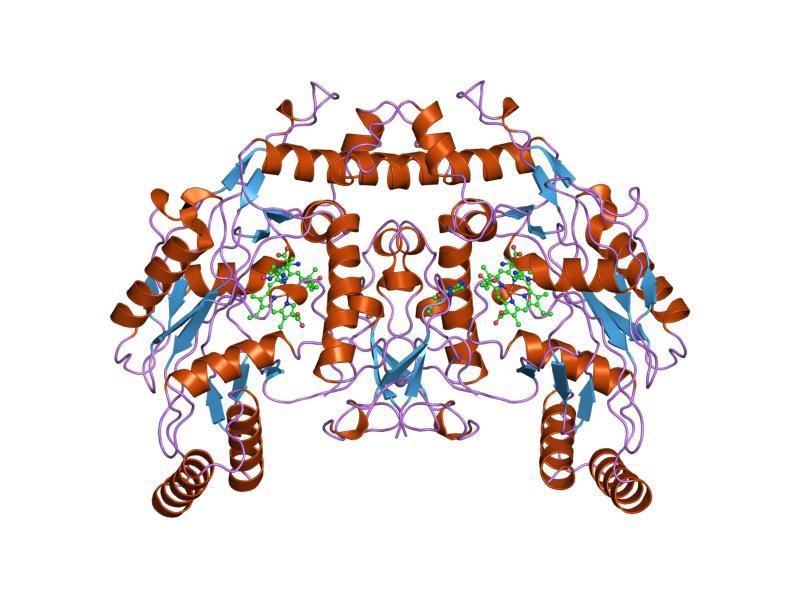
89 minute read
Gasotransmitters: New Frontiers in Neuroscience
BY AUDREY HERRALD '23
Cover Image: A threedimensional representation of the nitric oxygen synthase (NOS) protein, a critical player in the synthesis of nitric oxide (NO), the first identified gasotransmitter Created by Jawahar Swaminathan and MSD staff at the European Bioinformatics Institute, from Wikimedia Commons Introduction The average adult brain is comprised of about 120 billion neurons (Herculano-Houzel, 2001). Communication between the neurons in this vast network is critically important for cognition, behavior, and even physiological function; in short, neural signaling is a critical element of life (Neural Signaling, 2001). This signaling generally occurs through one or some combination of two mechanisms: electrical transmission and chemical transmission. The “all-or-nothing” action potential is a relatively well-known example of electrical transmission, and endogenous signaling molecules called neurotransmitters are equally familiar. However, a lesser-known but profoundly important class of molecules regulates our cardiovascular, nervous, gastrointestinal, excretory, and immune systems—in addition to many cellular functions, including apoptosis, proliferation, inflammation, cellular metabolism, oxygen sensing, and gene transcription (Handbook of Hormones, 2016). Their name? Gasotransmitters.
Advertisement
The phrase “gasotransmitter” was first coined in 2002 when a team of researchers identified hydrogen sulfide (H2S) as the third gaseous signaling molecule of its kind (Wang et al., 2002). The rotten-egg smelling molecule joined nitric oxide (NO) and carbon monoxide (CO) in the group of molecules referred to as gasotransmitters. Since then, advancements in understanding of the cellular signaling process have led to the proposed identification of other gasotransmitters, like ammonia (NH3). These molecules dictate a wide variety of physiological processes, and the mechanisms of effect are accordingly varied. Section 1 of this paper will address the various functional mechanisms of the three broadly accepted gasotransmitters, in addition to providing a clearer profile of what, exactly, these gasotransmitters look like and how they mediate neural connectivity. Section
2 will address the role of gasotransmitters in a number of neurological diseases and psychiatric conditions, along with any potential avenues for gasotransmitter-related treatment.
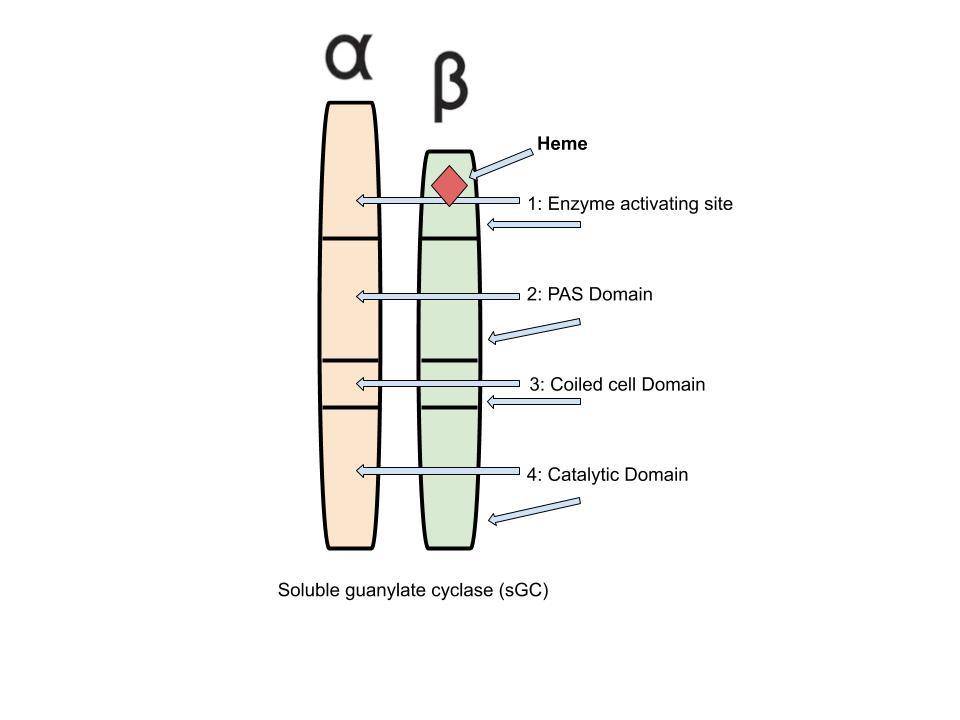
Form and Function What are gasotransmitters? Gasotransmitters are gaseous signaling molecules produced within the body. Their characterization as such is recent, and discussions regarding the molecules’ official designation are still ongoing; some suggest the term “gaseous messengers,” while others advocate for “small-molecule signaling species” (Wang, 2018). The term “gasotransmitter,” however, refers to a specific set of criteria that do not necessarily apply to alternate namings— hence the term’s selection for this article. Gasotransmitters, for example, are endogenous. This means that oxygen (O2), which can be classified as both a “gaseous messenger” and a “small-molecule signaling species,” cannot be a gasotransmitter (Wang, 2018). The proposal of alternate terms invites ambiguity. However, these proposals also point to an important truth: interest in gasotransmitters is quickly growing within the scientific community. Accordingly, the synthesis, form, and function of gasotransmitters are emerging with increasing clarity.
Currently, the three molecules widely recognized as gasotransmitters are nitric oxide (NO), carbon monoxide (CO), and hydrogen sulfide (H2S). Each of these primary gasotransmitters has a series of chemical derivatives (such as nitrite (NO2 −), nitrate (NO3 −), nitrous oxide (N2O), and nitroxyl (HNO) for NO). These derivatives are classified within the gasotransmitter family, even though some exist in non-gaseous forms, because they often perform signaling functions in place of their primary molecule or help to buffer fluctuations in gasotransmitter levels (Ida et al., 2014). The derivatives play important physiological roles, which are addressed in subsequent sections. First, though, it is important to understand the general functional mechanisms of the primary gasotransmitters. All molecules classified as gasotransmitters must fulfil six defining criteria (Wang, 2002):
(i) They are small molecules of gas.
(ii) They are freely permeable through membranes and therefore act without specified membrane receptors.
(iii) They are generated endogenously. (iv) They have well-defined, specific functions at physiologically relevant, generally exceptionally low, concentrations.
(v) Their functions can be mimicked by exogenous counterparts.
(vi) Their cellular effects may or may not be mediated by secondary messengers, but specific final targets are implicated.
The first three criteria refer to the molecules themselves, while the last three refer to their physiological effects. First, there is the size and state requirement. “Small” here is defined as having a molecular mass between 28 and 34 amu, which excludes a vast array of endogenous species (Wang, 2002). The requirement of a gaseous state is also important; gasotransmitters exist either in gaseous form or are dissolved in circulation, intracellular fluid, and/or interstitial (between cells) fluid (Wang, 2018). Next comes membrane permeability. Gasotransmitters do not require cognate membrane receptors to interact with cells; their gaseous state enables them to ‘slip through the gate’ without the need for a gatekeeper. This is where gasotransmitters differ from well-known transmitters like hormones, neurotransmitters, and drugs, all of which require and interact extensively with cellular receptors. Third, gasotransmitters must be produced endogenously. Thus far, all identified gasotransmitters are not only produced endogenously, but produced specifically through highly regulated enzymatic processes. The careful bioregulation of these molecules
Figure 1: Representation of the enzyme soluble guanylate cyclase (sGC), the only known target of the NO gasotransmitter Created by Audrey Herrald, reference Elango, 2017
Figure 2: Molecular orbital diagram for NO Source: Wikimedia Commons
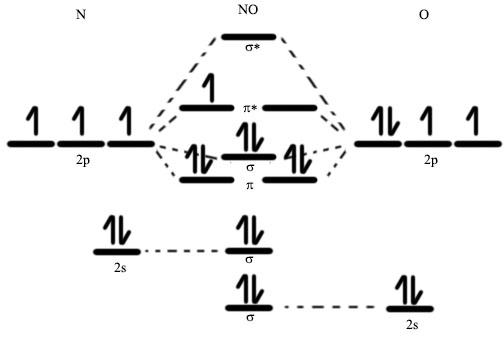
makes sense, as CO, NO, and H2S can all be highly toxic in unregulated conditions (CO and NO interfere with oxygen exchange and H2S is a respiratory tract irritant) (Wareham et al., 2018).
Now come the function-related stipulations for molecules in the gasotransmitter family. The fourth criterion has to do with both concentration and physiological effect: gasotransmitters must play a role that is both well-defined and specific to that molecule, and this specific molecular function must take place at a known (and generally low) endogenous concentration. This means that manipulating the concentration of gasotransmitters within the body should evoke specific physiological changes, in line with the identified role of the molecule. The fifth criterion has particularly noteworthy implications for the study of gasotransmitters. It states that exogenous (created outside of the body) substances can effectively mimic the activity of endogenous gasotransmitters. This possibility for mimicry allows scientists to selectively manipulate certain qualities (like concentration, for example) of the gasotransmitters, and then observe their physiological effects. Studies like these help researchers understand more about which characteristics of gasotransmitters hold particular significance. An often-utilized tool for these types of studies is a class of substances called NO-releasing compounds, which solve the issue of NO administration (high reactivity in air and concentration-dependent function) by reversibly storing and releasing NO under specific conditions (Cheng, 2019). The recent engineering of these “NO donors” is just one example of the many scientific developments elicited by our emerging understanding of gasotransmitters.
Finally, the sixth criterion refers to both signaling mechanism and signaling result. All gasotransmitters have certain physiological effects, from regulation of the immune, cardiovascular, and nervous systems to initiation of cellular events like apoptosis, inflammation, and proliferation (Handbook of Hormones, 2016). These physiological effects depend on a series of interactions between gasotransmitters and proteins. The sixth gasotransmitter qualification criterion clarifies that even though gasotransmitters must have specific physiological effects, these effects need not result from direct interaction with a gasotransmitter. In other words, the series of events from gasotransmitter to end-result can be long, provided that the path between the pair can be clearly traced. One simple example of this principle involves the dilation of blood vessels via NO signaling: while NO can induce vasodilation directly by binding to oxygencarrying myoglobin (thus enabling oxygendependent muscle cells in vasculature walls to dilate), NO might also interact with the enzyme guanylyl cyclase (GC) to initiate a chain reaction with a series of intermediaries prior to inducing blood vessel dilation (Ormerod et al., 2011). Either way, according to the six generally accepted criteria listed above, NO passes the test and is classified as a gasotransmitter.
Meet the threesome Though technological advancements and new research keep leading to the proposal
Figure 3: Electron configuration for Fe2+
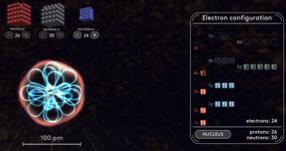
Created with MEL VR Virtual Chemistry by Audrey Herrald.
of additional gasotransmitters, the family of signaling molecules currently consists of three definite members: Nitric oxide (NO), carbon monoxide (CO) and hydrogen sulfide (H2S) (Shefa et al., 2017; Wang et al., 2020). Each of these molecules interact differently with various physiological targets, and their mechanisms of synthesis are unique. First, the knowns: scientists believe that they have successfully identified many of the biological targets for NO, CO, and H2S. The mechanisms of interaction with these targets, however, remain somewhat ambiguous; and researchers still hope to uncover the identities of the proteins that modulate gasotransmitter function, the effect of gasotransmitter production on other gasotransmitters, and the characteristics of gasotransmitter sensor proteins, among other questions. Future research will build in part upon two key areas of gasotransmitter understanding, as outlined by field-pioneer Rui Wang: interactions with producers, and interactions with targets (Wang, 2018).
First, for producer interactions: the identification criteria for gasotransmitters stipulate that gasotransmitters must be created inside the body. This makes synthesis an important element of gasotransmitter function. Each of the three main gasotransmitters, H2S, NO, and CO, are produced through enzymatic processes. Hydrogen sulfide production depends primarily upon three enzymes: cystathionine γ-lyase (CSE), cystathionine β-synthase (CBS), and 3-mercaptopyruvate sulfurtransferase (MST) (Zhu 2017). Enzymatic production of NO is catalyzed by three subtypes of nitrous oxide synthase (NOS) enzymes, known as eNOS, iNOS, and neuronal NO synthase (nNOS) (Wang 2018). Lastly, endogenous CO is produced following a catalysis process that involves oxygenase (HO). In these enzymatic processes, different amino acids—either obtained through consumption of protein-rich foods or produced through the endogenous breakdown of other consumed proteins—bind to one of the enzymes mentioned above. When this bond occurs, slow-moving chemical reactions accelerate greatly (more than a millionfold), resulting in the production of new substances (Castro, 2014). Variations of this enzymatic synthesis generate H2S, NO, and CO.
Upon production, gasotransmitters begin interacting with their physiological targets. Importantly, though one might read that gasotransmitters regulate the immune system or increase breathing rate, (Handbook of Hormones, 2016), gasotransmitters (like other bodily signaling molecules) seldom act directly on these systems. In reality, newly synthesized molecules of H2S, NO, and CO tend to exert small effects on specific targets, the results of which trigger physiological processes that might indeed lead to improvements in the immune system or an uptick in respiration. Each gasotransmitter has a wide range of initial targets and a wider range of physiological functions, many of which likely remain to be discovered. However, a few key mechanisms for physiological function have been thoroughly outlined. The next section provides a demonstrative example of one such pathway: the reduction of tension in blood vessel walls, also known as “vasorelaxation,” initiated by the gasotransmitter NO (Wang 2018).
Nitric Oxide and Vasorelaxation: From synthesis to final effect
Nitric oxide (NO) has only one known target, called soluble guanylate cyclase (sGC). sGC “... though one might read that gasotransmitters regulate the immune system or increase breathing rate, gasotransmitters (lie other bodily signaling molecules) seldom act directly on these systems.”
is an enzyme (encoded by three different genes) that is comprised of two subunits. Both subunits of the sGC enzyme have four domains, each involved in different elements of enzyme function. Figure 1 provides a visual representation of the two subunits and eight domains. One of the sGC subunits, the “beta” subunit, includes a small, adjunct molecule called a ligand. This specific ligand is histidine, a common amino acid, coordinated to an ironcontaining molecule known as a “heme.” The heme, Fe2+, is the specific target of NO.
Nitric oxide binds to the Fe2+ heme with particularly high affinity. Why? The answer lies in the arrangement of electrons associated with each substance. In NO, electrons from the nitrogen and oxygen atoms come together in such a way that one “molecular orbital,” called the pi* orbital, remains only partially filled. (Molecular orbitals are mathematical functions that help describe the probability of finding electrons in certain regions around the molecule.) The partially filled pi* orbital in NO, shown as lines without arrows (without “electrons”) in Figure 2, can help visualize the outer-most electrons that are associated with NO.
