabrication, with profound systemic implications in the world economy.
With every radical change of technological paradigm come new challenges and uncertainties and, in context of biodesign, one of the major obstacles to overcome is the current lack of sufficient
interconnection between those disciplines focused on experimental biology research and the design practice as a whole.
A convergence of both fields is, however, necessary for the emergence of these transformative new hybrid disciplines between biology and design, and that requires not only an alignment of objectives and strategies, but also the construction of a common framework of methodologies that enable for joint projects both scientifically backed advancements in understanding
biological processes for metabolism, molecular synthesis and techniques like gene editing, and provided with the scope of implementation and communication that the more explorative and speculative practices of design bring to the table (Chieza et al., 2019)
The idea of understanding biology (or traditionally, nature), design and technology as a continuum is, however, not new: “Descartes was the first who […] ventured to think of the animal as a machine” (Nietzsche, 1918, as cited in Lemm, 2020, p. 87). Even earlier, circa 1510, Da Vinci’s studies of human anatomy show a mechanistic interest in the natural sciences a source of inspiration and understanding for design but, also, as a subject of design.
We also find this dichotomy present in Binswanger interpretation of Freud’s conception of human nature, that comprises “first, a rigorous-scientific deconstruction of human nature, and second, a creative-interpretativeinterpretative reconstruction of human nature […] that belongs to a larger project of cultural renewal” (Binswanger, 1947, as cited in Lemm, 2020, p. 81).
In a more contemporary approach, posthumanist philosopher Rossi Braidotti defends the idea that “the possibility of a community between human and nonhuman life is ultimately based on […] ‘the ubiquity of technological mediation’” (Braidotti, 2016, as cited in Lemm, 2020, p. 171). Altering her notion of “technology as second nature” (ibid.), we find biodesign as the result of a radically transformative consideration of nature as second technology.
It is precisely within this philosophical framework1 that the topic of biodesign reaches its uttermost relevance, and perhaps even urgence, to provide substantial transformation of the way we, as a planetary species, think of our relationship with the very materials we assume as our technology, as our means for further survival, transformation, and evolution.
As the conception of material reality evolves from a structuralist viewpoint of the object as contained entity towards a broader poststructuralist understanding, in which the object becomes a series of entangled material, energetic, ecosystemic and ontological
"Design can be a mediator of the scientific and technological advances, without putting them to the service of nature exploitation and consumerism stimulation."
(Acosta & Romeva, 2010)
relationships (i. e., a “hyperobject” (Morton, 2013)), it becomes more obvious that there is an inseparable agency in the way we choose to materialize our technology.
This means that the way in which we think and speculate about our objects is, more than ever, able to create an impact in larger scale systems. At the same time, these objects must embody this systemic complexity by integrating a conception of processuality (Tonuk & Fisher, 2020) that goes beyond manufacturing, and extends into material sourcing (non-extractivism (Acosta, 2017)), disposal (waste reduction), degradation (environmental impacts and carbon cycle analysis), social impact (decolonization, accessibility, security, food, water and energy supply), etc.
In the context of ecosystemic and climatic collapse, the idea that design means addressing these issues becomes crucial. If, according to the definition of Herbert Simon, “to design is to devise courses of action aimed at changing existing situations into preferred ones”, then design becomes an essential tool for humanity to steer away of the current trend, and into other possible, more promising futures.
Provided that the nature of the issue is, inherently, an organic one, this design process must be guided and informed at all times by the biological sciences (ecology, biotechnology, synthetic biology), which create the scaffolding for solid speculation and later implementation of design solutions.
The term ‘algae’ refers to a broad variety of photosynthetic eukaryotic organisms that inhabit both marine and freshwater aquatic ecosystems, within the photic zone.
This definition includes both macroalgae (multicellular plant-like organisms commonly referred to as seaweed) and microalgae (microscopic, typically unicellular organisms that comprise phytoplankton).
The standard categorization of algae establishes seven different divisions: chlorophyta (green algae), phaeophyta (brown algae), rhodophyta (red algae), pyrrophyta (dinoflagelates), chrysophita (diatoms), euglenophyta (euglenoids) and glaucophyte (glaucophytes).
