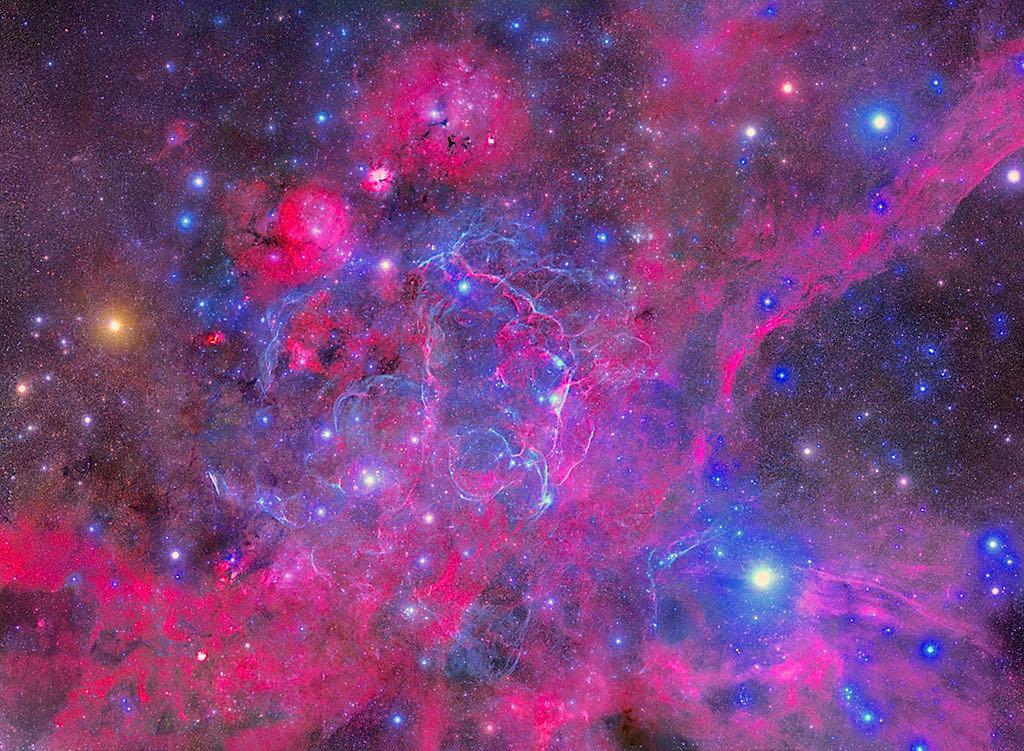
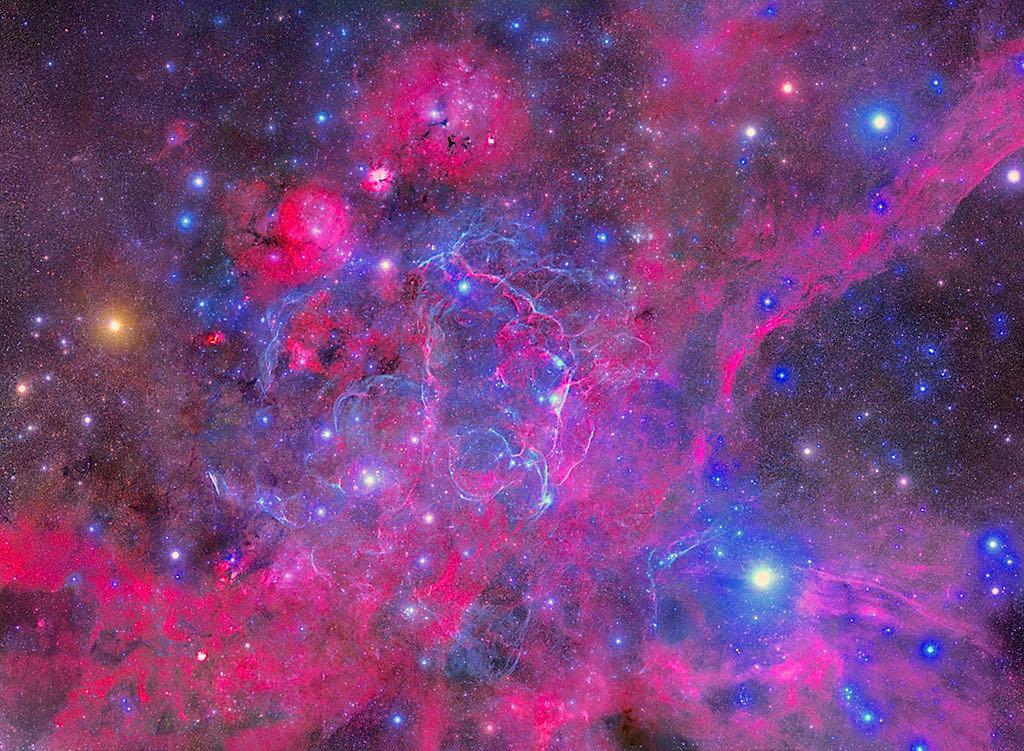
Beauty in the Beast
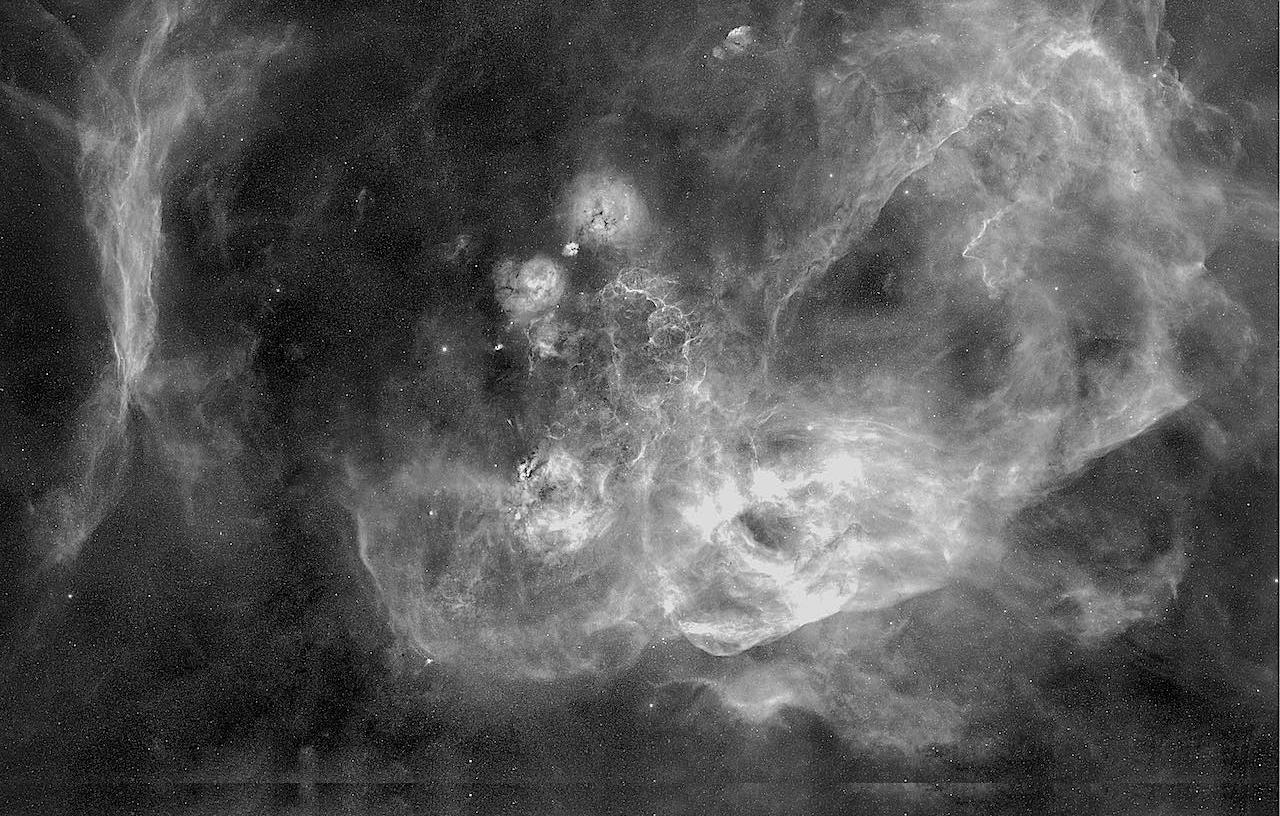
Named for the cosmic cloud hunting Australian astronomer Colin Stanley Gum (1924-1960), The Gum Nebula is so large and nearby it is challenging to image. This image is a crop from a 111-tile hydrogen alpha mosaic imaged from Coonabarabran, NSW by John Gleason. The nebula’s nearest edge is 450 light-years from Earth; the far edge is 1,500 light-years away. This 40-degree-wide field reveals numerous supernova remnants from a few thousand years to over a million years old. Multiple supernovae over such a lengthy time span suggest that the original star cluster formed progenitor stars weighing from 8 to 20 solar masses. This wide field view reveals traces of several supernovae embedded in The Gum Nebula. The Vela supernova remnant in the series of thread-like arcs in the center. The younger Puppis supernova remnant is above it to the left. The entirety of this complex is known as the Vela Molecular Ridge.
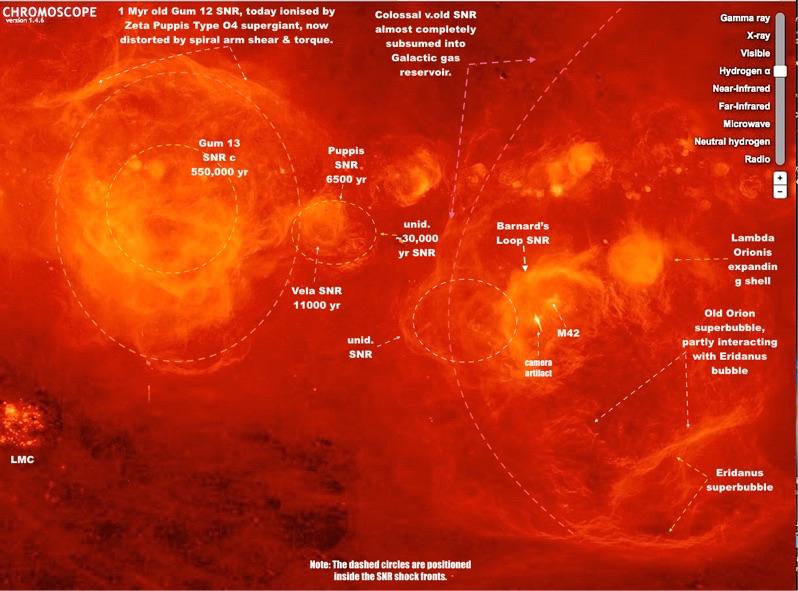
The region around the Vela SNR is a sizzly neighborhood. Hydrogen alpha emits its characteristic reddish radiation between 11,000 to 36,000 K. In supernova remnants the temperature can rise to more than 1 million K.
The Gum 12 Nebula in the upper left is an immense, tenuous, diffuse ancient supernova remnant, the largest in the entire celestial vault. It is invisible in the optical bands, but breathtaking in hydrogen alpha and far IR. Its center is located about 450 parsecs from the solar system. These fading wisps are dissipating remnants left over after multiple supernovae 1 to 3 million years ago. Gum 12 is the largest SN remnant in the Milky Way. It is an important study in the extent and age an expanding supernova bubble propagating outward from supernova explosions within a galactic arm. Its history includes interactions between the supernova blast wave and molecular clouds, collision with another supernova remnant, and triggered star formation on the leading edges of its shock front.