The heme on the beta subunit of sGC is Fe2+ , which can be visualized with a representation like the one in Figure 3. As in Figure 2, the arrows represent electrons. In general, for two species to bond by sharing electrons, these two species must possess valence electrons in comparable energy levels to one another. Further, the orbitals—the regions where these electrons are most likely to be found—must match up in a particular way. In the case of NO and Fe2+, if both species were to retain the electron organizations depicted in Figures 2 and 3, the electrons wouldn’t quite match up. However, a phenomenon known as “back bonding” enables electrons from Fe2+ to move from their highest-energy atomic orbitals (see the “3d” label in Figure 3) to the pi* molecular orbital (see the empty lines in the center of Figure 2) of NO (Cotton et al., 1999). Nitric oxide, with one unpaired electron, has a particular affinity for back bonding in appropriate situations (Cotton et al., 1999). A combination of back bonding, electrostatic attractions, and a number of related forces (all driven towards an end-goal of reducing the system’s free energy) allow the gasotransmitter to group itself tightly into molecular complex with the Fe2+ heme and the amino acid ligand, all just one element of the much larger sGC enzyme. Upon bonding, the presence of NO leads to more than a 200-fold increase in sGC activity (Offermanns et al., 2008). Activation of the heme on the beta subunit of sGC (see Figure 1) initiates activity in the catalytic region of the enzyme, where sGC catalyzes the transition of a common nucleotide called guanosine 5′-triphosphate (GTP) to an important messenger compound known as cGMP. Remarkably, sGC is one of only two enzymes that produces cGMP. This means that sGC (and thereby NO, being a key sGC transmitter) is intimately involved in many signal transduction pathways. One of these pathways capitalizes on the rise in cGMP concentration (a result of NOinitiated sGC activation) to set off a signaling cascade. cGMP-dependent protein synthesis, as well as cation channels that open and close in accordance with cGMP concentration, all contribute to the release of a chemical signal (myosin phosphate). Finally, myosin phosphate drives smooth muscle cells to release their stores of excess calcium—thereby relaxing the muscles and leading to NO-initiated vasodilation (Denninger et al., 1999).
The dilation of blood vessels is one physiological result of NO signaling, but the molecule has a wide variety of additional functions. Due to its many roles, loss of NO bioactivity contributes to disease in many conditions—making restoration of NO bioavailability an attractive therapeutic avenue for many diseases and disorders (Helms & Kim-Shapiro, 2013). And even though NO signaling affects regions throughout the body, one of its most prominent areas of impact, together with CO and H2S, is the nervous system. In the next section, several relations between gasotransmitters and neurological conditions are outlined.
Gasotransmitters and Neurological Conditions Gasotransmitters have been implicated in a number of neurological conditions, including Alzheimer’s disease, autism spectrum disorder, Parkinson’s disease, and multiple sclerosis (MS) (Steinert et al,. 2010). These disorders all have in common the degeneration or disruption of neural plasticity. Neural plasticity, or synaptic plasticity, is the ability of neurons in the brain and spinal cord to adapt in response to changes in the environment or damage to neural tissue (Sharma et al., 2016). This plasticity is critical for proper communication among neurons, maintenance of bodily homeostasis, and for the regulation of neural transmission (Shefa et al., 2018). It makes sense,
then, that disruptions to neural plasticity can harbor devastating effects; from psychiatric disorders like schizophrenia and bipolar disorder to neurodegenerative disorders like Alzheimer’s disease, the link between synaptic plasticity and many common neurological conditions is becoming increasingly clear (Shefa at al., 2018; Kumar et al., 2017). Now, as gasotransmitters—NO in particular—have emerged as important regulators of synaptic plasticity, the transmitters’ roles in neurological conditions are an increasingly common subject of investigation. (Shefa at al., 2018).
Among common neurodegenerative diseases, Alzheimer’s disease is one of the most closely associated with synaptic plasticity (Kumar et al., 2017). While the loss of synaptic plasticity— closely related to working memory—is not the cause of Alzheimer’s disease, it may be both a symptom and a pre-diagnosis warning sign; recent research provides in vivo evidence for reduced synaptic plasticity in the brain’s frontal lobe among early- and mid-stage Alzheimer’s disease patients (Kumar et al., 2017). Why might reduced plasticity be a problem? Dr. Sanjeev Kumar, lead author of a recent study on Alzheimer’s disease and synaptic plasticity, explains that healthy neuronal plasticity supports the brain’s “cognitive reserve,” or the protection that offsets poorer functioning in other brain areas and shields against the development of neurodegenerative disease (Kassam, 2017). Thus, any physiological process with a significant effect on synaptic plasticity would likely have an effect on neurodegenerative diseases like Alzheimer’s, as well—and gasotransmitters fit precisely this description. The upregulation or downregulation of gasotransmitters may be a cause of neurodegenerative disorders, and targeted enhancement of gasotransmitter function may prove therapeutic by restoring synaptic plasticity (Shefa et al., 2018).
Another facet of the relationship between gasotransmitters and neurological disorders is the effect of gasotransmitters on oxidative stressors. The term “oxidative stress” describes an imbalance between harmful free radicals and their ameliorating pair, antioxidants, within the brain (Pizzino et al., 2017). This imbalance, when present, leaves neural cells vulnerable to attack from unchecked free radicals—the results of which include protein misfolding, glia cell over-activation, mitochondrial dysfunction, and (in the worst cases) subsequent cellular destruction (Kim at al., 2015). Enter gasotransmitters. The molecules boast a variety of defenses against neurodegenerationinducing oxidative stress. NO, perhaps the most ubiquitous of the gasotransmitters, has the ability to activate a subset of brain receptors known as NMDA receptors, or NMDARs, which help defend against the harmful effects of free radicals (Huque et al., 2009). NMDARs also initiate a signaling pattern that leads to the generation of (more) endogenous NO. The increased levels of NO drive NMDAR activation, and this positive feedback loop can serve as a critical natural defender against oxidative stress (Shefa et al., 2018).
The other gasotransmitters, too, can help protect the brain from neurological disorders. H2S appears to have a role in defending against major depressive disorder; studies have shown robust anti-depressive effects via a signaling pathway known as the tropomyosin receptor kinase B -rapamycin (TrKB-mTOR) pathway (Hou et al., 2017). CO has been shown to restore synaptic function in both Alzheimer’s disease and schizophrenia, two vastly different disorders, though with the common characteristic of neural deterioration (McGlashan, 1998; Magalingam, 2018). Lastly, NO emerges again, this time as an upregulator of a messenger molecule called cyclic AMP (cAMP)—which binds a restorative protein called CREB and subsequently reduces the influx of Ca2+ during neural signaling; this action has been linked to reduced symptomology in both schizophrenia and major depressive disorder.
The possibilities for gasotransmitter-related treatment of neurodegenerative and neuropsychiatric disorders are undoubtedly numerous. The next hurdle to cross here will be the development of a more thorough understanding of the signaling mechanisms associated with these gaseous transmitters (Wang 2018). The identified links between gasotransmitters and synaptic plasticity, as well as between gasotransmitters and oxidative stress, are both promising signs that further work on gasotransmitters may improve treatments for currently currently incurable conditions.
Conclusion The term “gasotransmitter” is truly only as old as a typical first-year college student. A keyword-search reveals just four published books on the subject, undoubtedly preceding a host of additional works. Since one of the first defining papers on gasotransmitters was “The other gasotransmitters, too, can help protect the brain from neurological disorders. H2S appears to have a rolein defending against major depressive disorder.”
published 18 years ago, the molecular family has garnered significant scientific attention, and knowledge on the subject will only continue to grow. As it does, among the most exciting prospects is the development of new treatments for neurodegenerative diseases and other neurological disorders. Two of the most recent insights—that gasotransmitters seem to mediate neural repair and harbor protective effects against oxidative stress—are certain to generate subsequent investigation, and they might just offer insight as to the most effective mechanisms for management of neurodegenerative conditions (Shefa at al., 2017). In the meantime, researchers will likely continue to investigate the signaling mechanisms of these transmitters— particularly the identity of sensor proteins and the interactions between gases. New molecules are bound to join ammonia in working their way towards gasotransmitter-classification. The field is new and growing, with the potential for lifesaving clinical applications inching ever closer.
References
Cheng, J., He, K., Shen, Z., Zhang, G., Yu, Y., & Hu, J. (2019). Nitric Oxide (NO)-Releasing Macromolecules: Rational Design and Biomedical Applications. Frontiers in Chemistry, 7. https://doi. org/10.3389/fchem.2019.00530
Declines in plasticity reveals promising treatments for Alzheimer’s disease. (n.d.). Drug Target Review. Retrieved July 24, 2020, from https://www.drugtargetreview.com/ news/26979/plasticity-alzheimers/
Donald, J. A. (2016). Chapter 103—Gasotransmitter Family. In Y. Takei, H. Ando, & K. Tsutsui (Eds.), Handbook of Hormones (pp. 601–602). Academic Press. https://doi.org/10.1016/B978-0-12801028-0.00103-3
Herculano-Houzel, S. (2009). The Human Brain in Numbers: A Linearly Scaled-up Primate Brain. Frontiers in Human Neuroscience, 3. https://doi.org/10.3389/neuro.09.031.2009
Hoque, K. E., Indorkar, R. P., Sammut, S., & West, A. R. (2010). Impact of dopamine-glutamate interactions on striatal neuronal nitric oxide synthase activity. Psychopharmacology, 207(4), 571–581. https://doi.org/10.1007/s00213-009-1687-0
Hou, X.-Y., Hu, Z.-L., Zhang, D.-Z., Lu, W., Zhou, J., Wu, P.-F., Guan, X.-L., Han, Q.-Q., Deng, S.-L., Zhang, H., Chen, J.-G., & Wang, F. (2017). Rapid Antidepressant Effect of Hydrogen Sulfide: Evidence for Activation of mTORC1-TrkB-AMPA Receptor Pathways. Antioxidants & Redox Signaling, 27(8), 472–488. https://doi.org/10.1089/ars.2016.6737
Ida, T., Sawa, T., Ihara, H., Tsuchiya, Y., Watanabe, Y., Kumagai, Y., Suematsu, M., Motohashi, H., Fujii, S., Matsunaga, T., Yamamoto, M., Ono, K., Devarie-Baez, N. O., Xian, M., Fukuto, J. M., & Akaike, T. (2014). Reactive cysteine persulfides and S-polythiolation regulate oxidative stress and redox signaling. Proceedings of the National Academy of Sciences of the United States of America, 111(21), 7606–7611. https://doi.org/10.1073/ pnas.1321232111 Kim, G. H., Kim, J. E., Rhie, S. J., & Yoon, S. (2015). The Role of Oxidative Stress in Neurodegenerative Diseases. Experimental Neurobiology, 24(4), 325–340. https://doi. org/10.5607/en.2015.24.4.325
Kumar, S., Zomorrodi, R., Ghazala, Z., Goodman, M. S., Blumberger, D. M., Cheam, A., Fischer, C., Daskalakis, Z. J., Mulsant, B. H., Pollock, B. G., & Rajji, T. K. (2017). Extent of Dorsolateral Prefrontal Cortex Plasticity and Its Association With Working Memory in Patients With Alzheimer Disease. JAMA Psychiatry, 74(12), 1266–1274. https://doi.org/10.1001/ jamapsychiatry.2017.3292
Magalingam, K. B., Radhakrishnan, A., Ping, N. S., & Haleagrahara, N. (2018, March 8). Current Concepts of Neurodegenerative Mechanisms in Alzheimer’s Disease [Review Article]. BioMed Research International; Hindawi. https://doi.org/10.1155/2018/3740461
McGlashan, T. H. (1998). The profiles of clinical deterioration in schizophrenia. Journal of Psychiatric Research, 32(3–4), 133–141. https://doi.org/10.1016/s0022-3956(97)00015-0
Ormerod, J. O. M., Ashrafian, H., Maher, A. R., Arif, S., Steeples, V., Born, G. V. R., Egginton, S., Feelisch, M., Watkins, H., & Frenneaux, M. P. (2011). The role of vascular myoglobin in nitrite-mediated blood vessel relaxation. Cardiovascular Research, 89(3), 560–565. https://doi.org/10.1093/cvr/cvq299
Pizzino, G., Irrera, N., Cucinotta, M., Pallio, G., Mannino, F., Arcoraci, V., Squadrito, F., Altavilla, D., & Bitto, A. (2017). Oxidative Stress: Harms and Benefits for Human Health. Oxidative Medicine and Cellular Longevity, 2017. https://doi. org/10.1155/2017/8416763
Purves, D., Augustine, G. J., Fitzpatrick, D., Katz, L. C., LaMantia, A.-S., McNamara, J. O., & Williams, S. M. (2001). Neural Signaling. Neuroscience. 2nd Edition. https://www.ncbi.nlm. nih.gov/books/NBK10882/
SHARMA, N., CLASSEN, J., & COHEN, L. G. (2013). Neural plasticity and its contribution to functional recovery. Handbook of Clinical Neurology, 110, 3–12. https://doi. org/10.1016/B978-0-444-52901-5.00001-0
Shefa, U., Yeo, S. G., Kim, M.-S., Song, I. O., Jung, J., Jeong, N. Y., & Huh, Y. (2017, March 12). Role of Gasotransmitters in Oxidative Stresses, Neuroinflammation, and Neuronal Repair [Review Article]. BioMed Research International; Hindawi. https://doi.org/10.1155/2017/1689341
Steinert, J. R., Chernova, T., & Forsythe, I. D. (2010). Nitric oxide signaling in brain function, dysfunction, and dementia. The Neuroscientist: A Review Journal Bringing Neurobiology, Neurology and Psychiatry, 16(4), 435–452. https://doi. org/10.1177/1073858410366481
Wang, R. (2018). Chapter 1 Overview of Gasotransmitters and the Related Signaling Network. 1–28. https://doi. org/10.1039/9781788013000-00001
Wareham, L. K., Southam, H. M., & Poole, R. K. (2018). Do nitric oxide, carbon monoxide and hydrogen sulfide really qualify as ‘gasotransmitters’ in bacteria? Biochemical Society Transactions, 46(5), 1107–1118. https://doi.org/10.1042/ BST20170311
Yakovlev, A. V., Kurmasheva, E. D., Giniatullin, R., Khalilov, I., & Sitdikova, G. F. (2017). Hydrogen sulfide inhibits giant depolarizing potentials and abolishes epileptiform activity of neonatal rat hippocampal slices. Neuroscience, 340, 153–165.
https://doi.org/10.1016/j.neuroscience.2016.10.051 Zabłocka, A. (2006). [Alzheimer’s disease as neurodegenerative disorder]. Postepy Higieny I Medycyny Doswiadczalnej (Online), 60, 209–216.
