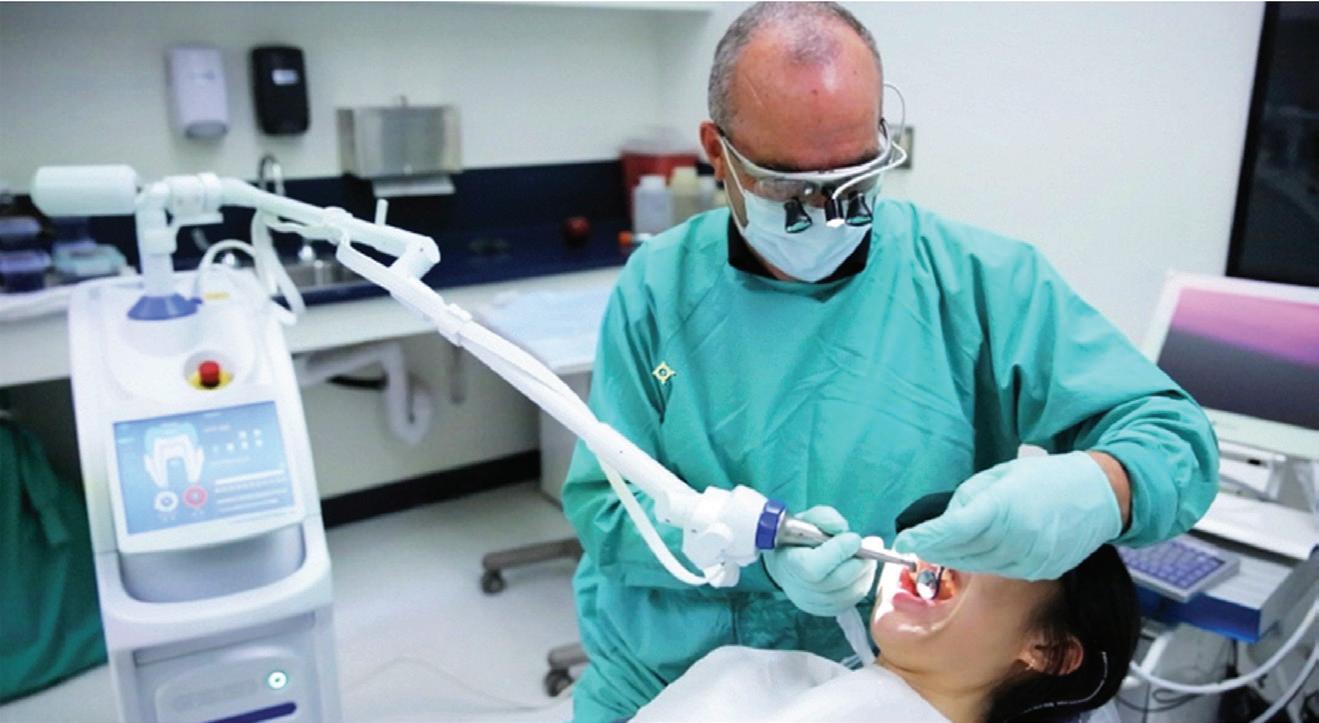
15 minute read
Tooth surface protectionfrom concept to clinic
By Emeritus Professor Laurence J. Walsh AO
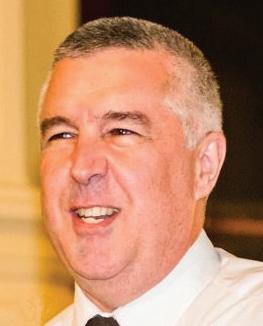
The concept of protecting enamel and root surface from dental caries is a very old idea. Going back into the writings of GV Black, he proposed placing margins for class II cavities into a zone that he had identified histologically as not being affected by the caries process.
Unfortunately, using the histology methods available at his time, which precluded sectioning of teeth with associated soft tissues, he did not realise that this zone, which was spared from demineralisation, was unfortunately in fact the zone where the junctional epithelial cells attached. Despite this false start, much work has been done to develop the concept of tooth surface protection into its most modern forms.
Resin-based materials
In the 1960s, the work of Richard Simonsen on resin-based fissure sealants was instrumental in establishing the concept of protecting pits and fissures by covering the enamel with an unfilled resin.1,2
Later studies of fissure sealants applied to erupted permanent molar teeth showed high effectiveness for the areas where the sealant materials remained over time.3-5 The principle that arises from this is that resins are an inert material which covered the surface to prevent acid produced by dental plaque biofilms coming into contact with the enamel. Later work done in my lab by Jason Yap6 showed that modern fissure sealant materials and nano filled resins protect enamel from acid attack in the same way as the early materials of the 1960s. At the edge of the resin material, where a cariogenic dental plaque biofilm is present, a zone of demineralisation develops. Hence, with such materials, it is important to cover the area that you want to protect.
This then leads onto the major problem with resin-based materials, which was the difficulty of reliably bonding a resin to partially erupted teeth where crevicular fluid, dental plaque and saliva were likely to be present. All of these local factors can impede the adhesion of resin to etched enamel. Adding to this, on erupting teeth, the most superficial layers of the enamel are aprismatic and as a result, do not etch particularly well.
Areas of enamel may be present on newly erupted teeth that are not fully mineralised.7 To address these concerns, the concept of using glass ionomer cement as a less technique-sensitive and more reliable fissure sealant approach for erupting teeth emerged.8,9 The GIC forms a transition zone with the enamel, creating a region of greatly increased resistance to acid dissolution. This acidresistant zone remains even when gross fracture of the overlying cement occurs because of high occlusal stresses.