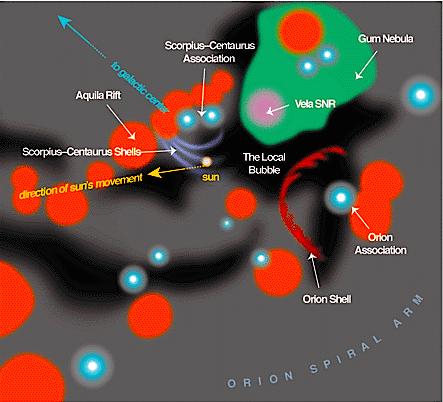
Location of the Vela SNR, Gum Nebula, Orion Shells, Sco-Centaurus stellar association, and Aquila Rift, all within the Orion Spur, which partially bridges the Sagittarius–Carina Arm and the Perseus Arm. Currently, the Sun is passing through the Local Bubble, shown in violet, which is flowing away from the Scorpius-Centaurus Association of young stars. The Local Bubble is a low-density vacuity in the interstellar medium (ISM), which is shown in black. The green Gum 12 Nebula is a region of hot ionized hydrogen gas. Inside the Gum Nebula the Vela Supernova Remnant is shown in pink. The Vela Remnant is slowing and will in time fragment into partial shells of material, which in turn will dissolve into the Galactic gas medium. Graphic by Linda Huff and Priscilla Frisch, APOD 17 Feb 2002.
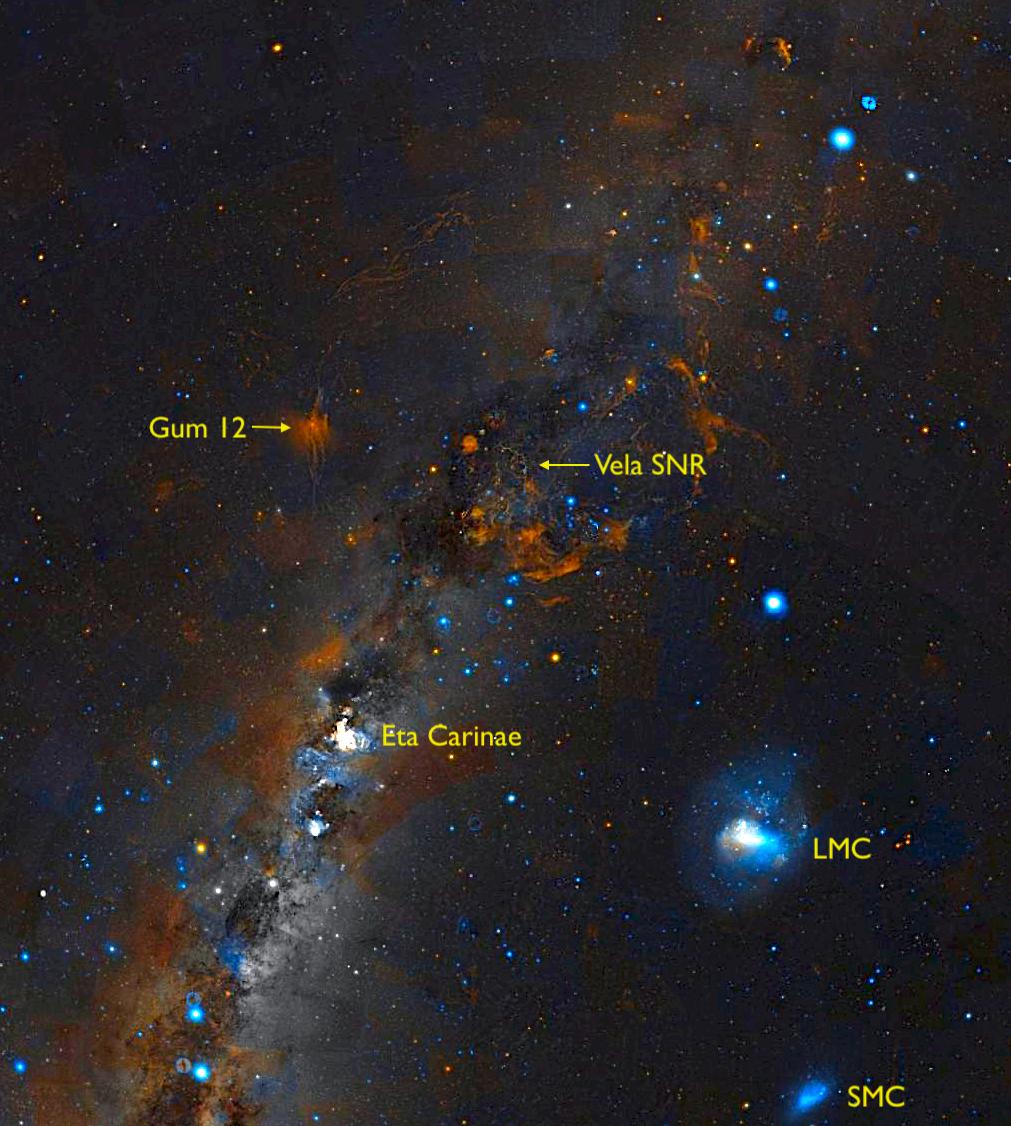
The Vela SNR and the entire Gum Nebula complex are invisible in the visual band — and barely discernible even in this saturation-enhanced clip. The complexity of the Milky Way that we perceive as a vast panoply of ghostly glitter is actually a very slowly boiling Galactic tea kettle. The Galactic disc boils with seething gas bubbles and stellar steam, but the boil is so slow we see it only as seemingly unchanging puff patches in the sky. It is indeed a wonder that anything so fragile and fleeting as life can occur anywhere in this maelstrom. Considering that we see only about one percent of the Galaxy’s total luminosity, what our eyes tell us about the universe is roughly the same as what an ant’s eyes tell the ant about the history of humanity.
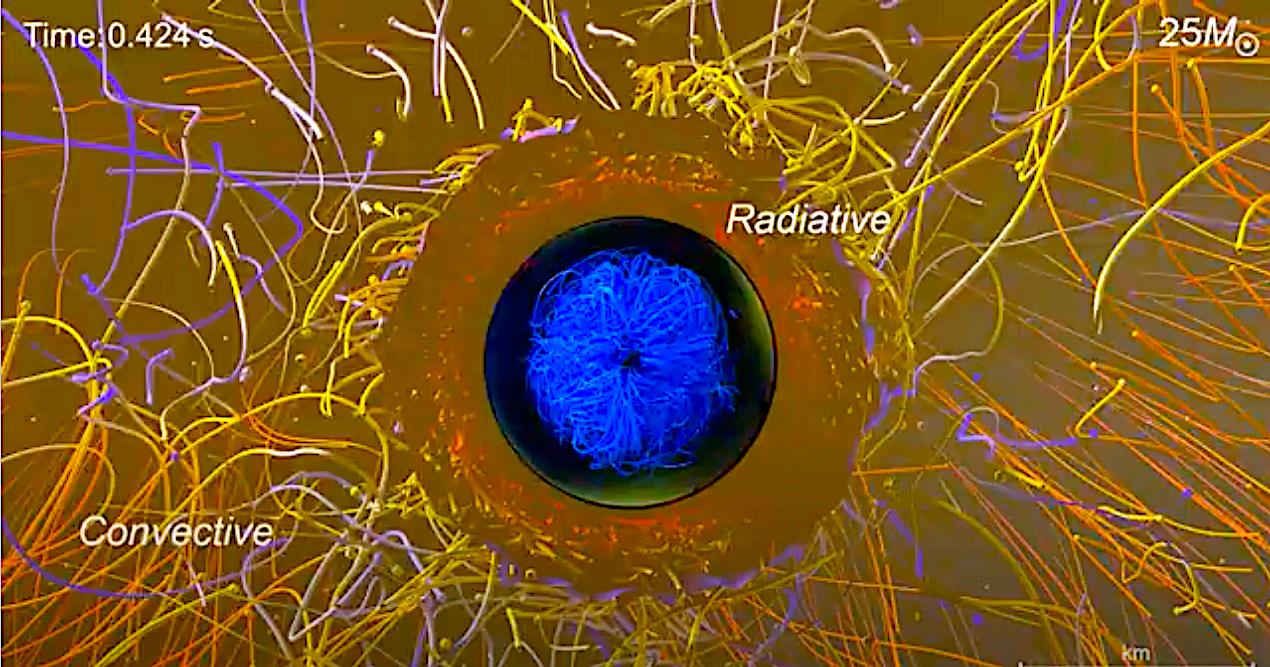
Visualizing the Supernova Explosion of a 25-Solar-Mass Star
https://youtu.be/0DSk1wPc_cE
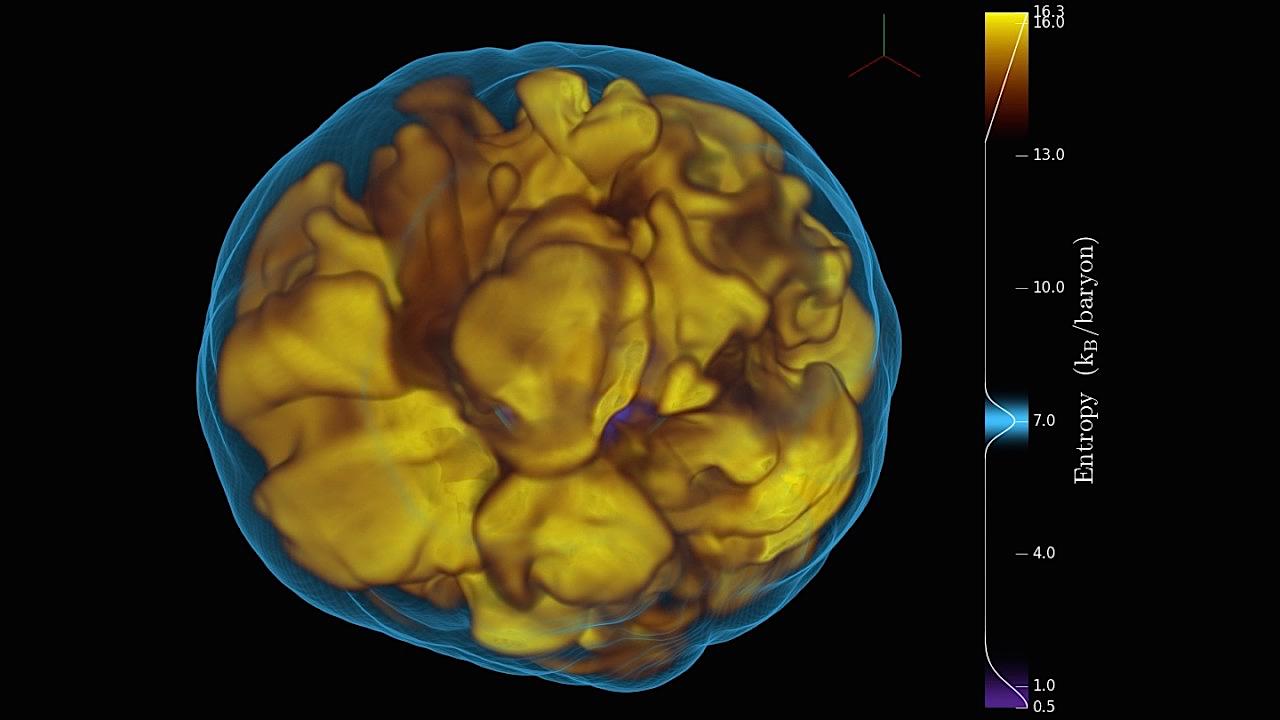
Detailed description of a core collapse supernova https://www.youtube.com/watch?v=Yt-SBT7nNfU
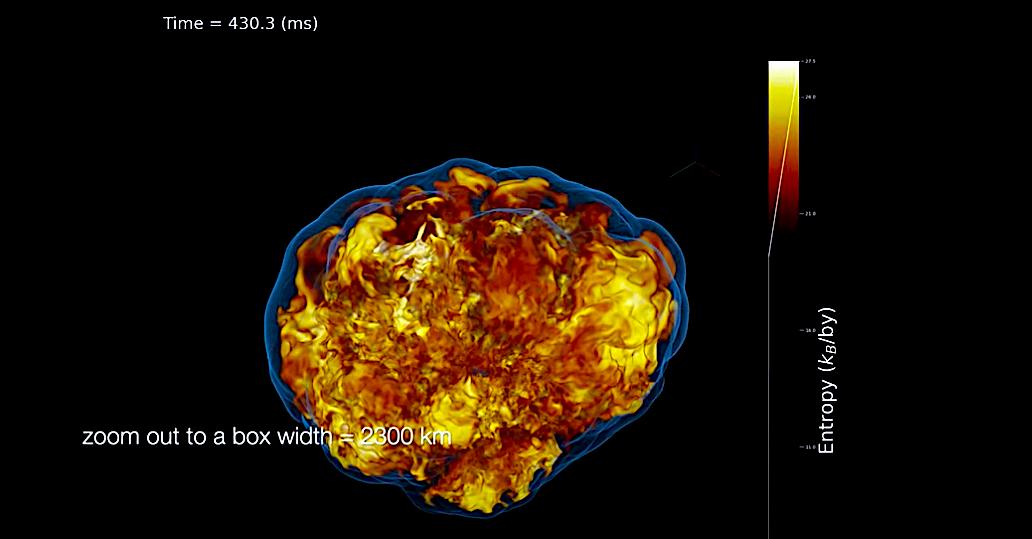
3D Self-consistent Core-Collapse Supernova Simulation
https://www.youtube.com/watch?v=bxKwMGgAkdQ
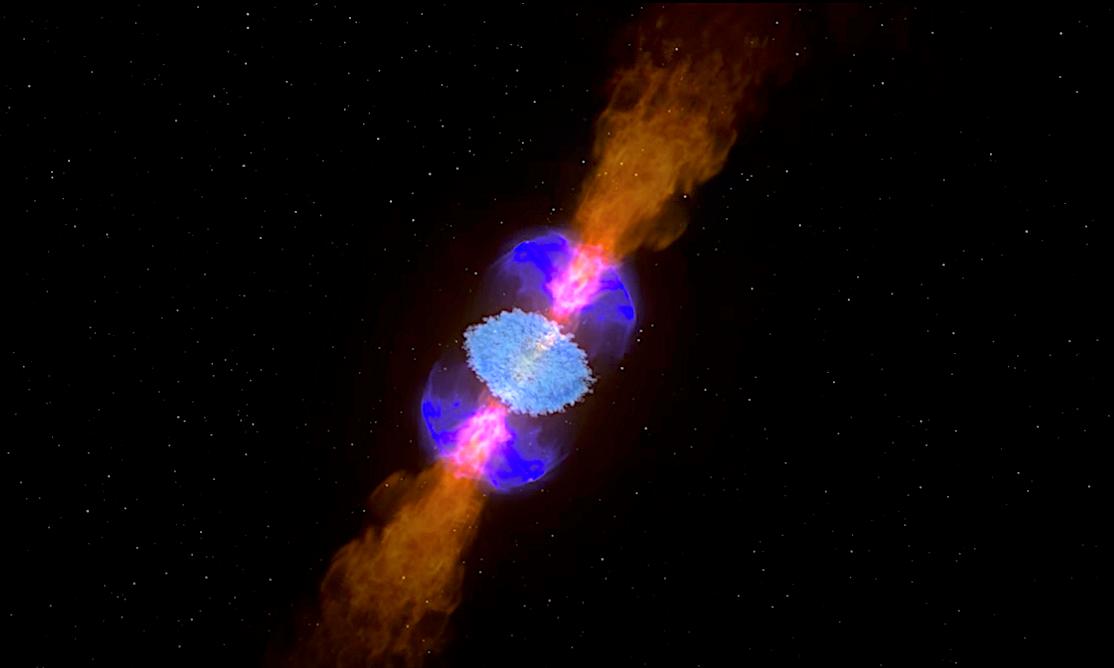
Supernovae: The Most Extreme Explosions
https://www.youtube.com/watch?v=lAgwMGRvJrM&t=628s
Supernova remnants are not simply hot, dense bubbles that get thinner as they get bigger. During the violent moments before the star’s explosion, its core was a seething, writhing, gyrating mass so fissile and unstable it barely held itself together. The above simulations showed the maelstrom in a core-collapse star’s interior before the whole thing was blown to bits.
The outermost layers of the expanding ball do not erupt into a nice, tidy sphere. There is a lot of free atomic hydrogen gas blown from the outer layers of the star which expands in a wobbly spherical ball, but there are also multitudes of shreds and blobs in the star’s deep convection cells that retain their chemical identity when it disrupted. Some of the blobs were originally deep inside the star, down to the surface of the fusion core. As those blobs hurtled into space, they distended, writhed, fragmented. The thin space into which they flew