The Science of Anti-Aging
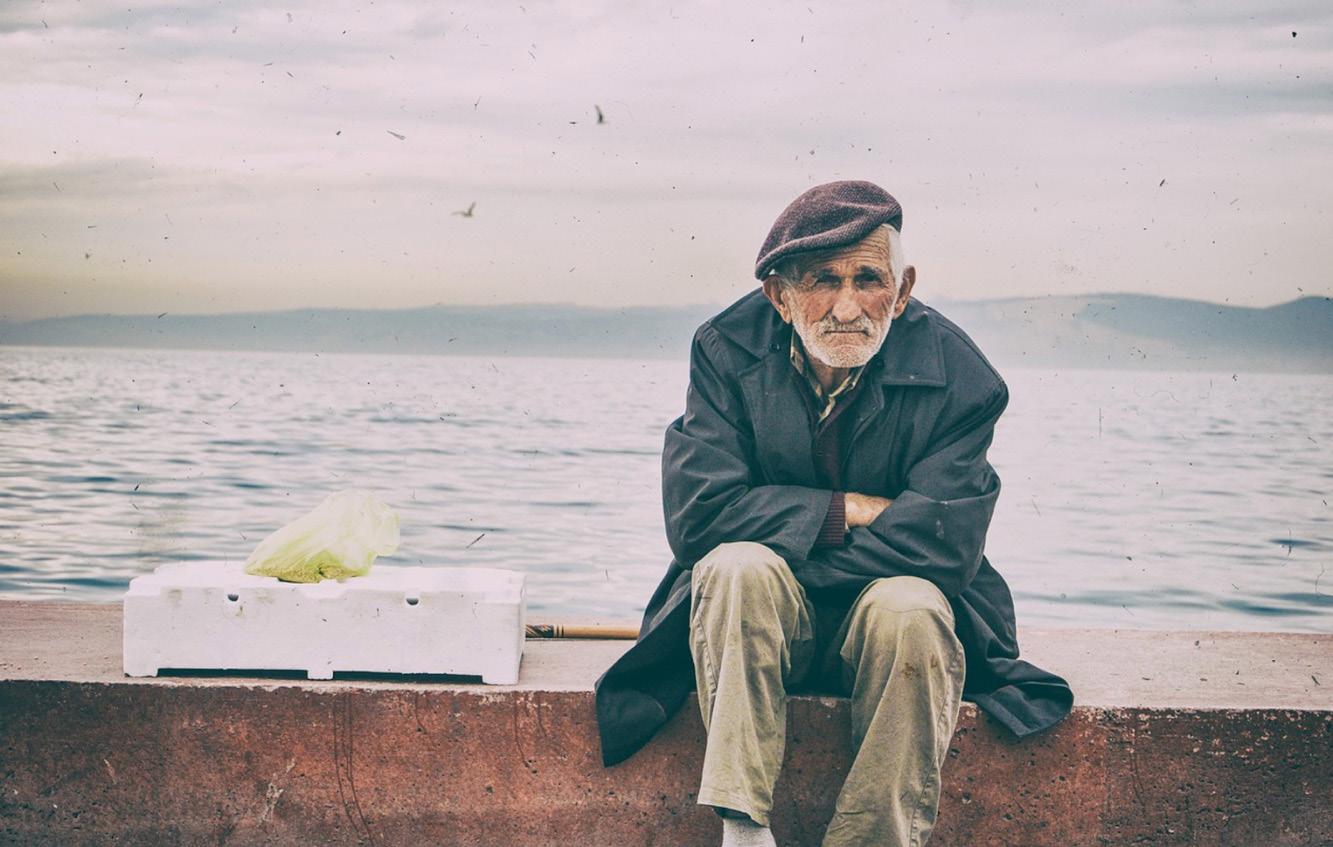
BY AVISHI AGASTWAR, MONTA VISTA HIGH SCHOOL SENIOR
Cover Image: Old man sitting at banks of river
Source: Image by Isakarakus from Pixabay Introduction to Aging Anti-aging medicine has long been a topic of discussion among biogerontologists – scientists who study the process of aging. They apply their understanding to discover methodologies and medicinal processes to delay the aging process. Anti-aging is the process of delaying old age or the onset of ailments and medical conditions that accelerate the process of getting old and eventually lead to death (Juengst, 2013). Research in this domain has been highly sought after by individuals looking for ways to live longer and healthier lives. Scientific research and development-based companies like Calico, AgeX, BioAge, BioViva, and the Longevity Fund are working towards addressing the large gaps in our understanding about the science of aging. This research involves interventions like DNA repair, enhancement of the immune system, synthesis and degradation of proteins, cell replacement, and cell regeneration. From a scientific perspective, aging is defined as the gradual decline in the body’s capacity for cellular repair and increased risk of age-related pathology. At the cellular level, aging manifests in the form of nuclear DNA mutations, changes in the rates of synthesis of cells, and heightened levels of protein degradation (Hayflick, 2004). While bodily functions are hampered, the speed at which cellular aging happens varies between individuals as well as between different cells within a particular individual (Rattan, 2014). From an evolutionary perspective, this process of gradual degradation is explained by the notion that the rapid and efficient processes of body function repair are essential only until a species procreates. After procreation, there is decreased need for efficient functioning to continue indefinitely. In other words, evolution selects for a species capacity to reproduce, not to live a very long life.
Figure 1. Life expectancy at birth by world region from 1950 to 2050
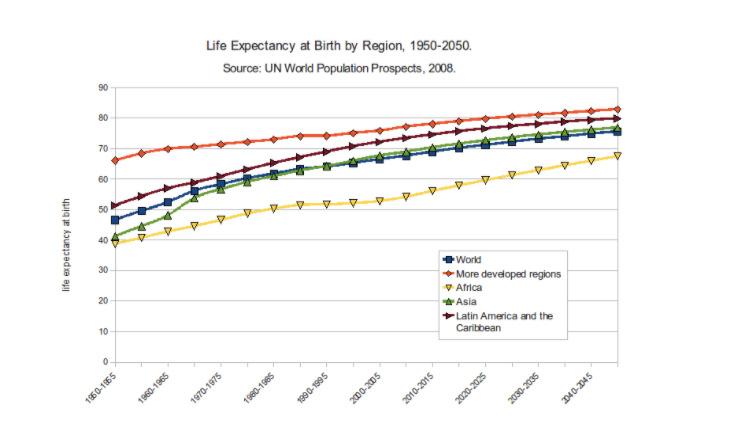
Source: Wikimedia Commons
Cellular repair mechanisms are monitored by genes called the Longevity Assurance Genes. These genes overlook the maintenance and repair functions of our cells. DNA repairing ability, counteracting stress, and prevention of unregulated proliferation of cells is positively correlated to enhanced lifespan of an individual. These abilities are deeply encoded in the genetics of the individual. This is supported by the fact that until very recently in the evolutionary timeline of our species – that spans millions of years – the Homo sapiens’ life expectancy was close to 30 years (Rattan, 2004). In 1900, the average life expectancy was 49 years; today, as a result of antibiotics and vaccination, it has jumped to 73 years, surpassing the evolutionarily essential lifespan (Hayflick, 2004). However, biotechnology and medical science are yet to find a solution to the problem of chronic diseases.
Approaches to Counter-Aging Anti-aging research is multifaceted and must be approached from multiple angles. Consequently, scientists have three general paradigms for framing their research. The first of these is to look at the eventual onset of chronic ailments brought about as a result of old age (Laberge et al., 2019). Scientists operating under this paradigm do not intend to increase the lifespan of the human body, but instead work to improve the quality of life of people as they age. As a result, people not only live longer but live healthier and remain a contributing part of society. This is valuable to society in that it has the potential to lower healthcare and social security expenditures dramatically. The second anti-aging approach is to work towards a longer lifespan by delaying the processes that result in aging. Scientists working under this paradigm claim that an average life expectancy of 112 years is a reasonable prediction (Juengst, 2013). Finally, the third and most ambitious paradigm is to attempt to reconfigure and revitalize the basic cellular processes that take place in the human body, slowing down the process of aging and potentially extending the human lifespan. The goal is to backpedal the damage already done by aging in adults and preemptively prevent such damage in young people. It is accomplished by using DNA reconfiguration to specific amino acids to make targeted changes to the operation of a person’s body (Juengst, 2013). “DNA repairing ability, cunteracting stress, and prevention of unregulated proliferation of cells is positively correlated to enhanced lifespan of an individual."
Figure 2. The skin is part of the integumentary system and consist of multiple layers that have a role in anti-aging
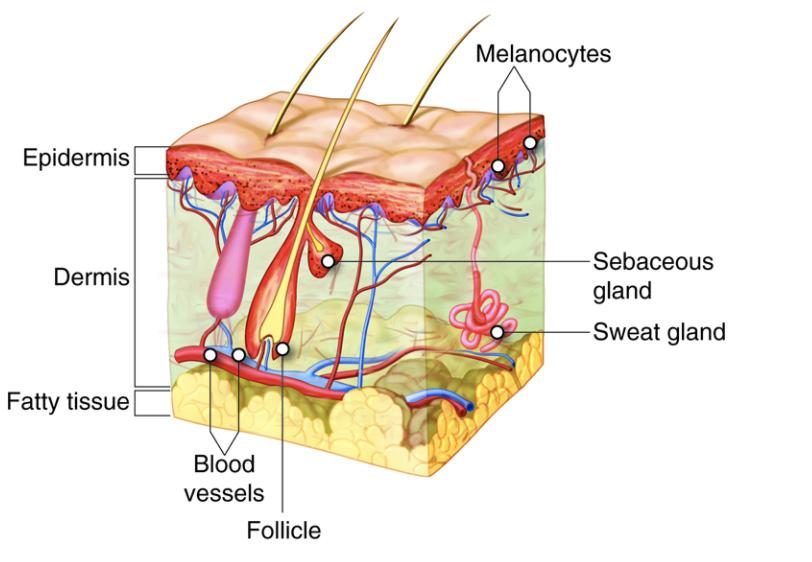
Source: Open Learning Initiative
Figure 3. Cyronics chambers at the Cryonics Institute. Source: Wikimedia Commons
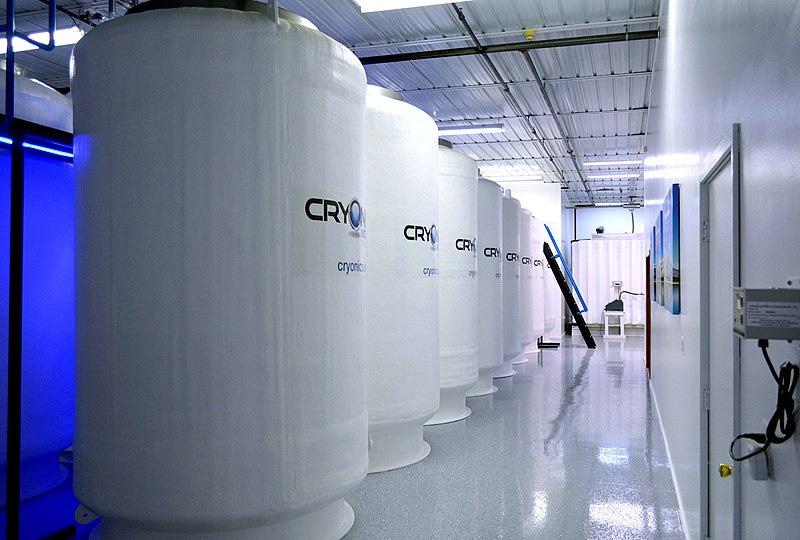
“Another reearch firm named AgeX is working on induced tissue regeneration that can harness the regenerative capability of human tissue and repair it.” Current Scientific and Commercial Research in Anti-Aging As of right now, there are no robust anti-aging medicines on the market that have yielded any substantial benefits for the human body. With that said, there is a lot of ongoing research in the field, as demonstrated by the examples that follow. In Brisbane, California, scientists at Unity Biotechnology are tackling the issue of cellular senescence. Senescence occurs when cells stop dividing further, causing age-associated illnesses like inflammation and degradation of tissues in the body and the extracellular environment that surrounds them. The researchers at Unity Biotechnology diligently remove these senescent cells from the bodies of their patients (Unity Biotechnology, n.d.). Cellular senescence is irreversible because there is currently no known process that can cause a senescent cell to regenerate further. Cellular senescence does serve a purpose – it is known to suppress cancer development at an early stage - but it also contributes to many age-related pathologies later in life (Campisi and Judith, 2013).
Another research firm named AgeX is working on induced tissue regeneration that can harness the regenerative capability of human tissue and repair it. This research looked at certain genes that changed their state from on or off at the time of loss of regenerative potential. They have identified gene COX7A1 as a marker for cells that have lost the regenerative potential and have subsequently formulated a technique to suppress the expression of this gene.(AgeX Therapeutics, n.d.).
Scientists at Cryonics are working to preserve one’s health by putting a corpse in cryo-sleep by cooling it to liquid nitrogen temperatures. This procedure is based on the idea that a recently deceased body still has the tissues intact. When the recently deceased body is put into cryosleep, it preserves the tissues (Cryonics Institute, 2013). Scientists hope that future scientific advancements have the potential to restore the body to a healthy state (Best, 2008). Meanwhile, CRISPR biomedical technology offers the ability to locate, edit, and manipulate DNA and, as a result, influence functions of any organism; this capability has accelerated biotechnology and medicinal research (Hsu et al., 2014).
Research into the phenomenon of hormesis has taken precedence in the field of anti-aging. These efforts rest on the principle that the functional integrity and life of a cell can be maintained without having to make radical alterations to the mechanism controlling its reproductive lifespan. In general, hormesis involves the regulated administration of microdoses of an otherwise harmful stimulus that has been shown to yield an increase in the lifespan of an organism (Rattan, 2004). The theory behind these microdoses is that a limited amount of stress for a controlled duration can cause a spike in the stress response of a cell, which facilitates tissue maintenance, and therefore counteracts the process of tissue aging (Rattan, 2004). The researchers at Sinclair Lab at Harvard Medical School are working with
Figure 4. Food and Drug Administration’s (FDA) Typical Drug Development and Approval Process
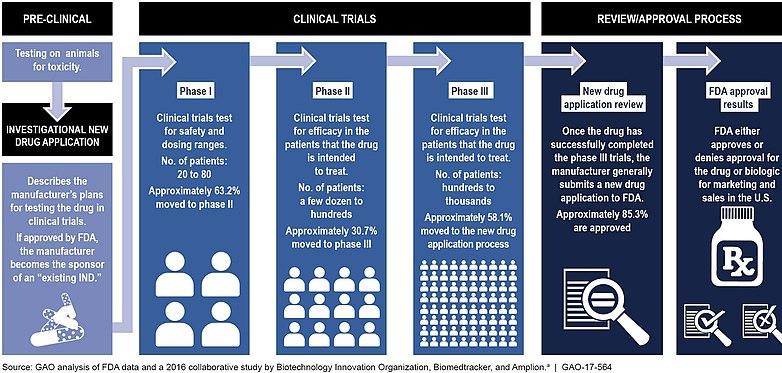
Source: Wikimedia Commons
Nicotinamide Adenine Dinucleotide (NAD+), which has presented itself as a promising molecule for hormesis therapy. It is observed that NAD+ concentration in the tissues gradually decreases as the human body ages. These researchers have used another compound Nicotinamide Mononucleotide abbreviated as NMN to synthesize NAD+ in the body and thus increase its concentration in the tissues. NMN is made from Vitamin B and is naturally occurring in our body. NMN is a precursor to NAD+ and promotes its production. NMN is sent to the cells through the small intestine and converted to NAD+ using the NMN transporter Slc12a8 (Sinclair Lab, n.d.).
Process of Regulatory Approval The field of anti-aging medicine is not devoid of false or misguided claims. One common example of this is the advice to take a high dosage of certain vitamins and hormones for anti-aging purposes, often without prescription from medical professionals. Companies are directly marketing these supplements mostly on the basis of their anti-aging properties, yet these approaches are neither data-driven nor sufficiently backed by scientific research. Although some of these techniques have been used to treat certain diseases in the body of the elderly, they do not alter the aging process as a whole.
Before a medical treatment can be put on the market, it must be approved by the US Food and Drug Administration (FDA). This process is rigorous and involves multiple steps, including discovery and research, pre-clinical trials, clinical trial, FDA review and FDA post-market safety assessment, which increases the cost and time needed to develop a new treatment. The FDA itself does not conduct trials for the drug, but oversees the validation process. The FDA also inspects the facilities where the drugs will be manufactured to ensure quality of production is maintained. Nevertheless, the approval process is important because it helps establish the effectiveness and validity of the treatment and helps consumers be aware of the risks involved (U.S. Food and Drug Administration).
Ethical Concerns Regarding AntiAging Research The topic of anti-aging treatments also provokes many ethical questions regarding the science and technology involved. One concern is the actual benefit of living longer in the first place - a longer life does not necessarily mean more years in good health. In addition to these concerns about the quality of life, a longer lifespan would also mean an added burden to the healthcare system (Juengst, 2013). That being said, many companies working in this field of research are working towards improving healthspan, (i.e. the number of years that a person lives a healthy life and without any chronic ailments), not just extending people’s lifespan (Crimmins, 2015). The subsequent ethical reasoning revolves around researches and companies adhering to FDA guidelines and do not leave out any necessary steps in the drug development process in order to cut costs or time required. Another ethical concern to be addressed is the widespread non-discriminatory access to any drugs or procedures that are developed.
Conclusion Biogerontologists have classified aging as the “One concern is the actual benefit of living longer in the first place - a longer life does not necessarily mean more years in good health.”
decline in molecular repair that leads to agerelated diseases. Factors like DNA mutation, inflammation, cellular senescence, and protein degradation determine the onset and the extent of age-related diseases. For the past few centuries, advances in medical science have increased average human life expectancy by nearly 50 years; this has led to an increasingly large elderly population, and at the same time, increased the incidence of pathology related to old age. Different groups of scientists follow varied approaches to tackle aging, including lengthening the healthspan to retracing and revitalizing organs functionality. This is a principle that anti-aging scientists ought to consider carefully when proposing new therapeutics – improving lifespan but neglecting to preserve or improve quality of life would be a negative development from both a humanitarian and an economic perspective.
References
Best, B. P. (2008, April). Scientific justification of cryonics practice. Rejuvenation research. https://www.ncbi.nlm.nih.gov/ pmc/articles/PMC4733321/.
