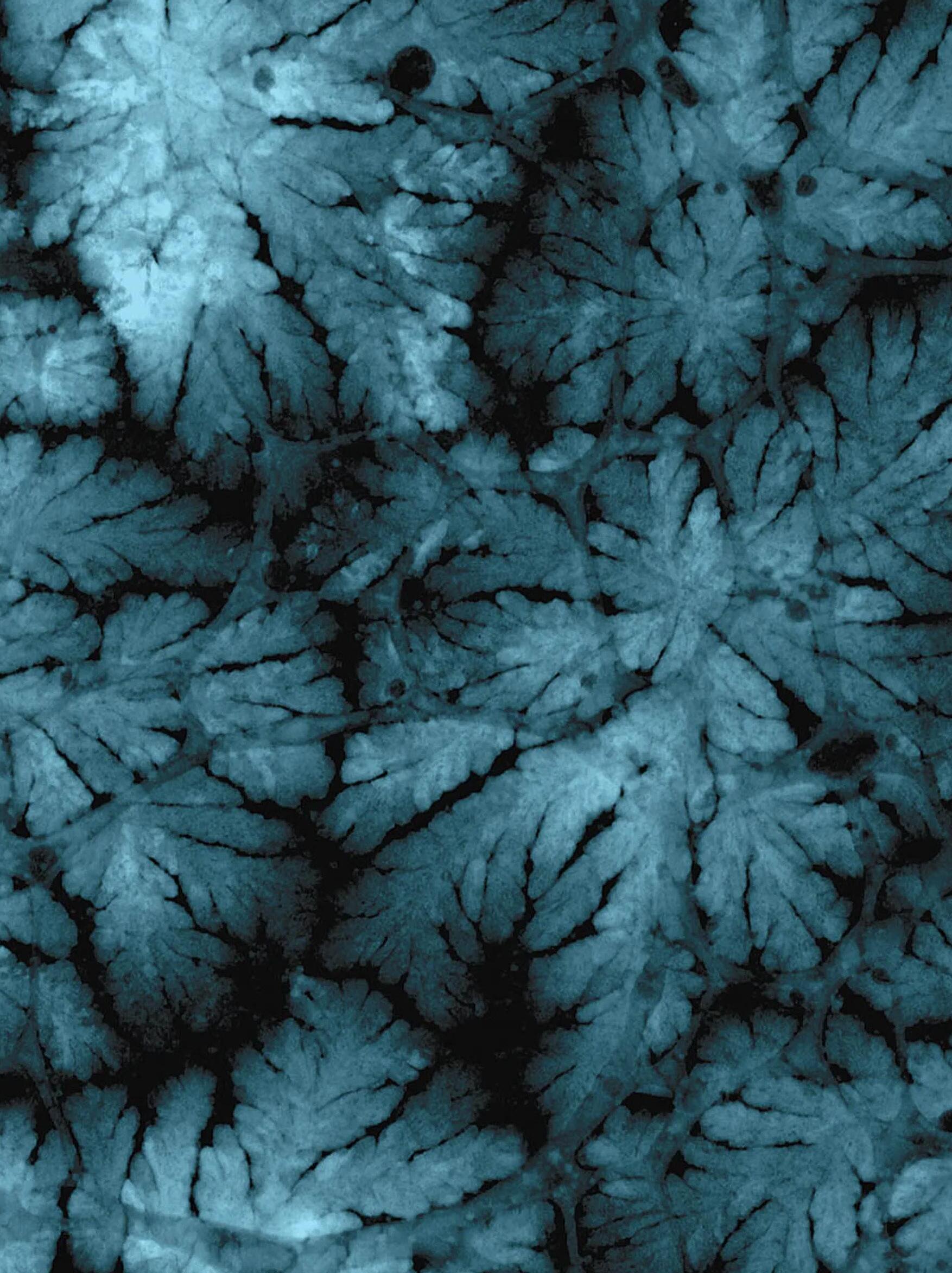
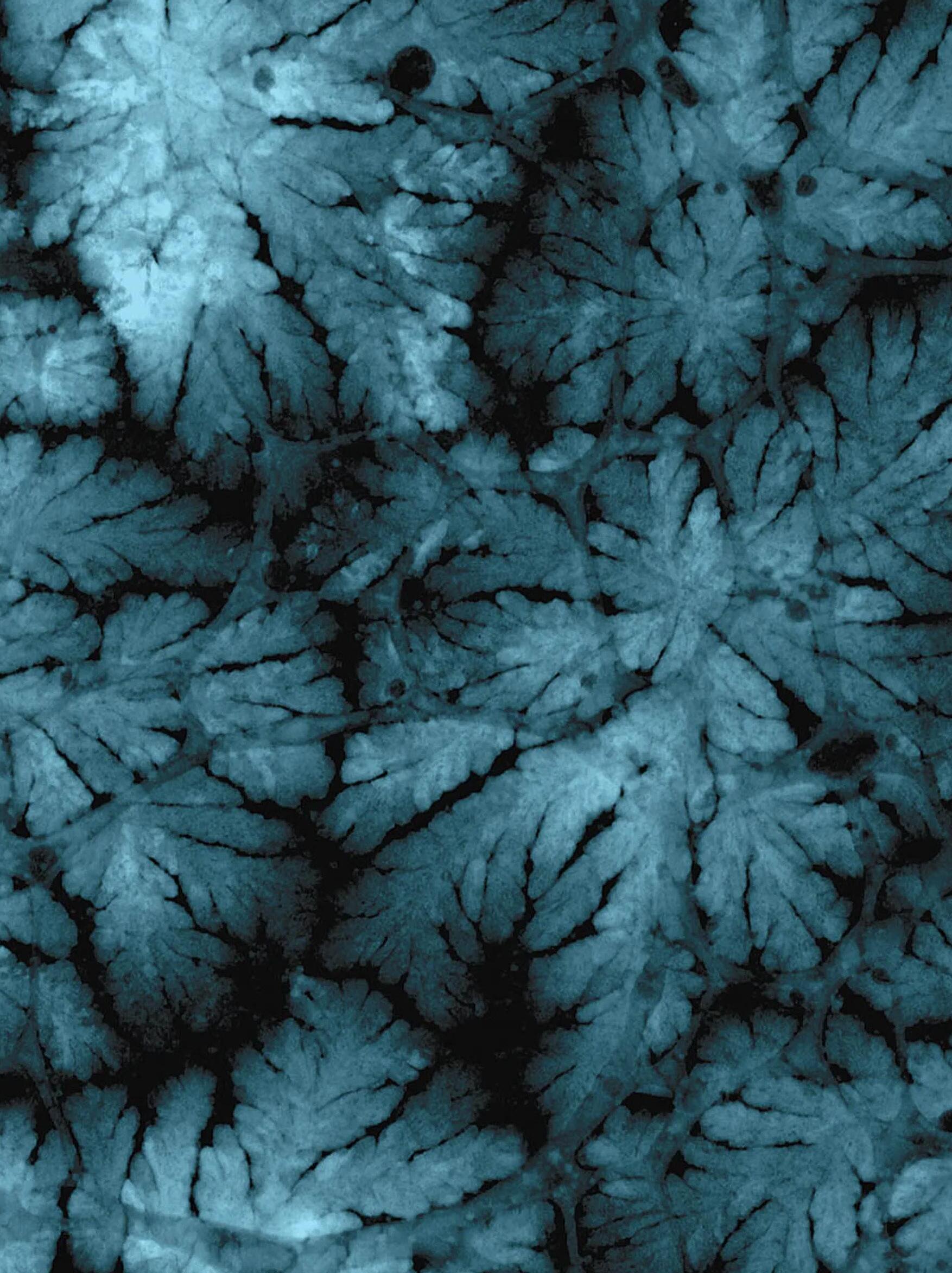
R Y SAFETY
ECS, a prestigious nonprofit professional society, has led the world in electrochemistry, solid state science and technology and allied subjects since 1902, providing a rigorous and high-quality home for the whole community.
ECS is dedicated to moving science forward by empowering researchers globally to leave their mark on science. The Society connects a diverse and representative constituency of members and nonmembers to accelerate scientific discovery, facilitate the engagement of an inclusive network, and champion the dissemination of research to support a sustainable future.
For more information on becoming a member, or publishing in ECS publications, visit electrochem.org
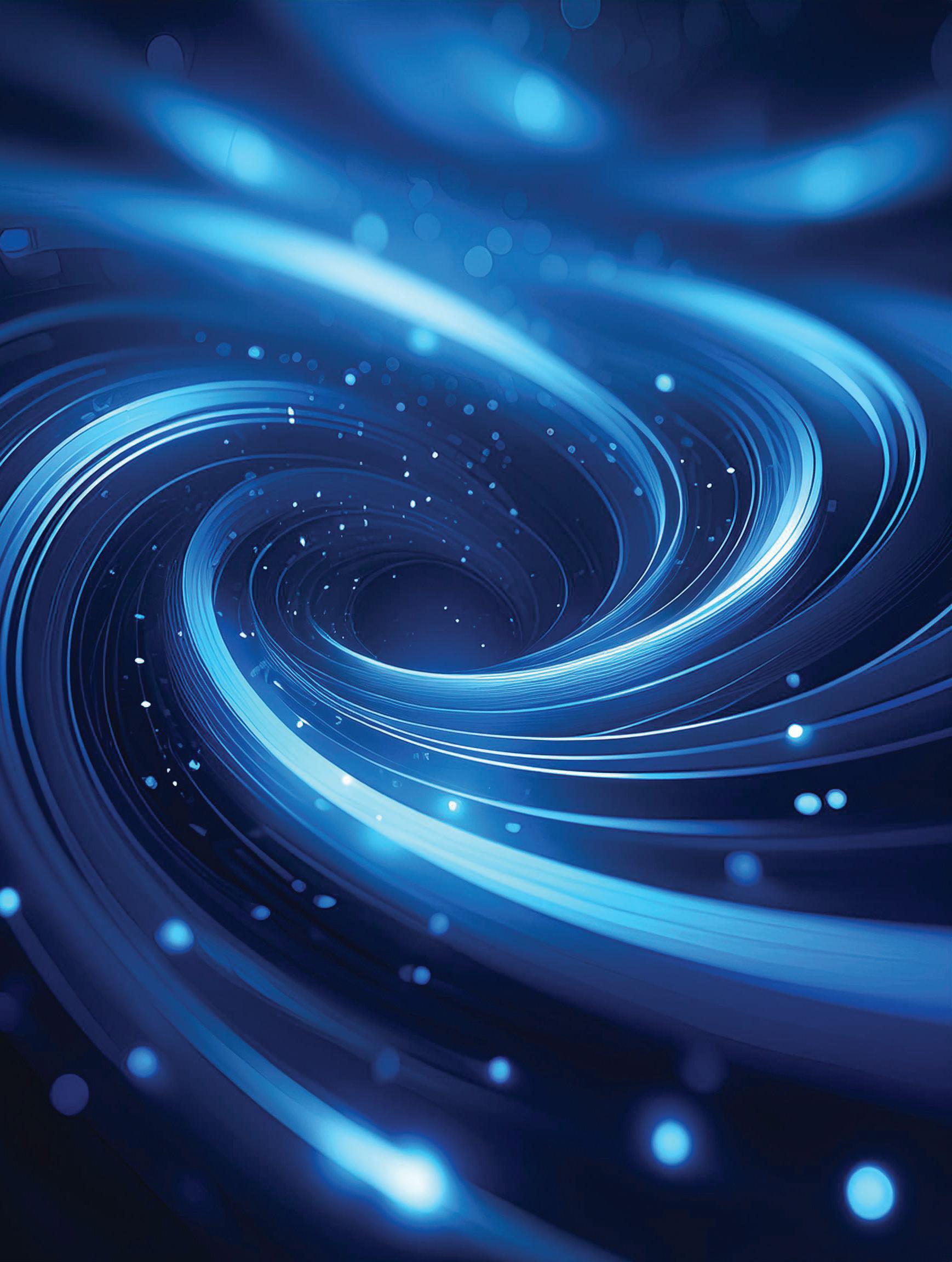
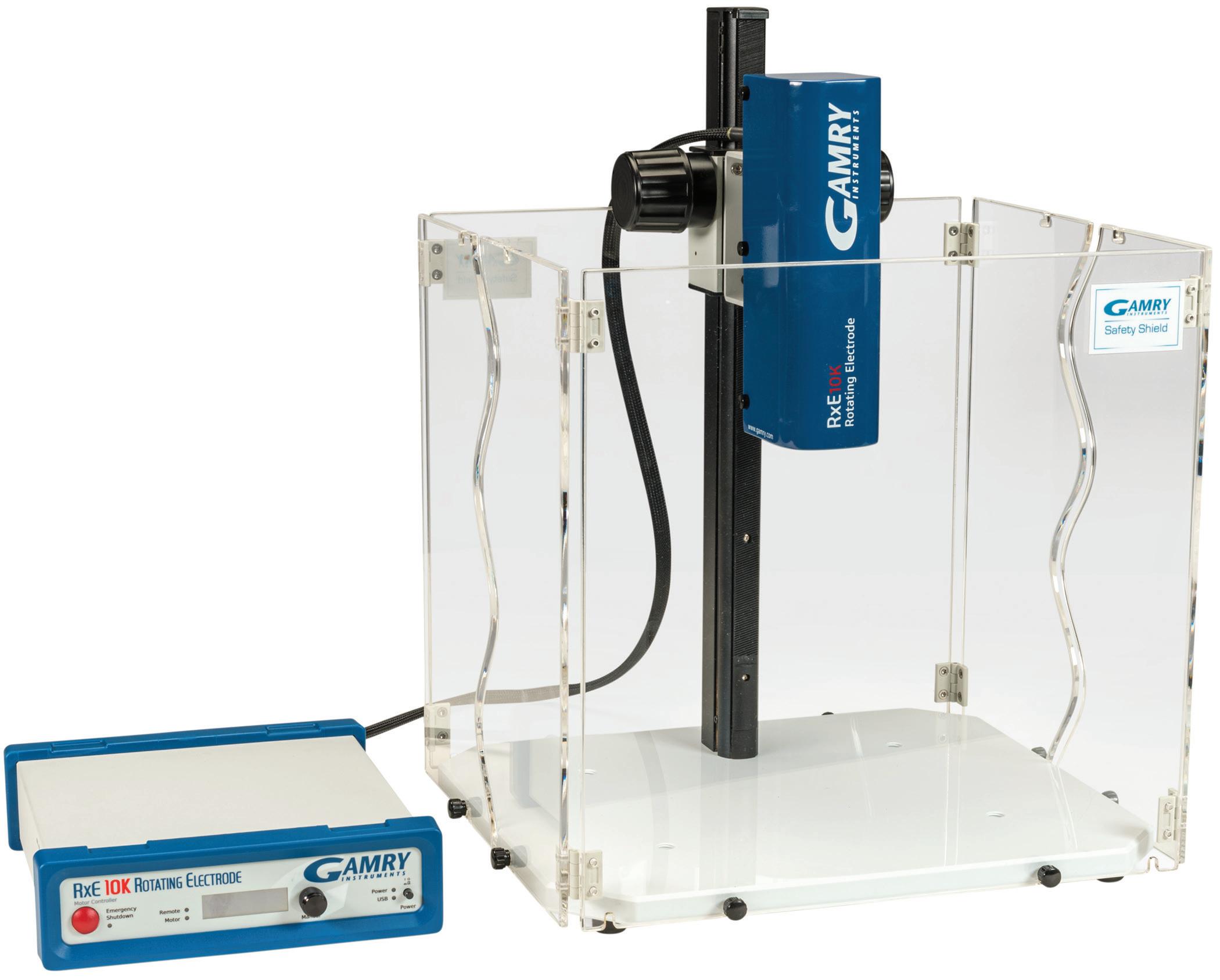
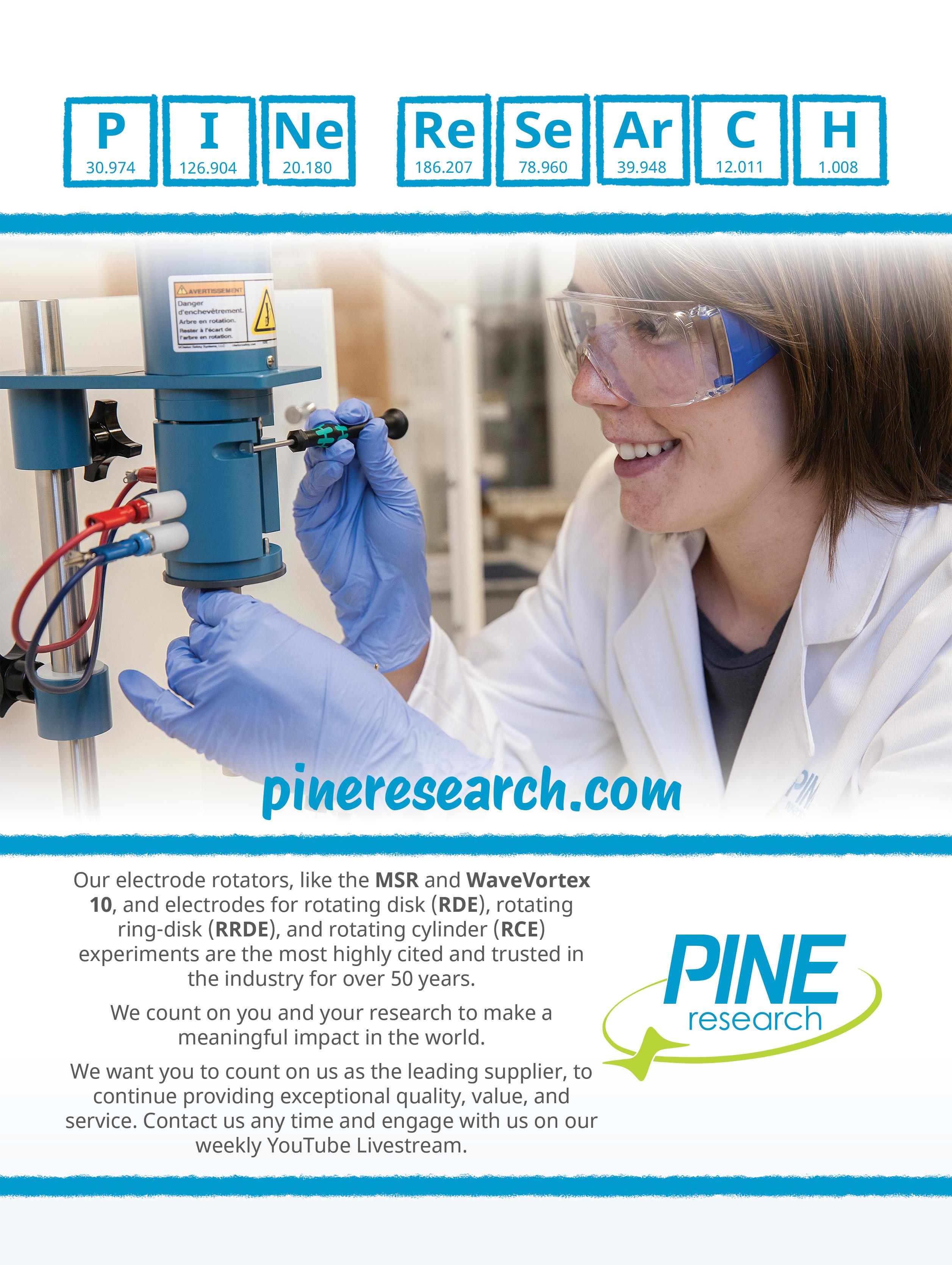
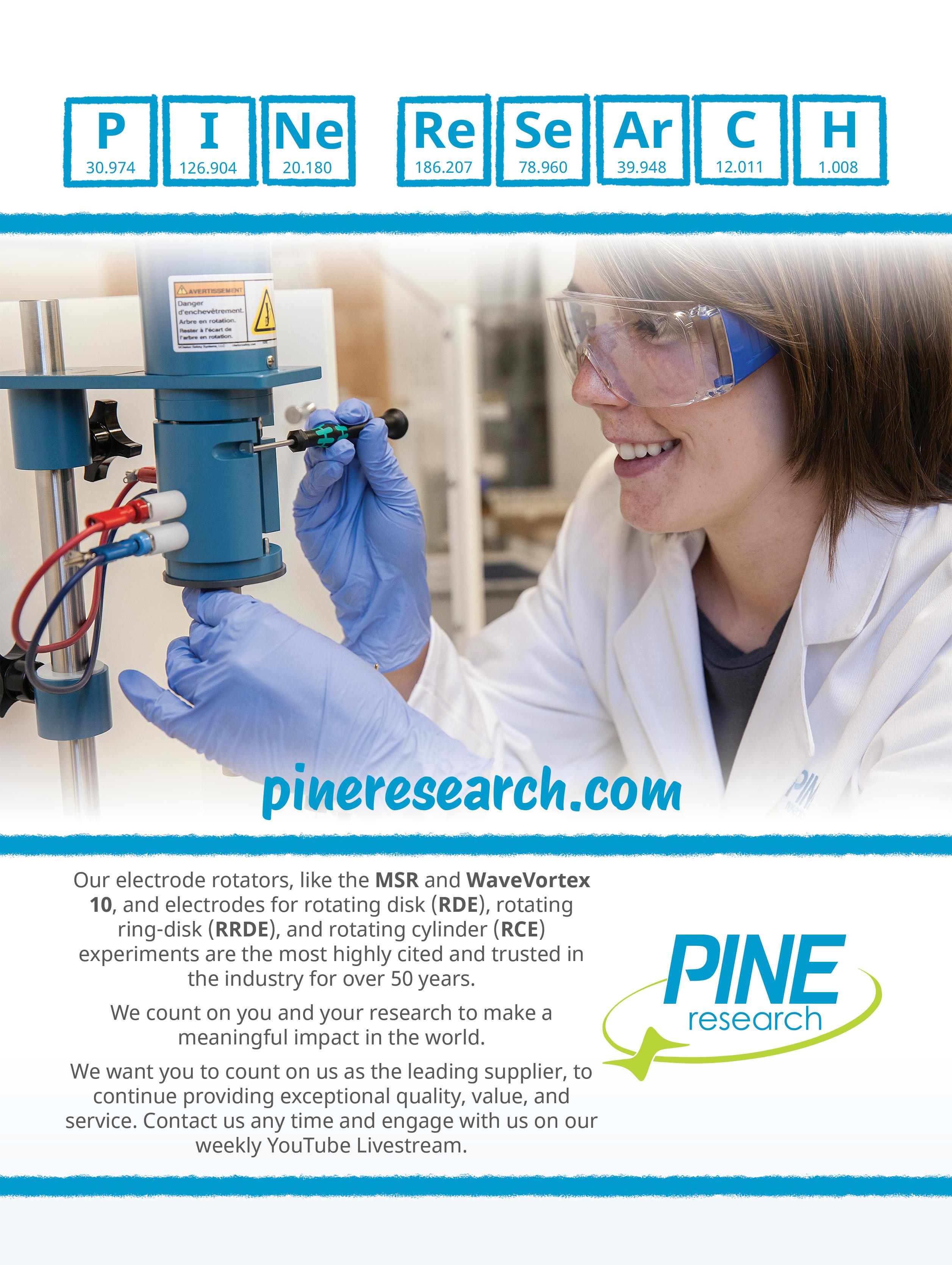
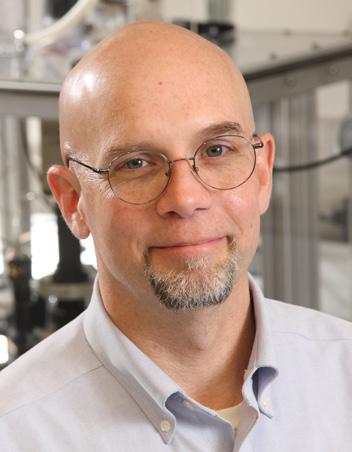
Godspeed, Glenn Stoner
As you read this in October or later, I will have finally stopped writing “2023” and will have fully embraced the new year. What I haven’t done is gotten over the loss of Glenn Stoner last summer. I still cannot think of Glenn without tearing up. Although John Scully collaborated with me to write an obituary for Glenn in the fall 2023 issue of Interface, I realized there is so much more to say. He was so much more than a colleague and mentor, although he was great at being both. He was an exemplar of how we should treat one another, even those with whom we disagree at times. He was always kind, and he would try to see things from the other perspective. At times, he could even be convinced that he had been wrong, but he never took a discussion as an assault on, or an assessment of, his character or worth. He was more comfortable in his skin than anyone else I have ever met. Glenn also established a workplace culture in which sharing was the norm. For those of you not in academia, the words “faculty” and “sharing” are rarely, if ever, used together in a positive sentence. But Glenn imbued into everyone that mi casa, su casa was the way we do things here. To this day, we share space, equipment, graduate advising, grant management, and program reviews to mention just a few. He managed with a gentle hand, but one that was universally respected.