was not evenly spread. Space throbs with atomic and molecular clouds, star clusters, slender gas/dust lenses, and long sheets of gas/dust filaments. The massive molecular clouds from which stars are born are vaporous on their outer surfaces — 10 to 100 atoms per cubic centimeter on the exterior but up to a million or more atoms per cc in their brutally cold cores — but they make up for it in size, 50 to 300 light years in diameter and many million suns in mass. “Empty” space is riven with dense pockets of gas from turbulent shocks that propagate incessantly in a spiral arm disc thanks to the shear and torque of the disc rotating at different speeds.
A supernova shock wave is a messy overdense region hurtling into a messy underdense region in a messy spiral arm. Space is a housekeeper’s nightmare.
Within a few hundred years the blobs of progenitor gas distend as they expand into the clumpy Galactic medium. Bubble-like shells and writhing streamers evolve. Most young supernovae bubbles, e.g., the Tycho SN in 1572, are still gravitationally contiguous enough that they retain their basic round shape (which is common with white dwarf supernovae). That coherence of shape is maintained for hundreds to thousands of yeas as the expanding bubble adds mass from the gas that it compresses ahead of it. The rounded shapes will slowly degrade as more and more gas is snowplowed ahead of it, until the point where the supernova shell has accumulated about 10 times its original mass.
After a few ten thousands of years the remnant disperses into the complex set of filaments, intersecting rings and shards (e.g., the Pencil Nebula, which part of the Vela SNR). Each filament has its own local velocity, chemical composition, and vector toward which it is expanding. The slight differences in chemical composition from filament to filament trace back to the layers inside the progenitor star where the blob was hurled by the blast. Some blobs lob through space like cosmic shrapnel. Vela has at least five of these blobs which have held together 11,000 years.
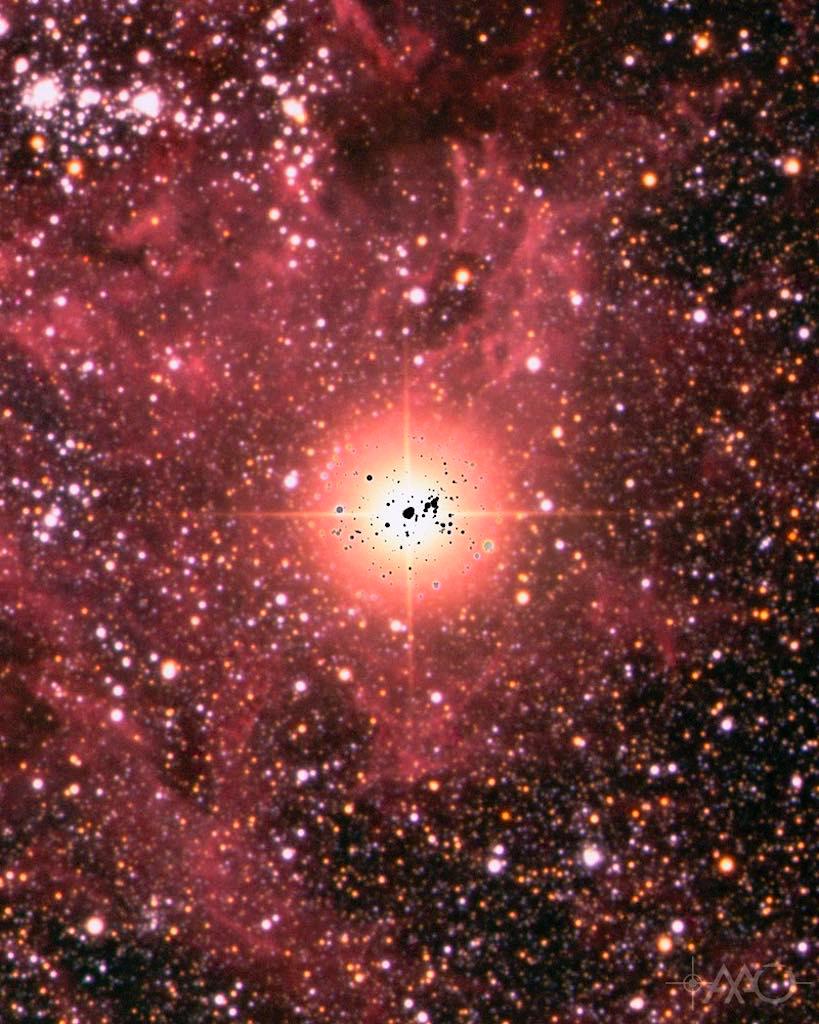
The Large Magellanic Cloud supernova 1987A two weeks after the initial detonation was detected as an excess of galactic neutrinos by the Ice Cube observatory at the South Pole in February 1987. The redyellow ball of light belies the rather small amount of energy that ended up in the visual bands. Far more energy arrived in the UV and X-ray bands. All of it was emitted by gas propagating hypersonically (as high as Mach 1000) through the thin, cold interstellar gas. Since it is difficult to visualize how large this light patch was, David Malin of the AAU in Australia superimposed a negative photograph of the foreground stars on our side of the blast. The dots have been sized according to their diameters, red giants being the largest. The star which went supernova had been previously observed and catalogued as Sanduleak –69°202. Photograph made from plates taken in March 1987. The image width is about 8 arc min. Source: David Malin, Australian Astronomical Observatory.