The Case for Cryonics: Cryonics Institute. The Case for Cryonics | Cryonics Institute. https://www.cryonics.org/about-us/thecase-for-cryonics/.
Center for Drug Evaluation and Research. Drug Development & Approval Process. U.S. Food and Drug Administration. https:// www.fda.gov/drugs/development-approval-process-drugs.
Crimmins, E. M. (2015, November 10). Lifespan and Healthspan: Past, Present, and Promise. OUP Academic. https://academic. oup.com/gerontologist/article/55/6/901/2605490.
Hayflick, L. (2004, June 1). Aging: The Reality: "Anti-Aging" Is an Oxymoron. OUP Academic. https://doi.org/10.1093/ gerona/59.6.B573.
Hsu, P. D., Lander, E. S., & Zhang, F. (2014). Development and Applications of CRISPR-Cas9 for Genome ... https://www.cell. com/cell/fulltext/S0092-8674(14)00604-7.
Judith CampisiBuck Institute for Research on Aging, J. C. (2013). Aging, Cellular Senescence, and Cancer. Annual Reviews. https://www.annualreviews.org/doi/10.1146/annurevphysiol-030212-183653.
Juengst, E. T., Binstock, R. H., Mehlman, M. J., & Post, S. G. (2003, February 28). Antiaging Research and the Need for Public Dialogue. https://science.sciencemag.org/ content/299/5611/1323.full.
NE. Sharpless, R. A. D. P., C. Lopez-Otin, M. A. B., SE. Artandi, H. M. B., ME. Carlson, C. S., NS. Gavrilova, L. A. G., Rink, J. C., … MB. Schultz, D. A. S. (1970, January 1). Stem cells and anti-aging genes: double-edged sword-do the same job of life extension. Stem Cell Research & Therapy. https://doi.org/10.1186/s13287017-0746-4.
Research: The Sinclair Lab: Harvard Medical School, Department of Genetics. Research | The Sinclair Lab | Harvard Medical School, Department of Genetics. https://genetics.med. The Science – Unity Biotechnology. UnityBiotech. https:// unitybiotechnology.com/the-science/.
SI;, R. Aging, anti-aging, and hormesis. Mechanisms of ageing and development. https://pubmed.ncbi.nlm.nih. gov/15063104/.
Technology. AgeX Therapeutics. https://www.agexinc.com/ technology/.
Yohn , C., O'Brien, R., Lussier, S., Berridge, C., Ryan , R., Guermazi, A., … An, M. Senescent Synoviocytes in Knee Osteoarthritis Correlate with Disease Biomarkers, Synovitis, and Knee Pain. ACR Meeting Abstracts. https://acrabstracts. org/abstract/senescent-synoviocytes-in-knee-osteoarthritiscorrelate-with-disease-biomarkers-synovitis-and-knee-pain/.
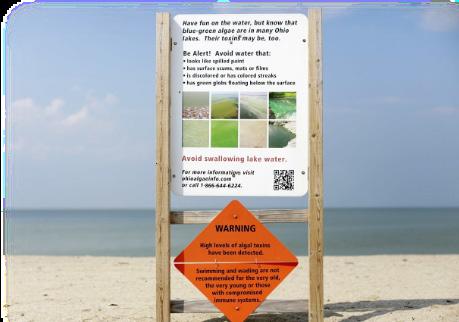
Algal Blooms and Phosphorus Loading in Lake Erie: Past, Present, and Future
BY BEN SCHELLING '21
Cover Photo: Lake Erie Beach Sign Source: Wikimedia Commons History
“You’re glumping the pond where the
Humming-Fish hummed!
No more can they hum, or their gills are all gummed. So I’m sending them off. Oh their future is dreary. They’ll walk on their fins and get woefully weary in search of some water that isn’t so smeary.
I hear things are just as bad up in Lake Erie” (Dr. Seuss, 1971)
Lake Erie has always held the reputation as the most polluted of the Great Lakes. One of the most infamous environmental disasters in history took place on the Cuyahoga River in Cleveland, Ohio. In June of 1969, the river was so polluted from the surrounding industrial city that it caught on fire, with flames reaching over five stories high (Ohio History Connection). While this was not the first time a fire broke out on the river (it has happened over a dozen times in recorded history), it did spark a movement that eventually led to the formation of the United States Environmental Protection Agency (EPA). While there has not been a fire on Lake Erie water since, there have been algal blooms, a much more complex environmental concern.
In the 1960s, the population along the coast of Lake Erie was growing and so was the pollution entering the lake. Laundry detergents with high phosphorus concentrations were common at this time as well. Along with the phosphorusrich detergent, tons of human sewage made its way into the lake, unnaturally increasing the phosphorus concentration (Hasler, 1969). The excess phosphorus increased the phytoplankton biomass on the lake (Burns and Ross, 1972). This process is called eutrophication. Eutrophic simply means a body of water with abundant nutrients to support
Figure 1: A map of the bathymetry of Lake Erie. Red areas represent shallow water and blue represent deep water. Note that the west-most basin is the shallowest
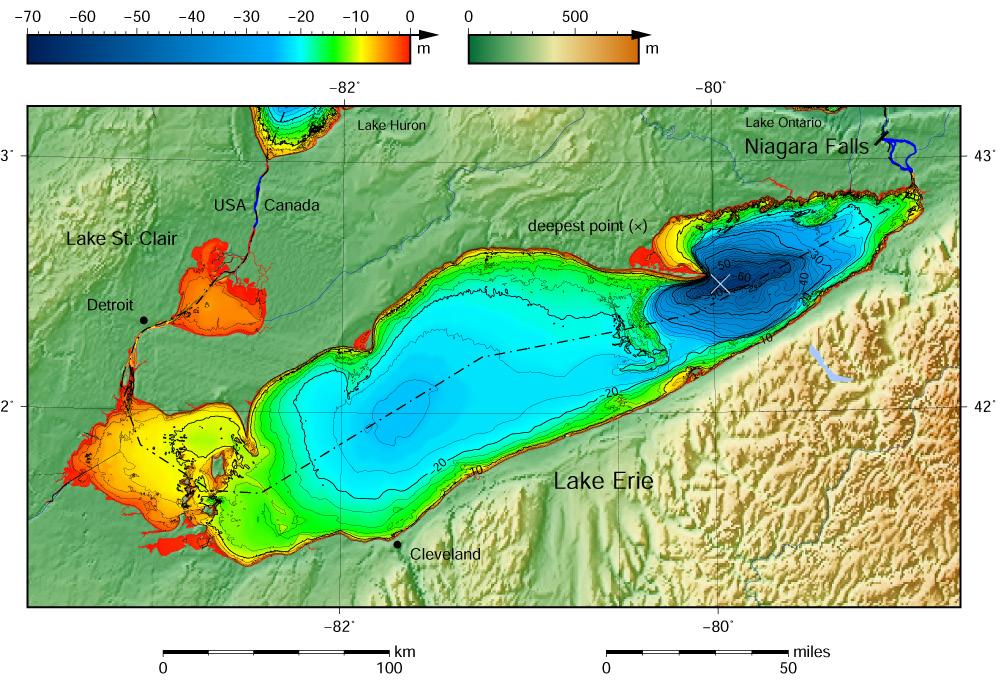
Source: NOAA, 2020
life within it. The shallower the lake, the more eutrophic (RMB Environmental Laboratories). This is because in shallow lakes the littoral zone, the portion of the lake where light can reach the bottom, is greater. When light reaches the bottom, photosynthetic life proliferates. Lake Erie is the shallowest of the Great Lakes, so it is the most susceptible to eutrophication. The western basin of the lake is extremely shallow, so it is even more susceptible than the rest of the lake (Figure 1). When the phytoplankton die, they sink to the bottom and decompose, which requires oxygen. When large masses of phytoplankton decompose, they require so much oxygen that they decrease the dissolved oxygen level of the lake and can create “dead zones”. Dead zones are portions of water that have such low dissolved oxygen concentrations that they cannot support life (NOAA, 2020).
Phosphorus loading is highlighted as a key driver of eutrophication. Unlike other nutrients, such as nitrogen, phosphorus has no gaseous cycle. Once phosphorus is in the water or sediments, it remains there much longer than other nutrients (Schindler, 1977). The low oxygen condition of Lake Erie caused a trophic cascade, and consequently, the abundance of benthic invertebrate species decreased. Mayfly nymphs, a benthic invertebrate, were once found at an abundance of 500 per square meter, but in the late 1960s, they were found in densities of only five per square meter, which is a 99% decrease (Hasler, 1969). At the same time, the populations of popular commercial fish such as herring, walleye, and blue pike were decreasing as well. Lake Erie’s reputation was so poor that reporters named it “the dead lake” (Ashworth, 1982). Notably, Dr. Suess mentions the lake’s poor health in his book The Lorax (Figure 2).
While popular media slammed Lake Erie, dedicated scientists worked hard to find the root of the issue and develop a solution. In 1969, Arthur Hasler published a compelling paper titled “Cultural Eutrophication is Reversible.” Hasler highlighted the main issue – the point source-pollution from detergents and sewage – and emphasized that, “It is of the greatest urgency to prevent further damage to water resources and to take corrective steps to reverse present damages.” In 1972, the newly formed EPA released an extensive report on the health of Lake Erie titled “Project Hypo”. Project Hypo investigated the causes of the low dissolved oxygen conditions in the lake’s hypolimnion (the deepest and coldest water) that caused the trophic cascade. The report suggests that if the phosphorus loading into the lake in 1970 was much smaller, that the total phytoplankton biomass in 1970 would have been much smaller as well (Noel and Ross, 1972).
Shortly after the publication of Project Hypo, the United States and Canadian governments jointly created the Great Lakes Water Quality Agreement (GLWQA), 1972. This agreement set a goal of 6.0 mg/L of O2 and limit of 1 mg/L of phosphorus. To achieve these goals, the agreement set a total annual limit of 11,000 metric tons (MT) of phosphorus discharge into “Unlike other nutrients, phosphorus has no gaseous cycle. Once phosphorus is in the water or sediments, it remains there much longer than other nutrients.”
Figure 2: The Lorax, written by Dr. Seuss in 1971, contains a verse about Lake Erie and its dismal water conditions:
"You're glumping the pond where the Humming-Fish hummed!
No more can they hum, for their gills are all glummed So I'm sending them off. Oh, their future is dreary They'll walk on their fins and get woefully weary in search of some water that isn't so smeary I hear things are just as bad up in Lake Erie."
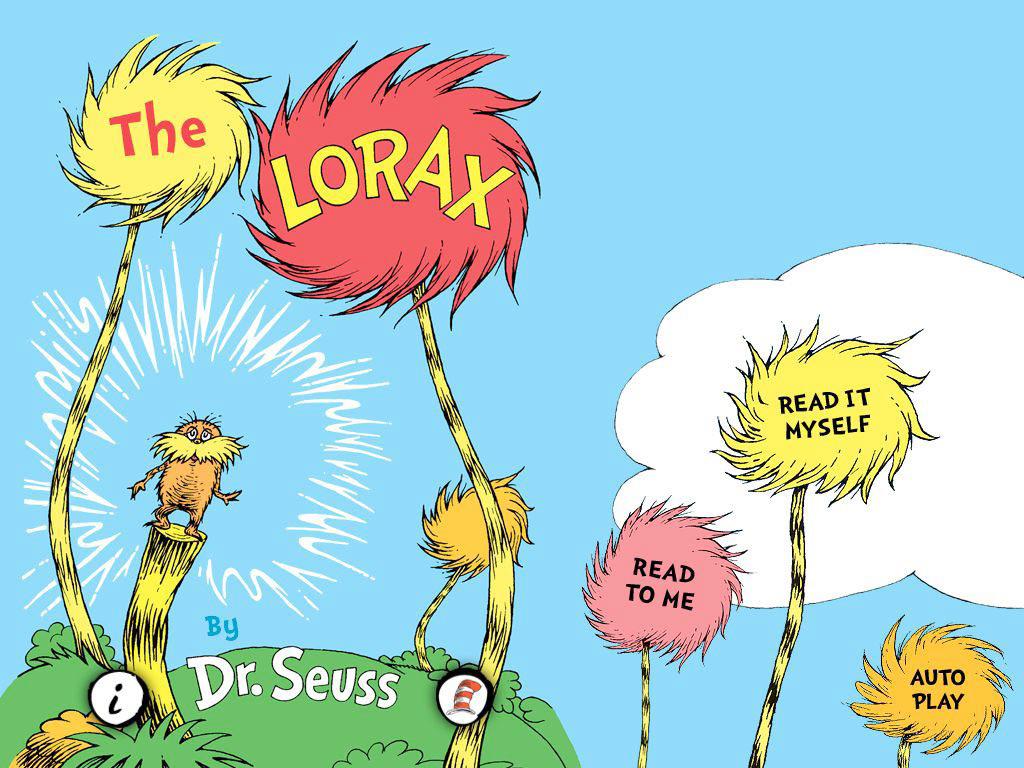
Source: Available under the Creative Commons License at Pixy.org; Book written by Dr. Seuss (1971)
Lake Erie. While the GLWQA of 1972 focused on point sources from sewage, the 1978 amendment also mandated the reduction of phosphorus heavy detergents (United States Government and Canadian Government, 1972). For the most part, the GLWQA was a success. From 1972 to 1975, the nearshore phytoplankton biomass decreased by 42% (Nicholls et al., 1977). This was the first evidence to suggest that Lake Erie was making a comeback. Eventually, the total annual phosphorus discharge into the lake fell below the 11,000 MT limit and the health of Lake Erie improved. An assessment of phosphorus and phytoplankton from 1970 to 1987 suggested, “cautious optimism on the phosphorusreduction program” Makarewicz and Bertram, 1991). Phosphorus levels were reduced from 15,260 MT per year to 2,445, an 84% reduction. Similarly, the phytoplankton biomass across the whole lake was reduced to 89% of its original size before the GLWQA (Makarewicz et al., 1993; Makarewicz and Bertram 1991). The water quality in the lake improved so much that Dr. Seuss decided to remove the portion of The Lorax that mentions poor ecosystem heath of Lake Erie (Figure 2; Fortner, 2019).
Soluble Reactive Phosphorus In the early 1990s, it seemed that the GLWQA had sufficiently reduced phosphorous loading. But as early as the mid 1990s, phytoplankton masses began to reappear (Bridgeman et al., 2013). On average, there was no increase in total phosphorus (TP), and the total annual discharge was under the 11,000 MT limit. So, what could have caused this increase in phytoplankton biomass? The answer is soluble reactive phosphorus (SRP).
Phosphorus is found in many different forms. The biggest distinction is between bioavailable phosphorus (soluble reactive phosphorus or “SRP”, sometimes called dissolved reactive phosphorus “DRP”) and non-bioavailable phosphorus (non-reactive phosphorus “NRP”). Bioavailable phosphorus is a form that is readily available for uptake by any organism. Nonbioavailable phosphorus, such as iron-bound phosphates, are transported as particles into the lake, settle in the sediment, and are never used by algae or bacteria (Hecky et al., 2004). In the 1990s, the fraction of SRP that made up TP increased (Figure 3).
Figure 3: The top figure displays total phosphorus (TP) discharged measured in metric tons (MT) from the Maumee River from 1991 to 2019. The red portion represents the non-reactivate phosphorus (NRP) and the blue represents the soluble reactive phosphorus (SRP). The bottom figure represents the ratio of soluble reactive phosphorus (SRP) to
total phosphorus (TP). Data is from National Center for Water Quality Research (Heidelberg University, 2020). Image created by author in JMP 14.2.0.
Dreissenid Mussels Around the time Lake Erie was making a comeback, invasive Dreissenid mussels were introduced into the great lakes from vessels in the Proto-Caspian Sea (Zhang et al., 2011). They were most likely transported in the ballast water; water contained on a ship to help control buoyancy. It is estimated that 80% of the total bottom of Lake Erie was colonized by the mussels by 1992 (Makarewicz et al., 2000). There are two types of Dreissenid mussels, zebra and quagga. Both species are benthic feeders and play a major role in the nutrient cycling within the lake.
Some organisms, such as Dreissenid mussels, can alter the chemistry of non-bioavailable phosphorus to a bioavailable form. This is a process known as nutrient remineralization: the transformation of particle nutrients (nonbioavailable) that have settled out of the water column into soluble forms (bioavailable) that can be transported through the water column and made available to growing algae and bacteria (Conroy et al., 2005). Dreissenid mussels can filter through 30% of the suspended matter each day, which is much faster than other organisms (Makarewicz et al., 2000). Benthic detritovores eat the feces of Dreissenid mussels which furthers the nutrient remineralization process and produces even more bioavailable phosphorus (Hecky et al., 2004).