Glenn showed that one does not have to be a dictator to be an effective graduate advisor. His influence as a mentor has stretched across generations, as his students followed his lead, consciously or unconsciously. You can hear Glenn when you listen to his students, his students’ students, or even his students’ students’ students, interact with their employees, collaborators, or students. The trust he had in others’ abilities and motivations created a drive in them to not disappoint him. His students and colleagues would run through walls for him because he would, and did, run through walls for them. The pride he had in his students from the day they started throughout their career was absolute and unwavering. You simply always felt better about yourself when you left a conversation with Glenn. Your success was more important to him than his success.
John Scully and I hosted a 50th anniversary celebration of Glenn’s establishment of the Applied Electrochemistry Laboratory at the University of Virginia on June 1, 2024. It was a great event, with over 130 alumni and friends gathering in Charlottesville to look back in amazement of how fast time flies. George Cahen was there, which was important as his was the first of the over 250 graduate degrees earned by students working in what is now the Center for Electrochemical Science and Engineering. Many others wanted to attend, but the commitments and vagaries of life intervened, sometimes at the last minute. We did have a few people join in via Zoom for the memorial service for Glenn in the afternoon, and we recorded the after-dinner speeches that evening, as well as a slideshow that looped throughout the evening. The images on the slides were interesting, in part because of the change in the quality of photos from 1973 compared to 2023, but more so because seeing ourselves and others as we were 10 to 50 years ago is a hoot. EJ Taylor, the senior member of our “Looking at Patent Law” series tag team, has barely changed since his 1980s graduate school days except for some distinguished graying at the temples. Maria Inman, the junior member of the tag team, was also there. She represents the resilience of the human spirit as she has been immensely successful after somehow overcoming both the trauma of being my first postdoctoral associate and working with EJ in her role as the chief technical person at Faraday Technologies. (Rumor has it that she had much bad karma to work off from a previous life, and her other choice was to come back as a dung beetle. She flipped a coin and lost.)
Although it can be hot and very humid in Charlottesville in early June, we lucked out and the weather was perfect. It allowed many to pay their respects at Glenn’s final resting place, which is within shouting distance of our building. I am certain that he is keeping an eye on us. We will do everything in our power not to disappoint him.
Until next time, be safe and happy.
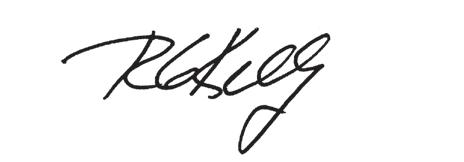
Rob Kelly Editor
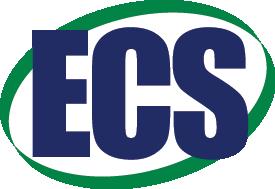
Published by:
The Electrochemical Society (ECS) 65 South Main Street Pennington, NJ 08534-2839, USA
Tel 609.737.1902, Fax 609.737.2743 www.electrochem.org
Editor: Rob Kelly
Guest Editors: Paul R. Shearing and Thomas P. Barrera
Contributing Editors: Christopher L. Alexander, Christopher G. Arges, Scott Cushing, Ahmet Kusolgu, Donald Pile, Alice Suroviec
Senior Director of Publications: Adrian Plummer
Senior Director of Engagement: Shannon Reed
Production Editor: Kara McArthur
Graphic Design & Print Production Manager: Dinia Agrawala
Staff Contributors: Frances Chaves, Genevieve Goldy, Mary Hojlo, Christopher J. Jannuzzi, John Lewis, Anna Olsen, Fern Oram, Jennifer Ortiz, Francesca Di Palo, JaneAnn Wormann
Advisory Board: Brett Lucht (Battery Division)
Dev Chidambaram (Corrosion Division)
Vaddiraju Sreeram (Dielectric Science and Technology Division)
Luca Magagnin (Electrodeposition Division)
Qiliang Li (Electronics and Photonics Division) Katherine Ayers (Energy Technology Division) Cortney Kreller (High-Temperature Energy, Materials, & Processes Division)
Paul Kenis (Industrial Electrochemistry and Electrochemical Engineering Division)
Eugeniusz Zych (Luminescence and Display Materials Division)
Jeff Blackburn (Nanocarbons Division)
Shelley Minteer (Organic and Biological Electrochemistry Division)
Stephen Paddison (Physical and Analytical Electrochemistry Division)
Larry Nagahara (Sensor Division)
Publications Subcommittee Chair: Francis D'Souza
Society Officers: Colm O'Dwyer, President; James (Jim) Fenton, Senior Vice President; Francis D'Souza, 2nd Vice President; Robert Savinell, 3rd Vice President; Gessie Brisard, Secretary; Elizabeth J. PodlahaMurphy, Treasurer; Christopher J. Jannuzzi, Executive Director & CEO
Statements and opinions given in The Electrochemical Society Interface are those of the contributors, and ECS assumes no responsibility for them.
Authorization to photocopy any article for internal or personal use beyond the fair use provisions of the Copyright Act of 1976 is granted by The Electrochemical Society to libraries and other users registered with the Copyright Clearance Center (CCC). Copying for other than internal or personal use without express permission of ECS is prohibited. The CCC Code for The Electrochemical Society Interface is 1064-8208/92.