What happens aer the detonation
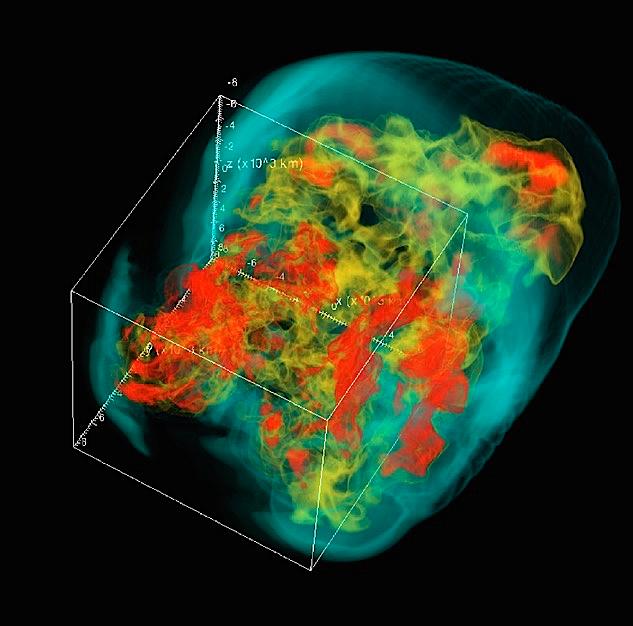
The highly chaotic, filamentary, bubble-like character of a Type II core collapse supernova shock wave two seconds after the infalling mass of the initial collapse has bounced off the surface of the neutron star. Already the key features of the late-phase supernova remnant are apparent — filaments, bubbles, threads, high-density hot spots. This MHD simulation shows only the region around the core bounce in which the actual explosion has occurred. The thick envelope layers of the star surrounding this core will be shattered into clumps and threads during the ballistic rebound of the chaotic masses seen here. Note the lopsidedness — a hallmark of core collapse implosions.
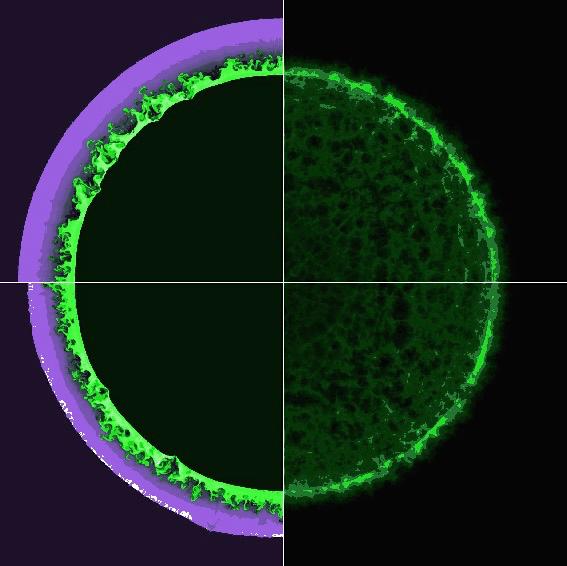
Snapshot of a lower-mass 8–11 solar mass supernova blasting into the blissfully unaware outer envelope of the star. The writhing fingerlings of exploding gas burst violently into the envelope, forming curlicue RayleighTaylor instabilities as they crash into the envelope gas (purple). Each of the volumes enclosed by the space between those fingerlings may retain enough internal gravitational coherence to end up a blob or filament flying into space. The fingerlings themselves are already a super-hot gas and will stay a gas as they move out into space. This is how the Crab Nebula, SNR 1006, and Tycho 1572 SNR in Taurus acquired their beautiful blob-&-thread structure.
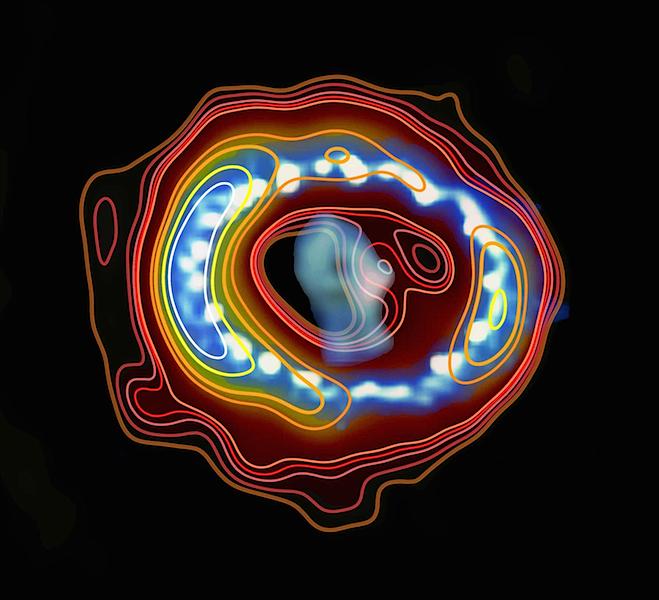
HST image with graphic overlay showing the torus of radio emission in the SN1987a supernova in the Large Magellanic Cloud. Astronomers in Australia and Hong Kong assembled this image in 2013 to combine an overlay of radio emission (contours) with a Hubble Space Telescope image of the supernova (blue ring and bright spots). This image traces a compact source, a pulsar wind nebula called a plerion, in the center of the radio emission. The Crab Nebula pulsar is another example of a plerion SNR. Source: ICRAR Australia.
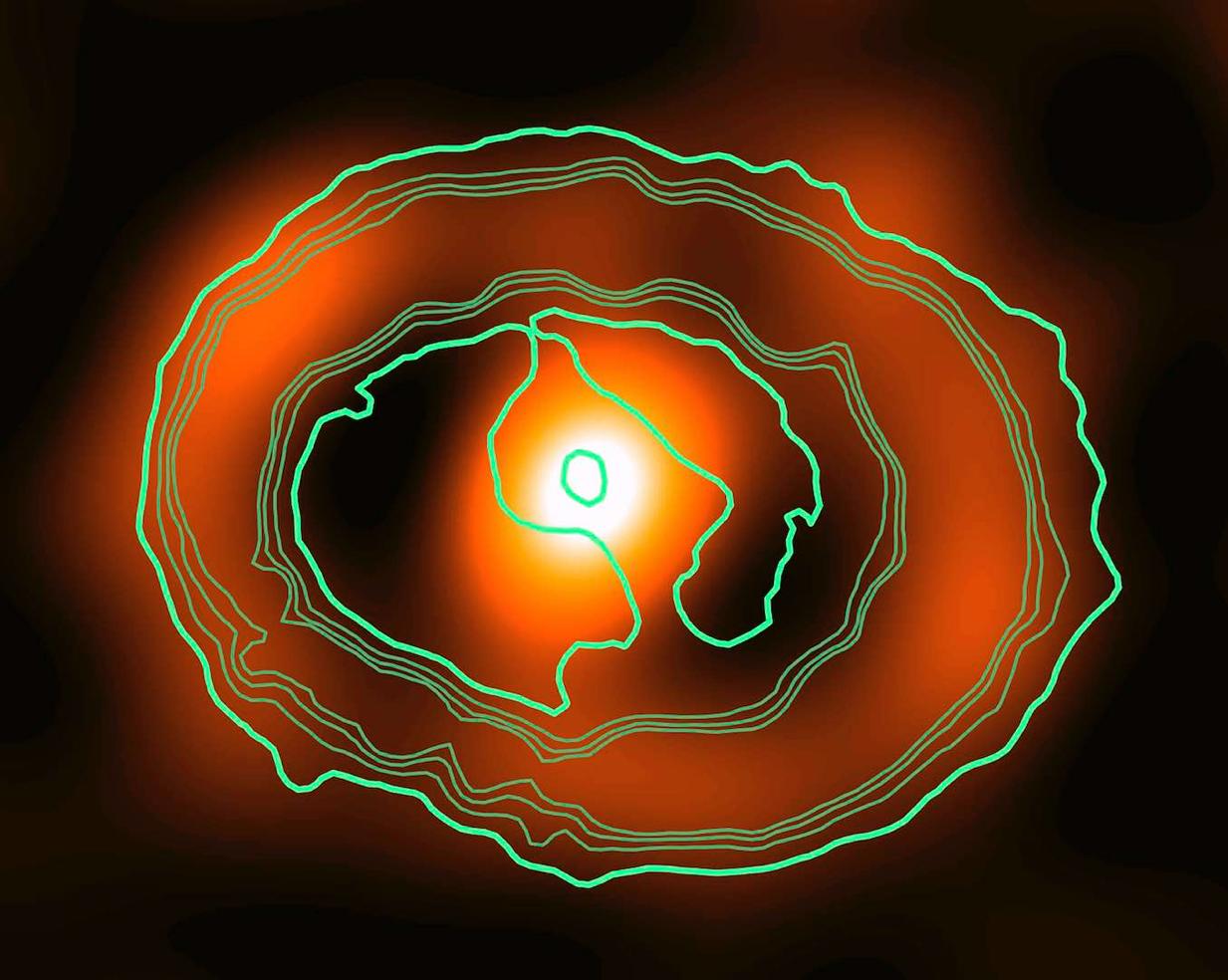
YouTube of time-lapse video showing the aftermath of the supernova SN 1987a in the LMC over a 25-year period from 1992 to 2017. The shockwave is slamming outward into pre-supernova debris rings that the star expelled in its seething adolescence as it swerved between red supergiant/blue loop/blue supergiant stages before ending it all in a high-speed supernova crash.
And we think OUR kids are a handful!
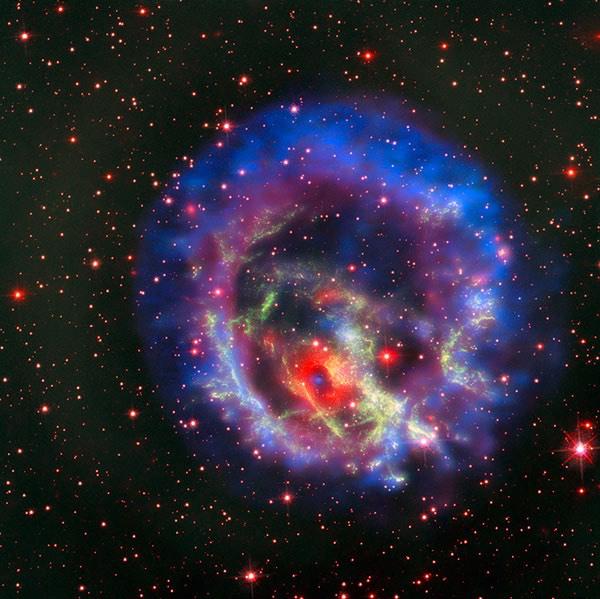
This image of the supernova remnant E0102 shows both X-rays (blue) and visible light (red and green). The faint X-ray source at the center of the red ring is the neutron star progenitor of the supernova. The larger X-ray ring was known for a long time, but data from the ESO Very Large Telescope in Chile led to the discovery of the smaller ring, which emits visible light at a narrow range of wavelengths. The greenemitting gas blobs on the outer periphery are self-cohesive clumps from deep within the star that were ejected during the explosion.

Before a supernova like SN 1987a explodes, it goes through a prolonged death agony. SN 1987a’s progenitor star Sanduleak -69°202 underwent both a red and blue supergiant phase. During these phases, it ejected material into concentric ejecta rings speeding away from the star. As a high-mass star transitions between supergiant phases, the star pauses in a stasis called a Blue Loop in which less gas is ejected. The creates a multi-bubble structure that astronomers can use to reverse engineer the sequence of a supernova’s death throes. When the core finally goes supernova, it ejects material at a much higher velocity than during its earlier supergiant phases. The hypersonic shock overtakes slower ejection shells and slams into them so hard that rebound shocks move backward toward the original progenitor star. Cosmic rays are protons and electrons violently accelerated as they ricochet back and forth between forward and reverse shocks. A cosmic ray particle can build the kinetic mass of a premiere-league pitcher’s zingiest fastball.