Glass ionomer cements
The next logical development from studying the impact of glass ionomer cements used in the early 1990s in field trials of atraumatic restorative dentistry was the concept of deliberately placing high fluoride releasing glass ionomer cements onto partially erupted teeth, as a form of tooth surface protection.10 The underlying principle here is that materials such as GC Fuji VII not only display extremely high fluoride release, but also impressive recharging abilities when the material is subsequently exposed to a fluoride containing toothpaste, a fluoride gel or another fluoride product.11 When present in thin layers, fluoride ions will move through the material from the oral environment to become incorporated into the enamel. In this way, the overlying Fuji VII helps to not only protect the enamel of the tooth, but also to encourage its greater mineralisation. Laboratory studies of Fuji VII reveal that the material provides a wide zone of protection beyond its borders, defending the enamel even from strong carious attacks from organic acids.6,12 Repeated attacks by strong mineral acids will of course eventually cause the Fuji VII to dissolve away, sacrificing itself to protect the underlying tooth.12 This can be likened to a sacrificial anode effect, drawing a parallel to the electrochemistry behind a modern domestic electrical hot water system.
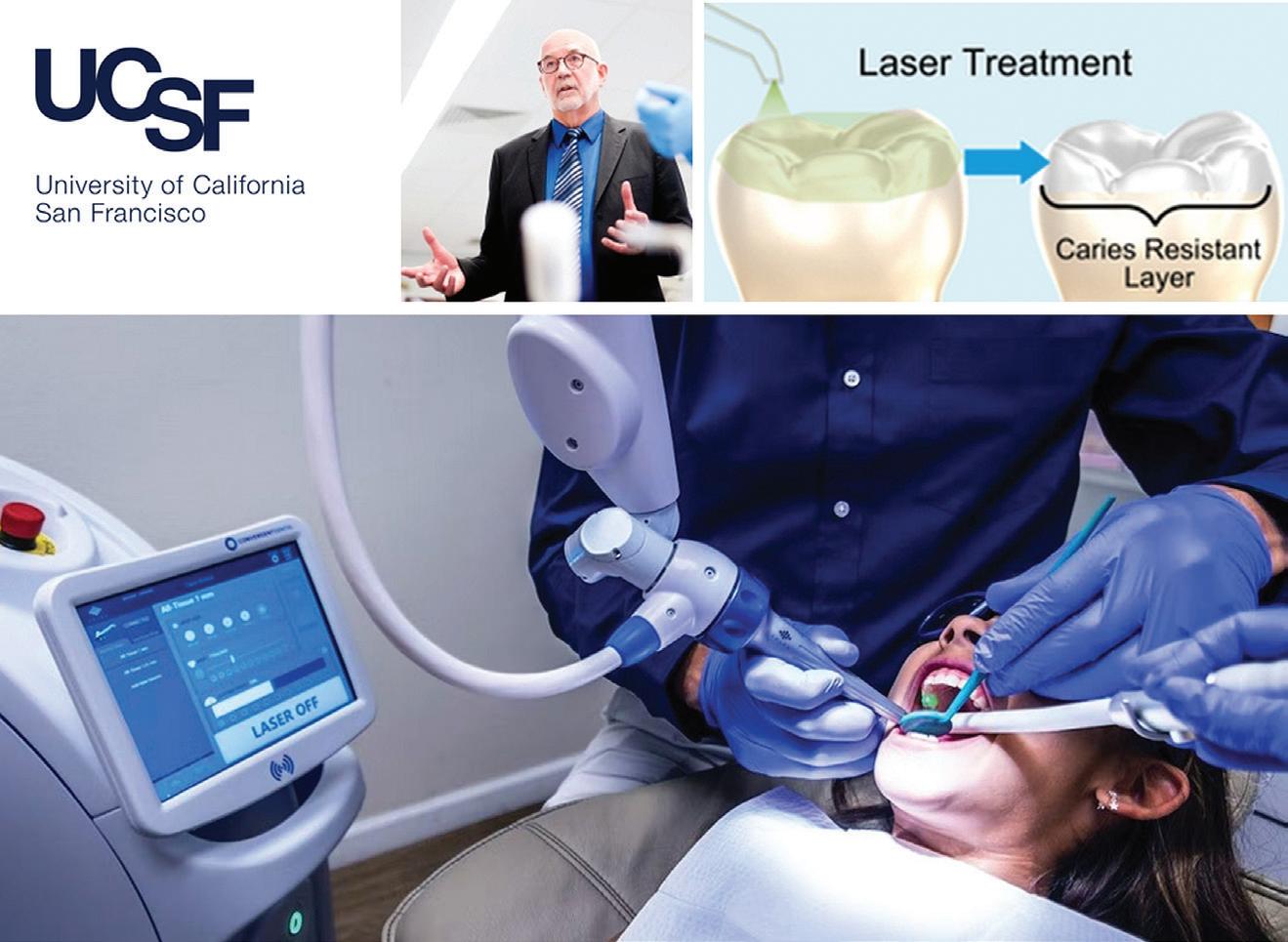
Having found this approach using GIC highly successful on erupting permanent teeth, the concept was then extended to root surfaces. Certain sites in the mouth are known to be highly prone to root surface caries, most notably the buccal furcation area of mandibular molar teeth and the buccal surfaces of the mandibular premolar teeth. These surfaces show the highest attack rates for root surface caries. In a patient with a high caries risk, for example due to salivary gland hypofunction from polypharmacy, Fuji VII can be painted in a thin layer onto the root surface.10 The fluoride released alters the metabolic behaviour of the overlying dental plaque biofilm, reducing its acid production. The later development of Fuji VII EP, which contains CPP-ACP, added further to the concept because the release of CPP from this material causes prebiotic effects,13,14 encouraging the growth of health associated bacteria which naturally inhibit the growth of acid tolerant and acid producing cariogenic bacteria.15,16
Let there be light
The idea of altering the chemical structure of teeth using intense light goes back to the 1980s. It was driven very much by dental scientists who had an interest in the physical chemistry of enamel mineral.17 By using wavelengths of light that were absorbed strongly into enamel, superficial heating could be achieved.18 As a result, the chemical composition of the enamel could be altered, as well as its porosity.19-21 Sufficient amounts of applied heat could even create a liquefied form of tooth mineral, which could flow over the tooth surface to reshape existing pits and fissures, removing their deep recesses.18 A similar approach could also be used to sinter synthetic hydroxyapatite particles onto the surfaces of teeth.22
A key consideration for pulpal safety was that the carbon dioxide laser radiation had to be delivered in a pulsed manner, to prevent inadvertent thermal stress.23
Much of the early work used the carbon dioxide laser and the Er:YAG laser, since the far and middle infrared wavelengths of light produced by these lasers (e.g. 2.94 and 9.3 to 10.6 µm) were all strongly absorbed in enamel and were also able to vaporise any unbound water in the enamel. The idea of deliberately lasing surfaces transition from the laboratory, to in situ studies using enamel slabs in removable appliances, through to clinical assessments. Numerous narrative and systematic reviews of the positive effects of infrared laser energy on enamel have been published in recent years.24-27,38
More recently, there have been clinical trials undertaken of 9.3 µm carbon dioxide laser treatments for caries prevention at the University of Southern California in San Francisco, where Drs John Featherstone and Peter Rechmann have been pioneers of the concept of using far infrared laser energy to enhance enamel resistance to carious attack (Figure 1).39-41
Light plus fluoride
The next conceptual development was to explore the interaction of topical fluoride gels with applied laser energy.28-35 This was tested by applying fluoride gel followed by the laser, or in the reverse sequence, assessing parameters such as fluoride uptake, chemical conversions within the enamel, surface microhardness, surface loss and resistance to standardised challenges that replicate dental caries or dental erosion, such as lactic acid solutions or hydrochloric acid solutions, respectively. Studies of fluoride uptake showed that a threefold improvement could be obtained over simply applying the fluoride gel onto the tooth surface alone.