Given their ability to perform photosynthesis, analogous to that of algae, cyanobacteria are often also included in the taxonomy of algae as ‘green-blue algae’ (Schoch et al., 2020), despite being prokaryotic organisms.
The vast variety of species that fall under the common categorization as algae allows for a similarly ample range of products and applications, including medical products, cosmetics, pigments, energy generation, environmental remediation, etc. (Sharma et al., 2016).
Currently, macroalgae are cultivated all throughout the world for human consumption, as they constitute an integral part of many cuisines, but the consumption and farming of seaweed is dominated by countries in East Asia: “The major seaweed producing nations are China (47.9%) and Indonesia (38.7%) followed by the Philippines (4.7%) and the Republic of Korea (4.5%)” (Food and Agriculture Organization, 2018, cited in Kelly, 2020).
Some of the most notable species of edible algae are:
• Red algae: Nori (Porphyra and Pyropia, mainly P. yezonesis and P. tenera), agar-agar (extracted from Gracilaria and Gelidiaceae), dasima (Saccharina japonica) and guso (Euheuma).
• Green algae: Sea grapes (Caulerpa lentillifera), gutweed (Ulva intestinalis), sea letucce (Ulva spp.) and Chlorella spp., these last being a genus of microalgae commonly used as a supplement.
• Brown algae: Kombu (Laminariaceae), wakame (Undaria pinnatifida), cochayuyo (Durvillaea antarctica) and the Sargassum spp.
• Cyanobacteria: Some species of
the genus Arthrospira, most notably Arthrospira spirulina, also used as a health supplement.
In addition to their traditional consumption, similar to that of conventional vegetables, certain species of algae are cultivated so they can be refined through a series of chemical processes into polysaccharides, namely carrageenan (mainly red algae) and alginate, extracted from brown seaweed (Kelly, 2020), both used as gelling/stabilizing agents in the food, biotechnology, and pharmaceutical industries.
The use of seaweed as a fertilizer is also widespread and well established, with documents of its use as early as the 2nd century, where it was used also as protopackaging and livestock feed (Cotas & Pereira, 2019), and scientific evidence of its positive effects in crops dating back to 1965 (Aitken & Senn, 1965). Seaweed fertilizer is currently available in various formats, from seaweed meal to liquid. More recent research has further explored its capacity to improve the stress tolerance of crops and the quality of the soils not only by being a rich nutrient source, but also as a combination of its effects as a soil conditioner, its osmoprotective qualities, the interaction of the phytohormones present in seaweed with the cellular activity of crops, its capacity for bioremediation of polluted soils, and its positive impact in the microbial composition of the soil (Raghunandan et al., 2019).
Some of the other emerging applications of seaweed, due to their early stages of experimentation, will be further discussed later in this document.
As cited before, the records of historical usage of seaweed for various applications in scattered locations around the globe attest that it is intrinsically a versatile resource that has aided for ages in the
Figure 3. Kunstformen der Natur (1904), a series of botanical drawings of seaweed by German naturalist Ernst Haeckel.development of techniques for survival (Cotas & Pereira, 2019). Furthermore, for the aforementioned civilizations, seaweed has constituted not only a part of their diet, shelter, clothing, fishing and, overall, material culture, but also a part of their rites, celebrations, and ceremonial activities (Thrustan et al., 2018).
Provided the presence of local varieties with different chemical and mechanical properties around the world, local traditional knowledge consequently becomes a crucial indicator of the potentiality of local species of seaweed for different fields of application (Manly Seaweed Forest Festival, 2021) and, as such, a significant amount of resources have to be invested in re-gaining and keeping alive these forgotten “seaweed cultures” in order to respond to the urgency that the environmental crisis adds to the development of these new bio-based solutions.
Its social significance consequently extends beyond mere cultural preservation, and its potentialities to resolve current global affairs as food insecurity (dietary uses, nutritional supplements, fertilizers, polluted soil remediation, livestock feed), freshwater supply and wastewater management (water filtering), energy supply (third generation biofuels), microplastic pollution (bioplastics), health (pharmaceutical applications), etc., are key to address the Sustainable Development Goals adopted by the member countries of the United Nations in 2015 (Sutherland et al., 2021 ).