The influence of Dreissenid mussels on a lake ecosystem depends on the physical conditions of the lake. In deeper water, Dreissenid mussels will decrease the SRP concentration, but in shallower water, they will enhance it (Steinmetz, 2015). A study of 61 lakes across Michigan found that lakes with more zebra mussels have a smaller population of phytoplankton because zebra mussels may graze on phytoplankton (Raikow et al., 2004). Interestingly, lakes with low nutrient concentrations showed an increase in Microcystis, a species of cyanobacteria, with an increase in zebra mussel abundance. This may be due to the anti-grazing properties of the Microcystis strain. Similarly, in the Saginaw Bay, the introduction of Dreissenid mussels led to an outbreak of Microcystis (Bierman et al., 2005). This highlights the complex relationship between invasive Dreissenid mussels and their Lake Erie meets the criteria, being the shallowest of the great lakes, for an ecosystem to increase SRP with the introduction of Dreissenid mussels. While TP levels decreased, SRP levels increased partially due to the colonization of invasive Dreissenid mussels in Lake Erie (Conroy et al., 2005; Hecky et al., 2004; Kleinman et al., 2015; Steinmetz 2015; Zhang et al., 2011).
Tillage Another explanation for the increase in SRP is the start of no till and conservation tillage. Tilling is the agricultural preparation of soil by mechanical agitations such as digging, stirring, and overturning. The 1983 revision of the GLWQA, focused on reducing TP and preventing erosion, suggests no till and conservation tillage as a method to reduce TP run-off and erosion (United States Government and Canadian Government, 1972). No till practices also increase biological activity in the soil, which leads to better soil structure and improves water storage (Ulén et al., 2010). In the Maumee and Sandusky watersheds (the watersheds that drain into the western basin of Lake Erie), half of corn and soybean farmers practiced conservation tillage (Richards et al., 2002). At first, this practice appeared to work as TP loads decreased. The no till practices were a major driver in reducing particulate phosphorus (another term for NRP) run off into the western basin of Lake Erie (Richards et al., 2009). While reducing TP run off, no till practices were also enhancing the SRP run off (Baker et al., 2017; Kleinman et al., 2011; Smith et al., 2015a; Ulén et al., 2010).
However, no till and conservation tillage practices allowed for phosphorus to vertically stratify in the soil (Baker et al., 2017; Duiker and Beegle 2006; Jarvie et al., 2017). Soil stratification is the process of soil particles separating based on their physical properties, resulting in visible banding. Phosphorus stratification concentrates SRP near the soil surface, which heightens the possibility of run off (Baker et al., 2017, Duiker and Beegle 2006). Another potential pathway for SRP run off is through macropores (Smith et al., 2015b). Macropores are large clumps of soil that SRP concentrates in. Reduced tillage systems promote the development of macropores. In the watersheds of Lake Erie’s western basin, no till practices decreased TP losses by 69%, but doubled the “Around the time Lake Erie was making a comeback, invasive Dreissenid mussels were introduced into the great lakes from vessels in the ProtoCaspian Sea...”
Figure 3: The top figure displays total phosphorus (TP) discharged measured in metric tons (MT) from the Maumee River from 1991 to 2019. The red portion represents the nonreactivate phosphorus (NRP) and the blue represents the soluble reactive phosphorus (SRP). The bottom figure represents the ratio of soluble reactive phosphorus (SRP) to total phosphorus (TP). Data is from National Center for Water Quality Research
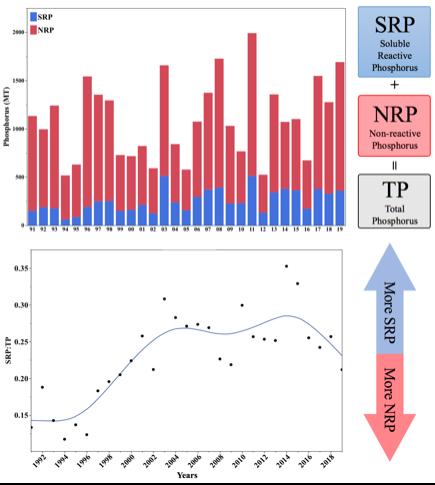
Source: Heidelberg University, 2020. Image created by author in JMP 14.2.0.
loss of SRP (Smith et al., 2015a). In Norway, a study found no till practices to reduce NRP run off but increase SRP run off by a factor of 4 (Ulén et al., 2010). Once thought of as a conservation practice to reduce phosphorus loading, reduced tillage provides a new phosphorus source that stimulated algal bloom growth into the new millennium.
Toledo Water Crisis From August 2nd to 4th, 2014, the water supply for the city of Toledo was contaminated. On August 2nd, at 2am, the governor of Ohio put out a no drink order on the water supply (WTOL, 2019). The water supply was contaminated with Microcystin, a toxin produced by the cyanobacteria Microcystis aeruginosa. When this is found at a concentration over 1 mg/L, the water is considered unsafe to drink (Kasich et al., 2016). Toledo was not prepared to supply 400,000 people with alternative drinking water. There was no secondary water supply for emergencies (Jetoo et al., 2015). By 12pm, the governor declared a state of emergency (WTOL, 2019). It would be three whole days before the water in Toledo was safe to drink again.
The most common freshwater bloom-forming cyanobacteria is Microcystis aeruginosa. M. aeruginosa releases a toxin called microcystin that has many adverse health effects. Exposure to microcystin during recreational activities can lead to acute illness causing abdominal pain, headache, sore throat, vomiting, diarrhea, blistering around the mouth, and pneumonia (United States EPA, 2020a). Ingestion though drinking water can cause more serious health effects such as liver or kidney failure and may even increase the risk of ALS (Banack et al., 2015). While microcystin poisoning is rare, it can cause death. Dogs that encounter
contaminated water are at high risk, and there are many reported deaths due to M. aeruginosa ingestion in dogs (Stewart et al., 2008). There are reports of human and dog illnesses caused by the toxin in 50 countries and 27 states (United States EPA, 2020a).
While M. aeruginosa naturally occurs in freshwater systems, the high abundance seen today is almost entirely anthropogenic, or due to human activity. Similar to other phytoplankton, an unnatural amount of phosphorus can promote excessive growth (Haney and Ikawa 2001; Michalak et al., 2013). Increasing water temperatures as a result of climate change also promotes M. aeruginosa growth (Liu et al., 2011). When water temperatures are higher for longer periods of time, lake stratification is more pronounced for longer. Lake stratification is when the lake is separated into different temperature zones. When the air is warm enough, the top layer of the lake warms while the bottom remains cold. This separation reduces mixing between temperature zones, allowing for M. aeruginosa, which is more buoyant than water, to ascend to the surface where blooms form (Paerl and Huisman, 2009; Wood et al., 2017). Higher atmospheric CO2 concentrations are also advantageous for M. aeruginosa growth. M. aeruginosa displays phenotypic plasticity; a change in morphology, behavior, or physiology based on environmental conditions. The phenotypic plasticity of M. aeruginosa allows it to fix carbon from the atmosphere at a greater rate than other aquatic organisms (Ji et al., 2020).
Current Legislation After the 2014 Toledo water crisis, the state of Ohio created new legislation to prevent a similar event in the future. The first legislation passed was Senate Bill 150. This bill requires certification by the Ohio Department of Agriculture (DOA) for anyone who applies fertilizer to 50 acres of land (Cera et al., 2014). The certified card holder must record the type of fertilizer being applied and who is applying it, as well as the time, place, and rate of application. This information must be available for audits by DOA. Currently, Ohio is the only state with legislation of this kind (Snyder, 2018). Violation of the law can result in the loss of fertilizer registration or refusal to register someone for fertilizer application. This law was made effective on August 21, 2014. The second legislation was Senate Bill 1, effective July 3, 2015. This bill set restrictions on the fertilizer application methods to reduce the SRP discharge into the lake. Senate Bill 1 states that no person in the watershed of the western basin of Lake Erie may apply fertilizer or manure on snow-covered or frozen soil, if the top two inches of soil are saturated from precipitation, or if the weather forecast calls for greater than 50% chance of precipitation exceeding 1 inch in a 12 hour period (Gardner and Peterson, 2015). It may seem odd, but December often has the greatest SRP loading of any month (Figure 4). The average discharge from the Maumee River varied significantly by month for TP (Figure 4: top, ANOVA: P<0.0001, F1,12=14.38) and for SRP (Figure 4: bottom, ANOVA: P<0.0001, F1,12=12.03). According to Dr. Baker, it is important to avoid winter application for two reasons. The first reason is that SRP is concentrated at the surface from no till practices and the crop residue that is left to decompose at the surface. Crop residue is the plant biomass that remains in the ground after a harvest, and it is often made up of the stems of plants. The second reason that Dr. Baker highlights is that the rainfall in December is elevated, causing large discharges of water carrying SRP.
Senate Bill 1 also mandates that fertilizer and manure must be injected into the ground as opposed to broadcast application, where the fertilizer is applied to the soil surface. According to Dr. Baker, injection prevents phosphorus run off in two ways. First, it reduces the buildup of phosphorus at the surface that is already enhanced by phosphorus stratification. This type of phosphorus loss is known as an acute loss; the loss of phosphorus from immediate rainfall before it interacts with the soil. The second reason is the physical intrusion of injection disrupts the formation of macropores that act as a “pipeline” for SRP to travel from agriculture to the water. This type of phosphorus loss is known as a chronic loss; the loss of phosphorus from the soil itself.
The GLWQA saw great success in only a matter of years, so should we expect the 2015 Senate Bill 1 to cause rapid change as well? By using phosphorus data from 2000 to 2019, there is not a significant difference before and after the legislation for TP (ANOVA: P=0.26, F1,12=1.28) or for SRP (ANOVA: P=0.40, F1,12,=0.71). Before Senate Bill 1, the average TP load by month was 89.6 MT and after it is 108 MT (Figure 5). Before the average SRP load by month was 24.4 MT, “After the 2014 Toledo water criis, the state of Ohio created new legislation to prevent a similar event in the future.”
Figure 4: The average phosphorus loads into the Maumee River by month from 2000-2019. The top displays TP and the bottom displays SRP, both are measured in MT. Error bars represent 1 SE around the mean. Data is from National Center for Water Quality Research
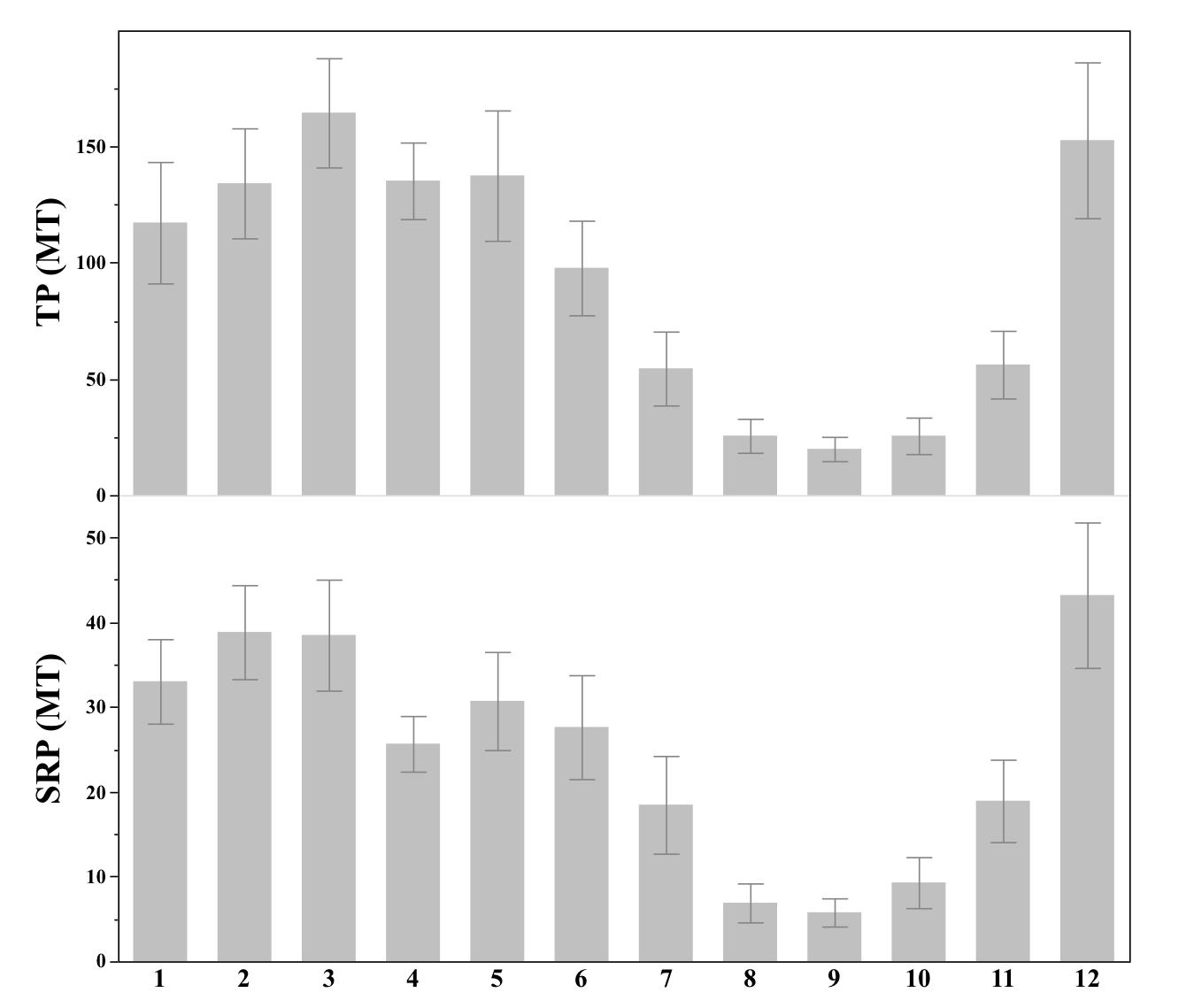
Source: Heidelberg University, 2020. Image created by author in JMP 14.2.0.
after it is 26 MT (Figure 5).
Does this mean that Ohio Senate Bill 1 was a failure? It is hard to say. Agricultural practices are not the only factors influencing run off. The weather has a large impact on the amount of phosphorus discharge. For example, the phosphorus loads in 2019 were extremely large (Figure 3). This is not a result of farmers violating the law. Rather, as suggested by Dr. Baker, it was the result of an unusual amount of rainfall in 2018 and 2019. The large amount of rain washed loads of phosphorus into the lake. While a large load, the SRP levels were one third less than expected from this amount of rainfall. Dr. Baker predicts that this is because farmers had fewer opportunities to apply fertilizer due to the heavy rain. The result of the high loads in 2019 was one of the largest blooms on record. It was so large that it covered an area six times the size of Cleveland and could be seen from space (Johnston, 2019). Although it was before the 2015 legislation, the record-setting 2011 bloom was also a result of extreme meteorological events and long-term agricultural practices, not short-term phosphorus loads that year (Michalak et al., 2013). The 2011 bloom biomass reached a size of 40,000 MT and the 2019 bloom biomass reached a predicted 46,300 MT, topping the previous (Obenour et al., 2014; Scavia et al., 2019). The 2014 bloom was only 22,000 MT, less than half that of 2011 and 2019. Neither the 2011 nor the 2019 bloom caused a water crisis like the much smaller 2014 bloom.
Toledo was unlucky in 2014. The bloom formed around the intake for the water treatment plant. The M. aeruginosa rich water that collected in the Maumee Bay was blown east on the southern shoreline and past the Toledo water intake (Steffen et al., 2017). This assessment of the 2014 crisis is correct according to Dr. Baker. He also notes that, along with prevailing winds and biomass movement at the surface, the location of the mass within the water column contributed to the drinking water contamination. The water treatment plant intake is not at the surface, where blooms are most prevalent. When it is windy, as it was in August 2014, the bloom is stirred up and brought down to the intake.