This work extended through to in situ studies and then into the clinical setting. In special needs clinical practice, this method was quite useful for patients with severe salivary gland hypofunction (e.g. Sjogren’s syndrome or post head and neck radiotherapy), as it made treated surfaces less likely to decay and break down over time.36
From a photobiological perspective, when using a light-based therapy, it is important to determine its action spectrum, which is the region or regions of
Table 1. Tooth surface protection approaches for caries prevention
light that are responsible for the effect and which are most effective for therapeutic use. As an example of the action spectrum effect, one can use various standardised photobiological light sources to simulate solar radiation and then determine which parts of the ultraviolet, visible and infrared spectrum are responsible for effects such as photo-ageing, cataract formation and erythema (sunburn). During my training as a photo biologist, I determined the action spectrum for sunburn using sensitive measures of invisible inflammatory changes that pre-seed the visible appearance of redness in the skin.37
Applying the same thinking to the light and fluoride interaction, a series of studies at UQ were undertaken to map out the action spectrum of the effect, testing wavelengths through the visible spectrum and well into the infrared.28,29 While we and others were able to demonstrate that all the wavelengths tested were effective to some degree, we noticed a particularly high efficacy for wavelengths in the visible blue-green regions. It is perhaps no coincidence that the same wavelengths have the greatest penetration through water.
Part of the journey of exploration was to test such effects using light emitting diodes. This leverages the recent transition of dental curing lights away from halogen lamps and plasma arc lamps to LEDs. When one converts the exposure parameters for blue and green lasers (488, 515 and 532 nm) to the 460-470 nm wavelength range of a traditional dental curing light, the required 15 J/cm2 equates to one 20 second curing cycle for an LED light with a 6-8mm diameter tip and optical power of 1000-1500 mW (Figure 2). This is a fortunate convenience since it makes the clinical application of the concept quite straightforward.
In recent years, work has shown that a range of hard tissue laser wavelengths in the middle infrared range are also effective for enhancing fluoride uptake and improving resistance to acid attack. There have been published systematic reviews of the laboratory studies, as well as of the multiple clinical trials which have been conducted of light enhanced fluoride therapy.24,25,27 Of note, the definition used in the current edition of the ADA Schedule and Glossary for item 121 mentions the activation of the remineralising agent, which is a contracted version of the initial longer description dating back to 2008 which explicitly mentioned lasers and fluoride used together.
When considering what situations might suit the application of a light-enhanced fluoride approach, one must consider carefully the underlying chemistry of what is happening. There are over six different physical and chemical processes involved, with a major one being the conversion of surface hydroxyapatite into fluorapatite.30 This is responsible for an increase in surface microhardness and greater resistance to attack by both organic and mineral acids. This effect requires the availability of fluoride ions in an phase on the tooth surface.
Knowing this, there is no known value or benefit in using a curing light when one is applying a fluoride varnish, including a fluoride varnish containing CPP-ACP, or Tooth Mousse Plus. In the case of the varnishes, there is rapid precipitation of calcium fluoride granules, which form a layer several microns thick over the enamel surface. This layer can take up to a week to dissolve. The light does not interact with the calcium fluoride. In the case of Tooth Mousse Plus, the fluoride is initially bound to the peptide and then when released forms part of the neutral ion species which interacts with and penetrates deeply into the tooth surface. The stoichiometry of the product is such that there is not a gross excess of free fluoride ions.