ISSN : Print: 1064-8208 Online: 1944-8783
The Electrochemical Society Interface is published quarterly by The Electrochemical Society (ECS), at 65 South Main Street, Pennington, NJ 08534-2839 USA. Subscription to members is part of membership service. © Copyright 2024 by The Electrochemical Society. *“Save as otherwise expressly stated.”
The Electrochemical Society is an educational, nonprofit 501(c)(3) organization with more than 8,500 scientists and engineers in over 75 countries worldwide who hold individual membership. Founded in 1902, the Society has a long tradition in advancing the theory and practice of electrochemical and solid state science by dissemination of information through its publications and international meetings.


https://orcid.org/0000-0002-7354-0978
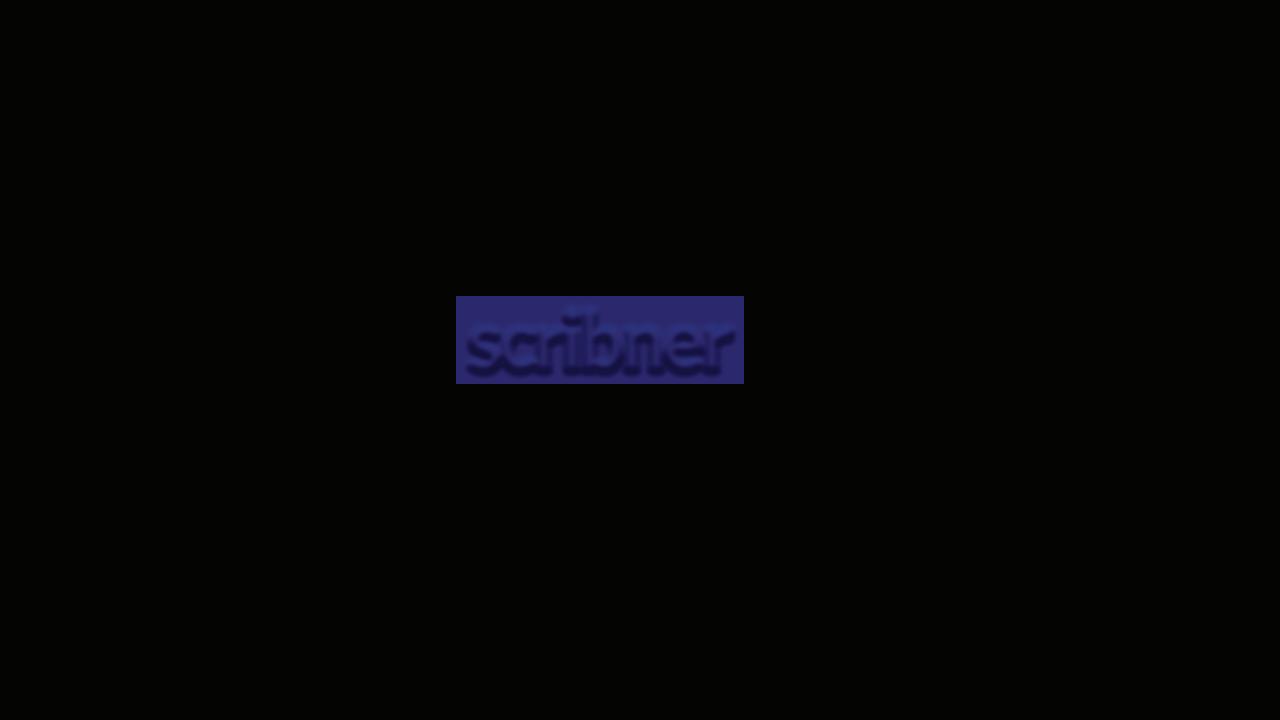
LET’S TALK INNOVATION
Enhanced Diagnostics
EIS Over Full Power Supply Range
Improved User Experience Factory KOH Ready
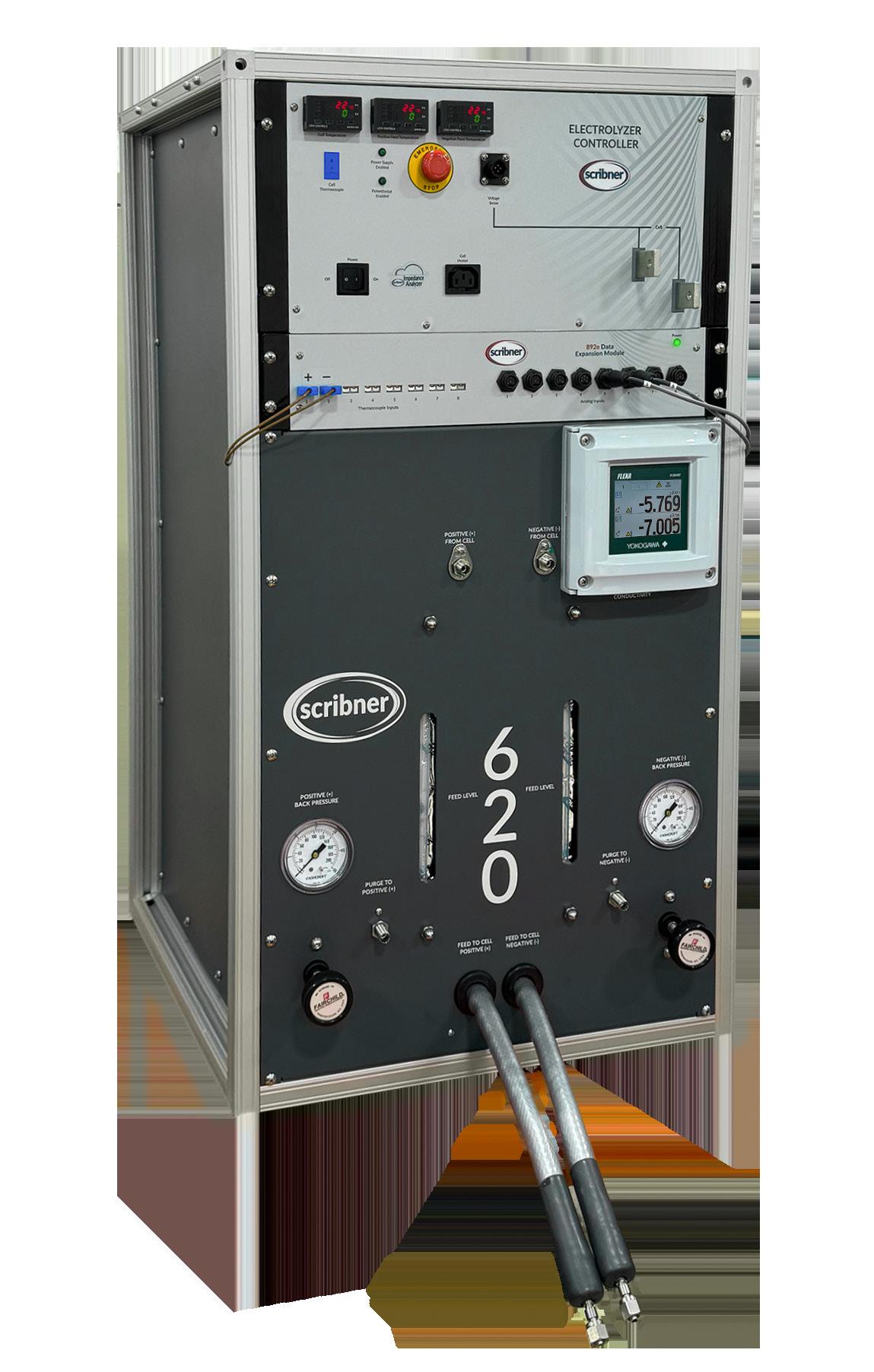
Unattended Operation
Advanced Liquid Feed Management
Intuitive Controls FlowCell Fully Integrated Software
Scribner’s 2nd Generation
Battery Safety
by Paul R. Shearing and Thomas P. Barrera
Insights into Thermal Runaway of Cylindrical Lithium-ion Batteries by Internal Temperature Sensors
by Max Feinauer, Markus Hölzle, and Thomas Waldmann
Achieving Passive Thermal Runaway Propagation Resistance in Li-ion Battery Packs
by Alexander Quinn, Jacob Darst, Matthew Keyser, Paul T. Coman, Thomas P. Barrera, Paul R. Shearing, David Petrushenko, Donal P. Finegan, Eric Darcy
Modeling of Li-ion Battery Thermal Runaway: Insights into Modeling and Prediction
by Paul T. Coman, Andrew Weng, Jason Ostanek, Eric Darcy, Donal P. Finegan, Ralph E. White
A Multi-scale Framework for Advancing Battery Safety Through Early Calorimetric Analysis of Materials and Components
by Alex Bates, Loraine Torres-Castro, Paul Albertus, Partha P. Mukherjee, Judith Jeevarajan, Nathan Johnson, Bhuvsmita Bhargava, Zixuan Wang, Taiwo Ogundipe, Bairav S. Vishnugopi, Avijit Karmakar, Wan Si Tang
High-Speed Imaging for Investigating Battery Failure Mechanisms
by Hamish T. Reid, Inez Kesuma, Mark Buckwell, James B. Robinson, Paul R. Shearing
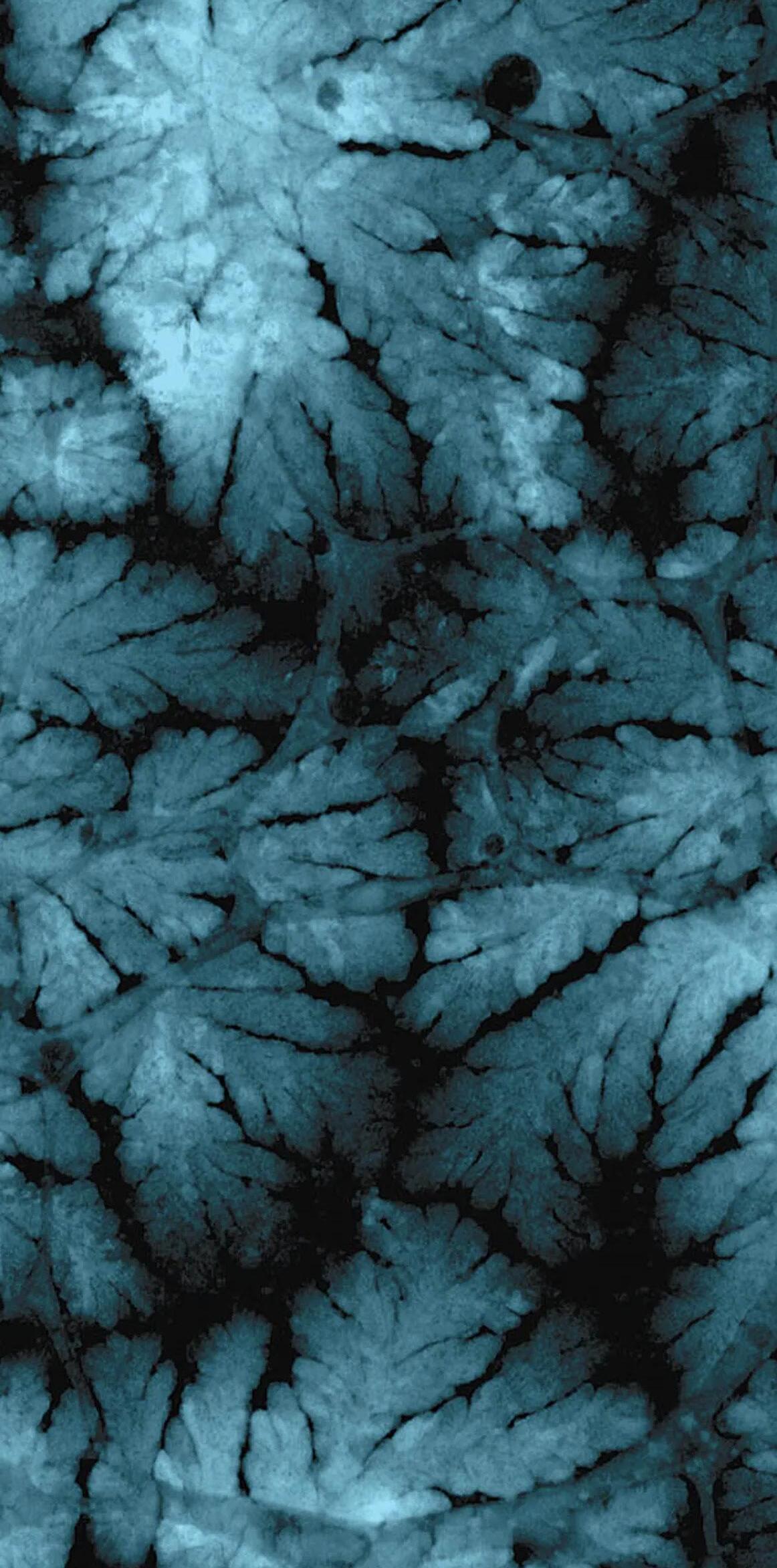
Vol. 33, No. 3 Fall 2024
3 From the Editor: Godspeed, Glenn Stoner
7 Pennington Corner: Membership by Association 12 PRiME 2024 Preview
Society News
Websites of Note
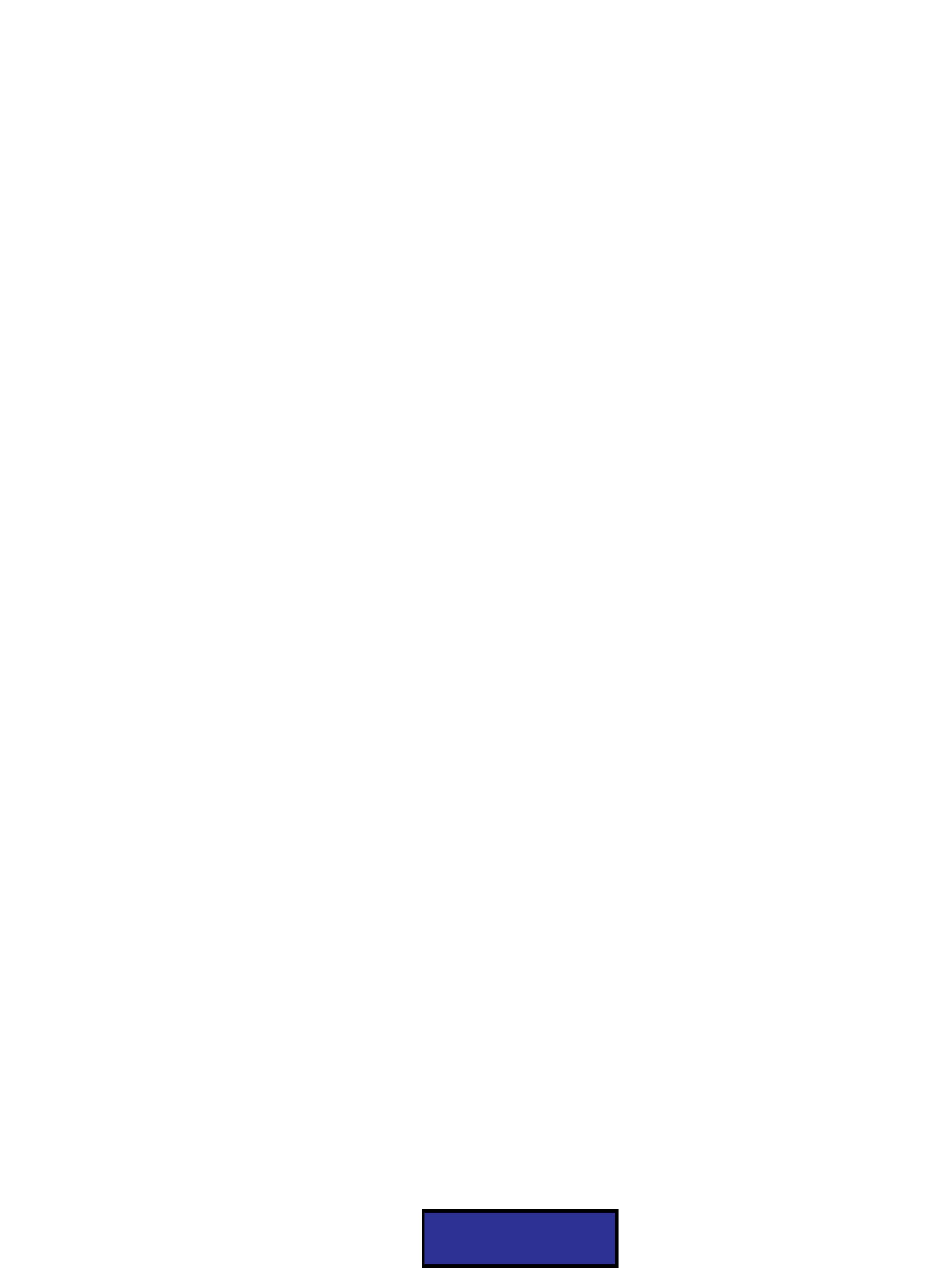
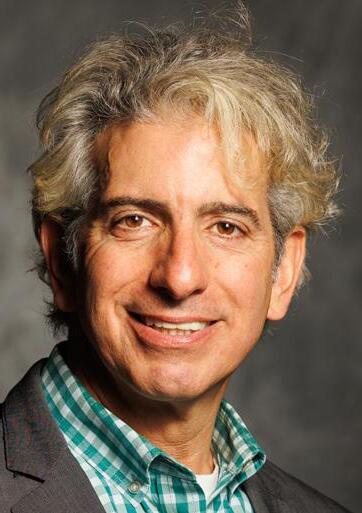
HMembership by Association
ow big is ECS? I am often asked that. Although it seems like a straightforward question, there are, in fact, many ways to answer it. One approach is simply to look at the number of Members. I am happy to report that since hitting a low of around 6,500 during the depths of the COVID-19 pandemic, our membership has not only rebounded well beyond pre-pandemic levels, but it is also at its highest level in over a decade with nearly 9,000 members!
As a member-led, member-driven organization, this growth is fantastic news. However, it is not the full story. The ECS community extends well beyond our Members to include a larger, very engaged group of individuals working around the world to serve the Society’s mission to advance theory and practice at the forefront of electrochemical and solid state science and technology. This group includes—but is not limited to—more than 15,000 authors whose work we publish yearly in our archival journals; 6,000 peer reviewers who are vital in ensuring the quality of the work appearing in those journals; and the 10,000+ anticipated attendees at our 2024 events and technical meetings—like the amazing event just held in San Francisco and the one we look forward to hosting soon in Hawaii!
ECS journal. Upon opting into the Associate category, benefits such as discounts on ECS events, products, and services (e.g., Open Access publishing fees), begin immediately. Although Associates do not receive the same discounts as Members and cannot vote or hold an elected position with the Society, there are still significant benefits to joining as an Associate.
While the board had many reasons for approving this new category of membership, the main drivers came from the operations side of the ECS house. I describe them as “accuracy” and “advocacy.”
Accuracy reflects the fact that we truly touch, engage with, and share in the advancement of the ECS mission with a far larger group than just our traditional, dues-paying Members. The Associate category enables us to more accurately account for the size of the community working to advance ECS’s mission.
“The ECS community extends well beyond our Members to include a larger, very engaged group of individuals working around the world to serve the Society’s mission to advance theory and practice at the forefront of electrochemical and solid state science and technology. ”
This broader look at the ECS community highlights the fact that those we serve—and represent—are a far larger group than is implied by only the number of Members. In recognizing the importance of viewing the ECS community through that lens, I am proud to announce that the ECS Board of Directors, working with the Individual Membership Committee chaired by E. J. Taylor, has approved the creation of a new member category designed to recognize, reward, and most importantly, encourage deeper engagement with the Society.