Algae thus presents as a promising ingredient for mor sustainable alternative futures (Climate Change Cluster et al., 2021), whose industrial applications and consumer products are yet to be imagined.
Despite the proven potential of algae to become a key material source for transformation in the design field, its implementations as of today are still very limited and experimental.
Given that algae-based manufacturing materials are still in an early development phase and are (with very few exceptions) not commercially available, most of the work has been carried out by independent artists and designers that have explored the qualities of these future materials often with limited resources and using an intuitive, experimental approach.
However, these experiments are not only valid but extremely valuable, as they expand the palette of applications and material variations that the future industry will have as precedents when developing upscale production. In sum, they widen the horizon of future possibilities.
In this sense, the work of Jessie French is crucial to understand the state of the art in the world of algae design. Whether it’s large translucent sheets of biopolymers, bioplastic dishware, or algal compounds used as ceramic glazing, her work is based around material design using
seaweed as the primary element.
In her installations (Figure 6), Julia Lohmann, founder of the art and design platform Department of Seaweed, uses traditional craftmanship techniques to transform algae into penetrable structures, and explores the potential of kelp as a raw material, in a similar way that indigenous Australians created tools to transport water using the impermeable properties of kelp.
Similarly, Violaine Buet, also a part of the Department of Seaweed, employs weaving, cutting and threading as a way to create garments from leather-like dried seaweed.
Also in the field of seaweed as textiles, material designer Nienke Hoogvliet’s SEA ME rug (Figure 5) and collection uses seaweed yarn to create a variety of products aiming towards a lower environmental impact.
The work of Blond & Bieber, particularly the collection Algaemy, focuses on the use of microalgae for pigment extraction, showcasing the versatility of algae beyond their structural qualities, presenting as a promising substitute to more polluting mineral and synthetic dyes, with fashion and textile production being one of the world’s most freshwaterpolluting industries (European Parliament, 2020).
A radically different approach from manufacturing with seaweed materials is to create systems that acts as a support for the growth of microalgae and makes use of their metabolic activity.
In the case of EcoLogic Studio’s Air Bubble (Figure 7), an inflatable plastic structure provides a controlled environment for a series of microalgae bioreactors to purify the polluted urban air at a rate of 100 liters per minute by using the energy of footsteps to passively pump air into the filtering system.
SolarLeaf constitutes another attempt at integration of algae bioreactors in the frame of built environment, in this case to act as a bio-chemical solar energy generating façade, generating hot water and potentially even biofuel.
The project The Metabolic Museum by Kate Scardifield showcases an experimentation with algae in biobased composite materials for carbon sequestration, conceiving bricks as long term storage for CO2.
Figure 7. Air Bubble (2021) by EcoLogic Studio, using Chlorella spp. and motion activated pumps to purify urban air.A bioeconomy can be defined as “an economy where the basic building blocks for materials, chemicals and energy are derived from renewable biological resources” (Kautto & McCormick, 2013).
In a more tangible definition, the European Commission defines bioeconomy as the “production of renewable biological resources and the conversion of these resources and waste streams into value added products, such as food, feed, bio-based products and bioenergy” (European Commission, 2012).
Other sources offer a deeper insight on the scope of the term, indicating that there are three distinct but overlapping visions on bioeconomy:
“The bio-technology vision emphasizes the importance of bio-technology research and the application and commercialization of biotechnology in different sectors of the economy. The bioresource vision focuses on processing and upgrading of biological raw materials, as well as on the establishment of new value chains. Finally, the bio-ecology vision highlights sustainability and ecological processes that optimize the use of energy and nutrients, promote biodiversity, and avoid monocultures and soil degradation.” (Bugg et al., 2016)
Given the systemic implications of these definitions, it is safe to assume that it encompasses the activity of a wide range of industries, both established and emerging, that deal with the production, processing, distribution, and waste management of organic matter.
Firstly, the bioeconomy comprehends the growth of organisms for human applications, namely crops, livestock farming, aquaculture, silviculture, and potentially fungiculture, bacterial farming, and algaculture.