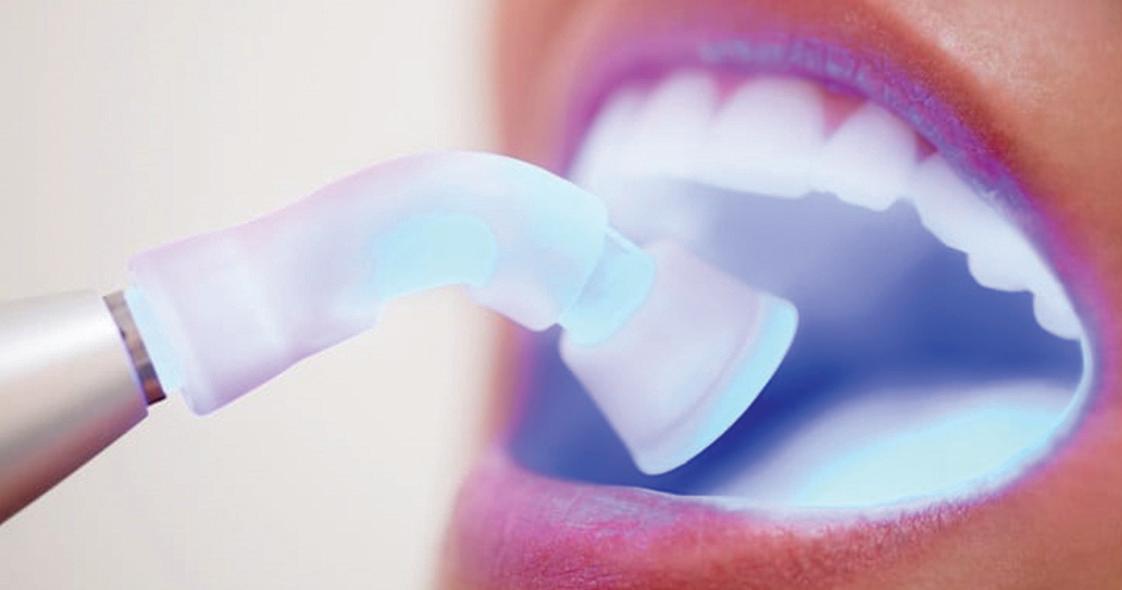
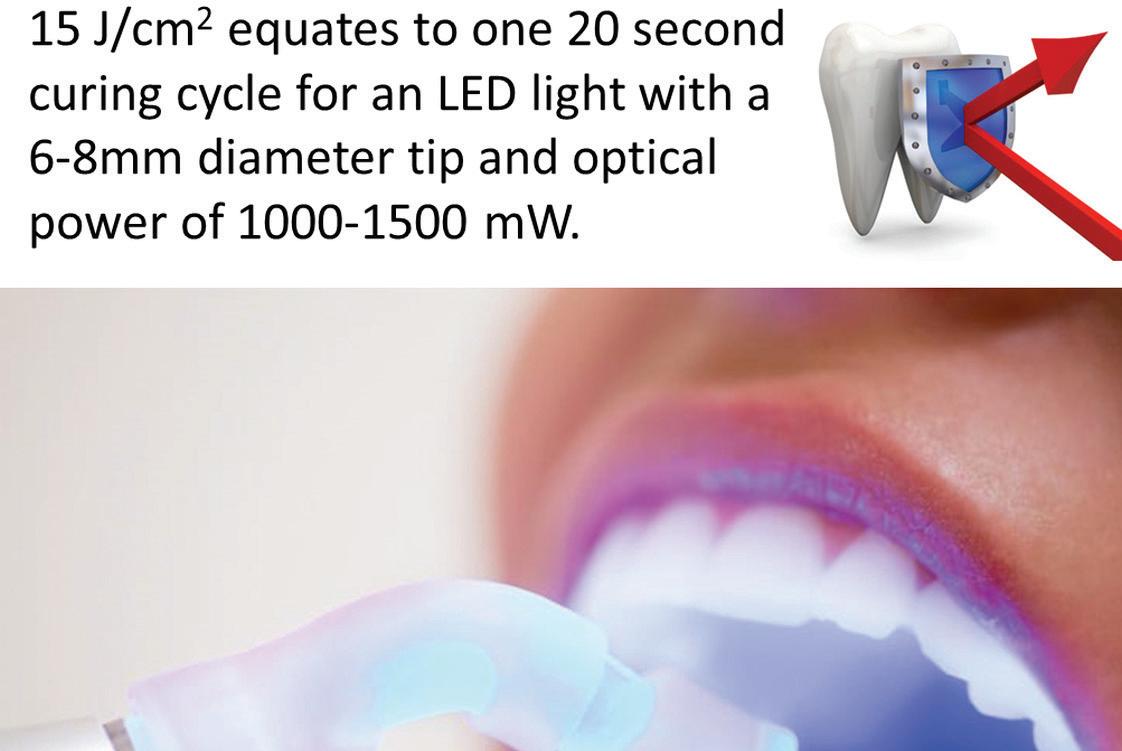
One situation where there could be significant amounts of free fluoride ions is immediately following the application of stannous fluoride or silver fluoride. The issue here is the susceptibility to oxidation and light sensitivity of the stannous and silver ions respectively. When products such as SDI Riva Star or Riva Aqua are used in combination with potassium iodide, one has to remember that silver iodide will be formed and this will be very light-sensitive. All halide compounds of silver are exceptionally light sensitive and even metallic silver itself is unusually sensitive to certain wavelengths of violet light (around 420 nm), which cause a phenomenon known as plasmon resonance. One must also remember that a darkened tooth surface becomes a broad-spectrum absorber of light and so any intense light applied to the tooth can cause thermal stress to the dental pulp as heat is conducted through the dentine.
Key recommendations for the use of various tooth surface protection measures are given in Table 1.
Chemical synthesis of enamel
In the laboratory, there are well-known methods for chemical synthesis of hydroxyapatite and fluorapatite. These can produce the materials in microscopic aggregates or as nanoparticles, depending on the method of synthesis. The challenge has always been, can such methods be applied on the surface of teeth to recreate enamel that has been lost? Over 40 years of work on the concept of chemical synthesis of enamel has been done and the most that has been achieved by any research group has been to generate layers that are up to 0.5 µm in thickness. To give a sense of scale, this is approximately the thickness of a single bacterial cell and more than 25 times less than the amount of enamel that would be lost as a result of drinking a single can of black cola soft drink. A fundamental limitation of the concept of chemical synthesis of enamel is that it lacks the organic matrix structure which guides the formation of enamel by ameloblasts. Further, through the enamel there are various complex arrangements of enamel prisms to create structural support systems (such as the Rainey ridge in molar teeth), which are difficult if not impossible to recreate.
Sintering studies using synthetic apatites have been conducted and these can generate sizeable portions of tooth like structure using additive manufacturing laser sintering methods. As a point of history, the first successful work of this type was done in the early 1990s.22 The major limitations encountered with this approach include the fact that it is a benchtop rather than intraoral method, as well as the predictable challenges working with very small apatite particles because of electrostatic effects that cause clumping and aggregation. The sintering process itself alters the chemistry of the apatite, leading to conversion to beta tricalcium phosphate. This makes the resultant material inferior from a structural point of view (lower compressive strength), unsuitable for bonding and highly opaque in appearance and thus aesthetically unacceptable.