Beginning in 2025, ECS will offer an Associate membership category to qualifying constituents. However, unlike ECS Members who are required to pay annual dues, Associates pay no fees to join ECS. Instead, Associate membership is based solely on an individual’s engagement with the Society. Qualifications for Associate status might include presenting a paper at an ECS meeting, authoring or peer-reviewing a manuscript submission, or authoring a paper published in an
Advocacy describes another important aspect of the ECS mission. In June, I was honored to participate in an official visit to the US Congress on Capitol Hill in Washington, DC, with colleagues from the Materials Research Society (MRS). It was an incredible opportunity for ECS, and an amazing experience for me personally. I stood with ECS Past President Gerardine Botte, and shared the importance of the ECS mission with the leadership of the United States. We sought their support and partnership in advancing that mission for the benefit of all humanity. That is what all of us who engage with ECS—Members and future Associates—do; we leverage the electrochemical and solid state sciences to improve the human condition.
It is my sincere hope that you join us in this noble effort, however you choose to engage with your community at The Electrochemical Society.
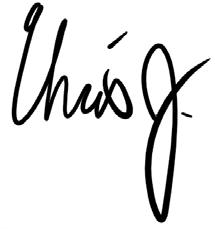
Christopher J. Jannuzzi ECS Executive Director/Chief Executive Officer https://orcid.org/0000-0002-7293-7404


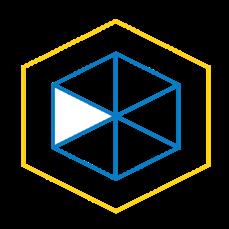
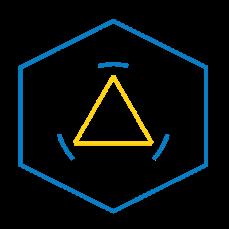
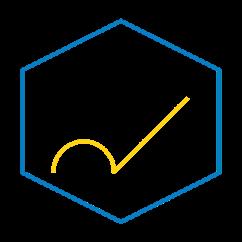
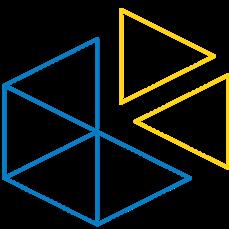
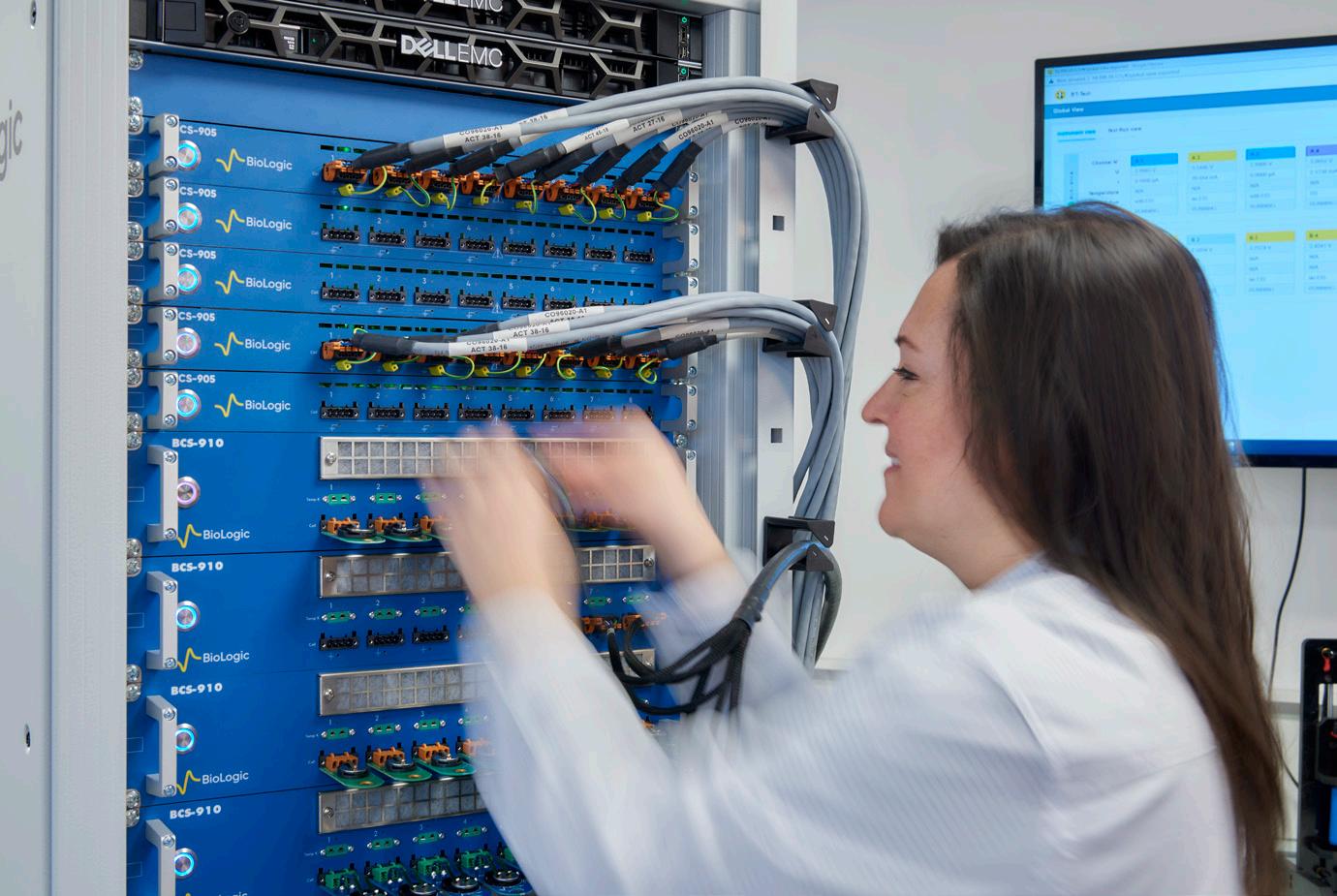
Run reliable & safe tests
Comprehensive from test to batch analysis
Control & measure with high precision from few µA to 120 A Adapt
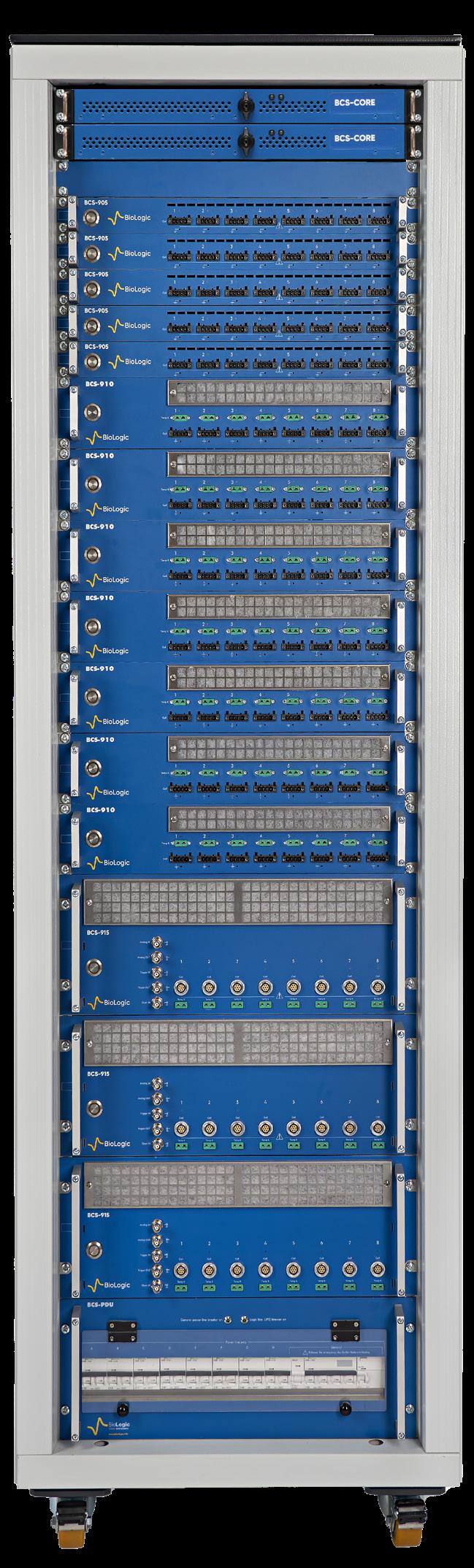
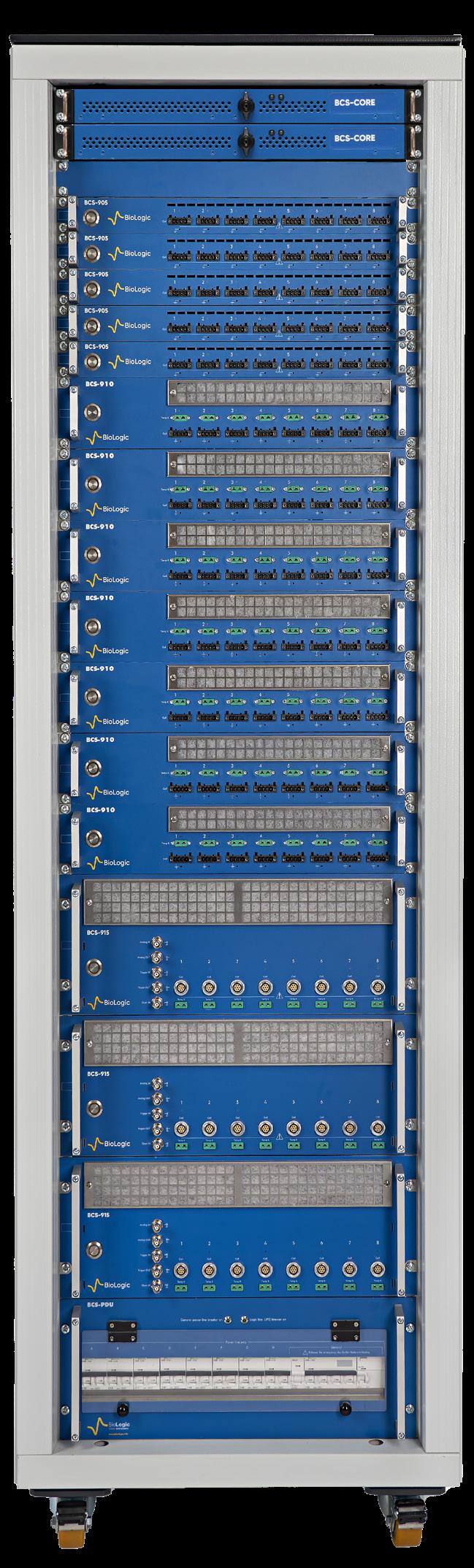
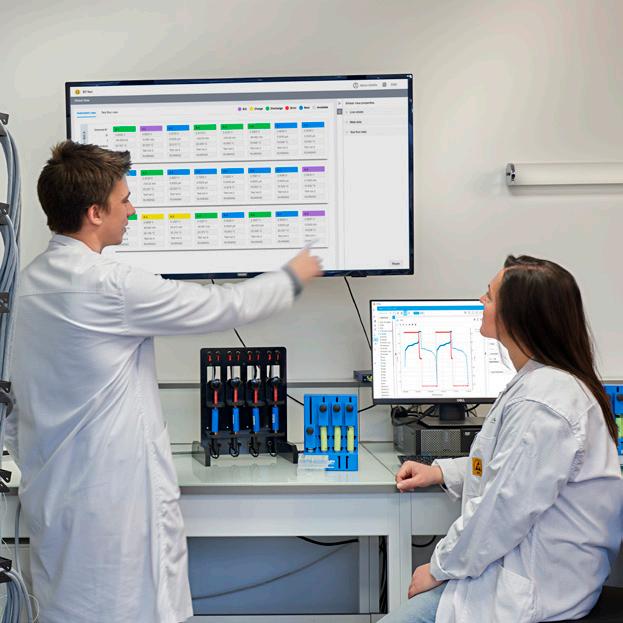
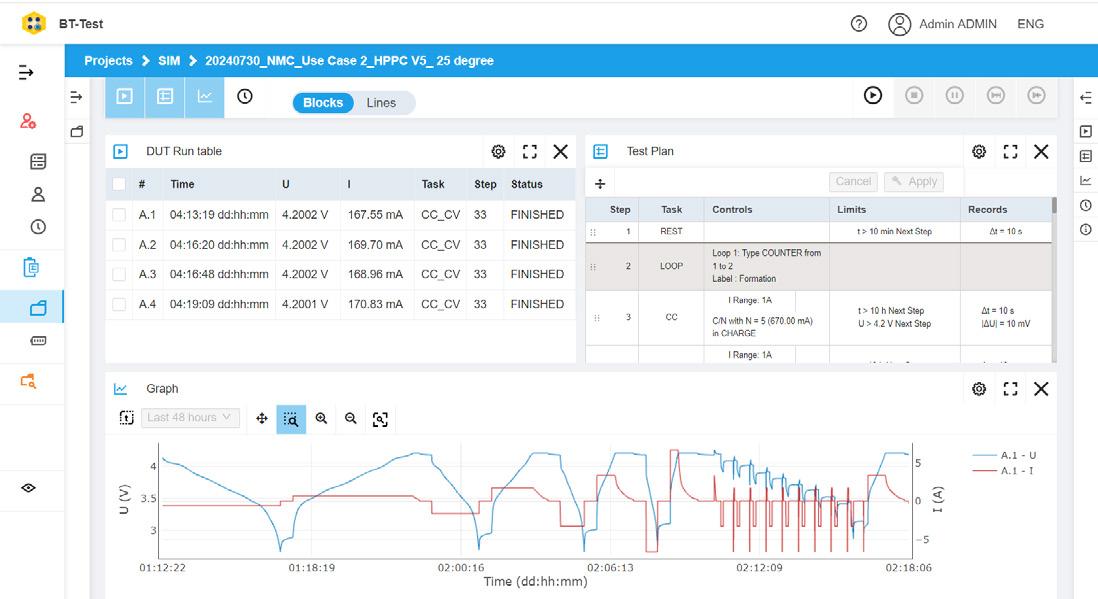
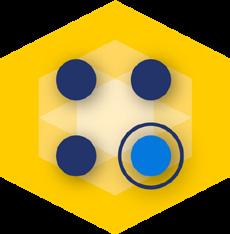
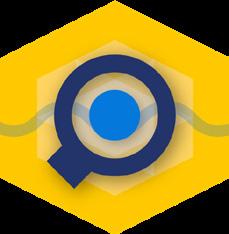
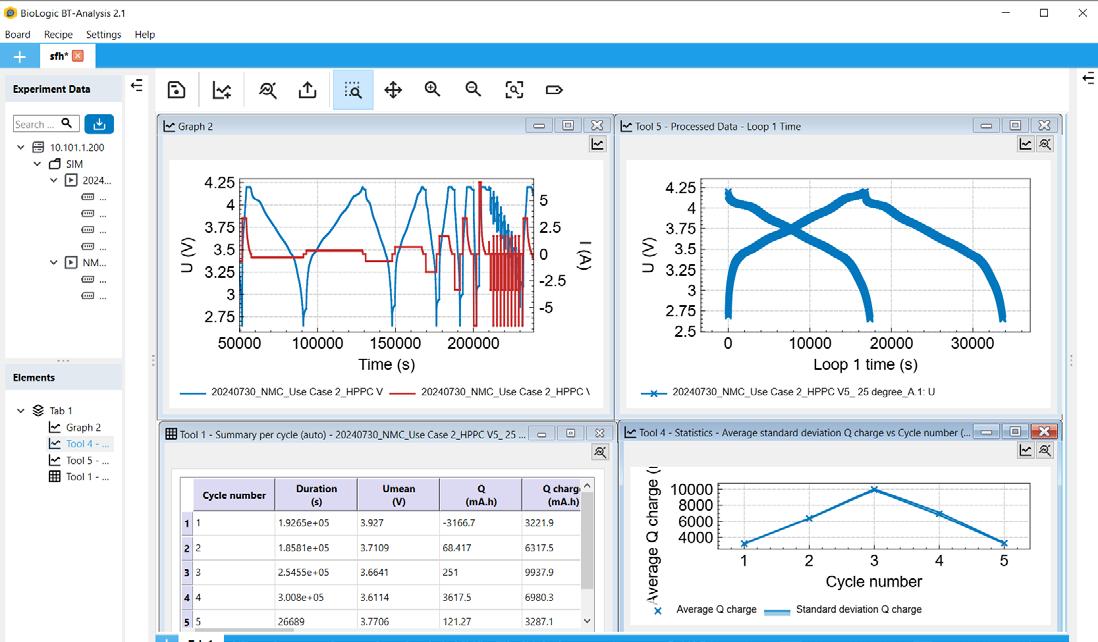
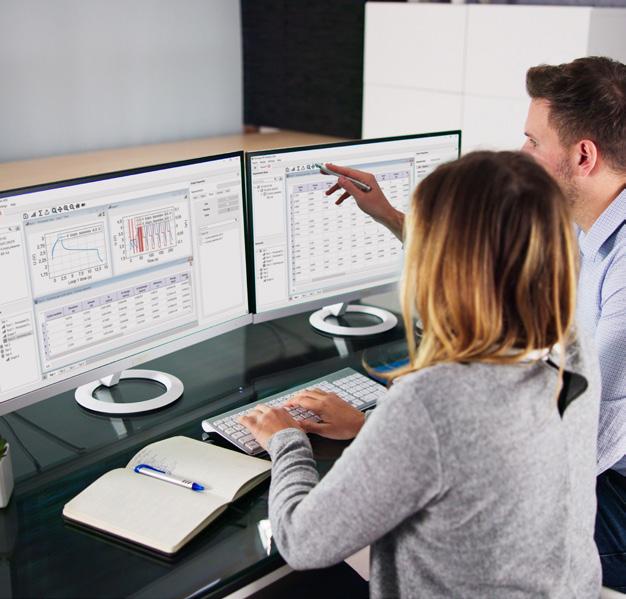
Battery testing with the highest accuracy and precision
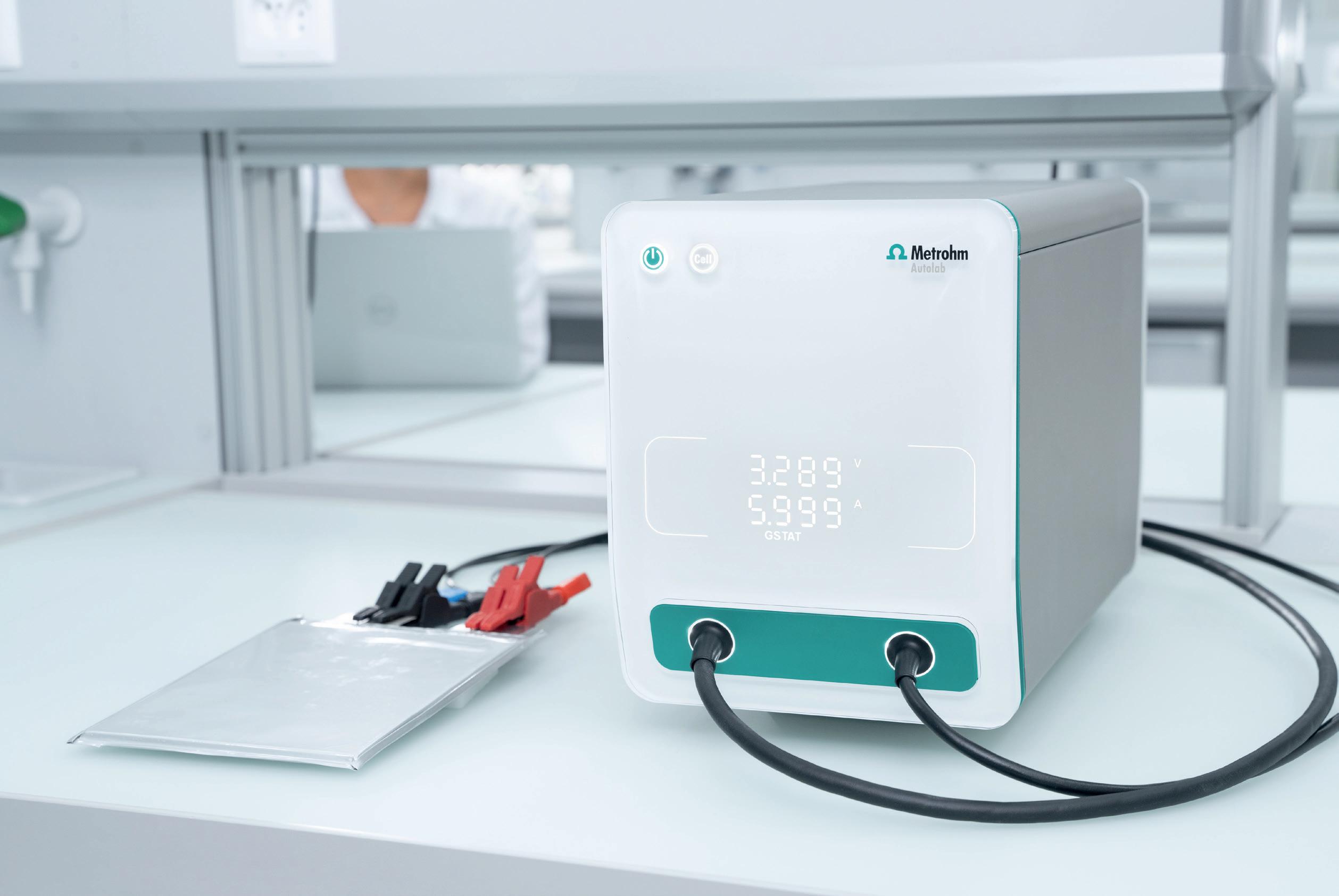
Set safety limits and real-time temperature sensing
Highly flexible and simple Safety
Continuous and automatic data export
On-board memory ensures data safety during long measurements
Built-in procedure
library bursting with essentials to get you started in no time
Command-based procedure editor to design the most elaborate test cases
New battery testing environment with VIONIC powered by INTELLO
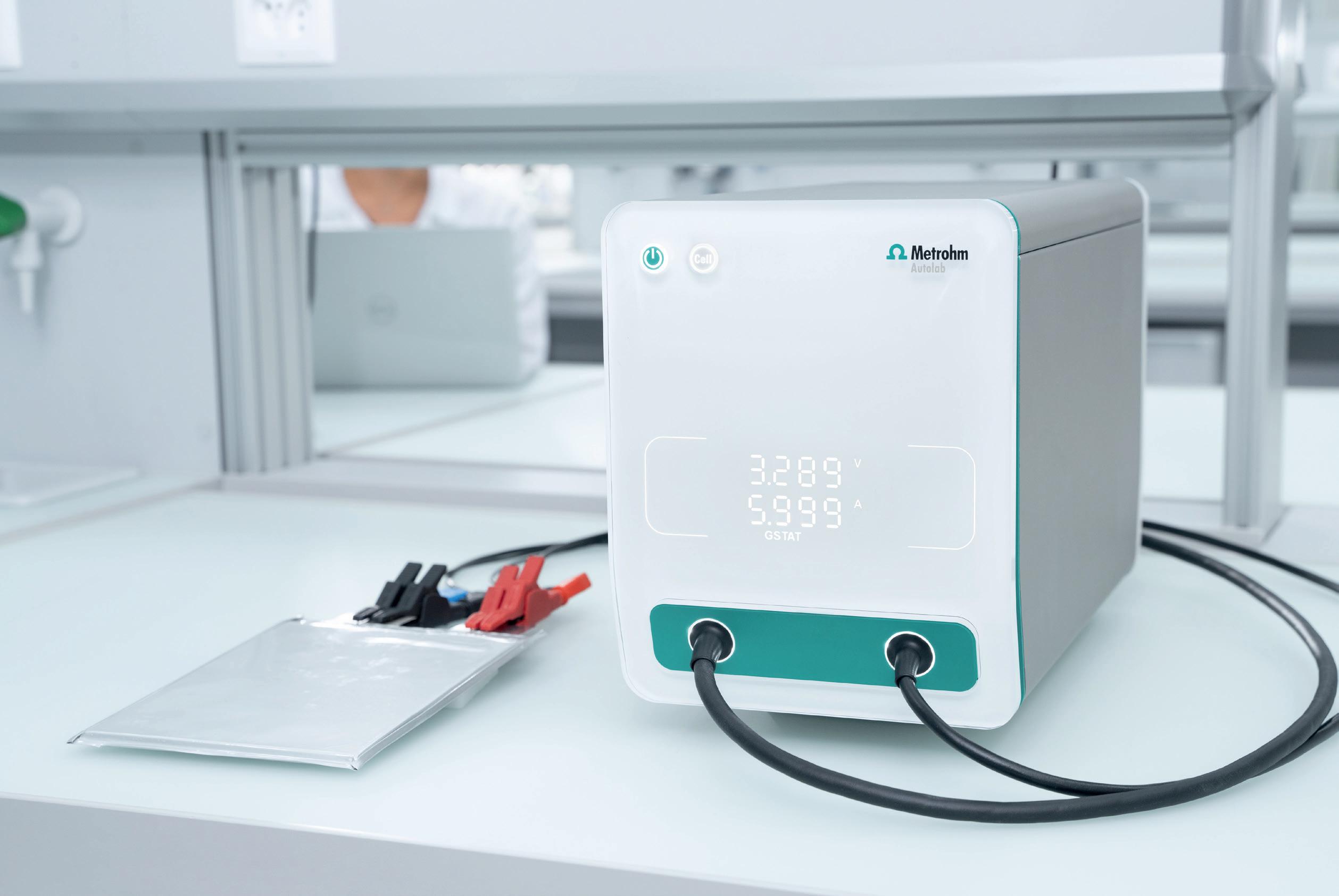
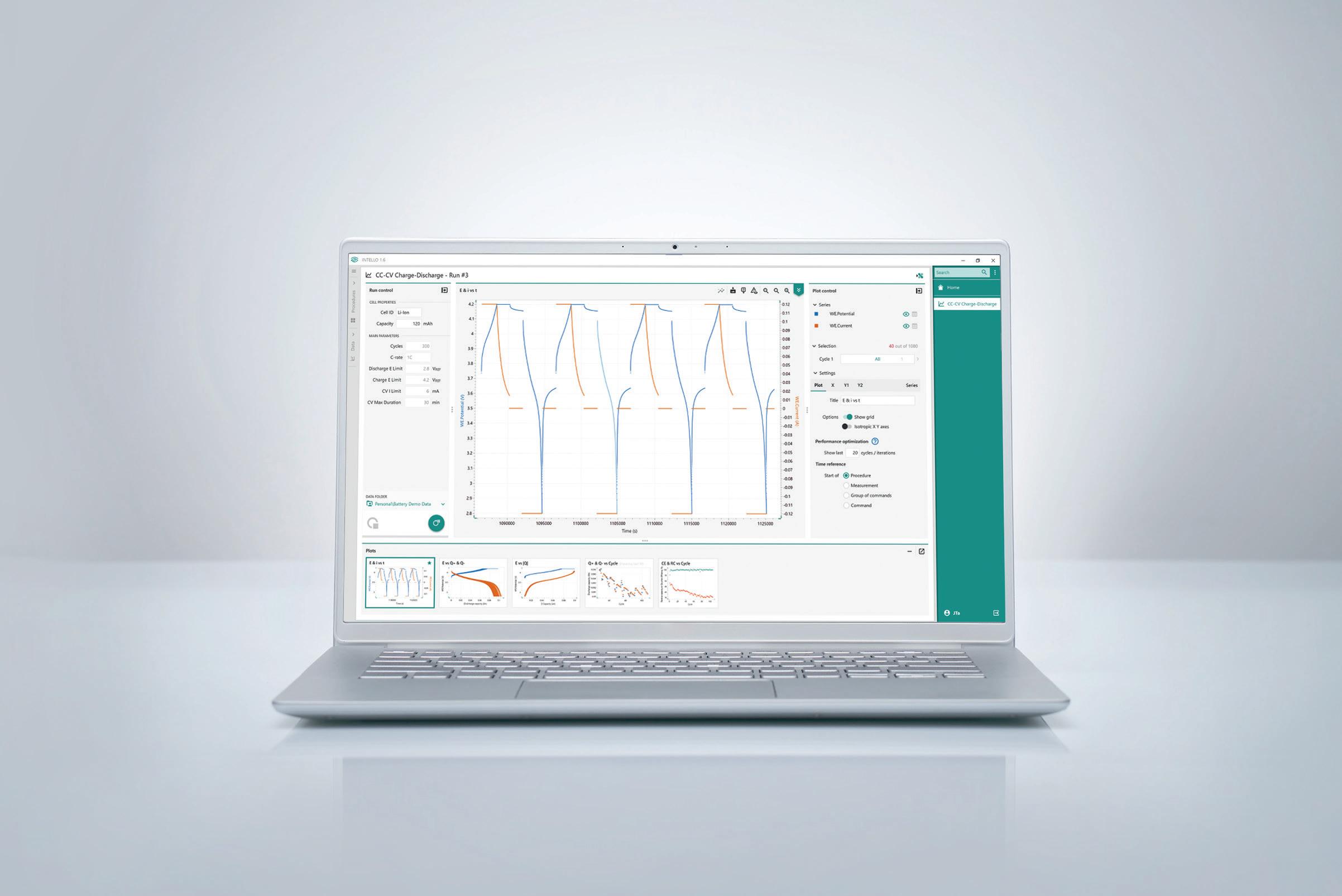
Ready-to-use and fully customizable testing methods
Custom cycling tests (CC, CC-CV, C-rate capabilities)
Cycles and repeat loops with user-defined end-conditions
Deep insights with advanced techniques such as GITT and PITT
High accuracy Impedance Spectroscopy (EIS)
Maximum insights by measuring EIS at any time during the test
Wide range of currents allows for EIS on cells with up to tens of Ah capacity
Simultaneous EIS measurements for both anode and cathode or full cell with an accuracy up to 99.75%
EIS measurements up to 10 MHz for the characterization of solid state batteries
Direct insights with real-time data analysis and smart plotting
Capacity (charge, discharge, total) evaluation
Coulombic efficiency and capacity retention calculation
dQ/dV (differential capacity) representation
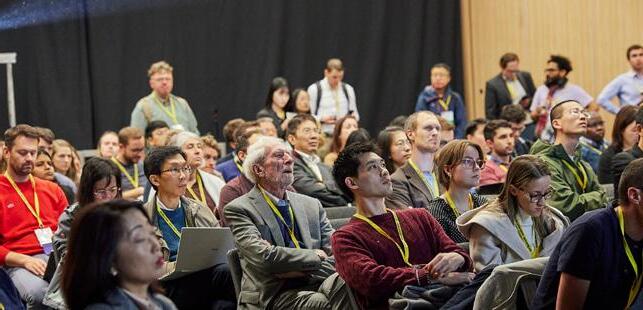
PRIME
2024
TJOINT INTERNATIONAL MEETING
of The Electrochemical Society of Japan, The Korean Electrochemical Society, and The Electrochemical Society
• HONOLULU, HI, USA • OCTOBER 6-11, 2024
he organizers of PRiME 2024 are pleased to welcome everyone back to Honolulu for this, the ninth gathering of PRiME! Since 1987, this joint Pacific Rim international meeting of The Electrochemical Society (ECS), The Electrochemical Society of Japan (ECSJ), and The Korean Electrochemical Society (KECS) has been one of the world’s preeminent meetings on electrochemical and solid state science. This international conference attracts the most active researchers in academia, government, and industry—professionals and students alike—and provides a forum to share their results and discover the latest research from their peers. Make sure to start planning now for these technical and networking opportunities:
• Six days of technical programming across 50 symposia;
• Over 4,800 abstracts;
• More than 3,200 oral presentations, including over 700 invited talks by the world’s leading experts;
• Over 1,500 posters over three evening poster sessions;
• Five full-day Short Courses offered on Sunday;
The Electrochemical Society, ECS
The mission of ECS is to advance theory and practice at the forefront of electrochemical and solid state science and technology, and allied subjects by encouraging research, discussion, critical assessment, and dissemination of knowledge in these fields. Our members are making key discoveries that address global sustainability challenges such as renewable energy, food safety, water sanitation, and medical diagnosis and care.