In this field, algae have been found to be of incalculable value. From its cultivation for human consumption, to its use as agricultural fertilizers (Raghunandan et al., 2019) and its symbiotic use in seafood production by aquaponics (Goddek et al., 2019), algae allow for more controlled nutrient cycles in the primary sector.
Some notable emerging uses of seaweed in this regard are the trials in supplementation of Asparagopsis spp. in livestock feed as a way to reduce methane emissions from the animals’ digestive system by over 80% (Roque et al., 2021), as well as the integration of seaweed biofilters in river deltas to prevent nutrient runoff from agricultural exploitations into marine habitats.
Secondly, the industries devoted to processing said raw materials into higher value products comprise the food and feed, agrichemical, bioenergy, biochemical, pharmaceutical, biomedical, cosmetic, manufacturing, and construction industries. Intimately related to these commercial products, the way in which they are made accessible to the customers (retail and packaging industries) are essential to support a transformation towards sustainable practices, by integrating new systems and product cycles that minimize waste while adapting to the specifications of these new bio-based products.
Lastly, the way industrial byproducts, household waste, biological residues and end-of-lifecycle waste are managed in relation to ecosystems involves the industries of wastewater treatment, landfill management, waste valorization, recycling, and environmental remediation.
Thanks to their ability to incorporate micronutrients, diatoms, a type of microalgae can be used for remedying heavy-metal contamination in water ecosystems (Marella et al., 2020).
Crucially, the way these material and energy outputs can be circled back into our structures of consumption creates a field of opportunity for bioeconomies to exploit low value waste and transform it into high value compounds using the metabolic capabilities of living organisms, that can be engineered for that specific scenario using techniques explored by synthetic biology.
Good example of this are HRAPs (High-Rate Algal Ponds), an emerging technology that uses green microalgae to remove pollutants from wastewater using cellular nutrient exchange triggered by photosynthesis, hence reducing the need for external non-renewable energy sources. The low value exceeding algal
biomass is then collected and converted (most commonly by anaerobic digestion) into higher value biofuel, generating a circular waste-to-energy revaluation (Craggs et al., 2010).
In sum, the bioeconomy builds upon green (agricultural), blue (marine and freshwater), white (industrial use of enzymes and microorganisms), red (health and pharmaceutical), and grey (waste management) biotechnology (Kautto & McCormick, 2013) to improve quality of life for humans while ensuring a sustainable management of the resources and ecosystems.
It seems clear, at this point in time, that biodesign is not but to design with and for the entanglement of life. That said, the implications of working with organic life are, at least in the frame of Western philosophy, of a different nature to those of using inorganic matter.
The origin of modern bioethics is marked by the synthesis of common morale and medical tradition by the National Commission for the Protection of Human Subjects of Biomedical and Behavioral Research in the so-called Belmont Report, that contained three principles of bioethics.
These three principles are respect for persons, referring to the subject’s autonomy and explicit consent in participation or non-participation, without external pressures, beneficence, implying that the maximization of benefits and the minimization of possible short and longterm risks, and justice, that alludes to the fair treatment of the subjects without discrimination.
Albeit ambitious, these definitions are specific to humans and can barely translate to our interactions with other organisms that possess a different degree of consciousness and conscience.
In this light, trans-species psychology offers a broader set of guidelines that can be employed when analyzing interspecies relationships, which should be approached from a perspective of horizontal parity rather than a vertical gradient of inequality (Bradshaw & Watkins, 2006).
Applied to biodesign, this code of ethics is defined as per the following principles (Biodesigned, 2021):
Unity: Refers to the need for social justice to be constructed as a medium and result of a universal interconnection under biology.
Interbeing: Promotes a hybridization of self and collective, human and nonhuman, species and environment, to further explore entanglement as a condition for informing sustainability.
Humility: Indicates both the need to embrace interdisciplinary and multicultural dialogue as a necessary tool for collaboration towards a more desired state of the art.
Safety: Considers the systemic implications of biodesign in the environment, advising caution and preservation of biosecurity. An example
of this is the exploration of synthetic DNA with twelve independently replicating nucleotides, instead of the common eight (Campos & Benner, 2018), to prevent potentially dangerous interactions between genetically modified organisms and naturally present organisms.