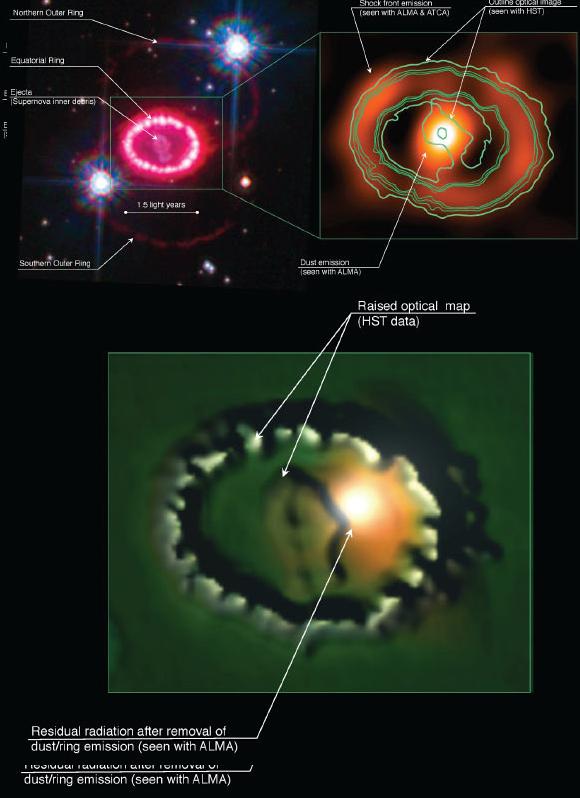
• Upper left panel: SNR1987A as seen by Hubble in 2010.
• Upper right panel: SNR1987A as seen by the Australia Telescope Compact Array and the Chilean Atacama Large Millimeter/submillimeter Array.
• Bottom panel: a computer generated visualization of the remnant showing the possible location of the pulsar. The “diamond necklace” structure is caused by the blast wave hitting dust-rich gas clumps. Image credit: ATCA / ALMA / G. Zanardo et al / NASA / ESA / K. France, University of Colorado, Boulder / P. Challis and R. Kirshner, Harvard-Smithsonian Center for Astrophysics.
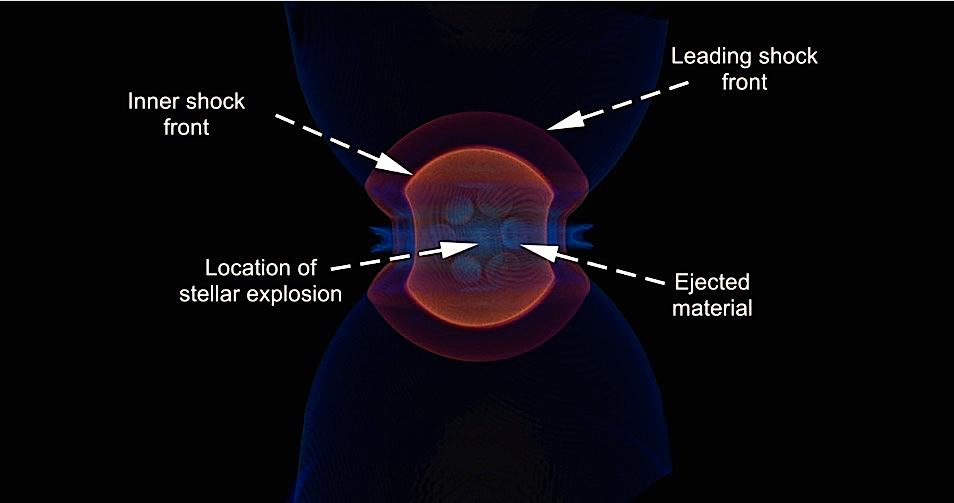
Full-motion video of a three-dimensional simulation of the development of SN 1987a between 1989 and 2014. The model shows that the SNR developed more strongly and rapidly on one side. Lopsided SNRs are not unusual. There are several reasons for deformed SNR expansion shells — an off center detonation in the progenitor; the presence of a nearby binary companion; or significant inhomogeneities in the surrounding interstellar medium. See this ICRAR Australia web page for 163 more astronomy visualisations and lectures.
The Vela SNR Xray surface is still energetic enough to emit X-rays even after 11,000 years. Note the multiply punctured bubble morphology. The white spot in the upper right is the younger, hotter Puppis SNR, four times more distant than Vela.
Source: ROSAT/ XMM Newton.
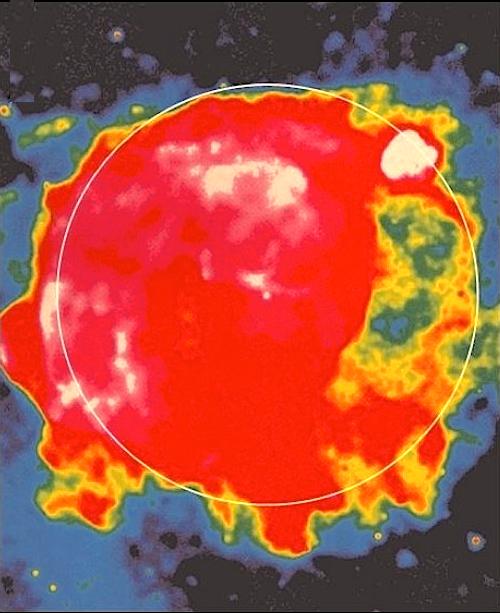
Right: Several milliseconds before the collapse of the iron core into a neutron ball, the regions shown in red are a seething cauldron of giant density bubbles writhing and intermingling so chaotically that it beggars verbal description. The net effect is to destroy the star’s once-intense magnetic field (blue lines). Without the stabilizing effect of a magnetic field, the exploding core is governed by the physics of density gradients in extreme gravity. One effect is the ejection of giant blobs of matter that move ballistically, faster than the star’s unbound gas. These literally spit into space.
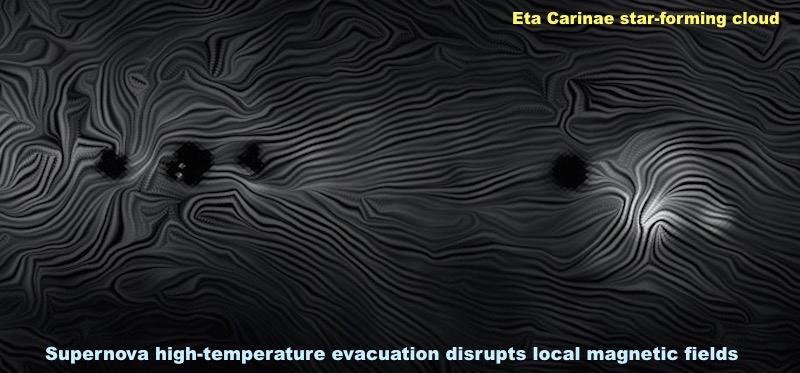
Deep within the core of the pre-SN star, the intense writhing bubbles of extremely dense gas destroy the core’s magnetic field. The now magnetically chaotic core expands with the rest of the envelope into the Galactic medium. By the time the bubble reaches the state shown in this image, the tiny magnetic hole in the core of the star has blown a gigantic magnetic hole in the galaxy disc. Here we see four of them.
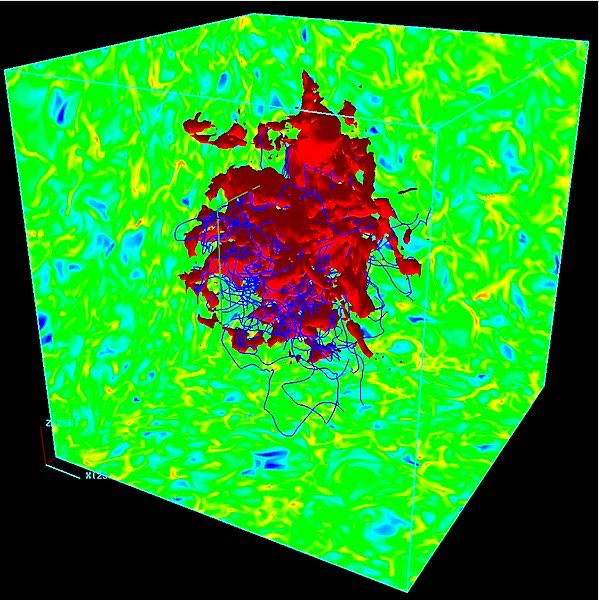
Flying through a supernova remnant would be like flying through a hailstorm with baseball-sized stones.
The Vela SNR was examined as an X-ray source by researchers using the 1990s era ROSAT satellite, which was designed to survey the heavens in X-ray bands. In this image and the one on the upper left of the previous page, the surface of the Xray emission is raggedly spherical. Images in the optical bands show a tattered patchwork of disrupted arcs expanding into the tenuous gas of the disc. The dramatically different appearances tell us much about the complex matrix of gas clouds that invisibly inhabit the disc plane of a spiral galaxy.
When a supernova detonation bursts through the photosphere of its star, for the first 100,000 to one million seconds (>1 day to 11 days) an extremely hot fireball of gas blasts out in all directions at hypersonic velocities as high as Mach 1000. The gas behaves as a fluid as it moves into the cold, gas of the interstellar medium (ISM). The ISM has typically fewer than ten hydrogen atoms per cc and is a nippy 50–100 K. The expanding shock front flash-heats the ambient gas so much that it blazes in X-rays — it is much too hot to emit in the optical band. The Vela SNR is still glowing in X-ray after ~11,000 years.
During the early days after the blast the ejected gas was expanding faster than the ambient sound speed — so fast that shock waves could not pile up disc gas in front of the fireball. Instead it simply elbowed its way through. The fireball did not expand evenly in all directions because of its clumpy composition. Today’s X-ray surface still reveals the under- and overdensities in the original round fireball shape, which we see at the mottled surface structure.
After a period of 100 to 200 days the shock wave slows to 200–400 km sec. At this velocity disc gas can pile up in front of it as compression fronts. Once the expanding gas has piled roughly ten times the mass of ambient gas it begins to emit strongly in H-alpha and O-III in the optical band. We see the vivid results of this stage in the blue OIII emission in astro-images of Vela’s shreds. The OIII / Hα gas fronts can be 5 to 20 light years thick.
Eventually the blast wave catches up with the earlier supergiant ejecta shells and crashes into them. One result is a rebound wave which (a) compresses the shocked gas in front of it to incandescence (red Hα in astro images) and (b) rebounds back toward the original star. High-energy cosmic rays are generated in the rebound interface. The fact that the ROSAT image was snapped roughly 11,000 years after the initial explosion reveals a great deal about the violence of a supernova and the feeble resistance of interstellar gas. Today the X-ray shell is immense — 230 light years across — yet it is still hot enough to generate copious quantities of X-rays.