The Electrochemical Society of Japan, ECSJ
ECSJ was founded to foster scientific and industrial progress in the fields of electrochemistry and industrial physical chemistry. Rapid changes in both academic and industrial environments have been seen, with important increases in the scope covered by the discipline of electrochemistry, as well as in the circumstances affecting the industry in the intervening years. ECSJ continues to remain responsive to this rapid progress and to contribute significantly to advances in this field.
• Six Professional Development Workshops running throughout the week;
• 14 hours of exhibit hall time over three days;
• Daily morning and afternoon coffee breaks;
• Complimentary WiFi in meeting rooms;
• Special program for nontechnical registrants.
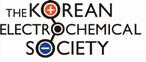
The Korean Electrochemical Society, KECS
KECS is dedicated to promoting academic and technological developments of electrochemical science in both theory and application. KECS also provides training, education, and many opportunities for researchers and engineers to make significant advancements in the field of electrochemistry-based science and technology.
Technical Co-Sponsors
Japan Society of Applied Physics (JSAP)
Semiconductor Physics Division, Korean Physical Society (KPS) Society of Polymer Science, Japan (SPSJ) Korea Photovoltaic Society (KPVS)
Korean Institute of Chemical Engineers (KIChE) The Electrochemical Society of Taiwan (ECSTw) Electrochemistry Division of the Royal Australian Chemical Institute (EDRACI) Semiconductor Physics Division of The Chinese Physical Society (CPS)
Hydrogen Energy Systems Society of Japan (HESS)
The Plenary Lecture Monday, October 7
“Coordination Nanosheets — Electro-functional 2D Polymers of Metal Complexes”
Hiroshi Nishihara, Tokyo University of Science and The University of Tokyo
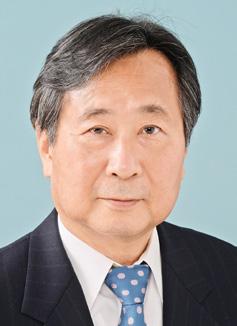
Hiroshi Nishihara is Vice President of Tokyo University of Science and Emeritus Professor of The University of Tokyo (U-Tokyo). His research area is electrochemistry, coordination chemistry, organometallic chemistry, photochemistry, and material science. Since 2013, when Prof. Nishihara synthesized the first example of conducting coordination nanosheets which are organic/ inorganic hybrid 2D materials, he and coworkers have created various coordination nanosheets and discovered their unique properties and functions. In 1982, he completed a DSc at U-Tokyo then was appointed Research Associate at Keio University. He was a Visiting Research Associate at The University of North Carolina at Chapel Hill from 1987 to 1989, then promoted to Associate Professor at Keio University in 1992. He became Professor at The University of Tokyo in 1996, a position he held until he retired
in 2020. Prof. Nishihara has received numerous awards, including the 2016 Chemical Society of Japan Award, 2015 Japan Society of Coordination Chemistry Award, and in 2011, Doctor Honoris Causa from the Université de Bordeaux. He is a Fellow of The Electrochemical Society of Japan (2020) and Fellow of the Royal Society of Chemistry (2014). Prof. Nishihara has served academic societies in diverse roles that include as President of the Japan Society of Coordination Chemistry (2016–2020), President of The Electrochemical Society of Japan (2016–2017), Vice President of The Chemical Society of Japan (2013–2015), and Vice President of the International Society of Electrochemistry (2011–2013). He joined The Electrochemical Society in 1998 and has served with distinction on Society committees and in officer positions for the ECS Japan Section.
His lecture focuses on the rich science and technology of coordination nanosheets relating to electrochemistry and electronics.
PRiME 2024 Partners
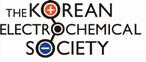

SPECIAL EVENTS
(Consult the meeting app for times.)
Opening Reception
Get a taste of Honolulu and kick off an exciting week! All attendees are welcome for light snacks, an open bar, ample networking time, and a chance to finally relax from your travel into town.
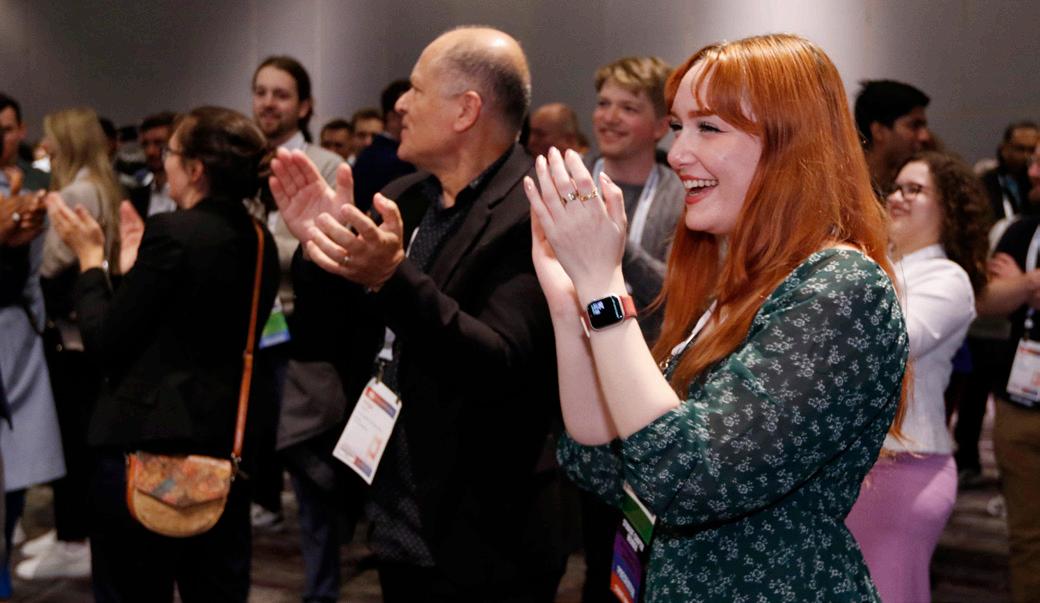
Student Mixer*
Wrap up the meeting’s first full day with friends and peers. Students and early-career professionals mingle in a relaxed setting and enjoy light hors d’oeuvres and refreshments. Be there! Sponsored by Pine Research and Scribner.
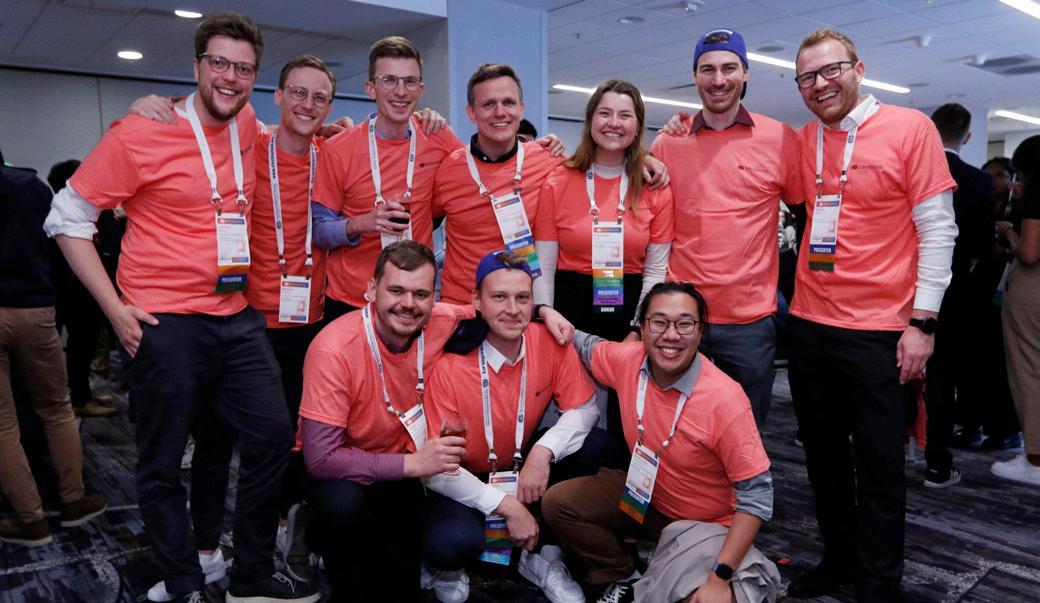
General and Student Poster Sessions
With hundreds of posters to explore, don’t miss a minute of these sessions. Grab a snack, wander the aisles, review presentations, talk to authors, and get to know our exhibitors. These sessions are a great way to end the day!
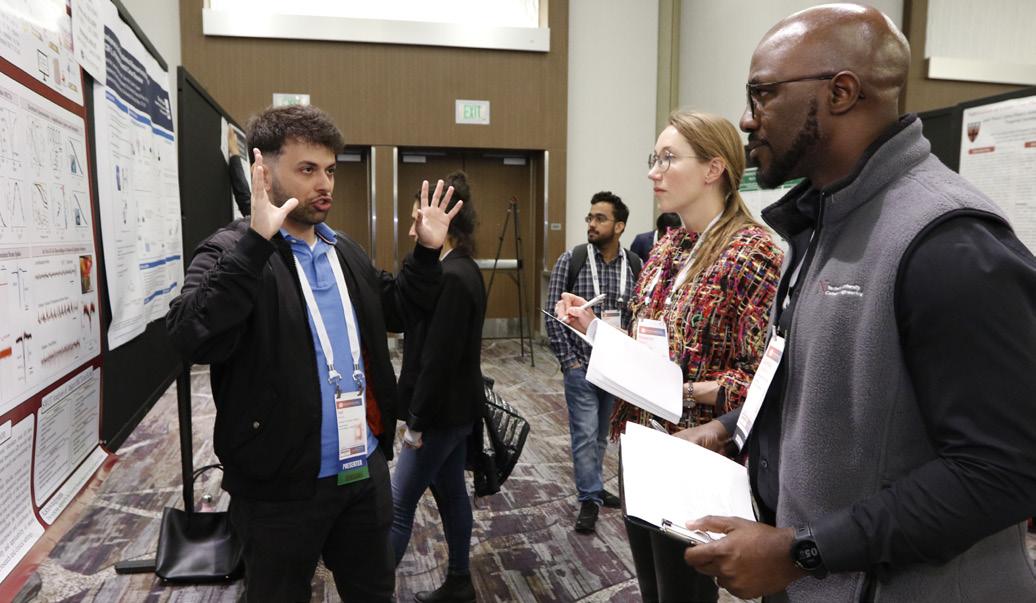
Exhibit Hall
Take time to explore electrochemistry and solid science’s leading vendors’ exhibits. Then stop by the exhibit hall for Poster Sessions, Networking Breaks, Professional Portraits, and the ECS, ECSJ, and KECS booths.
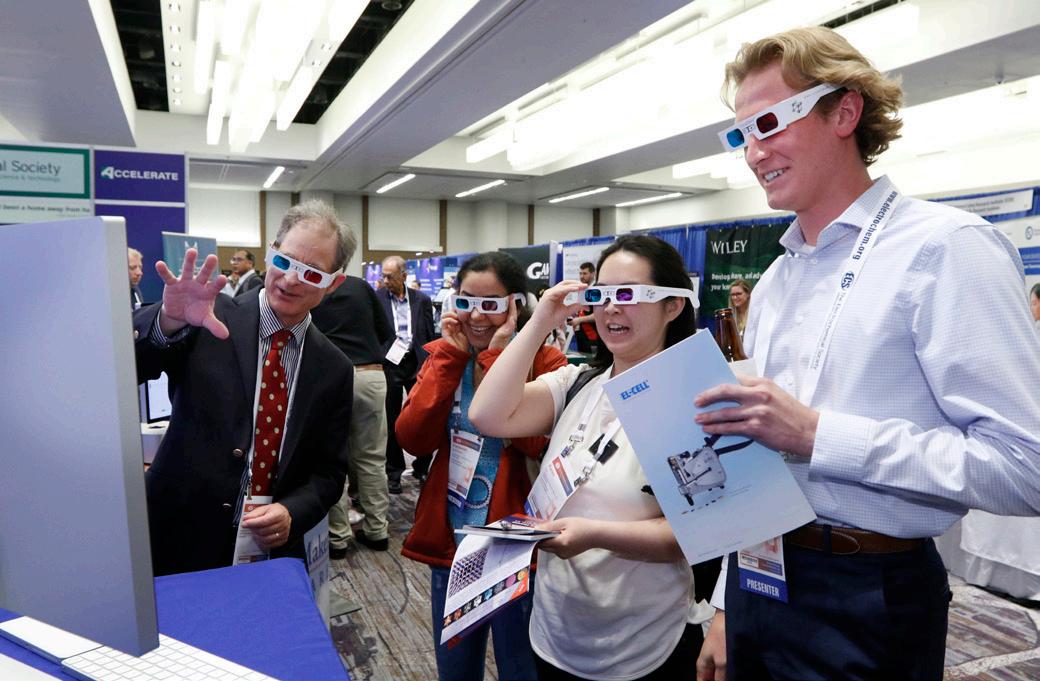
PRiME 2024 Luau*
Enjoy a taste of Hawaiian traditions and join the PRiME ohana to close out the week with the lasting memories of our luau! Music, dance, food, drinks, and a spectacular setting will all be served up for your enjoyment, so make sure to get your ticket soon!
*These events require pre-registration or purchase of a separate ticket.