Equality: Highlights other forms of life as equal to humans from a moral standing, aiming to reduce exploitative practices and suffering that come from human supremacism.
This change of ontological perspective widely conditions our interaction with otherness (Schmeer, 2019) linking with a broad intent to decolonize thinking, that is, to end a mentality of understanding the other as a subject for domination and conquest, and, instead, as an interdependent being in the frame of ecosystemic interlocking.
From a design thinking perspective, decolonization also means to overcome a culturally imposed preconception of use scenarios based upon social contexts, and concepts like hygiene, privacy, and personal space, that vary from country to country, as to not enforce or prioritize one vision over another based on historical cultural dominance.
In our human context, decolonizing also means to revert the impact of past philosophical structures that were subordinate to geopolitical interests of domination, and to shift the focus into traditionally exploited communities by offering them means to intellectually and materially grow out of their current status of subalternity.
Biodesign has proven to be yet another tool that can be utilized towards the goal of decolonization, addressing the material
needs of these communities. In relation to this goal, EcoLogic Studio’s BioBombola provides a low-tech solution for an algae bioreactor that could be used by impoverished communities to meet their nutritional needs.
Synthetic biology, especially its crop applications, are often also in the spotlight when discussing a positive impact in the Global South as a source of food, nutrition and energy (Ginsberg & Chieza, 2018), given that these solutions are intrinsically off-grid, providing a chance to reduce dependency from neocolonial powers.
Another example is the application of synthetic biology to develop sustainable bio-based products like ColoriFix, that help mitigate the environmental impacts that industrialized regions in the Global South typically endure, creating a shift of paradigm that considers the environmental integrity of former colonies as a priority over the patterns of overconsumption of resources in developed economies.
It becomes clear that biodesign has a potential to deeply transform the way we use our resources and manage our ecosystems within these now bio-based economies, but also to be a mechanism for sociopolitical and cultural agency in the global scene.
“The rearing of aquatic animals or the cultivation of aquatic plants for food.” (Oxford Languages, 2022) Aquaponics
“A system of aquaculture in which the waste produced by farmed fish or other aquatic creatures supplies the nutrients for plants grown hydroponically, which in turn purify the water.” (Oxford Languages, 2022)
“A type of biodegradable plastic derived from biological substances rather than petroleum.” (Oxford Languages, 2022)
“A polymeric substance occurring in living organisms, e.g. a protein, cellulose, or DNA.” (Oxford Languages, 2022)
“The use of either naturally occurring or deliberately introduced microorganisms to consume and break down environmental pollutants, in order to clean a polluted site.” (Oxford Languages, 2022)
“The action or process of a state withdrawing from a former colony, leaving it independent.” (Oxford Languages, 2022) Metabolism
“The chemical processes that occur within a living organism in order to maintain life.” (Oxford Languages, 2022)
“Nutrient pollution is the process where too many nutrients, mainly nitrogen and phosphorus, are added to bodies of water and can act like fertilizer, causing excessive growth of algae. Nutrients can run off of land in urban areas where lawn and garden fertilizers are used.” (National Ocean Service, 2021 )
“The mechanism by which the cells of an organism protect themselves against osmotic stress.” (Wikitionary, 2019)
“Surface layer of the ocean that receives sunlight. The uppermost 80 m (260 feet) or more of the ocean, which is sufficiently
illuminated to permit photosynthesis by phytoplankton and plants, is called the euphotic zone. Sunlight insufficient for photosynthesis illuminates the disphotic zone, which extends from the base of the euphotic zone to about 200 m. The thicknesses of the photic and euphotic zones vary with the intensity of sunlight as a function of season and latitude and with the degree of water turbidity. The bottommost, or aphotic, zone is the region of perpetual darkness that lies beneath the photic zone and includes most of the ocean waters.” (Britannica, 1998)
“Plant hormones (phytohormones) are chemicals produced by plants that regulate their growth, development, reproductive processes, longevity, and even death. These small molecules are derived from secondary metabolism and are responsible for the adaptation of plants to environmental stimuli.” (Yamaguchi et al., 2010)
“Relating to or involving the study of processes rather than discrete events.” (Oxford Languages, 2022)
“Synthetic biology is a field of science that involves redesigning organisms for useful purposes by engineering them to have new abilities. Synthetic biology researchers and companies around the world are harnessing the power of nature to solve problems in medicine, manufacturing and agriculture.” (National Human Genome Research Institute, 2019)
“Trans-species psychology is the field of psychology that states that humans and nonhuman animals share commonalities in cognition (thinking) and emotions (feelings). It was established by Gay A. Bradshaw, American ecologist and psychologist.” (Wikipedia, 2022)
“The term ‘waste valorization’ refers to any industrial processing activities aimed at reusing, recycling, or composting from wastes, useful products, or sources of energy.” (Kabongo, 2013)
Acosta, A. (2017). Post-Extractivism: From Discourse to Practice—Reflections for Action. Alternative Pathways to Sustainable Development, p. 77-101. OpenEdition Journals. https://doi. org/10.4000/poldev.2356
Acosta, G., Romeva, C., (2010). From Anthropocentric Design to Ecospheric Design: Questioning Design Epicentre. Design Theory and Research Methodology, p. 29-38. The Design Society.