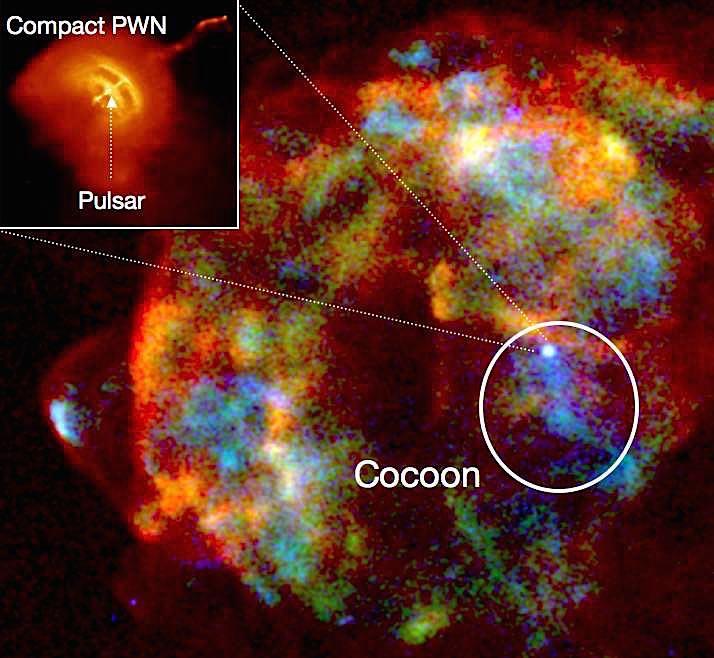
The neutron star at the heart of Vela spins around its axis once every 11.2 seconds (89.3 milliseconds). Gas spinning around its equatorial plane is funnelled toward the poles via magnetic fields. There it is ejected at near-relativistic velocities. The result is a pulsar wind jetting outward along the polar axis. The pulsar wind originated very early in the neutron star’s career. The primordial jet blasted through the star’s envelope sooner and faster than the cooler optical-band gas behind it. The result was large clumps of the star’s matter being ejected like howitzer projectiles. Those blobs are still detectable even today. Watch this ICRAR video to see how it works.
Right: Most disc galaxies produce many supernovae going off across a long time span. See the SILCC simulations to understand what a violent history this bequeathes to a galaxy. Multiple supernovae across a long time, say 100 million years, eventually produce a galactic chimney. Here, the galactic chimney erupting from the center of the Milky Way (yellow-orange areas) is attributed to the supermassive black hole at the center of our galaxy. Similar chimney structures result from multiple supernovae. Credit: Gabriele Ponti/MPE/INAF and Mark Morris/UCLA.
Read more in this phys.org report.
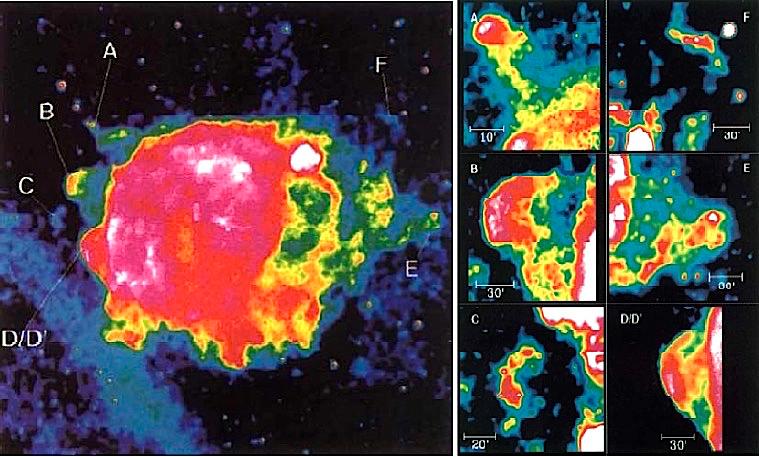
Above: Spectral analysis of the several large blobs A–DD’ moving far ahead of the supernova blast wave showed that each of them contained ejecta with different spectral abundances. Fragment A above was silicondominated, while fragments B and D were neon, magnesium, and oxygen dominated. These elements are produced in different layers of a star’s atmosphere. Silicon blobs originate so deep in a star that they are just above the exploding core. This confirmed the hypothesis that the thick envelopes in a star whose core is undergoing neutron-star collapse are not atomised by the neutrino barrage or detonation shocks. Instead, some envelope material is blown off in large self-coherent clumps. Different clumps originate in different layers of the pre-SN star in which metals of a given species dominate. Fragment D/D’ compresses the Pencil Nebula.
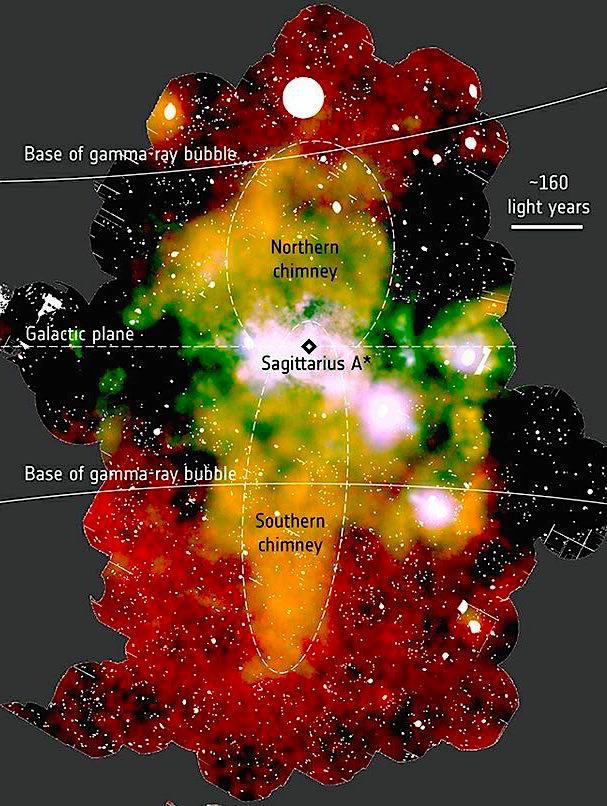
Right: This image of the Vela supernova remnant focuses on “shrapnel” fragments of materials ejected from deep within the star. The composition of these shrapnel was curious because one of them, indicated by letter A in the image, shows a high silicon abundance in the X-ray spectrum while other pieces of shrapnel showed a rich content of oxygen, neon and magnesium.
Even more intriguing, another bit of silicon shrapnel was detected at the letter G in the image. The Vela pulsar was right in the middle. This suggested that two bits of shrapnel were dredged up from different layers of the star and hurled out by the Vela pulsar’s polar jets. The silicon layer is where silicon fuses to become iron, generating 3-billion K temperatures. The silicon-burning phase lasts about 1 day, leaving only an iron core behind. Iron does not produce energy when it fuses; it consumes energy. Hence the enormous heat source in the center shut down and the iron core collapsed into a neutron star. Extreme-energy polar jets were quickly produced by the jets. These hurled large wads of the deep layers straight through the outer layers — just like shrapnel from a grenade. The fragments flew out and are seen today as objects A and G. They flew well beyond the boundary of the expanding fireball. This complex series of reactions occurred in the span of a few seconds.
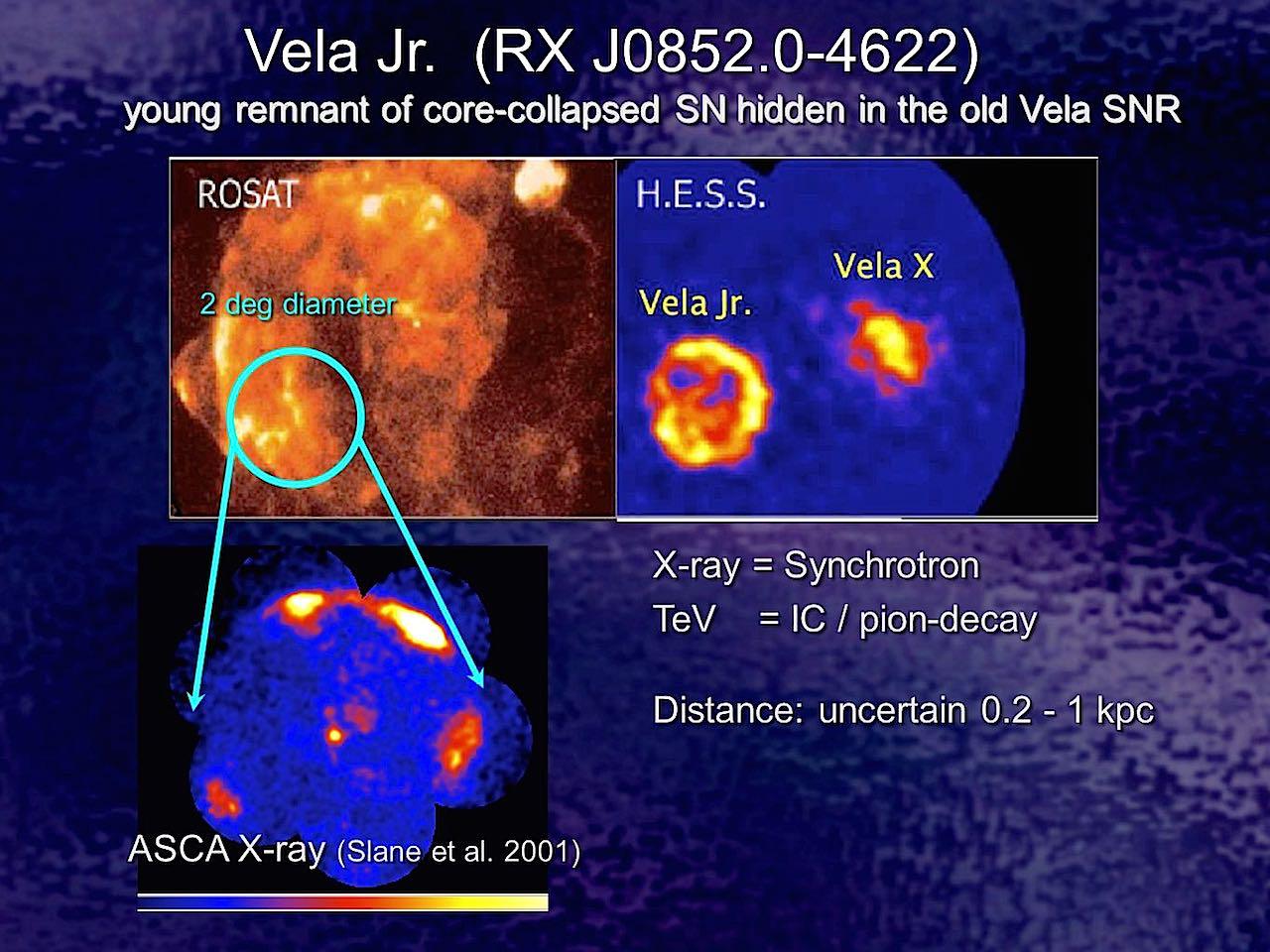
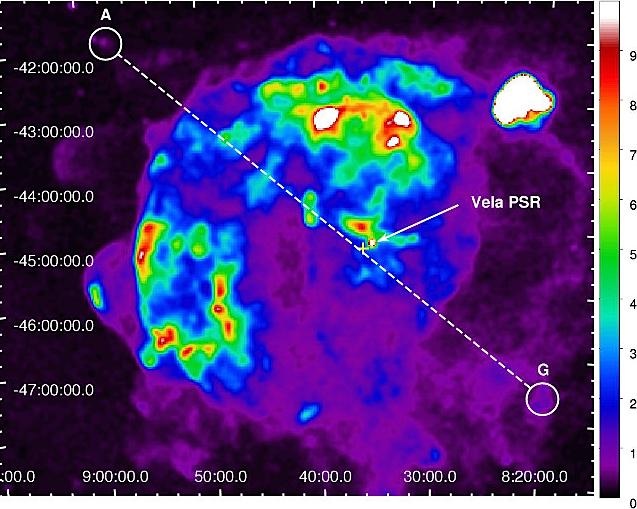
Left: The Vela SNR had a few surprises in store for astronomers tracking down the sky’s mysteries. In the 2000s the standard bands of X-ray, UV, optical, millimeter, micron, and radio were joined by the addition of gamma-ray telescope systems such as the H.E.S.S. twin Cherenkov radiation detector dishes in Namibia. In 2005 H.E.S.S discovered traces of extreme-energy teravolt cosmic rays emanating from the Vela SNR, but off-center from the pulsar region. Cherenkov radiation is photon emission generated by electrons helixing around magnetic field lines. The H.E.S.S. detections had energy levels 1000 times greater than gamma, which is produced when extremeenergy cosmic rays strike atoms in the Earth’s atmosphere and initiate an energy-loss sequence starting with pion decay, which results from teravolt cosmic rays impact ordinary protons. Gamma-energy cosmic rays were already known to originate in the colliding-wind shock front of expanding SNRs, but the H.E.S.S. data suggested that a new source was not actually related to the Vela SNR.