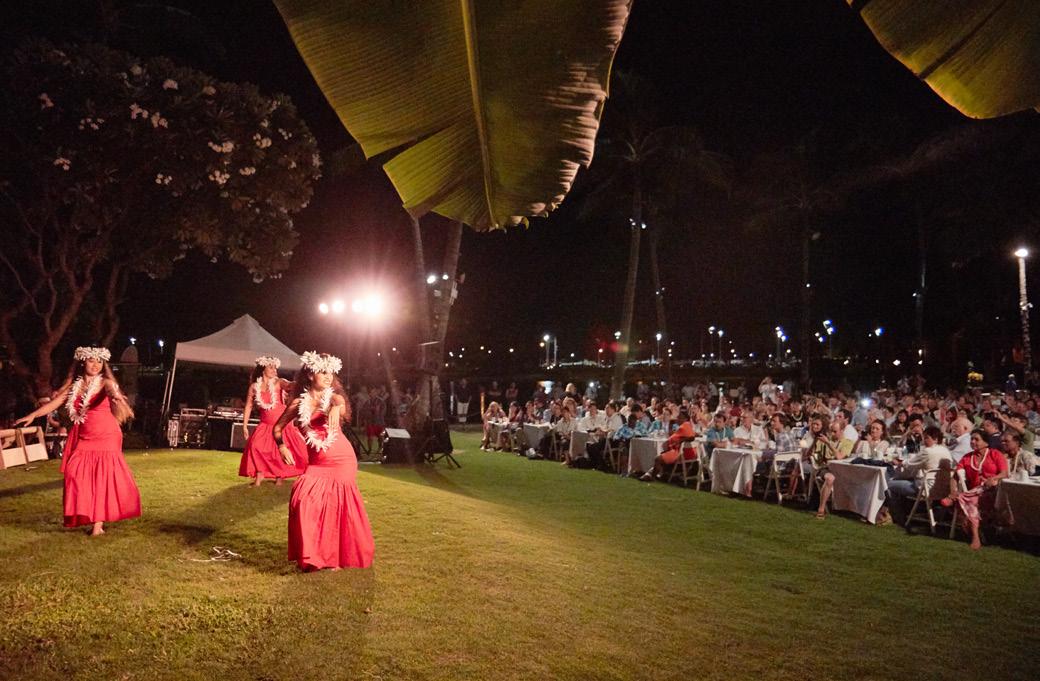
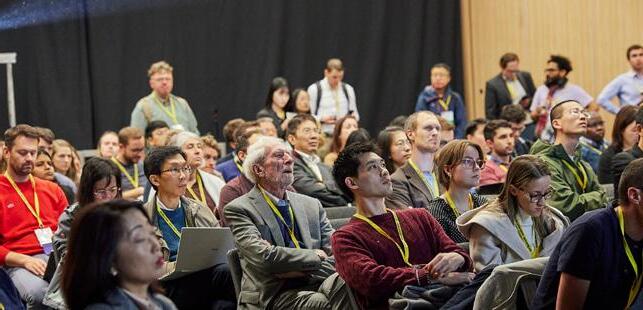
(continued from previous page)
JOINT INTERNATIONAL MEETING
PRIME 2024 • HONOLULU, HI, USA • OCTOBER 6-11, 2024
Symposium Topics
A Batteries and Energy Storage
A01 New Approaches and Advances in Electrochemical Energy Systems
Brett Lucht, Pooi See Lee, Xinliang Feng, Tahoon Kim, James Saraidaridis, Mani Manivannan, Chockkalingam Karuppaiah, Yuliya Preger, Nobuyuki Imanishi, Yukari Sato, Sang-Young Lee, U Hyeok Choi, Hyun-Kyung Kim, Ho Seok Park, Zhong-Shuai Wu
ECS Energy Technology, ECS Battery, ECS Industrial Electrochemistry and Electrochemical Engineering
A02 Batteries with Li Chemistries and Beyond: In Honor of Marca Doeff
Feng Lin, Shirley Meng, Yijin Liu, Jason Croy, Chixia Tian ECS Battery
A03 Accelerating Next-Generation Battery R&D Through DataDriven Approaches
Wei Wang, Eric Dufek, Robert Dominko ECS Battery
A04 Advanced Characterization Techniques in Battery Research
Seongmin Bak, Koji Amezawa, Feng Lin, Kyung-Wan Nam, Sanjeev Mukerjee
ECS Battery, ECSJ Battery, KECS Battery
A05 Advanced Lithium-Ion Batteries for High-Performance Electric Vehicle Applications
Min-Sik Park, Woosuk Cho, Maiyong Zhu, Jung Ho Kim, Genki Kobayashi, Kyung Yoon Chung, Kyu-Young Park
ECS Battery, ECSJ Battery, KECS Battery
A06 Fast Energy Storage Materials and Devices
Ho Seok Park, Jeffrey Long, Veronica Augustyn, Thierry Brousse, Dominic Rochefort, Patrice Simon, Andrea Balducci, Yong-Yao Xia, Hyungseok Kim, Masashi Ishikawa, Katsuhiko Naoi, Soshi Shiraishi, Masayuki Morita, Nae-Lih (Nick) Wu, Ping Liu
ECS Battery, KECS Capacitor, ECSJ Capacitor
A07 Li-ion and Li Metal Batteries: Electrodes, Electrolytes, and Interphases
Wei Tong, Bin Li, Yuki Yamada, Ruhul Amin, Seung-Wan Song, Zhengcheng Zhang, Hochun Lee, Wu Xu, Won-Hee Ryu, Hee-Tak Kim, Jongwoo Lim, Seung-Ho Yu, Hongkyung Lee, Kang Xu, HuiChia Yu
ECS Battery, ECSJ Battery, KECS Battery
A08 Materials and Interfacial Electrochemistry for Solid State Batteries
Jong-Won Lee, Jusik Kim, Yasutoshi Iriyama, Kyung Yoon Chung, Yoon Seok Jung, Yong Min Lee
ECS Battery, ECSJ Battery, KECS Battery
A09 New Materials for Non-Lithium Based Next-Generation Batteries
Hun-Gi Jung, Kyung Yoon Chung, Seung-Taek Myung, Jang-Yeon Hwang, Masaki Matsui
ECS Battery, ECSJ Battery, KECS Battery
B Carbon Nanostructures and Devices
B01 Carbon Nanostructures: From Fundamental Studies to Applications and Devices
Jeff Blackburn, Ardemis Boghossian, Daniel Heller, Simon Corrie
ECS Nanocarbons
C Corrosion Science and Technology
C01 Corrosion General Poster Session
Dev Chidambaram, Eiji Tada
ECS Corrosion
C02 High-Temperature Corrosion and Materials Chemistry 16
Xingbo Liu, Makoto Nanko, Paul Gannon, Dev Chidambaram, Torsten Markus, Jan Froitzheim, E. Opila
ECS High-Temperature Energy, Materials, & Processes, Electrochemical Society of Japan
C03 Critical Factors in Localized Corrosion 11
Gerald Frankel, Sannakaisa Virtanen, Eiji Tada, Rajeev Gupta, Dev Chidambaram, Samantha Gateman
ECS Corrosion
C04 Pits and Pores 10: Nanomaterials - Fabrication, Properties, and Applications
Petra Granitzer, Shinji Yae, Kazuhiro Fukami, Kurt Kolasinski, Giovanni Zangari, Jan Macak, Hiroaki Tsuchiya
ECS Corrosion, Electrochemical Society of Japan
C05 Atmospheric and Marine Corrosion 3
Masayuki Itagaki, Lloyd Hihara, Hideki Katayama, Yoshinao Hoshi
ECS Corrosion, Electrochemical Society of Japan
D Dielectric Science and Materials
D01 Photovoltaics for the 21st Century - 20th Anniversary: New Materials and Processes
Hiroki Hamada
ECS Dielectric Science and Technology, Korean Electrochemical Society, Electrochemical Society of Japan
D02 Semiconductors, Dielectrics, and Metals for Nanoelectronics and Plasma Nanoscience 2
Uroš Cvelbar, Thorsten Lill, Hiroki Kondo, Kay Song, Peter Mascher, Oana Leonte, Kuniyuki Kakushima, Sunghwan Lee, Yaw Obeng, Steve Kilgore, Durga Misra, Eva Kovacevic
ECS Dielectric Science and Technology
E Electrochemical/Electroless Deposition
E01 Electrochemical Deposition for Functional Materials and Energy Applications
Natasa Vasiljevic, Xochitl Dominguez-Benetton, Jin Young Kim, Uk Sim, Soon Hyung Kang, Sudipta Roy, Timothy Hall, Kyoungsuk Jin, Dae-Hyun Nam, Philippe Vereecken, Stanko Brankovic, Andreas Bund, Adriana Ispas, Massimo Innocenti, Luca Magagnin, Krishnan Rajeshwar, Jung Kyu Kim
ECS Electrodeposition, ECS Energy Technology
E03 Electrodeposition and Electroless Deposition for Interconnects in Integrated Circuits and 3D Packaging
Masanori Hayase, Rohan Akolkar, Qiang Huang, Nikolay Dimitrov, Fumihiro Inoue, Myung Jun Kim
ECS Electrodeposition
F Electrochemical Engineering
F01 Advances in Industrial Electrochemistry and Electrochemical Engineering
Paul Kenis, Chockkalingam Karuppaiah, Elizabeth Biddinger
ECS Industrial Electrochemistry and Electrochemical Engineering
F02 Modeling Electrochemical Systems for Transportation Applications 3
Taylor Garrick, John Staser, Steven DeCaluwe, Kevin Leung
ECS Industrial Electrochemistry and Electrochemical Engineering, ECS Battery, ECS Energy Technology
F03 Tutorials and Advances in Electrochemical Impedance Spectroscopy
Mark Orazem, Masayuki Itagaki, Burak Ülgüt, Vincent Vivier
ECS Industrial Electrochemistry and Electrochemical Engineering, ECS Corrosion
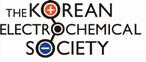

F05 Towards Electrolysis for Sustainability: Innovations in Materials and Processes
Jin Soo Park, Paul Kenis, Sang Hoon Kim, Sunghyun Uhm, K. Andreas Friedrich, Mitsuru Higa, Damilola Daramola
ECS Industrial Electrochemistry and Electrochemical Engineering, Korean Electrochemical Society
G Electronic Materials and Processing
G01 Atomic Layer Deposition and Etching Applications 20 Fred Roozeboom, Stefan De Gendt, Jolien Dendooven, Jeffrey Elam, Oscar van der Straten, Andrea Illiberi, Ganesh Sundaram, Rong Chen, Oana Leonte, Thorsten Lill, Matthias Young, Bhaskar Bhuyan, Alexander Kozen
ECS Electronics and Photonics, ECS Dielectric Science and Technology
G02 18th International Symposium on Semiconductor Cleaning Science and Technology (SCST 18)
Sangwoo Lim, Koichiro Saga, Paul Mertens, Ismail Kashkoush, Jason Keleher, Takeshi Hattori
ECS Electronics and Photonics
G03 SiGe, Ge, and Related Compounds: Materials, Processing, and Devices 11: In Memory of Qizhi Liu
Jean-Michel Hartmann, Mikael Östling, Vibhor Jain, Xiao Gong, Gianlorenzo Masini, Judson Holt, Andreas Mai, Atsushi Ogura, Osamu Nakatsuka, Wengang Bi, Andreas Schulze
ECS Electronics and Photonics, ECS Dielectric Science and Technology
H Electronic and Photonic Devices and Systems
H02 Thin Film Transistors 17 (TFT 17)
Yue Kuo
ECS Electronics and Photonics, ECS Dielectric Science and Technology
H03 Low-Dimensional Nanoscale Electronic and Photonic Devices 17 Yu-Lun Chue, Jyh-Ming Wu, Colm O’Dwyer, Motofumi Suzuki, Song Jin, Sang-Woo Kim, Federico Rosei, Johnny Ho, Zhiyong Fan, Qiliang Li, Jr Hau He, Gary Hunter, Kuniharu Takei, Lain-Jong Li, Peter Mascher
ECS Electronics and Photonics, ECS Dielectric Science and Technology
H04 Gallium Nitride and Silicon Carbide Power Technologies 14
Michael Dudley, Balaji Raghothamachar, Noboru Ohtani, Mietek Bakowski
ECS Electronics and Photonics
H05 Electronic, Thermal, and Electrochemical Properties of Metal Organic Frameworks (MOFs) 3: Technology, Applications, and Emerging Devices
Christopher Wilmer, Helmut Baumgart, Christof Wöll, Hiroshi Kitagawa, Paolo Falcaro, Mark Allendorf, Gang Wu
ECS Electronics and Photonics, ECS Energy Technology, ECS HighTemperature Energy, Materials, & Processes
H06 Chromogenic Materials and Devices
Atsushi Aoki, Norihisa Kobayashi, Chi Hwan Han
ECS Electronics and Photonics, Korean Electrochemical Society, ECSJ Chromogenic Research
H07 Piezocatalysis
Jyh-Ming Wu, Jun Chen, Hsueh-Shih Chen, Che-Ning Yeh, Hongwei Huang, Chris Bowen, Chih-Yen Chen, Xudong Wang, Wenzhuo Wu
ECS Energy Technology, ECS Dielectric Science and Technology, ECS Electronics and Photonics, ECSTw
I Fuel Cells, Electrolyzers, and Energy Conversion
I01A Polymer Electrolyte Fuel Cells and Water Electrolyzers 24 (PEFC&WE 24) - Fuel Cell Electrocatalyst Activity and Durability
Hiroyuki Uchida, Peter Strasser, Yong-Tae Kim, Ulrike Kramm, Katsuyoshi Kakinuma, Jeong Woo Han, Karen Swider-Lyons
ECS Energy Technology, ECS Battery, ECS Corrosion, ECS
Industrial Electrochemistry and Electrochemical Engineering, ECS
Physical and Analytical Electrochemistry, ECSJ PEFC, KECS Fuel Cells and Electrolyzers
I01B Polymer Electrolyte Fuel Cells and Water Electrolyzers 24 (PEFC&WE 24) - Electrolyzer Electrocatalyst Activity and Durability
Bryan Pivovar, Marian Chatenet, Don-Hyung Ha, Sang Hoon Joo, Karen Swider-Lyons, Hui Xu, Shigenori Mitsushima
ECS Energy Technology, ECS Battery, ECS Corrosion, ECS
Industrial Electrochemistry and Electrochemical Engineering, ECS
Physical and Analytical Electrochemistry, ECSJ PEFC, KECS Fuel Cells and Electrolyzers
I01C Polymer Electrolyte Fuel Cells and Water Electrolyzers 24 (PEFC&WE 24) - Ionomers, Membranes, and Separators for Fuel Cells and Electrolyzers
Ahmet Kusoglu, Deborah Jones, Peter Pintauro, Michael Hickner, Craig Gittleman, Karen Swider-Lyons
ECS Energy Technology, ECS Battery, ECS Corrosion, ECS
Industrial Electrochemistry and Electrochemical Engineering, ECS
Physical and Analytical Electrochemistry, ECSJ PEFC, KECS Fuel Cells and Electrolyzers
I01D Polymer Electrolyte Fuel Cells and Water Electrolyzers 24 (PEFC&WE 24) - Fuel Cell Electrodes, MEAs, and Diagnostics
Erik Kjeang, Adam Weber, Jens Eller, Marc Secanell, Segeun Jang, Karen Swider-Lyons
ECS Energy Technology, ECS Battery, ECS Corrosion, ECS
Industrial Electrochemistry and Electrochemical Engineering, ECS
Physical and Analytical Electrochemistry, ECSJ PEFC, KECS Fuel Cells and Electrolyzers
I01E Polymer Electrolyte Fuel Cells and Water Electrolyzers 24 (PEFC&WE 24) - Electrolyzer Electrodes, MEAs, and Diagnostics
Jasna Jankovic, William Mustain, Dario Dekel, Marc Secanell, Yuki Orikasa, Karen Swider-Lyons
ECS Energy Technology, ECS Battery, ECS Corrosion, ECS
Industrial Electrochemistry and Electrochemical Engineering, ECS
Physical and Analytical Electrochemistry, ECSJ PEFC, KECS Fuel Cells and Electrolyzers
I01F Polymer Electrolyte Fuel Cells and Water Electrolyzers 24 (PEFC&WE 24) - Cells, Stacks, and Systems for Fuel Cells and Electrolyzers
Balasubramanian Lakshmanan, Cynthia Rice, Karen Swider-Lyons, Christopher Capuano, Robert Mantz, Han-Ik Joh
ECS Energy Technology, ECS Battery, ECS Corrosion, ECS
Industrial Electrochemistry and Electrochemical Engineering, ECS
Physical and Analytical Electrochemistry, ECSJ PEFC, KECS Fuel Cells and Electrolyzers
I01Z Polymer Electrolyte Fuel Cells and Water Electrolyzers 24 (PEFC&WE 24) - Plenary Session
Karen Swider-Lyons
ECS Energy Technology, ECS Battery, ECS Corrosion, ECS
Industrial Electrochemistry and Electrochemical Engineering, ECS
Physical and Analytical Electrochemistry, ECSJ PEFC, KECS Fuel Cells and Electrolyzers
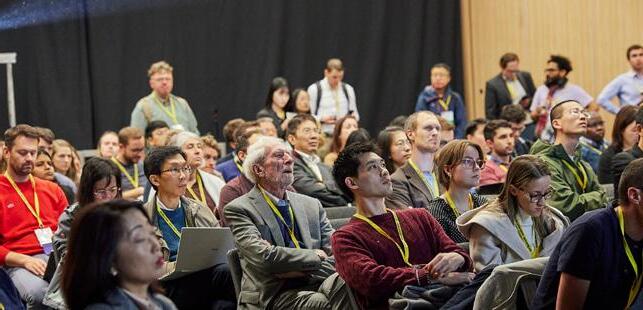
(continued from previous page)
PRIME
I02 Solid State Ionic Devices 15
JOINT INTERNATIONAL MEETING
Symposium Topics
Cortney Kreller, Hitoshi Takamura, Jong-Ho Lee, Xinfang Jin, Kannan Ramaiyan, Jagjit Nanda
ECS High-Temperature Energy, Materials, & Processes, ECS Battery, ECSJ Solid State Chemistry, Korean Electrochemical Society
I03 Seawater Electrodialysis and Electrolysis for Water-Energy Nexus
Hyunwoong Park, Xiao Su, Xing Xie, Dong Suk Han, Myeongjin Kim, Juan Lopez-Ruiz, Kangwoo Cho, Choonsoo Kim, Seung Woo Lee, Ho Shon, Chia-Hung Hou
ECS Industrial Electrochemistry and Electrochemical Engineering, ECS Physical and Analytical Electrochemistry, Korean Electrochemical Society
J Luminescence and Display Materials, Devices, and Processing
J01 Luminescence and Display Materials: Fundamentals and Applications: In Honor of Baldassare Di Bartolo Rong-Jun Xie, Jakoah Brgoch, Zhiguo Xia, Andries Meijerink, Mikhail Brik, Alok Srivastava, John Collins, Kailash Mishra, Won Bin Im, Joohoon Kim, Ik-Soo Shin, Donghoon Han, Seung-Ryong Kwon, Eugeniusz Zych
ECS Luminescence and Display Materials, Korean Electrochemical Society
K Organic and Bioelectrochemistry
K01 New Developments in Synthetic Organic Electrochemistry: In Memory of Tatsuya Shono, Tsutomu Nonaka, and Ikuzo Nishiguchi
Toshiki Nokami, Hisashi Shimakoshi, Kevin Moeller, Flavio Maran
ECS Organic and Biological Electrochemistry, Electrochemical Society of Japan
K02 Pioneering the Future with Bioengineering and Electrochemistry
Shelley Minteer, Koji Sode, Ariel Furst, Jeffrey Dick, Haesik Yang, Hitoshi Shiku, Wakako Tsugawa, Seong Jung Kwon, Seiya Tsujimura, Hisakage Funabashi
ECS Organic and Biological Electrochemistry, Korean Electrochemical Society, Electrochemical Society of Japan
L Physical and Analytical Electrochemistry, Electrocatalysis, and Photoelectrochemistry
L01 Physical and Analytical Electrochemistry, Electrocatalysis, and Photoelectrochemistry General Session
Stephen Paddison, Anne Co, Alice Suroviec
ECS Physical and Analytical Electrochemistry
L02 Molten Salts and Ionic Liquids 24 (MSIL-24) in Memory of Yasuhiko Ito
Mikito Ueda, David Durkin, Andreas Bund, Adriana Ispas, Vito Di Noto, W. Matthew Reichert, Robert Mantz, Hugh De Long, Allan East, Paul Trulove, Tetsuya Tsuda
ECS Physical and Analytical Electrochemistry, ECS
Electrodeposition, ECS Energy Technology, ECS Industrial Electrochemistry and Electrochemical Engineering, ECSJ Molten Salt
L03 Electrode Processes 15
Andrew Hillier, Nagahiro Hoshi, Kikuo Komori
ECS Physical and Analytical Electrochemistry, ECS Energy Technology
L04 Photocatalysts, Photoelectrochemical Cells, and Solar Fuels 14 Nianqiang Wu, Gary Wiederrecht, Tetsu Tatsuma, Tsukasa Torimoto, Mani Manivannan, Dongling Ma, Thomas Hamann, Han-Hee Cho, Ki Min Nam, Hyunwoong Park, Jong Hyeok Park, Jeongsuk Seo, Haining Tian, Yingjie Hang, Heli Wang, Jae-Joon Lee, Pawel Kulesza
ECS Energy Technology, ECS Physical and Analytical Electrochemistry, ECS Sensor, Korean Electrochemical Society
L05 Advanced Techniques for In Situ Electrochemical Systems 7 Svitlana Pylypenko, Hye Byon, Jongwoo Lim, Dong Young Chung, Iryna Zenyuk, Yun Jeong Hwang, Youngkook Kwon, Anne Co, Yingjie Zhang
ECS Physical and Analytical Electrochemistry, ECS Energy Technology, Korean Electrochemical Society
L06 Electrocatalysis at the Interface: From Fundamental Studies to Applications
Chang Hyuck Choi, Hyungjun Kim, Yingjie Zhang, Stefan Ringe, Dong Young Chung, Jinho Chang, Hyun Ahn
ECS Physical and Analytical Electrochemistry, Korean Electrochemical Society
L07 Fundamentals of Carbon Dioxide Reduction
Pawel Kulesza, Iwona Rutkowska, Paul Kenis, Plamen Atanassov
ECS Physical and Analytical Electrochemistry, ECS Energy Technology, ECS Industrial Electrochemistry and Electrochemical Engineering, ECS Organic and Biological Electrochemistry
M Sensors
M01 Recent Advances in Sensors Systems: General Session
Thomas Thundat, Jessica Koehne, Larry Nagahara, Dong-Joo Kim, Leyla Soleymani, Aida Ebrahimi, Lok-kun Tsui, Peter Hesketh, Dongmei Dong, Takeo Hyodo, Ajit Khosla
ECS Sensor, Electrochemical Society of Japan, Korean Electrochemical Society
M02 Chemical Sensors 14
Praveen Kumar Sekhar, Larry Nagahara, Tomoyuki Yasukawa, Masanobu Matsuguchi, Tsuyoshi Tanaka, Shinji Tamura, Takeo Hyodo, Vinoth Ponnusamy, Lok-kun Tsui, Rangachary Mukundan, Jessica Koehne, Thiagarajan Soundappan
ECS Sensor, Electrochemical Society of Japan
Z General
Z01 General Student Poster Session
Alice Suroviec, Naoaki Yabuuchi, Minhong Lim, Youngkook Kwon
All divisions of ECS, ECSJ, and KECS
Z02 A Lifetime of Cleaning the Planet: In Honor of Akira Fujishima
Larry Nagahara, Ajit Khosla, Milad Navaei, Raluca Van Staden, Tetsu Tatsuma, Tsukasa Torimoto, Hiroshi Irie, Pawel Kulesza, Mani Manivannan, Nianqiang Wu
ECS Sensor, ECS Physical and Analytical Electrochemistry, ECS Energy Technology, Interdisciplinary Science and Technology Subcommittee, Korean Electrochemical Society, Electrochemical Society of Japan
Z03 Innovation in Electrochemistry
Johna Leddy, K. Andreas Friedrich, Mitsuru Higa, Damilola Daramola, Paul Kenis, Trung Nguyen, Jun Hui Park, E. Jennings
Taylor, Byung-Kwon Kim, Andrew Herring, Jin Soo Park, Sang Hoon Kim, Sunghyun Uhm
ECS Physical and Analytical Electrochemistry, ECS Energy Technology, ECS Industrial Electrochemistry and Electrochemical Engineering, Korean Electrochemical Society
HOW ARE YOU REPRESENTED AT PRIME 2024
2,121 student abstracts
946 student posters
674 invited talks
25 ECS award & keynote talks 1,171 student oral talks
3,249 total oral talks
4,854 total abstracts
1,583 total posters
572 sessions
50 symposia
60 countries represented
PRIME 2024 BY THE NUMBERS
SOCIETY NEWS SOCIETY NEWS
Publications Update: From the Director’s Desk
by Adrian T. Plummer, MPA, PMP, Senior Director of Publications
As we navigate the ever-evolving landscape of scientific research, the importance of maintaining rigorous ethical standards in our scholarly endeavors cannot be overstated. Recent discussions, such as those highlighted in a recent article on Science.org,1 bring to light the pressing challenges our community faces concerning research misconduct and its implications for the integrity of the scholarly record.