Research suggests a viral outbreak among the M. aeruginosa population in the bay, causing an unusual toxin concentration (Erickson 2017, Steffen et al., 2017). A DNA analysis of the 2014 bloom reveals that a virus was spreading between the M. aeruginosa, causing the cells to lyse, or break open. The lysing of the cells released an unusual amount of microcystin toxin into the water, elevating the toxin concentration entering the water intake (Steffen et al., 2017). While the media labels this crisis as “unlucky”, the combination of winds
Figure 5: The average monthly phosphorus loading for the Maumee River, 2000-2019 (top: TP, bottom: SRP) before and after the passing of Ohio Senate Bill 1 in 2015. The error bars represent 1 SE from the mean and the values reported are the means. Data is from National Center for Water Quality Research
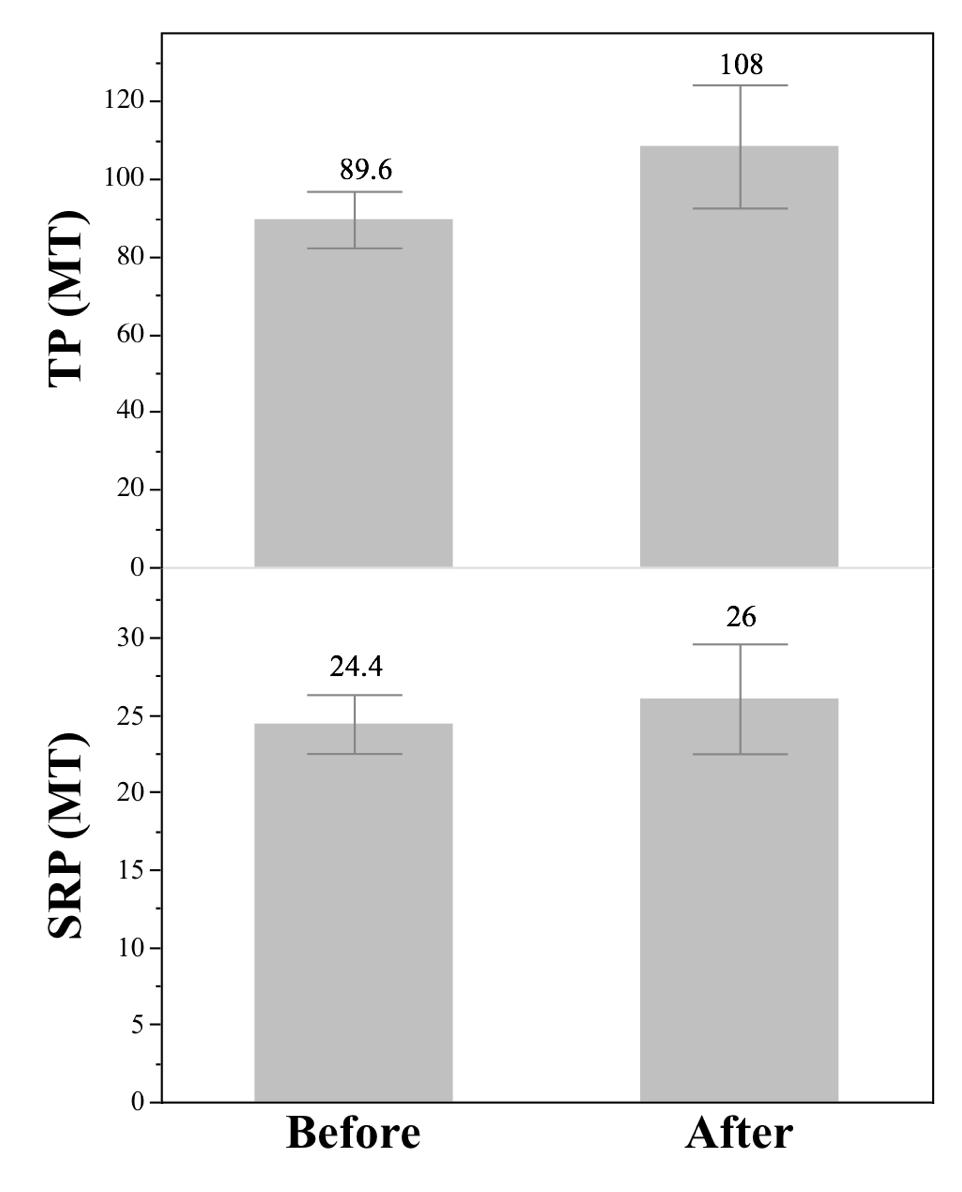
Source: Heidelberg University, 2020. Image created by author in JMP 14.2.0.
and viral outbreak is likely to happen again.
The question still remains: was Ohio Senate Bill 1 successful at reducing the risk of water contamination? The answer is not as clear as the success of the GLWQA, which showed relatively rapid results. This is an understandable difference since reducing point source pollution is simpler than non-point source pollution. Ohio Senate Bill 1 may be the best mitigation tactic given what is known. Unfortunately, the most influential factor impacting bloom formation is one that cannot be regulated by the law – it is governed by weather. The frequency of extreme weather events is currently much greater than it used to be, increasing the chances of another crisis. Therefore, a comparison between the two legislations is unfair. Today, it is an entirely different ballgame.
Future Legislation It is important to remember what is at stake when considering future policies for Lake Erie. There is a $15.1 billion dollar tourism industry on the coast of Lake Erie, most of which is concentrated in the western basin (Briscoe, 2019). The closure of beaches due to health concerns from algal blooms hurts this extremely lucrative tourism industry that many Ohioans rely on. More importantly, algal blooms threaten the water supply for over 11 million people (United States EPA, 2020b). The 2014 water crisis contaminated the water supply of over 400,000 people and cost an estimated $65 million (NOAA, 2015). The cost of damage from algal blooms is great but the potential health crisis from contaminating the water is much greater. In general, public health is most important. If scientists have identified agriculture as the major source of phosphorus causing blooms, then why don’t we eliminate the pollution source entirely (Briscoe, 2019)? Why did the state of Ohio not ban the use of fertilizers if public health is the number one priority? It is not that simple. The Ohio agriculture industry is massive. There are over 75,000 farms in Ohio, contributing $105 billion to the annual state economy and directly accounting for 13% of the state’s total business (Ohio Department of Agriculture, 2018). A law that completely restricts fertilizer application would destroy this massive industry, leaving thousands unemployed. The Ohio economy would take a huge hit, and the absence of products from Ohio’s agriculture industry to in-state and out-of-state markets would be disastrous. While public health still comes before economics, the downfalls of eliminating fertilizer application are too great to ignore. The solution must protect clean drinking water supplies without a major disruption to the largest Ohio industry. This is what Senate “The 2014 water crisis contaminated the water supply of over 400,000 people and cost an estimated $65 million.”
Bill 1 has started to do. There are restrictions on application methods to reduce drinking water contamination concerns, while still allowing the agriculture industry to move forward.
There are still possibilities for new policies that satisfy public health and the agriculture industry. One possibility is the improvement of riparian zones. Riparian zones are vegetation areas that act as buffers between agriculture run off and waterways. They have been successful in reducing phosphorus run off (Jaynes et al., 2014). So, why have we not increased the abundance and size of these buffer zones? Riparian buffers do not catch the run off using the current tile drainage system that is in place for the majority of Ohio farms. Tile drainage is a drainage system that collects excess water underground which is then transported though pipes that bypass the important riparian buffer zones. Currently, 49% of Ohio farms use tile drainage systems, which is much greater than the 14% national average (Zulauf and Brown, 2019). One possible solution is to redirect the drainage systems so the riparian buffers can catch excess nutrients. A tile drainage redirection method has been developed in Iowa and has proven to catch all nitrate runoff (Jaynes et al., 2014; Jaynes and Isenhart 2014). While this has not been proven to work for phosphorus run off, it is likely to do so, since riparian zones generally reduce all types of run off. This is an excellent opportunity for future research and has great promise for reducing phosphorus run off in Ohio.
The most promising opportunity to decrease Ohio run off, that was not mentioned in any current legislation, are blind inlets. Blind inlets are mat drainage structures that act as a buffer in the lowest point of a field. This is a buffer that interferes with water flow before it enters a drainage pipe or passes through a riparian buffer zone. Unlike the little research that has been done on tile drainage redirection, there has been significant research on blind inlets documenting the reductions in phosphorus loading in comparison to tile drainage methods. Compared to tile drainage, blind inlets can reduce TP loads by 60-71.9% and SRP by 50-79.4% (Feyereisen et al., 2015; Smith et al., 2015b; Smith and Livingston 2013). These numbers are extremely promising and the implementation of these drainage systems, while a significant structural change, is relatively simple. A combination of eliminating tile drainage, allowing for passage of water through riparian buffers and the inclusion of blind inlets is the ideal next step in reducing run off while supporting Ohio farms (See Table 1 for a breakdown of the potential improvements to Ohio agriculture legislation).
Statistical Methods The phosphorus data was retrieved form the National Center For Water Quality Research for the Maumee River (Heidelberg University, 2020). Negative concentrations and discharges were excluded from the data because they make no physical sense. All tests were performed on Log(X+1) transformed data. All statistics were done on JMP Pro 14.2.0.
Acknowledgements Special thanks to Doctor David Baker, a professor at Heidelberg University. Dr. Baker has 72 publications and has been cited more than 3,000 times. He has also been at the forefront of research on algal blooms and phosphorus loading in Lake Erie since the 1960s. His professional and informed opinions appear throughout the text.
Thank you to Melissa Desiervo, a PHD candidate at Dartmouth College in the Ecology, Evolution, Environment and Society graduate program. Melissa advised the statistical analysis.
This paper would not be possible without the general advising of Professor Michael Cox, Dartmouth College. Your guidance is greatly appreciated.
Key Terms Bio-available: The form of a nutrient that is available for a living organism to use. This is when the nutrient is not bound to an organic compound.
Dreissenid mussels: A type of mussel (zebra and quagga) that are from the Proto-Caspian Sea. They are invasive in Lake Erie and alter aquatic phosphorus cycling.
Eutrophication: The excessive growth of algae in a body of water due to an oversaturation of nutrients.
Great Lakes Water Quality Agreement (GLWQA): The 1972 agreement between the United States and Canadian governments that sets phosphorus loading limits and targets the reduction of point source pollution.
Macropores: Relatively large clusters of soil that can form from no till or conservation tillage.
Microcystin: The toxin that is produced by many cyanobacteria, most notably Microcystis aeruginosa.
Microcystis aeruginosa: This is the scientific name for the cyanobacteria that dominate the recent algal blooms in Lake Erie.
Mineralization: The decomposition of chemical compounds in organic matter, releasing nutrients in a soluble inorganic form that is bioavailable.
Trophic Cascade: Powerful indirect interactions that can control an entire ecosystem when a trophic level in a food web is suppressed.
Non-Reactive Phosphorus (NRP): The portion of TP that is non-bioavailable.
Ohio Senate Bill 1: Addresses agricultural regulations and application of fertilizer. Establishes Lake Erie water quality protections.
Ohio Senate Bill 150: Sets the reequipment of a permit to anyone who applies fertilizer to a plot of land greater than 50 acres.
Phytoplankton: All aquatic autotrophic life between 60 and 50 microns in length.
Stratification
Soil Stratification: The separation of soil types by their physical properties resulting in abrupt porosity changes at various depths.
Phosphorus Stratification: The accumulation of phosphorus at the soil surface where it can easily be transported by precipitation into a water source.
Lake Stratification: The separation of water into three layers based on temperature and density: Epilimnion (shallowest layer, warmest, least dense), Metalimnion (middle layer), and Hypolimnion (deepest layer, coldest, most dense).
Soluble Reactive Phosphorus (SRP): Also called dissolved reactive phosphorus (DRP), is the portion of TP that is bioavailable.
Tillage: The agricultural preparation of soil by mechanical agitation such as digging, stirring, and overturning. Conservation tillage: The practice of reducing the amount of tilling.
References
Ashworth, W. (1982). The Late, Great Lakes: An Environmental History.
Baker, D., Johnson, L., & Confesor, R. (2017). Vertical Stratification of Soil Phosphorus as a Concern for Dissolved Phosphorus Runoff in the Lake Erie Basin. Journal of Environmental Quality, 46, 1287–1295.
Banack, S., Caller, T., & Henegan, P. (2015). Detection of Cyanotoxins, β-N-methylamino-L-alanine and Microcystins, from a Lake Surrounded by Cases of Amyotrophic Lateral Sclerosis. Toxins, 7, 322–336.
Bierman, V., Kaur, J., & DePinto, J. (2005). Modeling the Role of Zebra Mussels in the Proliferation of Blue-green Algae in Saginaw Bay, Lake Huron. Journal of Great Lakes Research, 31, 32–55.
Bridgeman, T., Chaffin, J., & Filbrun, J. (2013). A novel method for tracking western Lake Erie Microcystis blooms, 2002–2011. Journal of Great Lakes Research, 39, 83–89.
Briscoe, T. (2019). The shallowest Great Lake provides drinking water for more people than any other. Algae blooms are making it toxic — and it’s getting worse. Chicago Tribute.
Burns, N., & Ross, C. (1972). Project Hypo: An Intensive Study of the Lake Erie Central Basin Hypolimnion and Related Surface Water Phenomena. United States Environmental Protection Agency. To revise the law governing the abatement of agricultural pollution, to require a person that applies fertilizer for the purposes of agricultural production to be certified to do so by the Director of Agriculture, to make other changes to the Agricultural Additives, Lime, and Fertilizer Law., no. 150 (2014).
Conroy, J., Edwards, W., & Pontius, R. (2005). Soluble nitrogen and phosphorus excretion of exotic freshwater mussels (Dreissena spp.): potential impacts for nutrient remineralisation in western Lake Erie. Freshwater Biology, 50, 1146–1162.
Dr. Suess. (1971). The Lorax.
Duiker, S., & Beegle, D. (2006). Soil fertility distributions in longterm no-till, chisel/disk and moldboard plow/disk systems. Soil and Tillage Research, 88, 30–41.
Erickson, J. (2017). Virus infection may be linked to 2014 Toledo water crisis. Michigan News.
Feyereisen, G., Francesconi, W., & Smith, D. (2015). Effect of Replacing Surface Inlets with Blind or Gravel Inlets on Sediment and Phosphorus Subsurface Drainage Losses. Journal of Environmental Quality, 44, 594–604.
Fortner, R. (2019). There’s Nothing Smeary About Lake Erie Anymore. Ohio Sea Grant.Addresses agricultural regulations and application of fertilizer, no. 1 (2015).
Haney, J., & Ikawa. (2001). A Survey of 50 NH Lakes for Microcystins (MCs). University of New Hampshire Scholar’s Repository, 127.
Hecky, R. E., Smith, R. E. H., & Barton, D. R. (2004). The nearshore phosphorus shunt: a consequence of ecosystem engineering by dreissenids in the Laurentian Great Lakes. He Canadian Journal of Fisheries and Aquatic Sciences, 61, 1285–1293.
Heidelberg University. (2020). National Center for Water Quality Research. Tributary Data Download. Jarvie, H., Johnson, L., & Sharpley, A. (2017). Increased Soluble Phosphorus Loads to Lake Erie: Unintended Consequences of Conservation Practices? Journal of Environmental Quality, 46, 123–132.
Jaynes, D., & Isenhart, T. (2014). Reconnecting Tile Drainage to Riparian Buffer Hydrology for Enhanced Nitrate Removal. Journal of Environmental Quality, 43, 631–638.
Jaynes, D., Isenhart, T., & Parkin, T. (2014). Reconnecting riparian buffers with tile drainage (2). Leopold Center Completed Grant Reports.
Jetoo, S., Grover, V., & Krantzberg, G. (2015). The Toledo Drinking Water Advisory: Suggested Application of the Water Safety Planning Approach. Sustainability, 7, 9787–9808.
Ji, X., Verspagen, J., & Van de Waal, D. (2020). Phenotypic plasticity of carbon fixation stimulates cyanobacterial blooms at elevated CO2. Science Advances.
Johnston, L. (2019). How big did the 2019 Lake Erie harmful algal bloom get? See the progression. Cleveland.Com. https:// www.cleveland.com/news/g66l-2019/09/204be17826609/ how-big-did-the-2019-lake-erie-harmful-algal-bloom-get-seethe-progression.html
Kasich, J., Taylor, M., & Butler, G. (2016). Public Water System Harmful Algal Bloom Response Strategy. Ohio Environmental Protection Agency.
Kleinman, P., Sharpley, A., & Johnson, L. (2015). Implementing agricultural phosphorus science and management to combat eutrophication. AMBIO, 44, 293–310.
Liu, X., Lu, X., & Chen. (2011). The effects of temperature and nutrient ratios on Microcystis blooms in Lake Taihu, China: An 11-year investigation. Harmful Algae, 10, 337–343.