Nature thought of it first
In the mid-1980s, I recall attending a symposium held as part of the International Association of Dental Research general meeting in North America. One of the major topics of discussion was what would be the design characteristics for a material that could be coated onto enamel to protect it. A range of people working in the field contributed their opinions and shared their experiences on what they had tried and found to be lacking in one way or another. The discussion shifted to then explore what the inherent usefulness was of pellicle, the saliva glycoproteins that adsorb onto the surface of exposed enamel. Up to this point, there had been a generally held view that pellicle was fundamentally either useless or harmful.
The latter concept grew from the recognition that several primary colonisers of teeth had adhesin molecules that bound to pellicle, allowing them to form a stable anchorage.
clinical
This whole concept of pellicle being inert turned out to be incorrect, since pellicle is now known to be inherently helpful as a means of protecting the enamel from the outside world.
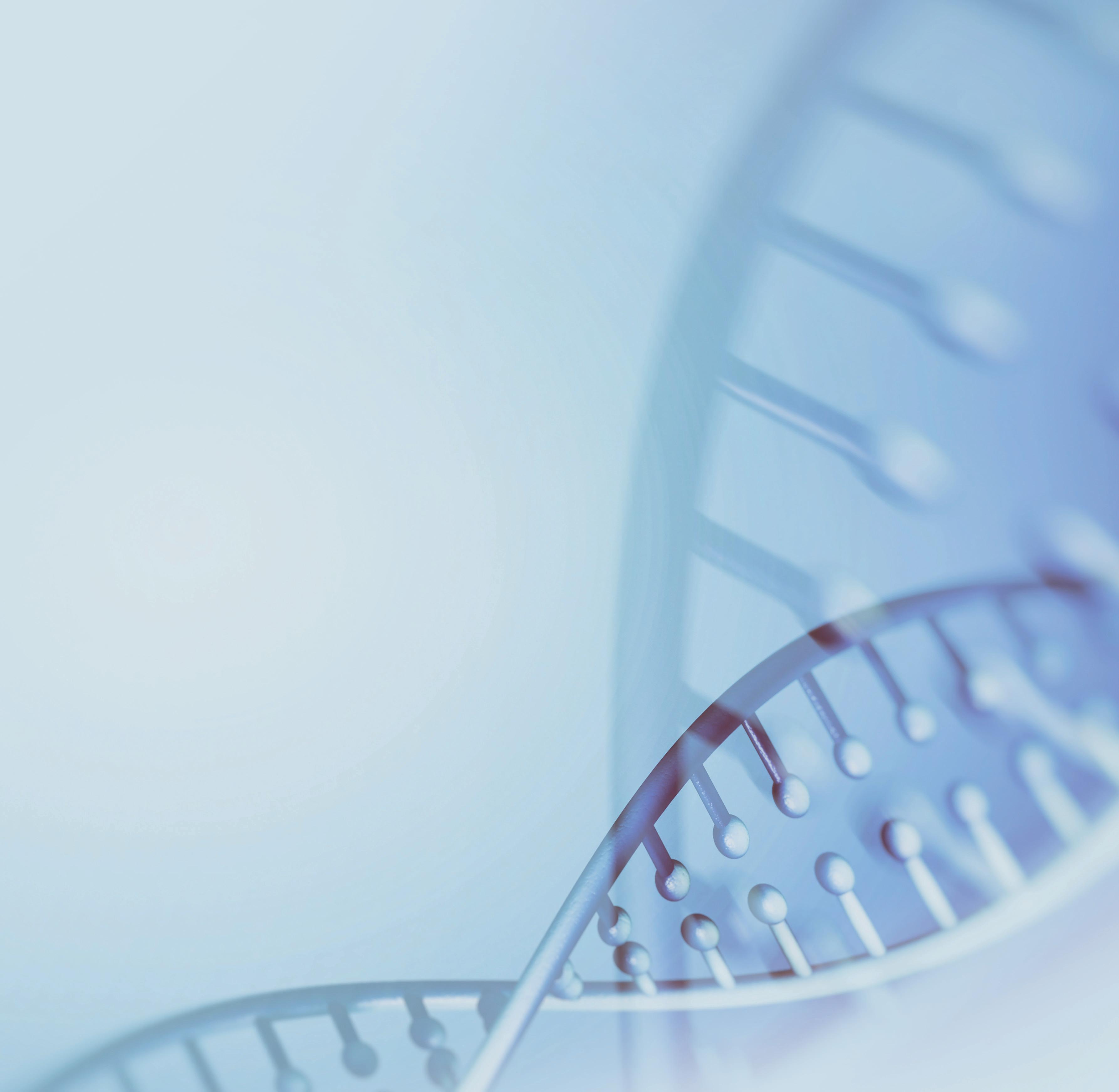
About the author
Emeritus Professor Laurence J. Walsh AO is a specialist in special needs dentistry who is based in Brisbane, where he served for 36 years on the academic staff of the University of Queensland School of Dentistry, including 21 years as Professor of Dental Science and 10 years as the Head of School. Since retiring in December 2020, Laurie has remained active in hands-on bench research work, as well as in supervising over 15 research students at UQ who work in advanced technologies and biomaterials and in clinical microbiology. Laurie has served as Chief Examiner in Microbiology for the RACDS for 21 years and as the Editor of the ADA Infection Control Guidelines for 12 years. His published research work includes over 390 journal papers, with a citation count of over 18,300 citations in the literature. Laurie holds patents in 8 families of dental technologies. He is currently ranked in the top 0.25% of world scientists. Laurie was made an Officer of the Order of Australia in January 2018 and a life member of ADAQ in 2020 in recognition of his contributions to dentistry.
References enhanced dental erosion. This explains the characteristic pattern of damage in patients with uncontrolled gastric reflux, where there is frequent regurgitation of gastric contents. The hydrochloric acid protein releases the pellicle, making the enamel completely bare and exposed, which then leads to its rapid dissolution. When pellicle is present, since it acts as a semipermeable ionic membrane, teeth are more protected from strong erosive challenges. Fortunately, manufacturers of toothpastes now understand the value of pellicle and make toothpastes with low levels of abrasivity, which means that they do not remove all traces of pellicle from the tooth surface during ordinary brushing, even for periods of up to 2 minutes. This invisible layer continues to protect us, even though we may not be aware of it.