At The Electrochemical Society (ECS), our publications embody our steadfast commitment to upholding the highest standards of research ethics. This commitment is crucial not only for preserving the credibility and trustworthiness of our publications but also for fostering an environment of integrity and excellence within the scientific community. It is the collective responsibility of authors, editors, and reviewers to adhere diligently to these ethical standards.
One essential resource for ensuring ethical compliance is the ECS Author Submission Guidelines and Policies,2 which provide comprehensive guidelines on manuscript preparation, submission, and the ethical responsibilities of authors. These guidelines are available on our official website and are updated regularly. Familiarizing yourself with these guidelines is a critical step in ensuring that your work meets the standards expected by ECS.
Additionally, I encourage all authors to review the authorship criteria outlined by the International Committee of Medical Journal Editors (ICMJE) 3 The ICMJE recommendations clearly define the roles and responsibilities of authors and contributors, emphasizing the necessity of substantial contributions and accountability for the content of the work. Adhering to these criteria helps to prevent issues such as honorary authorship and ghostwriting, which can undermine the integrity of scientific publications.
ECS is also a proud member of the Committee on Publication Ethics (COPE), 4 which provides further guidance on matters of
misconduct and allegations of misconduct. COPE’s resources and standards serve as an invaluable framework for addressing ethical issues and ensuring that any concerns are handled with the utmost rigor and transparency.
The challenges highlighted in the Science.org article underscore the vigilance required to protect the scholarly record. As members of a leading scientific society, we have a collective duty to uphold the principles of ethical research. This involves not only conducting research with honesty and transparency but also critically reviewing and validating the work of our peers.
Maintaining the highest standards of ethics in our research is not merely a procedural requirement; it is moral imperative. By adhering to the ECS guidelines, the ICMJE authorship criteria, and the COPE standards, we can ensure that our contributions to the field of electrochemistry and solid state science are both innovative and ethically sound. Together, let us uphold the values that define The Electrochemical Society and continue to advance the frontiers of knowledge with integrity and excellence. Let us work together to preserve the integrity of the scholarly record and contribute to the scientific community with unwavering commitment to ethical standards.
References
1. I. Oransky and B. Redman, Science, 383(6679), 131 (2024).
2. The Electrochemical Society, ECS Author Submission Guidelines and Policies (n.d).
3. International Committee of Medical Journal Editors, Defining the Role of Authors and Contributors (2023).
4. Committee on Publication Ethics, (n.d.).
Editorial Updates Fall 2024
Reappointments
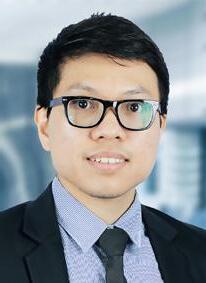
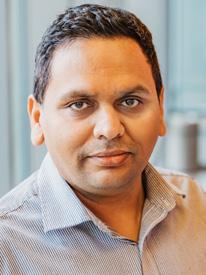
Itthipon Jeerapan
Reappointed as Associate Editor for ECS Sensors Plus for the two-year term July 17, 2024 – July 16, 2026
Praveen Sekhar
Reappointed as Associate Editor for ECS Sensors Plus for the two-year term August 6, 2024 – August 5, 2026
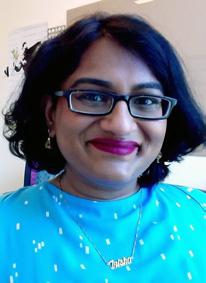
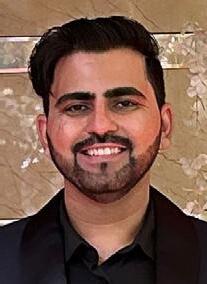
Trisha Andrews
Reappointed as Associate Editor for ECS Sensors Plus for the two-year term September 5, 2024 –September 4, 2026
Vishal Chaudhary
Reappointed as Associate Editor for ECS Sensors Plus for the two-year term July 17, 2024 – July 16, 2026
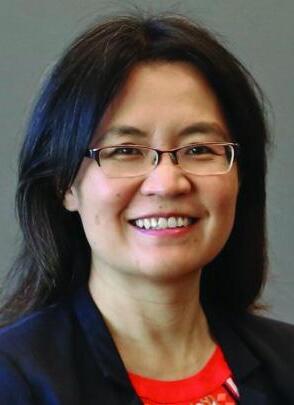
Yue Qi
Reappointed as Associate Editor for the ECS Journal of Solid State Science and Technology for the two-year term September 1, 2024 –August 31, 2026
Honoring Dr. Robert Savinell: A Legacy of Leadership and Innovation
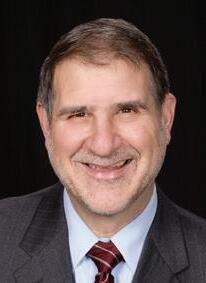
The ECS Publications Team is honored to recognize Dr. Robert Savinell for his outstanding contributions and unwavering dedication over the past eleven years as the Editor-in-Chief of the Journal of The Electrochemical Society (JES). As he transitions to his new roles as the 3rd Vice President of ECS and the Vice Chair of the ECS Publications Subcommittee, we reflect on his remarkable journey and on the indelible impact he has made on the field of electrochemistry.
Dr. Savinell’s journey with ECS has been marked by a deep commitment to advancing electrochemical science and engineering. His tenure as JES Editor-in-Chief has been characterized by an unwavering dedication to excellence, fostering a culture of rigorous scientific inquiry, and nurturing the next generation of researchers. Under his leadership, JES has seen substantial growth in its impact and reach, becoming a premier platform for the dissemination of groundbreaking research in electrochemistry.1
A Distinguished University Professor and the George S. Dively Professor of Engineering at Case Western Reserve University, Dr. Savinell’s academic and research contributions are both prolific and profound. His research interests span a wide range of topics within electrochemical engineering, including fuel cells, batteries, and electrochemical reactors. His work has been instrumental in advancing our understanding of electrochemical systems and their practical applications.2
Dr. Savinell’s scholarly impact is reflected in his extensive list of publications and citations. Among his most cited works are seminal papers on the modeling and optimization of electrochemical systems,
which have become foundational references in the field. His work on redox flow batteries and the development of innovative materials for energy storage and conversion has garnered significant attention and acclaim, influencing both academic research and industrial practices.3
Dr. Savinell has been a mentor and inspiration to countless students and colleagues, and most importantly a leader to our esteemed Editorial Board members. His commitment to education and mentorship has helped shape the careers of many successful electrochemists, who continue to build on his legacy of excellence. His leadership style, characterized by inclusivity, collaboration, and a relentless pursuit of knowledge, has set the standard for others to follow.
As Dr. Savinell steps into his new role within ECS, we look forward to his continued contributions to the Society and the broader scientific community. His vision and expertise will undoubtedly drive forward the mission of ECS to advance the theory and practice of electrochemistry and solid state science and technology. We are excited about the future and confident that under his leadership, ECS will continue to thrive and innovate.
We extend our deepest gratitude to Dr. Savinell for his exceptional service as Editor-in-Chief of JES. His legacy of leadership, innovation, and dedication will continue to inspire and guide us. We are privileged to have had the opportunity to work with him and look forward to his ongoing contributions to ECS in his new capacities.
References
1. The Electrochemical Society, Dr. Robert Savinell (2023).
2. Case Western Reserve University, Robert F. Savinell (2023).
3. Google Scholar, Robert F. Savinell (2023).
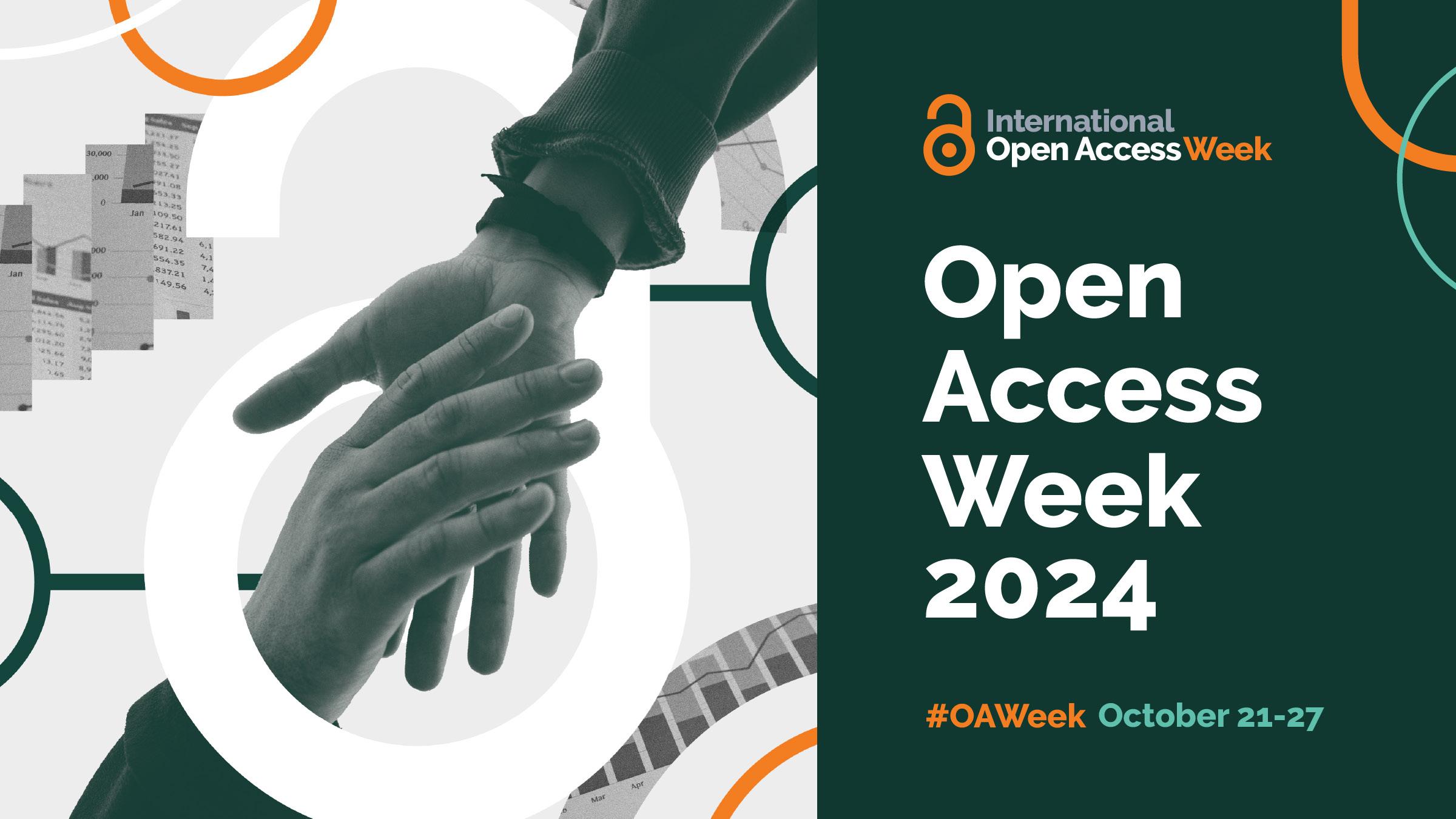
Robert Savinell
SOCIETY NEWS SOCIETY NEWS
ECS Board of Directors Report
The ECS Board of Directors held its fall gathering on Thursday, May 30, in conjunction with the 245th ECS Meeting in San Francisco. ECS President Gerardine Botte called the Board to order and kicked off what was to be one of the most impactful Board meetings held in recent years. In addition to the reports on the major operations and initiatives of the Society, key motions were passed to update ECS’s membership offerings; create a new officer position dedicated to increasing diversity, equity, and inclusion; streamline how the organization funds its many divisions; and launch a record number of new student chapters!
ECS Secretary Marca Doeff then presented the minutes from the previous board meeting and had the pleasure of announcing recently elected board members Sreeram Vaddiraju, ECS Dielectric Science and Technology Division Chair; Paul Kenis, ECS Industrial Electrochemistry and Electrochemical Division Chair; and Jeffrey Blackburn, ECS Nanocarbons Division Chair. Their two-year terms began immediately following the board meeting and end in May 2026. Congratulations and best of luck to our newly elected board members!
Following the Secretary’s Report, ECS Treasurer Lisa PodlahaMurphy discussed the state of ECS’s finances, noting a rebound in ECS’s investment portfolio and that the Society’s financial performance was far better than initially budgeted for the 2023 year. Despite investing over $90,000 in new products and services to advance the mission, strong meeting and publications revenue covered the majority of that investment, allowing us to preserve the gains in our investment portfolio. The bulk of Lisa’s presentation was devoted to revisions to ECS’s division funding plan. Developed over the last 18 months in close cooperation with division leadership, the Finance Committee, and staff, the new division funding plan simplifies and streamlines how divisions are funded and better aligns division funding with major Society operations. This is critical to ensuring that ECS and its divisions work in concert to advance our collective mission.
Next, Past President and Nominating Committee Chair Turgut Gür presented the officer slate for the 2025 ECS Election. ECS Secretary Marca Doeff and ECS Individual Membership Chair E. J. Taylor were unanimously approved to be on next year’s election slate for the position of 3rd Vice President. Congratulations to E. J. and Marca. You both “do the Society proud” and are outstanding candidates!
Director of Community Engagement Shannon Reed then reported on ECS membership for Individual Membership Committee Chair E. J. Taylor who was unable to attend the meeting. ECS membership continues to grow following our pandemic low a few years back and is now higher than at any point since 2015! Further evidence
of growth in membership is seen in the formation of the new ECS Thailand Section and 11 ECS Student Chapters. Located in India, Mexico, the Republic of Korea, Singapore, Thailand, and the United States, these new chapters highlight the truly global nature of ECS. Congratulations and best of luck to all our new chapters and student members!
The Membership Report concluded with ECS Executive Director/CEO Chris Jannuzzi presenting a motion to create a new membership category, called “ECS Associate.” Designed to lower the bar to entry into the Society and increase the opportunities for meaningful engagement with ECS, the Associate distinction will be available to nonmembers as a thank you for engagement with ECS, such as reviewing a paper, presenting at an ECS meeting, serving on an ECS committee, etc. Although they do not receive the same level of benefits afforded full members, Associates will receive discounts on ECS registration fees and other ECS products and services. Most importantly, Associates will be recognized and rewarded for being part of the global ECS community and advocates for advancing ECS’s vision and mission.