Makarewicz, J. (1993). Phytoplankton Biomass and Species Composition In Lake Erie, 1970 to 1987. Journal of Great Lakes Res., 19(2).
Makarewicz, J., & Bertram, P. (1991). Evidence for the Restoration of the Lake Erie Ecosystem. Oxford University Press, 41(4), 216–223.
Makarewicz, J., Bertram, P., & Lewis, T. (2000). Chemistry of the Offshore Surface Waters of Lake Erie: Pre- and Post-Dreissena Introduction (1983-1993). Journal of Great Lakes Research, 21(1), 82–93.
Michalak, A., Anderson, E., & Chaffin, J. (2013). Recordsetting algal bloom in Lake Erie caused by agricultural and meteorological trends consistent with expected future conditions. PNAS, 110(16), 644–6462.
National Oceanic and Atmospheric Administration. (n.d.). Bathymetry of Lake Erie & Lake Saint Clair. https://www.ngdc. noaa.gov/mgg/greatlakes/erie.html
National Oceanic and Atmospheric Administration. (2015). National Oceanic and Atmospheric Administration. (2020). What is a Dead Zone?https://oceanservice.noaa.gov/facts/ deadzone.html
Nicholls, K. H., Standen, D. W., Hopkins, G. J., & Carney, E. C. (1977). Declines in the Near-Shore Phytoplankton of Lake Erie’s Wester Basin Since 1971. Journal of Great Lakes Res, 3, 72–78.
Obenour, D., Gronewold, D., & Stow, C. (2014). 2014 Lake Erie Harmful Algal Bloom (HAB) Experimental Forecast: This product represents the first year of an experimental forecast relating bloom size to total phosphorus load.
Ohio Department of Agriculture. (2018). Ohio Agriculture. Farm Flavor. https://www.farmflavor.com/ohio-agriculture/
Ohio History Connection. (n.d.). Cuyahoga River Fire. Ohio History Central. https://ohiohistorycentral.org/w/Cuyahoga_ River_Fire
Paerl, H., & Huisman, J. (2009). Climate change: a catalyst for global expansion of harmful cyanobacterial blooms. Environmental Microbiology Reports, 1(1), 27–37.
Raikow, D., Sarnelle, O., & Wilson, A. (2004). Dominance of the noxious cyanobacterium Microcystis aeruginosa in low-nutrient lakes is associated with exotic zebra mussels. Limnology and Oceanography, 49(2), 482–487.
Richards, Baker, D., & Crumrine, J. P. (2009). Improved water quality in Ohio tributaries to Lake Erie: A consequence of conservation practices. Journal of Soil and Water Conservation, 64(3).
Richards, R. P., Baker, D., & Eckert, D. (2002). Trends in Agriculture in the LEASEQ Watersheds, 1975–1995. Journal of Environmental Quality, 31.
RMB Environmental Laboratories Inc. (n.d.). Lake Eutrophication. Lakes Monitering Program. https://www. rmbel.info/primer/lake-eutrophication/
Scavia, D., Manning, N., & Bertani, I. (2019). 2019 Western Lake Erie Harmful Algal Bloom (HAB) Forecast.
Schindler, D. W. (1977). Evolution of Phosphorus Limitation in Lakes: Natural Mechanisms Compensate for Deficiencies of Nitrogen and Carbon in Eutrophied lakes. Sicence, 195, 260–262. Smith, D., Francesconi, W., & Livingston, S. (2015a). Phosphorus losses from monitored fields with conservation practices in the Lake Erie Basin, USA. AMBIO, 22, 319–331.
Smith, D., King, K., & Johnson, L. (2015b). Surface Runoff and Tile Drainage Transport of Phosphorus in the Midwestern United States. Journal of Environmental Quality, 44, 495–502.
Smith, D., & Livingston, S. J. (2013). Managing farmed closed depressional areas using blind inlets to minimize phosphorus and nitrogen losses. Soil Use and Management, 29, 94–102.
Snyder, L. (2018). Overview: Senate Bill 150, Senate Bill 1. Together with Farmers.
Steffen, Davis, T., & Bullerjahn, G. (2017). Ecophysiological Examination of the Lake Erie Microcystis Bloom in 2014: Linkages between Biology and the Water Supply Shutdown of Toledo, OH. Environmental Science and Technology, 51, 6745–6755.
Steinmetz, M. (2015). Dreissenid Mussels Impact on Phosphorus Levels in the Laurentian Great Lakes. The Duluth Journal of Undergraduate Biology.
Stewart, I., Seawright, A., & Shaw, G. (2008). Cyanobacterial poisoning in livestock, wild mammals and birds – an overview.
Ulén, B., Aronsson, H., & Bechmann, M. (2010). Soil tillage methods to control phosphorus loss and potential sideeffects: a Scandinavian review. Soil Use and Management, 26, 94–107.
United States Environmental Protection Agency. (2020a). Human Health Effects Caused by the Most Common Toxinproducing Cyanobacteria. Health Effects from Cyanotoxins.
United States Environmental Protection Agency. (2020b). Lake Erie [Government]. The Great Lakes. https://www.epa.gov/ greatlakes/lake-erie
United States Government, & Canadian Government. (1972). The Great Lakes Water Quality. Wood, S., Borges, H., & Puddick, J. (2017). Contrasting cyanobacterial communities and microcystin concentrations in summers with extreme weather events: insights into potential effects of climate change. Erschienen in: Hydrobiologia, 785(71–89).
WTOL Staff. (2019). 5 years since the Toledo water crisis: A timeline of what happened. WTOL 11. https://www.wtol. com/article/news/local/protecting-our-water/5-years-sincethe-toledo-water-crisis-a-timeline-of-what-happened/51271a2414b-a34d-4b4a-9632-58e1c212d098
Zhang, H., Culver, D., & Boegman. (2011). Dreissenids in Lake Erie: an algal filter or a fertilizer? Aquatic Invasions, 6(2), 175–194.
Zulauf, C., & Brown, B. (2019). Use of Tile, 2017 US Census of Agriculture. Farm Doc Daily.
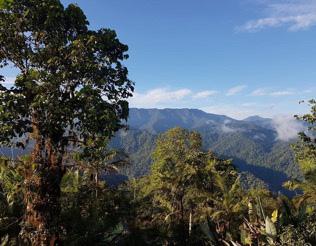
BY BETHANY CLARKSON, UNIVERSITY OF LINCOLN (UK) GRADUATE
Cover Image: A snapshot of the Santa Lucia Cloud Forest Reserve. This image was captured at the Santa Lucia Ecolodge, GPS coordinates N00°8.193 W078°36.458.
Photograph captured by author.
Abstract Specialized rewards with known antimicrobials such as resin are being increasingly used to develop understanding of plant-pollinator relationships, though the knowledge of how this varies between male and female dioecious plants, such as Cluisa, is still somewhat limited. This research, carried out at the Santa Lucia cloud forest reserve in Ecuador on a Clusia sp., aimed to explore differences between microbial growth on male and female Clusia sp. flowers. In total, 16 flowers were collected and stored in 100ml of sterile water for 60 minutes so that any antimicrobial compounds would infuse the water. The infusion was then used for further testing. Spread plating, Petri film culturing, and biochemical testing were performed on sixteen samples from four different Clusia plants (two males and two females) using a mixture of open flowers and young buds. Microbial investigation revealed that female flowers yielded a lower density and diversity of bacterial and fungal colonies present on Petri films than male flowers. Noticeably, there was a significant decrease in the number of fungal colonies present on female flowers. A possible reason is that female flowers have a shorter lifespan, and therefore support increased antimicrobial activity to make themselves more attractive to possible pollinators.
Keywords Pollinator reward systems, Clusia, Ecuador, Antimicrobial, Resin, Bees.
Introduction Antibiotic resistance is not only on the rise, but has been named “the greatest risk to public health” (Kumarasamy et al., 2010); antibioticresistant infections are projected to be responsible for an estimated 10 million deaths by 2050 (Davies et al., 2013). With increasing
Figure 1: A makeshift laboratory within the Ecolodge. This table was where most of the work was carried out. Equipment includes disposable 5ml pipettes, sterile water, ethanol, pH testing strips and samples of Clusia sp. flowers suspended in sterile water.
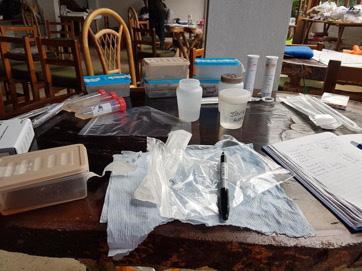
Photograph captured by author.
resistance comes a rising urgency to not only find new sources of antibiotics but also to make antibiotic treatment as efficacious as possible. Current research explores many potential sources of antimicrobials, from cockroaches to deep-sea fungi (Zhang et al., 2013; Ali et al., 2018). Plants are a common source of antimicrobial compounds; ongoing research shows plant species including Calamus aromaticus, Croton lechleri, and many others possess significant antimicrobial potential. Therefore, research into understanding these specimens is of the utmost importance (Salam and Quave, 2018; Khan et al., 2018). Indeed, a study by Kumar et al. (2006) on over sixty species of plants used in traditional Indian medicine showed that substantial sources of antimicrobials are found in flowers, leaves, and resin. This phenomenon is the basis of natural relationships like pollinator reward systems, which involves mutually beneficial interactions when pollinators, such as bees, receive ‘rewards’ from plants (primarily in the form of pollen or nectar). In return, the plants are pollinated by the bees (Simpson and Neff, 1981). In rarer cases, this pollinator reward system has been observed in nature using alternative rewards such as resins, particularly those containing antimicrobial properties. Though pollinator reward systems using resin are fairly rare, the genus Clusia, from the family Clusiaceae, has several species that have these relationships with male euglossine bees (de Faria et al., 2006).
The genus Clusia contains around 250 species native to tropical America, many of which produce a viscous resin containing high amounts of polyprenylated benzophenones named guttiferones. Guttiferones have shown significant antifungal, antimicrobial, and antihuman immunodeficiency virus (HIV) effects (Gustafson et al., 1992; Cruz and Teixeira, 2004). When used in pollinator reward systems, the resin has many different uses depending on the species of the bee collecting the resin and the current needs of the hive. For example, Apis bees use collected resin to seal their hive, whereas Eulaema and Euglossa bees use it in the construction of their nests. Antimicrobial resin, such as the resin produced by Clusia, can be used to protect food and larvae from bacteria and fungi (Patiny, 2012).
Interestingly, almost all known Clusia are dioecious, meaning they have differentiating secondary sex characteristics—which could include the properties of these resins (Luján, 2019). Abe (2001) showed that in another species of dioecious flower, Aucuba japonica, there are significant differences in the proportion of open flowers and the period of time for which flowers opened between male and female inflorescences. Not only did male inflorescences generally flower for longer, but they also had a higher proportion of flowers open at the peak of the flowering period. Therefore, it is possible that female flowers may show higher antimicrobial activity to compensate for the shorter time frame in which they are able to become pollinated, thus making them more desirable to pollinators.
Using an array of microbial culturing, including spread plating, culturing of Petrifilms, and biochemical testing, this project aims to “The genus Clusia contains around 250 species native to tropical America, many of which produce a viscous resin containing high amounts of polyprenylated benzophenones named guttiferones ”
Figure 2: A schematic representation of the Petrifilm grid. The areas highlighted are an example of four randomly selected squares, which would then be counted and multiplied up to get the total number of colonies on the Petrifilm.
Figure 3: Female sample number two. This image was captured as part of the cataloguing of the samples. This particular female flower measured 2cm in diameter and weighed 6.9g. Photograph captured by Samuel Shaw and used with permission. develop a further understanding of the differences in microbial flora to predict possible antimicrobial efficacy between male and female samples of flowers from Clusia sp.
Materials and Methods This research was carried out in the Santa Lucia cloud forest reserve, located at the far south of the Choco-Andean Rainforest Corridor, within the Pichincha province in Ecuador, in July 2018 (Figure.1). In this study, sixteen flowers were collected from four trees, two males and two females, of an unidentified species, from the genus Clusia, family Clusiaceae. Female sample 1 was collected at N00°07.084 W078°36.706, female sample 2 at N00°06.944 W078°36.114 and male samples 1 and 2 were taken at N00°07.110 W078°36.507 and N00°06.964 W078°36.155 respectively.
A rudimentary laboratory was set up with all the microbial culturing carried out close to a Bunsen burner to maintain as close to an aseptic environment as possible, so as to try to avoid contamination (Fig. 2). All equipment was cleaned with ethanol and sterile water between uses and a negative control of sterile water was used to ensure all of the cultured colonies were representative of the microbial growth present on the flowers and not a result of contamination.
Before testing, each flower was photographed, catalogued, stored in a plastic collection pot with 100ml of sterile water, and then left to infuse for sixty minutes. After infusion, the flowers were weighed using a 10g spring balance. Next, the water pH was measured (with pH strips), as was the sugar concentration (with a PAL-1 Atago digital refractometer, USA). The infused water was then put aside for microbial culturing. The microbial load was measured using a combination of spread plating and 3M Petrifilm Rapid Aerobic Count, Rapid Yeast and Mould and Rapid Coliform / E. coli plates (3M United Kingdom PLC, Bracknell, England). Spread plating was executed under aseptic technique, using 1ml of infused water. 1ml of sterile water was also cultured as a negative control.
Petrifilms for samples 1-4 of both sexes were prepared using 1ml of the infused water pipetted directly onto the films. Upon initial examination, many of the male films had too many colonies to count. To account for this, samples 5-8 were adjusted by using 0.5ml of the sample water and 500µl of sterile water. For each set of Petrifilms cultured, a control plate of 1ml of sterile water was also prepared.
To calculate the total colony count per plate, four equal sections of the petrifilms were chosen at random; the populations of those chosen areas were then counted and an average of the overall count was reached (Fig. 3).
All cultures were incubated for 24 hours in a field incubator made from a tin foil-lined box containing a mixture of bubble wrap and temperature-controlled hot water bottles, which maintained a temperature of 18-40°C, optimal for encouraging microbial growth.
Initially, it was found that some petrifilms had too many colonies to count. When this happened, the samples were diluted as described above; however, this methodology did not overcome the excess of colonies. It was therefore decided that quantifiable plates would be those with colony counts from 0-999, any higher would be estimated as 1000 colonies
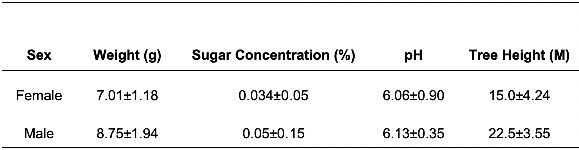
Table 1: Key findings from biochemical testing. The results of biochemical tests provide means and standard deviation of key findings including mass, height, pH and sugar concentration differences between samples taken from male and female Clusia sp. flowers.
for statistical purposes.
Differences between colony counts of samples taken from male and female flowers were first tested for normality using the AndersonDarling test. When the data were normally distributed,
a 2-sample t-test was used to test for significance between the number of female and male colonies formed on the Petrifilms. If the data were not normally distributed, a MannWhitney test was used to analyze the data.
Results The Clusia flowers used in this experiment had red and black petals, which were, on average, 2 cm in diameter. Their weights differed slightly between the sexes, with male inflorescences weighing slightly more (8.75±1.94g) than those of females (7.01±1.18g). Male trees also grew on average 7.5m taller than female trees (22.5±3.54m vs 15±4.24m).
Measurements of the sugar levels present revealed trace amounts in the sample, with concentrations low in both males (0.05±0.15) and females (0.034±0.05). Female samples
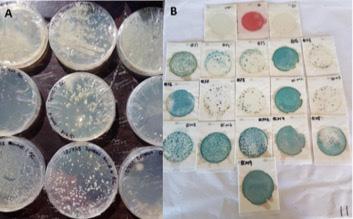
showed a minimally lowered pH level compared to the males (6.06±0.90 vs 6.13±0.35), but this difference is negligible and not statistically significant (Table 1).
Spread plating produced a myriad of results, as seen in Figure 5B. The presence of aerobic bacteria on the petrifilms is shown with a blue-green stain, which spread much more extensively on the male samples. All of the plates had less fungi than bacteria, and all fungal growths were seen exclusively on male samples. This correlates well with the Petrifilm data recorded and the negative controls showed no clear microbial growths, which suggests contamination did not play a significant role in these results.