16. Walsh LJ. Contemporary uses of CPP-ACP technologies in 2021. Part 2. Prebiotic actions. Australas Dent Pract. 2021;32(3):64-68.
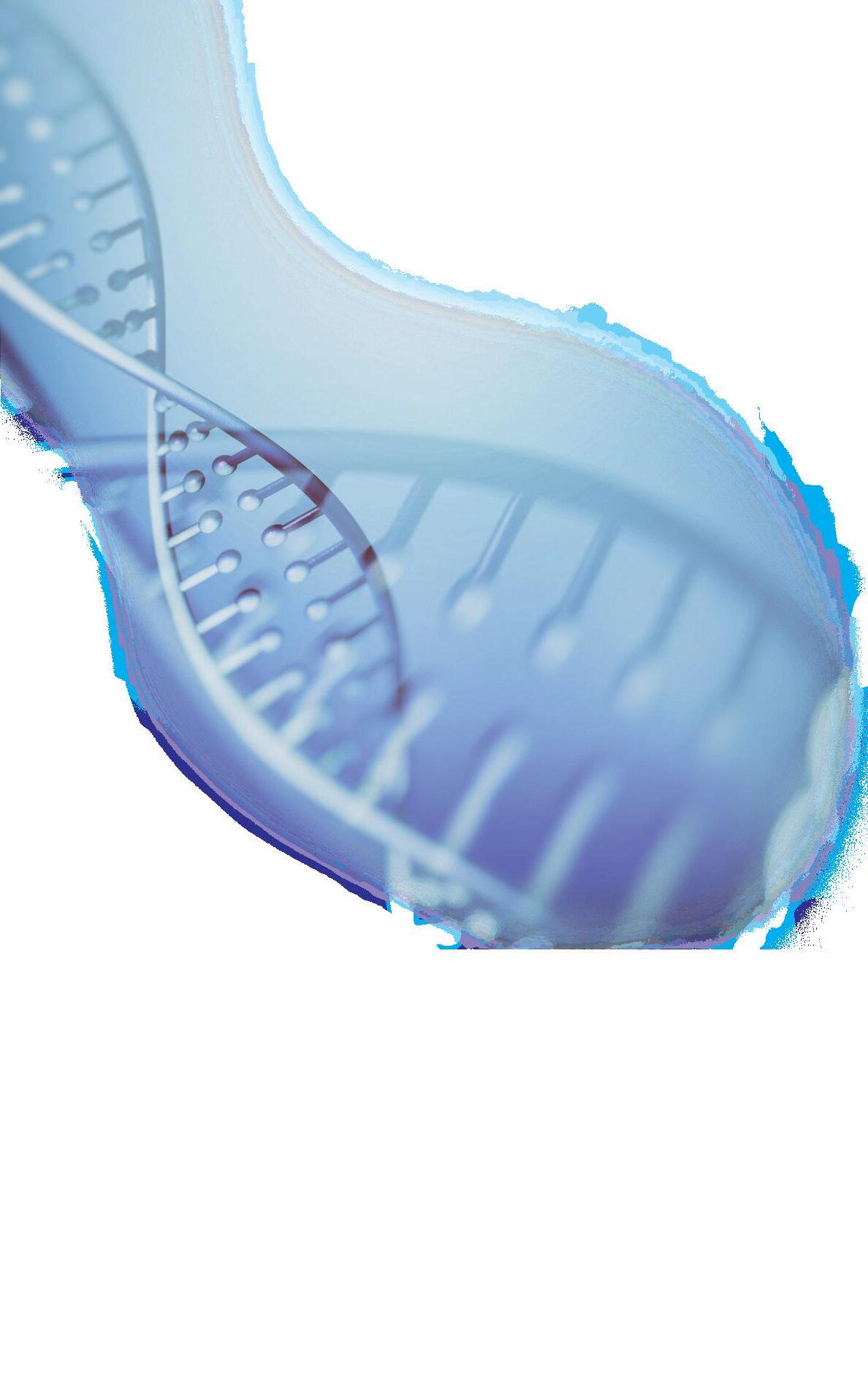
29. Vlacic J, et al. Laser-activated fluoride treatment of enamel as prevention against erosion. Aust Dent J. 2007;52(3):175-180.
2. Walsh LJ. Pit and fissure sealants: current evidence and concepts. Australas Dent Pract. 2006;17(6):122-124.
3. Charbeneau GT, et al. A filled pit and fissure sealant: 18-month results. J Am Dent Assoc. 1977;95(2):299-306.
4. Mejare I, et al. Caries-preventive effect of fissure sealants: a systematic review. Acta Odontol Scand. 2003;61(6):321-330.
5. Ahovuo-Saloranta A, et al. Pit and fissure sealants for preventing dental decay in the permanent teeth of children and adolescents. Cochrane Database Syst Rev. 2004;(3):CD001830.
6. Yap J, et al. Evaluation of a novel approach in the prevention of white spot lesions around orthodontic brackets. Aust Dent J. 2014;59:1-11.
7. Dennison JB, et al. Evaluating tooth eruption on sealant efficacy. J Am Dent Assoc. 1990;121(5):610-614.
8. Yip HK, Smales RJ. Glass ionomer cements used as fissure sealants with the atraumatic restorative treatment (ART) approach: review of literature. Int Dent J. 2002;52(2):67-70.
9. Frencken JE, et al. ART restorations and glass ionomer sealants in Zimbabwe: survival after 3 years. Community Dent Oral Epidemiol. 1998;26(6):372-381.
10. Walsh LJ. Tooth surface protection – well beyond just fissure sealing. Auxiliary 2014;24(4):22-24.
11. Arbabzadeh-Zavareh F, et al. Recharge pattern of contemporary glass ionomer restoratives. Dent Res J. 2012;9(2):139-145.
12. Perera D, et al. Acid resistance of glass ionomer cement restorative materials. Bioengineering 2020;7(4):150.
13. Mazzaoui SA, et al. Incorporation of casein phosphopeptide-amorphous calcium phosphate into a glass-ionomer cement. J Dent Res. 2003;82(11):914-8.
14. Mao B, et al. Casein phosphopeptide-amorphous calcium phosphate modified glass ionomer cement attenuates demineralization and modulates biofilm composition in dental caries. Dent Mater J. 2021;40(1):84-93.