Aitken, J. B., & Senn, T. L. (1965). Seaweed Products as a Fertilizer and Soil Conditioner for Horticultural Crops. Botanica Marina, 8(1). doi:10.1515/ botm.1965.8.1.144
Bugge, M., Hansen, T., & Klitkou, A. (2016). What Is the Bioeconomy? A Review of the Literature. Sustainability, 8(7), 691. MDPI AG. Retrieved from http://dx.doi. org/10.3390/su8070691
Bradshaw, G. A., & Watkins, M. (2006). Trans-species psychology: Theory and praxis. Spring: A Journal of Archetype and Culture, 75(1), 1-26.
Britannica, T. Editors of Encyclopaedia (1998). photic zone. Encyclopedia Britannica. https://www.britannica.com/ science/photic-zone
Campos, L., & Steven Benner. (2018). Other Genetic Alphabets. Journal of Design and Science. Retrieved from https://jods.mitpress.mit.edu/pub/ issue4-campos-benner
Cotas, J., Pereira, L. (2019). Historical Use of Seaweed as an Agricultural Fertilizer in the European Atlantic Area. Seaweeds as Plant Fertilizer, Agricultural Biostimulants and Animal Fodder. CRC Press.
Chieza, N., Ginsberg, A. D., Lee, S., & Agapakis, C. (2019). Design With Science. Journal of Design and Science. Retrieved from https://jods.mitpress.mit.edu/pub/ issue4-agapakis-lee
Climate Change Cluster, University of Technology Sydney Faculty of Design, Architecture and Building, & Institute for Sustainable Futures (2021). Scoping Algae Futures. Scoping a Circular Algae Bioplastics Industry in Regional NSW. Available at: https://scoping-algaefutures.com/
Craggs, R., Parlk J., Heubeck, S., & Sutherlands, D. (2013). High rate algal pond systems for low-energy wastewater treatment, nutrient recovery and energy production. New Zealand Journal of Botany, Volume 52, 2014 - Issue 1: Algal and cyanobacterial bioenergy and diversity, p. 60-73. Taylor & Francis.
European Commission, DirectorateGeneral for Research and Innovation, (2012). Innovating for sustainable growth - a bioeconomy for Europe, Publications Office. https://data.europa. eu/doi/10.2777/6462
Food and Agriculture Organization (2018). The global status of seaweed production, trade and utilization, Volume 124. Globefish Research Programme.
Ginsberg, A. D., & Chieza, N. (2018). Editorial: Other Biological Futures. Journal of Design and Science. https://doi. org/10.21428/566868b5
Goddek, S., Joyce, A., Kotzen, B., Burnell, G.M. (2019). Aquaponics Food Production Systems. Springer, Cham. https://doi. org/10.1007/978-3-030-15943-6_2
Kelly, J. (2020). Australian Seaweed Industry Blueprint. A Blueprint for Growth. AgriFutures Australia.