Vela Jr: Could there by another SNR hiding behind the hot emission of Vela?
Six years later a team led by Yasunobu Uchiyama of the Japanese Susaku satellite team verified suspicions raised in the H.E.S.S data. Indeed there was a very young “Vela Jr.” Type 1a white dwarf SN shell hiding under the skirts of the old Vela. Read the full report here.
The four types of supernova shells
Supernova remnants are classified into four categories based on their morphologies:
Shell type SNRs are structurally the simplest of all supernova remnants. They are characterised by a limb brightened shell of shock heated plasma generated as the blast wave of an SNR travels through the ISM. Limb brightening looks like a ring around the stellar remnant. It is caused by more material being along the line of sight near the edges of the remnant. SN rings not only look like a planetary nebula, they respond to an OIII filter like a planetary nebula.
Plerions, also know as pulsar wind nebulae have the bulk of their emission radiating from near the centre of the remnant, where there is a rapidly spinning neutron star. They are characterised by a highly energetic wind of relativistic electrons and positrons driven by the neutron star’s rotation. See this ICRAR simulation. The wind terminates in a shock where it meets the ISM. The particles are accelerated to relativistic energies. By diffusing away from the shock, the particles create a nebula of electrons/positrons which emits via synchrotron radiation from radio to soft gamma-ray bands.
Composite SNRs are remnants which exhibit a combination of the previous two morphologies, having both a well-defined shell, and pulsar wind nebulae emission. These are mostly young (~20, 000 yr), energetic SNRs containing a pulsar wind nebula that has yet to blow away its shell. Both the shell and the inner pulsar wind nebula are traced in the radio and X-ray bands. Mixed morphology SNRs also known as thermal-composite SNRs — are remnants which display a shell in the radio band, but also generate thermal X-ray radiation from hot plasma rather than a pulsar at the center of the remnant.
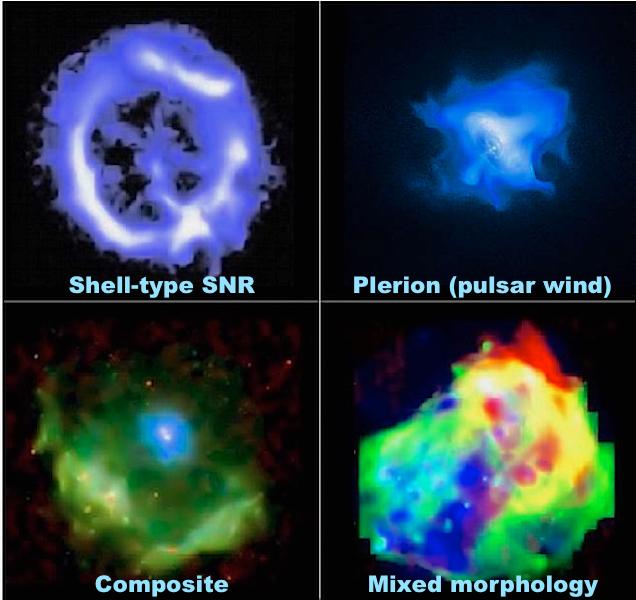
Examples of SNR morphological types. From top left to bottom right:
• (a) (SNR) E0102 - 72, a shell-type supernova remnant in the Small Magellanic Cloud. (Image Credit: NASA/CXC/SAO).
• (b) The Crab Supernova Remnant, the most famous pulsar wind nebula. Notice the centralised emission, shocks fronts, and lack of a shell. (Image Credit: NASA/CXC/Penn State/G.Garmire et al).
• (c) (SNR) Kes 75, notice hard X-ray emission from both a shell, and a pulsar near the centre of the remnant. (Image Credit: NASA/CXC/ GSFC/F. P. Gavriil et al).
• (d) Thermal composite (SNR) 3C 391. This image is a composite of X-ray emission (blue), radio emission (red), and IR emission (green).
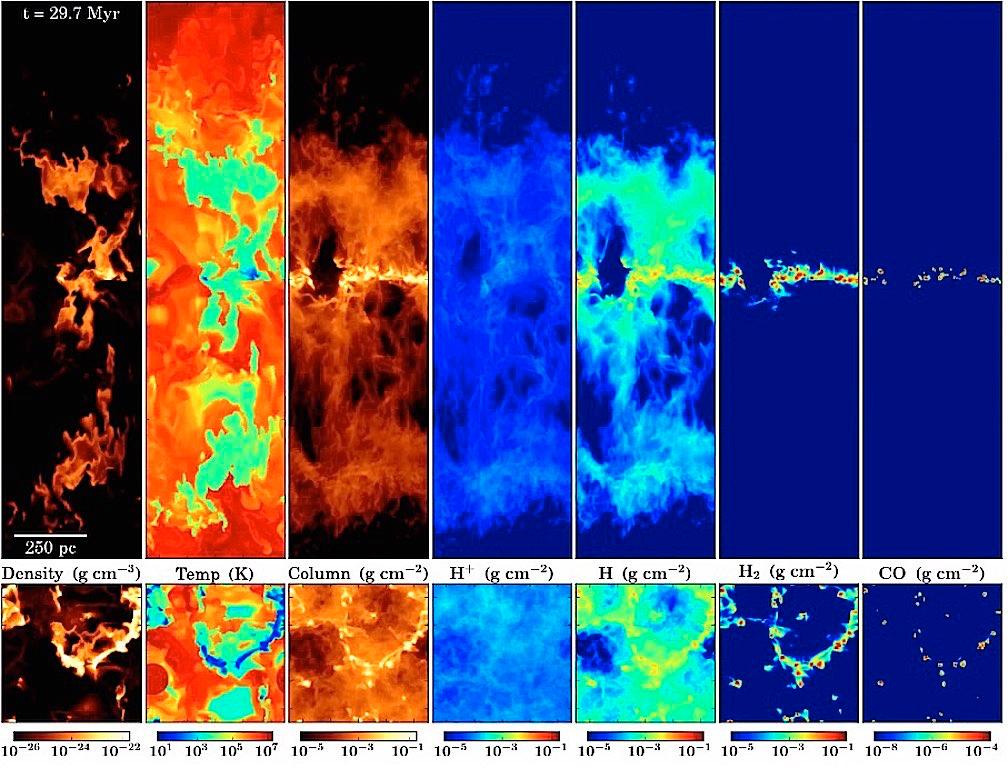
This is a snapshot of the state of a galaxy disc 29.7 million years after the beginning of a simulation calculated using 15 supernovae per million years. By this point 447 supernovae have exploded. The upper band shows gas distribution and temperature from the side; the lower squares show it as if seen from above. Running the full sim is a breathtaking lesson that our galaxy disc is a very violent place, about which we are blissfully unaware because of the enormous time scales involved.
An individual supernova makes many changes in the way its neighbourhood evolves over the first 10,000 to 20,000 years of the explosion. The metallicity of interstellar gas is enhanced by the elements hurled into space by the blast. The disc’s magnetic field has a hole blown into it by the super-hot gas ejecta, which takes centuries to restore. New, small star clusters sometimes form on the rim of an expending shell if it passes through a molecular cloud already dense and cold enough to undergo free-fall collapse. Particularly powerful blasts eject gas out of the thin disc into the thick disc and halo. The overall gas distribution in a galaxy is profoundly influenced by supernovae, especially in the very long term. When one steps back and looks at the life of a galaxy disc over 10 million years, anywhere between 50 and 100 supernovae may go off. The disruptive effects are visually spectacular. They also alter the composition and evolution of the disc. The SILCC Project in Garching, Germany has a fascinating array of simulations that show what happens to specific properties, e.g., gas temperature, metallicity, and gas availability for future star formation over cycles of a million years.
The really big picture
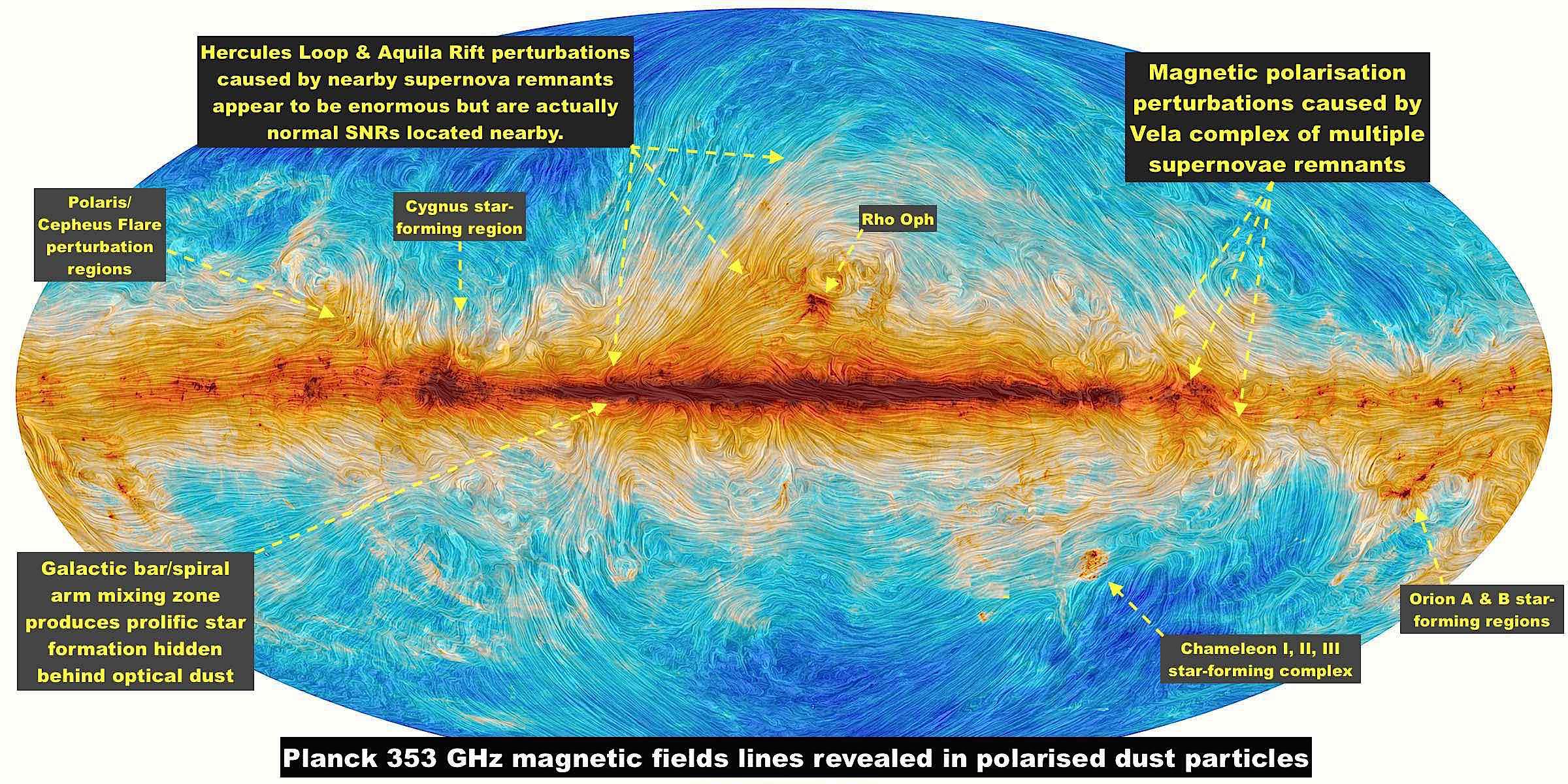
Readings
See supernova blast remnants with your own eyes, F. Michael Witkoski, Astronomy Magazine, June 16, 2023
The Vela Supernova Remnant – First Noticed Over a Hundred Years Ago, Scott Harrington, Cloudy Nights forum, first post March 1 2023. This article has abundant historical and observational detail for visual observers.