15. Philip N et al. Randomized controlled study to evaluate microbial ecological effects of CPP-ACP and cranberry on dental plaque. JDR Clin Trans Res. 2020;5(2):118-126.
17. Walsh LJ. Applications of infrared lasers in preventive dentistry. Dent Today 1990;6:1-4.
18. Walsh LJ, Perham SJ. Enamel fusion using a carbon dioxide laser: a technique for sealing pits and fissures. Clin Prev Dent. 1991;13(3):16-20.
19. Aminzadeh A, et al. Raman spectroscopic studies of CO2 laser-irradiated human dental enamel. Spectrochimica Acta. 1999;55:1303-1308.
20. Rodríguez-Vilchis LE, et al. Morphological and structural changes on human dental enamel after Er:YAG laser irradiation: AFM, SEM, and EDS evaluation. Photomed Laser Surg. 2011;29: 493–500.
21. Shahabi S, et al. FT-Raman spectroscopic characterization of enamel surfaces irradiated with Nd:YAG and Er:YAG lasers. J Dent Res Dent Clin Dent Prospects. 2016;10:207-212.
22. Walsh LJ. Burgeoning technology: future directions in oral health. In: Dental Perspectives. An overview of clinical issues facing community dentistry. 1998;2:6-8.
23. Sandford MA, Walsh LJ. Differential thermal effects of pulsed vs. continuous CO2 laser radiation on human molar teeth. J Clin Laser Med Surg. 1994;12:139-142.
24. Ramalho KM, et al. Erbium lasers for the prevention of enamel and dentin demineralization: a literature review. Photomed Laser Surg. 2015; 33:301-319.
25. Lombardo G, et al. Sub-ablative laser irradiation to prevent acid demineralisation of dental enamel. A systematic review of literature reporting in vitro studies. Eur J Paediatr Dent. 2019;20:295-301.
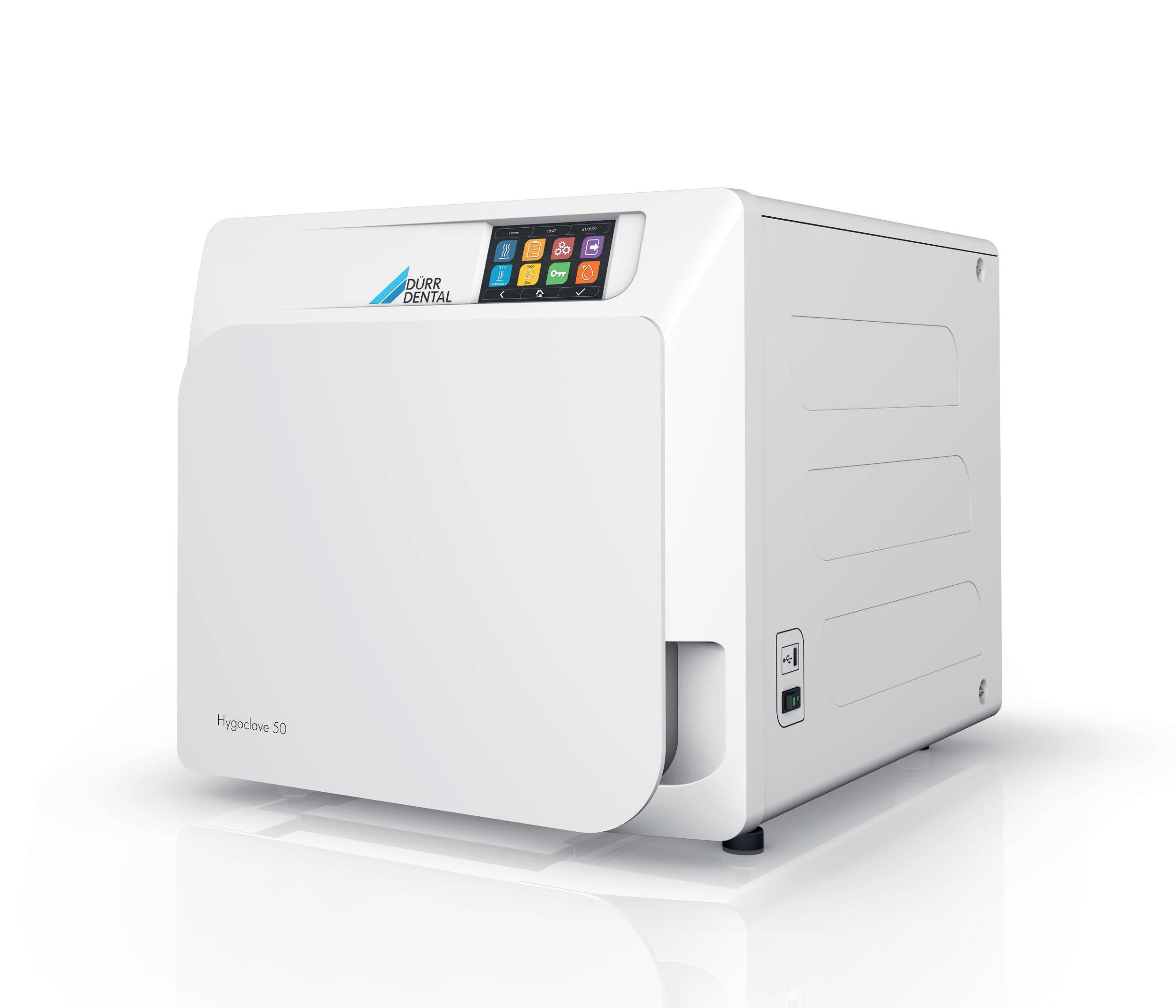
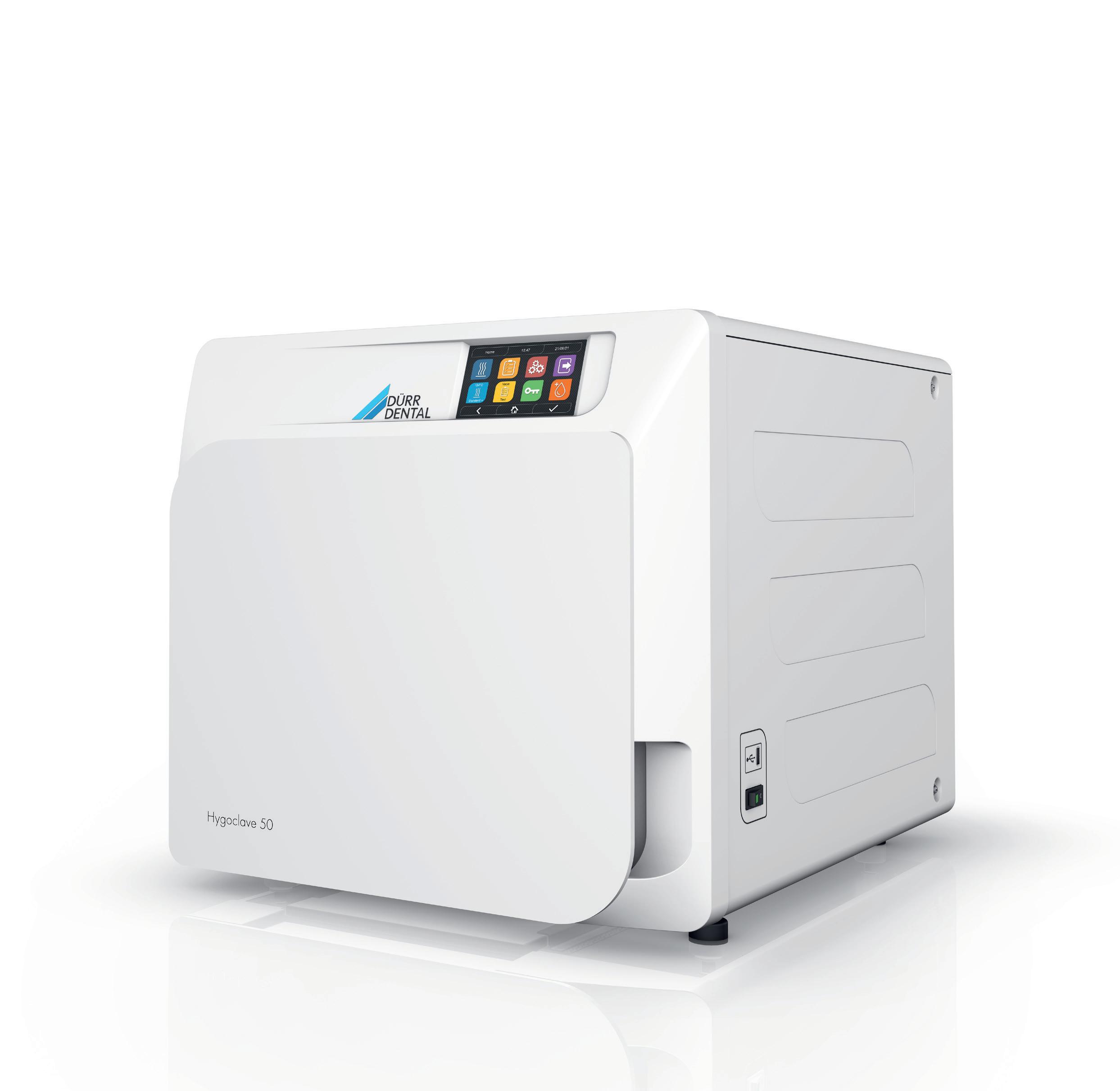
26. Al-Maliky MA, et al. Laser-assisted prevention of enamel caries: a 10-year review of the literature. Lasers Med Sci. 2020;35:13-30.
27. Pagano S, et al. Lasers to prevent dental caries: a systematic review. BMJ Open. 2020;10:e038638.
28. Vlacic J, et al. Laser-activated fluoride treatment of enamel against an artificial caries challenge: comparison of five wavelengths. Aust Dent J. 2007;52(2):101-105.