Lemm, V. (2022). Homo Natura: Nietzsche, Philosophical Anthropology and Biopolitics. Edinburgh: Edinburgh University Press. https://doi. org/10.1515/9781474466738
McCormick, K., & Kautto, N. (2013). The Bioeconomy in Europe: An Overview. Sustainability, 5(6), 2589–2608. MDPI AG. Retrieved from http://dx.doi. org/10.3390/su5062589
Morton, T. (2013). Hyperobjects: Philosophy and Ecology after the End of the World. University of Minnesota Press. http://www.jstor.org/stable/10.5749/j. ctt4cggm7
Mumford, T.F. and Miura, A. (1988). Porphyra as food: cultivation and economics. in Lembi, C.A. and Waaland, J.R. Algae and Human Affairs. Cambridge University Press, Cambridge. ISBN 0-52132115-8
Nietzsche, F. (1928). The Antichrist. Borzoi Pocket Books.
Pereira, L., Cotas, J. (2019). Historical Use of Seaweed as an Agricultural Fertilizer in the European Atlantic Area. Seaweeds as Plant Fertilizer, Agricultural Biostimulants and Animal Fodder. pp. 1–22.
doi:10.1201/9780429487156-1 ISBN 9780-429-48715-6 S2CID 213435775
Raghunandan, B.L., Vyas, R.V., Patel, H.K., Jhala, Y.K. (2019). Perspectives of Seaweed as Organic Fertilizer in Agriculture. In: Panpatte, D., Jhala, Y. (eds) Soil Fertility Management for Sustainable Development. Springer. https://doi. org/10.1007/978-981-13-5904-0_13
Roque, B., Venegas, M., Kinley, R., de Nys, R., Duarte, T., Yang, X., et al. (2021) Red seaweed (Asparagopsis taxiformis) supplementation reduces enteric methane by over 80 percent in beef steers. PLoS ONE 16(3): e0247820. https://doi. org/10.1371/journal.pone.0247820
Kiran, T., Saxena, A., Tiwari, A. (2020). Diatom mediated heavy metal remediation: a review. Bioresource Technology, (305), 123068–. https://doi. org/10.1016/j.biortech.2020.123068
Schmeer, J. (2019). Xenodesignerly Ways of Knowing. Journal of Design and Science. Retrieved from https://jods. mitpress.mit.edu/pub/6qb7ohpt
Schoch, C. L., Ciufo, S., Domrachev, M., Hotton, C. L., Kannan, S., Khovanskaya, R., Leipe, D., Mcveigh, R., O’Neill, K., Robbertse, B., Sharma, S., Soussov, V., Sullivan, J. P., Sun, L., Turner, S., & KarschMizrachi, I. (2020). NCBI Taxonomy:
a comprehensive update on curation, resources and tools. Database. The journal of biological databases and curation, 2020, baaa062. https://doi. org/10.1093/database/baaa062
Sharma, S., Pati, M., & Nayak, L. (2016). Uses of Seaweed and its Application to Human Welfare: A Review. International Journal of Pharmacy and Pharmaceutical Sciences. 8. 12-20. Innovare Academic Sciences.
Sutherland, D., McCauley, J., Labeeuw, L., Ray, P., Kuzhiumparambil, U., Hall, C., Doblin, M., Nguyen, L., Ralph, P. (2021). How microalgal biotechnology can assist with the UN Sustainable Development Goals for natural resource management. Current Research in Environmental Sustainability, Volume 3. ScienceDirect. 100050. ISSN 2666-0490. https://doi. org/10.1016/j.crsust.2021.100050
Thurstan, R.H., Brittain, Z., Jones, D.S. et al. (2018). Aboriginal uses of seaweeds in temperate Australia: an archival assessment. J Appl Phycol 30, p. 1821–1832. https://doi.org/10.1007/s10811-0171384-z
Tonuk, D., Fisher, T. (2020). Material Processuality: Alternative Grounds for Design Research. Design and Culture 12(2):1-21. Taylor & Francis. https://doi.org/ 10.1080/17547075.2020.1717779
United Nations (2015). Transforming Our World: The 2030 Agenda For Sustainable Development A/Res/70/1. Accessed online on September 12th, 2022 in:
https://sustainabledevelopment.un.org/ content/documents/21252030%20 Agenda%20for%20Sustainable%20 Development%20web.pdf
“A Biodesign Code of Ethics” Biodesigned: Issue 9, 11 November, 2021. Accessed [Sept, 12, 2022].