Ghostly Stellar Tendrils of the Vela Supernova Remnant, 2024 Image Processed by T.A. Rector, M. Zamani & D. de Martin, NSF NOIRLab
The Ghost Of A Giant Star – Vela Supernova Remnant, by Keith Cowing, European Space Observatory, November 13, 2022
Further research
arXiv:2306.10975: A detailed look at the thermal and non-thermal X-ray emission from the Vela supernova remnant with SRG. Abstract: Our goal is the characterization of the hot ejecta and shocked interstellar medium (ISM) associated to the Vela supernova remnant. A&A 676, A68 (2023).
arXiv:2306.01484 & arXiv:2303.12686: SRG/eROSITA and XMM-Newton observations of Vela Jr.
Abstract: The Vela supernova remnant complex is a region containing at least three supernova remnants: Vela, Puppis A, and Vela Jr. With the launch of the spectro-imaging X-ray telescope eROSITA, it became possible to observe the one degree wide Vela Jr in its entirety. A&A 673, A45 (2023).
arXiv:2110.11369: The Progenitor of the Vela Pulsar. Abstract: With Gaia parallaxes it is possible to study the stellar populations associated with individual Galactic supernova remnants (SNR) to estimate the mass of the exploding star. Submitted to MNRAS October 2021.
arXiv:2103.09637: X-ray emitting structures in the Vela SNR: ejecta anisotropies and progenitor stellar wind residuals. Abstract: The Vela supernova remnant (SNR) shows several ejecta fragments protruding beyond the forward shock (shrapnel). Recent studies have revealed high Si abundance in two shrapnel (A and G), located in opposite directions with respect to the SNR center. A&A 649, A56, 2021.
arXiv:2102.13449: A supernova remnant discovered in the SRG/eROSITA All-Sky Survey eRASS. Abstract: Supernova remnants (SNRs) are observable for about 6 – 15 x 104 years before they fade into the Galactic interstellar medium. With a Galactic supernova rate of approximately two per century, we can expect to have of the order of 1200 SNRs in our Galaxy. However, only about 300 of them are known to date … Here we report findings from the search for new SNRs in the eROSITA all-sky survey data which led to the detection of one of the largest SNRs discovered at wavelengths other than the radio: G249.5+24.5. A&A 648, A30 (2021).
arXiv:2009.06649: Simulating TeV gamma-ray morphologies of shell-type supernova remnants.
Abstract: Supernova remnant (SNR) shocks provide favourable sites of cosmic ray (CR) proton acceleration if the local magnetic field direction is quasi-parallel to the shock normal. Using the moving-mesh magneto-hydrodynamical (MHD) code AREPO we present a suite of… . Accepted for publication on MNRAS.
arXiv:2005.12869: Global X-Ray Properties of the Vela and Puppis A Supernova Remnants. Abstract: The Vela and Puppis A supernova remnants (SNRs) comprise a large emission region of ∼8’ diameter in the soft X-ray sky. The HaloSat CubeSat mission provides the first 0.4 7 keV soft X-ray observation of the entire Vela SNR and Puppis A SNR region with a single pointing and moderate spectral resolution. Accepted for publication in A-J, 2020.
arXiv:2005.06544: Search for Continuous Gravitational Waves from the Central Compact Objects in Supernova Remnants Cassiopeia A, Vela Jr. and G347.3-0.5. Abstract: We perform a sub-threshold follow-up search for continuous nearly-monochromatic gravitational waves from the central compact objects associated with the supernova remnants Vela Jr., Cassiopeia A, and SNR G347.3 0.5. Accepted for publication in A-J 2020.
arXiv:1907.02037: On the Gamma-Ray Nebula of Vela Pulsar. I. Very Slow Diffusion of Energetic Electrons within the TeV Nebula. Abstract: In this paper, the TeV-gamma-ray-emitting electrons in the Vela X PWN are approximated to be injected impulsively when the cocoon is formed due to the interaction between the SNR reverse shock and the PWN. ApJ 877 54 2019.
arXiv:1904.12035: The nature of the infrared counterpart and of the optical nebula associated with the Central Compact Object in Vela Jr. Abstract: The few kyr-old Vela Jr. supernova remnant (G266.2-1.2) belongs to the peculiar class of isolated neutron stars dubbed "Central Compact Objects" (CCOs). Accepted for publication in MNRAS.
arXiv:1811.05898: Discovery of a possible shock-cloud interaction on the Western edge of the Vela SNR. Abstract: A detailed analysis of the available multi-wavelength data shows that this source is located on the Western edge of the Vela supernova remnant. A detailed analysis of the available multi-wavelength data shows that this source is located on the Western edge of the Vela supernova remnant (SNR): the observations and the spectral energy distribution support a scenario where this gamma-ray source is the byproduct of the interaction between the SNR shock and a neutral Hydrogen cloud. ApJ Jan. 3, 2019.
arXiv:1809.02249: A Morphological Study of the Supernova Remnant RX J0852.0-4622 (Vela Jr.). Abstract: We conduct a multi-wavelength morphological study of the Galactic supernova remnant Vela Jr. We find that the RX J0852.0-4622 radio continuum emission can be characterised by a 2-dimensional shell with a radius of 11.8+/-0.6pc at an assumed distance of 750pc. Accepted for Publication ApJ.
arXiv:1808.03878: Investigating the Structure of Vela X. Abstract: Vela X is the prototypical example of a pulsar wind nebula whose morphology and detailed structure have been affected by the interaction with the reverse shock of its host supernova remnant. The resulting complex of filamentary structure and mixed-in ejecta embedded in a nebula that is offset from the pulsar provides the best example we have of this middle-age state that characterizes a significant fraction of composite SNRs. Accepted for publication in ApJ.
arXiv:1807.10551: Modeling the spatially resolved non-thermal emission from the Vela Jr. supernova remnant. Abstract: Vela Jr. is one of just a few known supernova remnants (SNRs) with a resolved shell across the whole electromagnetic spectrum from radio to very-high-energy (>100 GeV; VHE) gamma-rays. Its proximity and large size allow for detailed spatially resolved observations of the source makingVela Jr. one of the primary sources used for the study of particle acceleration and emission mechanisms in SNRs. A&A 618, A155 (2018)
arXiv:1807.04182: Inefficient cosmic ray diffusion around Vela X: constraints from H.E.S.S. observations of very high-energy electrons. Abstract: Vela X is a nearby pulsar wind nebula (PWN) powered by a ∼104 year old pulsar. Modeling of the spectral energy distribution of the Vela X PWN has shown that accelerated electrons have largely escaped from the confinement, which is likely due to the disruption of the initially confined PWN by the SNR reverse shock. The escaped electrons propagate to the earth and contribute to the measured local cosmic-ray (CR) electron spectrum. Accepted by ApJ 2018.
arXiv:1707.05215: Indications of a Si-rich bilateral jet of ejecta in the Vela SNR observed with XMM-Newton. Abstract: The Vela supernova remnant displays several ejecta which are fragment-like features protruding beyond the front of its primary blast shock wave. They appear to be "shrapnel", bowshock-shaped relics of the supernova explosion. One of these pieces of shrapnel (A), located in the northeastern edge of the remnant, is peculiar because its X-ray spectrum exhibits a high Si abundance, in contrast to the other observed ejecta fragments, which show enhanced O, Ne, and Mg abundances. We present the analysis of another fragment located opposite to shrapnel A with respect to the center of the shell, in the southwestern boundary of the remnant, named shrapnel G. A&A 604, L5 (2017)
arXiv:1701.04349: Unveiling Time Variability of Interstellar Lines in the Direction of the Vela Supernova Remnant II, Na D and Ca II. Abstract: In a survey conducted between 2011-12 of interstellar Na I D line profiles in the direction of the Vela supernova remnant, a few lines of sight showed dramatic changes in low velocity absorption components with respect to profiles from 1993-1994. Three stars - HD 63578, HD 68217 and HD 76161 showed large decrease in strength. Accepted for publication in MNRAS
arXiv:1612.00261: Discovery of new TeV supernova remnant shells in the Galactic plane with H.E.S.S. Abstract: Supernova remnants (SNRs) are prime candidates for efficient particle acceleration up to the knee in the cosmic ray particle spectrum. In this work we present a new method for a systematic search for new TeV-emitting SNR shells in 2864 hours of H.E.S.S. phase I data. Announced December 2016.
arXiv:1611.01863: Deeper H.E.S.S. observations of Vela Junior (RX J0852.0 4622): Morphology studies and resolved spectroscopy. Abstract: Aims. We study gamma-ray emission from the shell-type supernova remnant (SNR) RX J0852.0 4622 to better characterize its spectral properties and its distribution over the SNR. Methods. The analysis of an extended High Energy Spectroscopic System (H.E.S.S.) data set at very high energies (E > 100 GeV) permits detailed studies, as well as spatially resolved spectroscopy. Announced November 2016.
arXiv:1510.06494: Unveiling Vela Time Variability of Na I D lines in the Direction of the Vela Supernova Remnant. Abstract: High-resolution spectral profiles of Na I D lines from the interstellar medium towards 64 stars in the direction of the Vela supernova remnant are presented. This survey conducted mostly between 2011-12 complements an earlier survey of the same stars by Cha & Sembach done in 1993-96. Accepted in MNRAS.