There was a large difference between the number of colonies of yeast and mould specimens which grew on samples taken from female flowers (13±16.52) compared to those taken from male flowers (815±342.10) (Fig. 6). Most male samples had too many colonies to count (>1000 colonies) and, after quantifying these results (at 1000 colonies per plate), it showed that males had significantly more colonies, around 802 on average, than female “The presence of aerobic bacteria on the petrifilms is shown with a bluegreen stain, which spread much more extensively on the male samples.”
Figure 4: A representative sample of the spread plates which were produced. A wide selection of different morphologies is seen within the colonies grown on the different samples; one fungal growth can be seen in the bottom righthand plate. B: All the cultured petrifilms for aerobic bacteria are shown with both female and male samples included. Above, the negative controls for all three of the petrifilms are also visible. Female samples are labelled as F1-8 and males as M1-9.
Photographs captured by the author.
Figure 5: Mean and standard deviation of the number of yeast and mold colonies grown on Petrifilm samples taken from male and female Clusia sp. flowers.
Figure 6: Mean and standard deviation of the number of colonies of aerobic bacteria grown on Petrifilm samples taken from male and female Clusia sp. flowers. Aerobic bacteria appeared to grow well on all samples (Fig. 7), though male samples did show a higher number of colonies than female samples (508±422.76 in males as opposed to 346±436.92 in females). The spread plated samples yielded similar results (Fig. 5B). This equates to an average of 72 more colonies per Petrifilm on male samples, though this was not statistically significant (T=0.75, P= 0.464, DF=14).
The findings shown in Figure 8 were consistent with other results, in which colonies of coliforms were found on all the samples which were cultured, though there was no statistically significant difference between the number of colonies growing on male (143±142.88) and female (56±112.45) Petrifilm samples (Fig. 8; P=0.712, W=72.0, n=4).
There was relatively little growth on the Escherichia coli petrifilms, though samples taken from male flowers showed some growth, with a mean number of 2±3.94 colonies per plate. There were no colonies observed on the female samples (Fig.9); therefore, this data was not sufficient for statistical analysis.
Discussion Female flowers had lower colony counts across all findings, with a 60% decrease in the mean number of fungal colonies per female sample compared to male samples. This could be due to intrinsic antifungal properties in the female flowers. A study by Fernandez et al. (2016), on Clusia hilariana, showed that female plants not only have higher levels of specific essential oils than male plants, but that they also have some oils that male plants do not possess at all. One of these components is alpha cadinol, a powerful
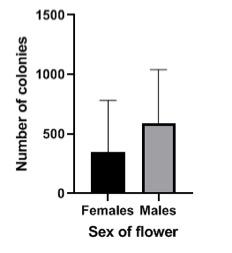
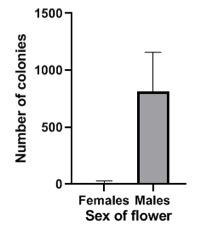
fungicide, which is around four times more prevalent in females (2.2%) than in males (0.5%) (Ho et al., 2012). Furthermore, Cruz and Teixeira (2004) showed that Clusia tends to produce a large quantity of polyprenylated benzophenones, which have been proven to exhibit a wide variety of pharmacological effects, including anti-inflammatory, anti-HIV activity, fungicidal, and antimicrobial activity. This may suggest that the fungicidal action is not in fact due to discrepancies between the components of the resin itself, but rather a difference in the amount of resin present in males and females. This is supported by the work of Porto et al. (2000) which found no divergence between the male and female versions of the major chemical structures of the resin, but did find that the concentrations of resin varied between males and females. Though these findings may offer an explanation as to how these specimens produce an antimicrobial effect, it is also important to understand why this occurs; one popular theory involves pollinator reward systems.
Pollinator reward systems are symbiotic relationships between plants and pollinators like birds and insects. Usually, pollinator reward systems offer pollinators nectar in exchange for pollinating the plants; however, some use non-sugar rewards, such as resin. This has been observed previously between several species of Clusia and several species of bees; the bees even select specific plants depending on the purpose for which the resin is required. Resin which hardens quickly can be used by species of Aphid bees to cement together structures for the hive or to seal the outside, whereas a softer and more malleable resin can be used for structuring cells within the hive, which may have to be remolded (Patiny, 2012). Antimicrobial resins may be used to defend structures from pathogens, therefore protecting food stores,
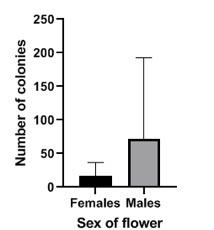
larvae, and the colony itself from attack (Farias et al., 2011; Ribeiro et al., 2011).
In addition, Llyod and Webb (1977), showed that, in a wide range of species, not only do male inflorescences tend to appear earlier in life and more frequently than females, but that male plants also tend to produce a higher number of inflorescences than females. If the plants are using the resin produced by the inflorescences to attract bees and allow for pollination, it could be that the females had a lower bacterial or fungal load due to an intrinsically higher antibacterial activity to compensate for the lower number of inflorescences (Evers et al., 2018). This outside pressure of selective pollinators choosing plants which offer specific rewards, such as antimicrobial resins, may explain why only trace levels of sugar were found; this particular species of Clusia may produce large amounts of resin rather than an abundance of nectar. On average, female samples also had a 32% lower sugar concentration than male samples, which may also be due to higher amounts of resin produced, as part of this compensatory mechanism.
Another explanation for the differences between male and female inflorescences could be a result of the antifungal effect of the female Clusia. More than 90% of terrestrial plants form mycorrhizal associations, in which the roots of the plant form a symbiotic relationship with a species of fungi. This relationship causes an increase in phosphorus available to the plant largely due to an increased surface area, using the arbuscular mycorrhizal fungal hyphal network (Bolduc, 2011). Though literature surrounding Clusia-mycorrhiza associations is fairly limited, it has been documented that Clusia develops much better when mycorrhized. This has also shown to be the case in a range of other species, leading to larger plants with an increase in the number of inflorescences, so it is possible this could also apply to Clusia (Lüttge, 2007; Scagel, 2003). The antifungal activity of a female may disrupt this relationship, leading to an average decrease in height of 7.5m compared to the male trees and fewer inflorescences. A similar effect has been seen in species growing close to Alliaria petiolata, which also shows antifungal activity (Stinson et al., 2006). This relationship could be investigated in future research by conducting staining on sections of male and female root systems, to search for the presence of fungi. Plant tissue analysis could also be used to look for a difference in phosphorus levels between male and female leaves, which would suggest a mycorrhizal association.
Several outliers were observed in this study, with certain flowers showing an abnormal bacterial count. One possible cause of these outliers is age. For example, male sample 2—a closed bud—showed no fungal growths. It is possible that because the bud was sealed shut, the fungi were unable to enter, hence none were present. Another outlier is female sample 4, which—despite the low microbial load of most female flowers—showed an extremely high bacterial count. Research by Tawaha and Hudaib (2012) on Thymus capitatus examined essential oils, which can show antibacterial effects, from buds and flowers and found that mature flowers yield 0.93% more oil. Though this research was not carried out on Clusia, it was carried out on members of the Lamiaceae family, which are also eudicots. This suggests the plant may produce more oil as it gets older; if the resin uses the same mechanism, that would suggest the buds in female sample
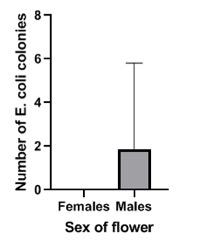
Figure 7: Mean and standard deviation of the number of colonies of coliforms grown on Petrifilm samples taken from male and female Clusia sp. flowers.
Figure 8: Mean and standard deviation of the number of colonies of E. coli grown on Petrifilm samples taken from male and female Clusia sp. Flowers.
4 were not old enough to produce as much antimicrobial resin as the mature flowers, resulting in higher levels of bacteria. Therefore, future studies should analyze flowers from a range of ages to test for differences in the level of antifungal activity.
This research is an exciting start to what is a relatively understudied area of science. Future applications could focus on obtaining a larger amount of data, with an increased number of repeats, so as to increase the validity of any findings. This could also be achieved by using more precise equipment, such as laboratory grade incubators and automated colony counters, to obtain more accurate results and a higher throughput of data. The next step would be to analyze the resin produced by both female and male samples of this species and look for any differences between the sexes and the compounds produced (such as alpha-cadinol) and for possible differences in the concentrations of resin produced by the sexes. This could then definitively prove why females show such a higher antimicrobial effect than males. Treatment of the resin with Diazomethane allows methylation of the resin, which would prevent it from decomposing and allow it to later be purified using silica column chromatography; this technique has been successfully used to analyze resin produced by Clusia flowers in the past (Porto et al., 2000).
The resin produced by the female samples of this species of Clusia may also be able to undergo toxicity testing, such as on cell lines and LD50 testing, as it is a potent antifungal and may well be able to be used in human or veterinary medicine in the future. It is important to consider all manners of toxicity in testing, as another species of Clusia, Clusia alata, has previously been shown to have mutagenic properties at very high doses (2000 mg/kg) (Moura et al., 2008). Currently, there seem to be no treatments available using Clusia extract, though previous testing for a potential antibiotic has been carried out using the stem, with poor results (Suffredini et al., 2006). It is possible that extracts using resin would be much more potent.
Conclusion Female Clusia sp. flowers were found to have a statistically significant lower number of fungal colonies than males (p<0.009); female samples also had fewer bacterial colonies than males, though this difference was not statistically significant. This may be due to a symbiotic relationship developed with bees as a pollinator reward system using antimicrobial resin. It is also possible that this inherent antimicrobial activity may be due to the presence of compounds such as polyprenylated benzophenones or alpha-cadinol.
Acknowledgements Many thanks to my fantastic project leaders Adrian Goodman and Catrin Gunther for all their support, advice and patience with me on this project, both in the field and in their support after. Also, my fellow academic, Sophie Ellis, for her continued advice and help throughout the duration of this research and its editing as well as the support and guidance of Clare Miller. Finally, thanks to the University of Lincoln funding department for funding received towards travel so this research could be undertaken.
References
Abe, T. (2001). Flowering phenology, display size, and fruit set in an understory dioecious shrub, Aucuba japonica (Cornaceae). American Journal of Botany, 88(3), 455-461.
Ali, S., Siddiqui, R. and Khan, N. (2018). Antimicrobial discovery from natural and unusual sources. Journal of Pharmacy and Pharmacology, 70(10), 1287-1300.
Armbruster, W. (1997). Exaptations link evolution of plantherbivore and plant-pollinator interactions: A phylogenic enquiry. Ecology, 78(6), 1661-1672.
Bolduc, A. (2011). The Use of Mycorrhizae to Enhance Phosphorus Uptake: A Way Out the Phosphorus Crisis. Journal of Biofertilizers & Biopesticides, 02(01).
Brundrett, M. (2002). Coevolution of roots and mycorrhizas of land plants. New Phytologist, 154(2), 275-304.
Davies, S., Fowler, T., Watson, J., Livermore, D. and Walker, D. (2013). Annual Report of the Chief Medical Officer: infection and the rise of antimicrobial resistance. The Lancet, 381(9878), 1606-1609.
de Faria, A., Matallana, G., Wendt, T. and Scarano, F. (2006). Low fruit set in the abundant dioecious tree Clusia hilariana (Clusiaceae) in a Brazilian restinga. Flora - Morphology, Distribution, Functional Ecology of Plants, 201(8), 606-611.
Evers, H., Jackson, B. and Shaw, S. (2018). Overseas Field Course Report. University of Lincoln.
Farias, J., Ferro, J., Silva, J., Agra, I., Oliveira, F., Candea, A., Conte, F., Ferraris, F., Henriques, M., Conserva, L. and Barreto, E. (2011). Modulation of Inflammatory Processes by Leaves Extract from Clusia nemorosa Both In Vitro and In Vivo Animal Models. Inflammation, 35(2), 764-771.
Fernandes, C., Cruz, R., Amaral, R., Carvalho, J., Santos, M., Tietbohl, L. and Rocha, L. (2016). Essential Oils from Male and Female Flowers of Clusia hilariana. Chemistry of Natural Compounds, 52(6), 1110-1112.
guttiferones, HIV-inhibitory benzophenones from Symphonia globulifera, Garcinia livingstonei, Garcinia ovalifolia and Clusia rosea. Tetrahedron, 48(46), 10093-10102.
Ho, C., Hua, K., Hsu, K., Wang, E. and Su, Y. (2012). Composition and antipathogenic activities of the twig essential oil of Chamaecyparis formosensis from Taiwan. Natural Product Communications, 7(7), 933-936.
Khan, B., Bakht, J. and Khan, W. (2018). Antibacterial potential of a medically important plant Calamus aromaticus. Pakistan Journal of Botany, 50(6), 2355-2362.
Kumar, V., Chauhan, N., Padh, H. and Rajani, M. (2006). Search for antibacterial and antifungal agents from selected Indian medicinal plants. Journal of Ethnopharmacology, 107(2), 182188.
Kumarasamy, K., Toleman, M., Walsh, T., Bagaria, J., Butt, F., Balakrishnan, R., Chaudhary, U., Doumith, M., Giske, C., Irfan, S., Krishnan, P., Kumar, A., Maharjan, S., Mushtaq, S., Noorie, T., Paterson, D., Pearson, A., Perry, C., Pike, R., Rao, B., Ray, U., Sarma, J., Sharma, M., Sheridan, E., Thirunarayan, M., Turton, J., Upadhyay, S., Warner, M., Welfare, W., Livermore, D. and Woodford, N. (2010). Emergence of a new antibiotic resistance mechanism in India, Pakistan, and the UK: a molecular, biological, and epidemiological study. The Lancet Infectious Diseases, 10(9), 597-602.
Lloyd, D. and Webb, C. (1977). Secondary sex characters in plants. The Botanical Review, 43(2), 177-216.
Luján, M. (2019). Playing the Taxonomic Cupid: Matching Pistillate and Staminate Conspecifics in Dioecious Clusia (Clusiaceae). Systematic Botany, 44(3), 548-559.
Lüttge, U. (2007). Clusia: A Woody Neotropical Genus Of Remarkable Plasticity And Diversity. Berlin: Springer, 235-239.
Moura, A., Perazzo, F., Maistro, E. (2008). The mutagenic potential of Clusia alata (Clusiaceae) extract based on two short-term in vivo assays. Genetics and Molecular Research, 7(4), 1360-1368. Patiny, S. (2012). Evolution of plant-pollinator relationships. Cambridge: Cambridge University Press, 51-54
Peres, M., Monache, F., Cruz, A., Pizzolatti, M. and Yunes, R. (1997). Chemical composition and antimicrobial activity of Croton urucurana Baillon (Euphorbiaceae). Journal of Ethnopharmacology, 56(3), 223-226.
Ribeiro, P., Ferraz, C., Guedes, M., Martins, D. and Cruz, F. (2011). A new biphenyl and antimicrobial activity of extracts and compounds from Clusia burlemarxii. Fitoterapia, 82(8), 12371240.
Salam, A. and Quave, C. (2018). Opportunities for plant natural products in infection control. Current Opinion in Microbiology, 45, 189-194.
Scagel, C. (2003). Inoculation with arbuscular mycorrhizal fungi alters nutrient allocation and flowering of Freesia x hybrida. Journal of environmental horticulture, 21(4), 196.
Simone-Finstrom, M., Gardner, J. and Spivak, M. (2010). Tactile learning in resin foraging honeybees. Behavioral Ecology and Sociobiology, 64(10), 1609-1617.
Simpson, B. and Neff, J. (1981). Floral Rewards: Alternatives to Pollen and Nectar. Annals of the Missouri Botanical Garden, 68(2), 301. Stinson, K., Campbell, S., Powell, J., Wolfe, B., Callaway, R., Thelen, G., Hallett, S., Prati, D. and Klironomos, J. (2006). Invasive Plant Suppresses the Growth of Native Tree Seedlings by Disrupting Belowground Mutualisms. PLoS Biology, 4(5), 140.
Suffredini, I., Paciencia, M., Nepomuceno, D., Younes, R. and Varella, A. (2006). Antibacterial and cytotoxic activity of Brazilian plant extracts - Clusiaceae. Memórias do Instituto Oswaldo Cruz, 101(3).
Tawaha, K. and Hudaib, M. (2012). Chemical composition of the essential oil from flowers, flower buds and leaves of Thymus capitatus hoffmanns. Journal of Essential Oil Bearing Plants, 15(6), 988-996.
Zhang, X., Zhang, Y., Xu, X. and Qi, S. (2013). Diverse DeepSea Fungi from the South China Sea and Their Antimicrobial Activity. Current Microbiology, 67(5), 525-530.