David Benjamin, ‘Bio fever’ Domus, published online on March 30, 2011 (http://www. domusweb.it/en/op-ed/biofever/). https://omuus.com/biodesign https://www.domusweb.it/en/ opinion/2011/03/30/bio-fever.html
https://jods.mitpress.mit.edu/pub/ issue4-agapakis-lee/release/1
https://seaweedforestsfestival.libsyn. com/talk-1-the-awe-and-wonder-of-ourunderwater-seaweed-forests-ocean-ifestival-i-great-southern-reef
https://sustainabledevelopment.un.org/ content/documents/21252030%20 Agenda%20for%20Sustainable%20 Development%20web.pdf
https://www.3dprintlife.com/alga https://jessiefrench.com/ https://www.julialohmann.co.uk/ https://www.britishmuseum.org/ collection/object/E_Oc1851-1122-2
https://violainebuet.com/home-en/ https://www.nienkehoogvliet.nl/portfolio/ seame/ https://www.nienkehoogvliet.nl/portfolio/ sea-me-collection/
https://www.theseaweedproject.nl/ https://blondandbieber.com/algaemy https://www.europarl.europa. eu/news/en/headlines/ society/20201208STO93327/ the-impact-of-textile-productionand-waste-on-the-environmentinfographic#:~:text=Textile%20 production%20is%20estimated%20 to,into%20the%20ocean%20a%20year https://www.ecologicstudio.com/ projects/air-bubble-air-purifying-ecomachine https://www.arup.com/projects/solar-leaf https://www.uts.edu.au/about/facultydesign-architecture-and-building/ staff-showcase/designing-wastefuture-biomaterials-carbon-storageexhibited-art-gallery-south-australia
https://www.australianseaweedinstitute. com.au/seaweed-biofilters
https://www.dezeen.com/2019/01/18/ margarita-talep-algae-bioplasticpackaging-design/ https://www.hhs.gov/ohrp/regulationsand-policy/belmont-report/read-the-
belmont-report/index.html
https://www.ecologicstudio.com/ projects/bio-bombola
https://colorifix.com/colorifix-solutions/
The elaboration of this document is accompanied with a field research task consisting of the recollection of seaweed specimens from natural environments in order to study them from a phenomenological approach, building an embodied understanding of their physical and chemical characteristics, their transformation and decomposition processes, and their entanglement to other living beings and their context.
After the on-site survey, retrieved specimens were identified and kept refrigerated before being sun-dried. The observed process consisted of the dehydration and loss of volume of the samples, as well as the formation of a saline crust on the surface that prevented the growth of fungi and other microorganisms for the 4 weeks of the observation.
The data collection for the aforementioned task is comprised of photographs, notetaking, and ambient sound measurements, that are presented in this annex.
DATE: 21/08/2022, 15:20h
LOCATION: Seven Shillings Beach
The beach is accessed through a set of stairs descending from Woodhara Council building. It is a public access urban beac with approximately 6 meters of width and 310 meters of length, facing northwest, 5.5km into the Sydney Harbour.
It is located in a mid-density residential area. With buildings located directly on the beach. Despite the environmental impact of construction so close to the shoreline, they create a barrier from traffic noise, making it very quiet (50-60dB). The only source of significant anthropogenic noise are the helicopters patrolling Sydney Harbour.
The water is, however, populated with private recreational boats, mostly anchored and not in use.
Only seagulls and grey fantails seem to populate the beach, in small numbers. There are also several people sunbathing but not engaging in water activities. There is a swimming net that protects about a quarter of the beach’s length, making it the only area where swimming is practiced.
The collection focused on the easternmost 20 meters of the shoreline, where the algae was being deposited in larger quantities due to the tides and the geometry of the shore.
Most of the collection was from seaweed that had been washed ashore, balthough some specimens were retrieved from the water. Specimens were washed and photographed before collection. Specimens that were found to contain other living creatures were photographed and released back in the water.
The retrieved seaweed was transported wet in a plastic container back to the studio for further analysis and categorization.