30. Vlacic J, et al. Photonic conversion of hydroxyapatite to fluorapatite: a possible mechanism for laser-activated fluoride therapy. J Oral Laser Appl. 2008;8(2):95-102.
31. Bevilácqua FM, et al. Fluoride uptake and acid resistance of enamel irradiated with Er:YAG laser. Lasers Med Sci. 2008;23:141-147.
32. Rios D, et al. In vitro evaluation of enamel erosion after Nd:YAG laser irradiation and fluoride application. Photomed Laser Surg. 2009;27:743-747.
33. Hossain M, et al. Effect of pulsed Nd:YAG laser irradiation on acid demineralization of enamel and dentin. J Clin Laser Med Surg. 2001;19:105–108.
34. Mei ML, et al. Prevention of dentine caries using silver diamine fluoride application followed by Er:YAG laser irradiation: an in vitro study. Lasers Med Sci. 2014;29:1785–1791.
35. Fekrazad R, Ebrahimpour L. Evaluation of acquired acid resistance of enamel surrounding orthodontic brackets irradiated by laser and fluoride application. Lasers Med Sci. 2014;29:1793–1798.
36. Walsh LJ. Clinical evaluation of dental hard tissue applications of carbon dioxide lasers. J Clin Laser Med Surg. 1994;12:11-15.
37. Walsh LJ. Ultraviolet B irradiation induces mast cell degranulation and release of tumour necrosis factor-alpha. Immunol Cell Biol. 73,226-233. 1995.
38. Xue VW, et al. Combined effects of topical fluorides and semiconductor lasers on prevention of enamel caries: a systematic review and meta-analysis. Photobiomodul Photomed Laser Surg. 2022;40(6):378-386.
39. Rechmann P, et al. Caries inhibition with a CO2 9.3 μm laser: An in vitro study. Lasers Surg Med. 2016;48(5):546-554.
40. Rechmann P, et al. In vitro CO2 9.3-μm shortpulsed laser caries prevention-effects of a newly developed laser irradiation pattern. Lasers Med Sci. 2020;35(4):979-989.
41. Badreddine AH, et al. Demineralization inhibition by high-speed scanning of 9.3 µm CO2 single laser pulses over enamel. Lasers Surg Med. 2021;53(5):703-712.