Next, Education Committee Chair Alice Suroviec reported on ECS’s educational activities, highlighting this year’s ECS Toyota Young Investigator Fellowship recipients: Prof. Zheng Chen, University of California, San Diego; Prof. Zhongyang Wang, University of Alabama; and Dr. Juner Zhu, Northeastern University. Each ECS Toyota Fellow receives $50,000 to advance their innovative research.
ECS Vice President and Technical Affairs Committee Chair Colm O’Dwyer’s report followed. He updated the Board on the latest information on ECS publications and meetings operations, noting the strong performance of both with over 3,000 abstracts received for the 245th ECS Meeting and nearly 5,000 for PRiME 2024. Together, these two critical operations lines continue to provide not only the majority of resources to advance the mission of ECS, but they are also the mechanisms by which the Society disseminates its community’s research to the broader world. Colm concluded his report by thanking Interdisciplinary Science and Technology Subcommittee (ISTS) Chair Jennifer Hite for her work expanding ISTS’s role in programming symposia across divisions and incubating new and emerging technologies within the Society’s field of interest.
The meeting concluded with the report from Honors and Awards Chair Adam Weber. He presented the motion to approve the 2024 Class of Fellows, along with this year’s Society award winners: Betar Gallant for the Charles W. Tobias Young Investigator Award, and Paul Kohl for the Edward G. Acheson Award.
Last, a motion to close the meeting was made, seconded, and unanimously approved. The Board will reconvene in October during PRiME 2024.
SOCIETY NEWS SOCIETY NEWS
2024–2025 ECS Toyota Young Investigator Fellows
Zheng Chen, Zhongyang Wang, and Juner Zhu received the 2024–2025 ECS Toyota Young Investigator Fellowships for projects in green energy technology. The fellowship program is a partnership between The Electrochemical Society and the Toyota Research Institute of North America (TRINA), a Toyota Motor North America (Toyota) R&D division that explores future technology. Through this program, ECS and Toyota promote innovative and unconventional green energy technologies born from electrochemical research and encourage young professionals and scholars to pursue battery and fuel cell research. The Fellows each receive a $50,000 grant to conduct the research outlined in their proposals and a one-year complimentary ECS membership. This is the tenth year that the fellowships have been awarded. Since its inception, the program has awarded more than $1.65M in research funding to 33 young investigators (including the 2024–2025 recipients).
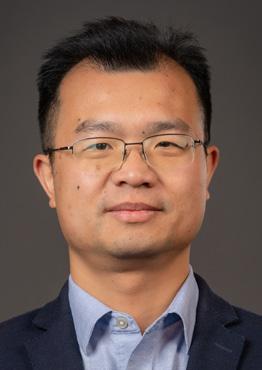
Zheng Chen
University of California, San Diego
“Enabling Greener and More Sustainable Materials for Electrochemical Energy Storage and Conversion”
Zheng Chen is Professor in the Aiiso Yufeng Li Family Department of Chemical and Nano Engineering, University of California, San Diego. His research focuses on materials development for applications in electrochemical energy, flexible devices, and a sustainable environment. Through the ECS Toyota Young Investigator Fellowship, Dr. Chen will address water soluble binders that offer potentially high value gain in cathode processing and address key concerns of anticipated PFAS-free material regulation. He will provide a realistic approach to investigation of the CO2 conversion mechanism and performance while addressing two key aspects of conversion: catalyst identity and CO2 binding.
Dr. Chen completed a PhD at the University of California, Los Angeles in 2012 under the supervision of Prof. Yunfeng Lu in the Department of Chemical and Biomolecular Engineering. His PhD work primarily related to the design and synthesis of nanostructured materials for electrochemical energy storage devices. From 2013 to 2016, he was a postdoctoral associate working with Prof. Zhenan Bao and Prof. Yi Cui at Stanford University, focusing on functional polymer materials for enhanced energy density, longer cycling lifetime, and improved safety of batteries.
The 2007 Talented Science Student Award was awarded to Dr. Chen by Tianjin University, where he received his BS in Chemical Engineering. His work has been recognized with the 2023 ECS Battery Division Early Career Award; 2018 NASA Early Career Faculty Award; 2018 ACS PRF Doctoral New Investigator Award; 2017 LG Chem BIC Award; 2012 UCLA Department Outstanding Graduate Award; 2011 MRS Graduate Student Silver Award; and 2011 Chinese Government Award for Outstanding Self-Financed PhD Students Studying Abroad. He was named an “Emerging Investigator” by Nanoscale (2021), Chemical Communication (2020), and Journal of Materials Chemistry C (2018). The organizer and chair of ECS meeting symposia, he has seven patents, is an associate editor of Battery Energy, and is author of three book chapters and 135 articles with an h-index is 58.
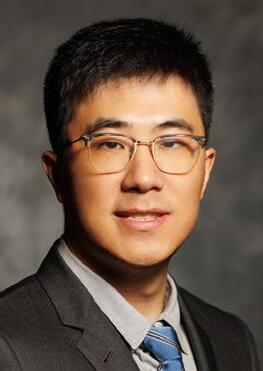
Zhongyang Wang
The University of Alabama
“Synthesis and Assessment of HydrocarbonBased Proton Exchange Materials for Enhancing Fuel Cell Technology”
Zhongyang Wang is an Assistant Professor of Chemical and Biological Engineering at The University of Alabama (UA). His research contributions include the investigation and deployment of bipolar membranes to advance the development of liquid-liquid fuel cells, and understanding mixed ionic/electronic transport in conjugated polyelectrolytes for renewable energy applications. Through the ECS Toyota Young Investigator Fellowship, Dr. Wang will advance the development of fuel cell technology by
addressing the precision synthesis of fully saturated hydrocarbonbased proton exchange membranes to replace long-standing Nafion ionomers.
Dr. Wang completed his PhD in Energy, Environmental, and Chemical Engineering at Washington University in St. Louis (WashU) in 2019 with advisor Vijay Ramani, followed by a postdoc co-advised by Paul Nealey and Shrayesh Patel at the University of Chicago (UChicago). His research has been recognized by the 2024 Hewson Faculty Fellowship of the Hewson Family Foundation and UA; 2022 Maria Lastra Excellence in Mentoring Award for Postdoctoral Researchers from UChicago; 2020 ECS Industrial Electrochemistry and Electrochemical Engineering Division H. H. Dow Memorial Student Achievement Award; and the 2020 Annual Research Impact Award from WashU. The author of 23 articles with an h-index of 17, he has three active and two pending US patents.
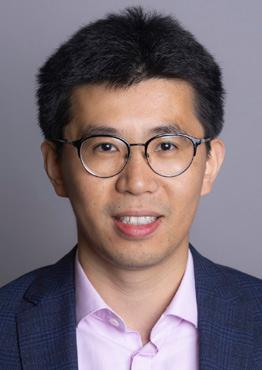
Juner Zhu
Northeastern University
“Mechano-Electrochemical Assessment of Spent Batteries for End-of-Life Decision-Making”
Juner Zhu is Assistant Professor of Mechanical and Industrial Engineering at Northeastern University (NU). The Zhu Group has two labs at NU, the Battery Sustainability Lab, and the Electro-Chemo-Mechanics Lab. His research contributions include safety-focused testing methods and models for EV batteries; multi-scale electro-chemo-mechanical characterization of battery materials and cells; and scientific machine learning (ML) algorithms for battery materials and systems. Through the ECS Toyota Young Investigator Fellowship, Dr. Zhu will investigate nondestructive techniques to identify common degradation modes in commercial Li-ion cells, presenting a quick and safe approach to endof-life cell defect identification, while also building a predictive ML library for future applications.
Before joining NU in August 2022, Dr. Zhu was a Research Scientist working jointly in the Departments of Mechanical Engineering and Chemical Engineering at the Massachusetts Institute of Technology (MIT). With Prof. Tomasz Wierzbicki as supervisor, he completed his PhD in Mechanical Engineering at MIT (2019) where he was a postdoc (2019–2021) and Research Scientist (2021–2022) under Prof. Wierzbicki and Prof. Martin Z. Bazant
Dr. Zhu is the author of 43 journal articles with an h-index of 27. He co-developed the 2020–2022 phase of the MIT Industrial Battery Consortium, acting as Executive Director working with eight world-leading companies in the areas of EV, battery, and consumer electronics. Dr. Zhu also worked as a materials engineer at Ford Motor Company and a battery analyst at Apple.
Dr. Zhu graduated with a BS and MS from Tsinghua University, where he received the 2015 Top Grade Fellowship, the highest honor for graduate students. He received the 2022 Haythornthwaite Foundation Research Initiation Grant by the Applied Mechanics Division (AMD) of the American Society of Mechanical Engineering (ASME). Dr. Zhu co-founded the Center for Battery Sustainability, a Northeastern and MIT joint research program with industry support in 2022, and in 2023, BattSafe Solutions LLC, where he is Chief Scientist.
(continued on next page)
(continued from previous page)
SOCIETY NEWS SOCIETY NEWS
2024–2025 ECS Toyota Young Investigators Fellowship Selection Committee
ECS gratefully acknowledges the service of the ECS Toyota Young Investigator Fellowship Selection Committee members who reviewed more than 160 proposals for the 2024–2025 program.
Toyota Research Institute of North America (TRINA)
• Timothy (Tim) Arthur, Senior Manager, Research Strategy Office, TRINA
• Masato Hozumi, Executive Engineer and Senior Manager, Materials Research Department, TRINA
• Charles (Chip) Roberts, Senior Research Manager, Materials Research Department, TRINA
• Gaohua Zhu, Senior Scientist, Materials Research Department, TRINA
• Liang Wang, Senior Scientist, Materials Research Department, TRINA
• Amy Prieto, Professor, Colorado State University
• Rana Mohtadi, Senior Principal Scientist, Materials Research Department, TRINA
• Nik Singh, Senior Scientist, Materials Research Department, TRINA
• Chen Ling, Senior Principal Scientist, Materials Research Department, TRINA
• John Muldoon, Senior Principal Scientist, Materials Research Department, TRINA
The Electrochemical Society
• John T. Vaughey, Senior Scientist, Argonne National Laboratory
• Joaquín Rodríguez-López, J. Andrew and Susan S. Langan Professorial Scholar, LAS Distinguished Professorial Scholar and Professor of Chemistry, University of Illinois UrbanaChampaign
ECS Toyota Young Investigator Fellowships
Fellows receive a $50,000 grant and one-year complimentary ECS membership. They are required to submit a midway progress report to ECS and, after one year of funding, a final written report. Within 24 months of the end of the research period, their findings are published open access in a relevant ECS journal and they deliver a presentation at an ECS meeting. TRINA invites the Fellows to present their research progress semiannually. After their fellowships end, Toyota may choose to enter into research agreements and continue collaborating with the Fellows.
The 2025–2026 ECS Toyota Fellowship cycle request for proposals opens in November 2024 with a submission deadline of January 31, 2025. Candidate interviews are scheduled for spring 2025 and Fellows’ names are announced in August 2025.
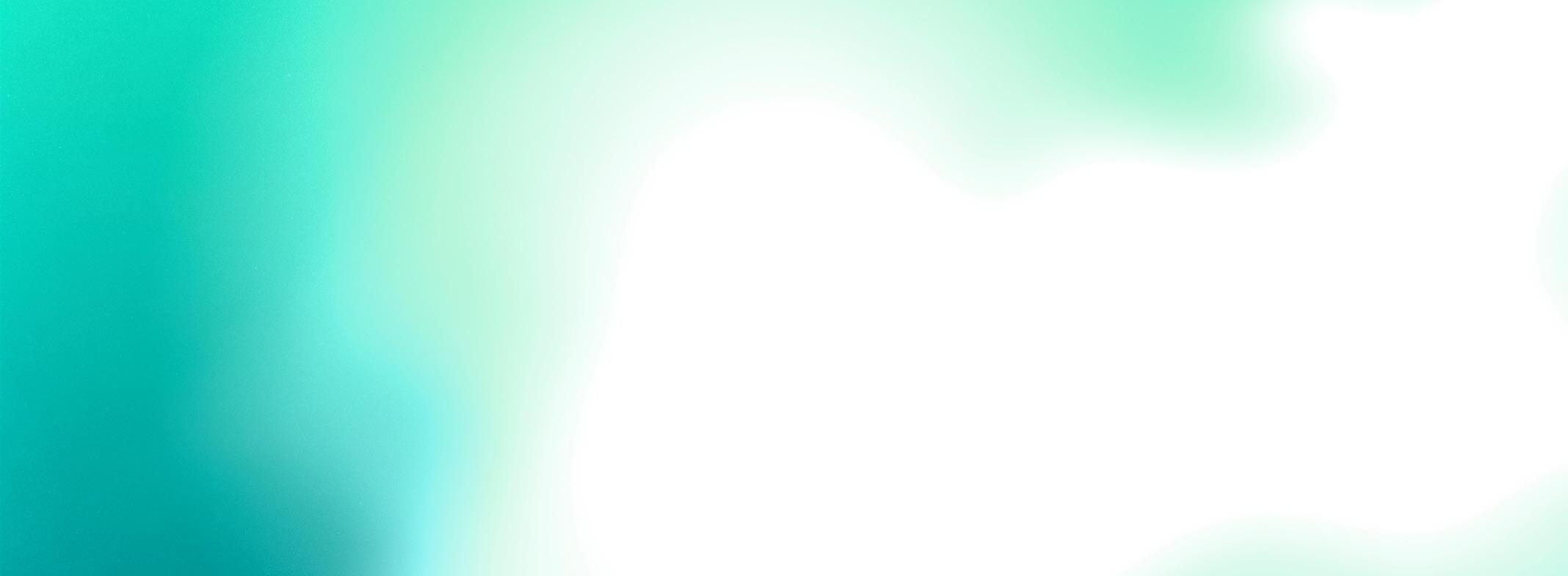
2024 Summer Fellowships
The following students received 2024 ECS Summer Fellowships, which fund students from June through August pursuing work of interest to the Society. Look for their fellowship reports in an upcoming Interface issue.
The Colin Garfield Fink Fellowship supports postgraduate researchers/engineers; ECS Summer Fellowships support students between the MS and PhD. Applicants must be ECS members enrolled in a college or university. ECS Summer Fellowship and Colin Garfield Fink Fellowship applications for 2025 are accepted from September 15, 2024 through January 15, 2025.
Colin Garfield Fink Fellowship
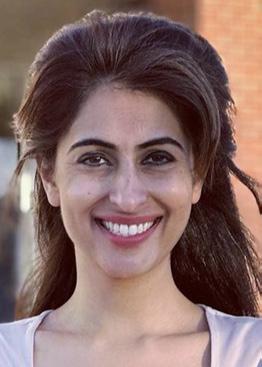
Maha Yusuf received the Colin Garfield Fink Fellowship for the postdoctoral research project, “In-situ Simultaneous Neutron and X-ray Tomography of Metal-Solid Electrolyte Interfaces in Anode-Free Solid-State Batteries.” She is a Presidential Postdoctoral Research Fellow in the Department of Mechanical and Aerospace Engineering and Andlinger Center for Energy and Environment at Princeton University. Her postdoc research combines her expertise in advanced neutron and X-ray–based characterization with physicsbased modeling and 3D manufacturing to engineer anodes for longlife lithium-metal–free solid state batteries.
Dr. Yusuf completed MS and PhD degrees in Chemical Engineering at Stanford University with support from the Schlumberger Faculty for the Future Fellowship and Diversifying Academia, Recruiting Excellence Fellowship. Her PhD research used advanced imaging diagnostic tools, particularly neutrons and X-rays, to understand the failure mechanisms of lithium-ion batteries during extreme fast charging (XFC).
Dr. Yusuf has received awards that include the 2024 ECS Energy Technology Division Graduate Student Award Sponsored by BioLogic, 2022 ECS Edward G. Weston Fellowship, 2022 American Chemical Society Chemical Abstracts Service Future Leader Award, and 2020 Stanford Distinguished Student Energy Lecturer Award. Dr. Yusuf completed a BE in Chemical Engineering at the National University of Sciences & Technology, Pakistan, after which she worked as a drilling engineer on oil and gas rigs in Colombia.
Edward G. Weston Fellowship
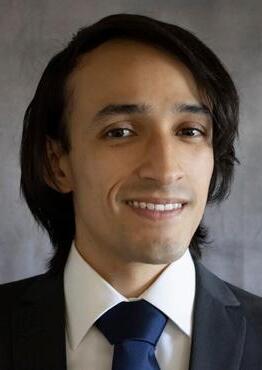
Raul A. Marquez will pursue “Understanding the Effects of Intermittent Water Electrolysis on Transition Metal Electrocatalysts” with support from the Edward G. Weston Fellowship. He is a fifth-year PhD student in Chemistry at the University of Texas at Austin. He completed his BE (2017) and MS (2020) at the Universidad Autónoma de Chihuahua.
Fellowships Raul has received include the UTAustin Provost’s Graduate Excellence Fellowship (2020–2025) and Mexican National Council of Science International Doctoral Fellowship Program (2021–2024), and his awards include the UT-Austin Jeff Byers Memorial Graduate Award (2024), Royal Society of Chemistry Poster Competition 1st Place (2024), and 243rd ECS Meeting General Student Poster Award 1st Place (2023). He is the author of 21 articles (10 as first author) with an h-index of 11. Raul is the Secretary of the ECS University of Texas at Austin Student Chapter.
Joseph W. Richards Fellowship
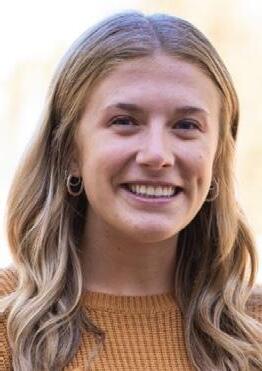
Courtney J. Weber’s Joseph W. Richards Fellowship project is titled “Development of an Electrochemical Aptamer-Based Sensor for the Continuous Detection of Stress Biomarkers.” She is a second-year PhD student in Chemistry at the University of South Carolina (USC) where she completed a BS in 2022. Courtney’s research, advised by Prof. Olja Simoska, focuses on fundamentally evaluating and implementing label strategies for analyte detection, based on using nucleic acid recognition elements at carbon ultramicroelectrode arrays, offering high reliability, enhanced sensitivity, lower limits of detection, reproducibility, and improved chemical specificity for stress biomarkers relevant to human health. She has received the USC Copenhaver Graduate Fellowship (2022), Southeastern Conference H. Boyd McWhorter Postgraduate Scholarship (2022), and the Joseph W. Bouknight Teaching Award (2022). Courtney has two published articles with two more under review.
F. M. Becket Fellowship
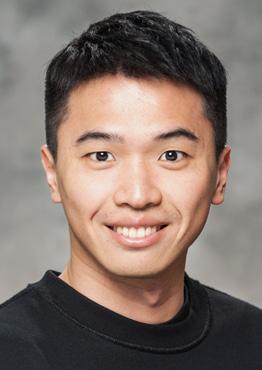
Po-Wei Huang will research “Investigating the Impact of Local Environment on Electrocatalytic Performance in a Bipolar Membrane-Based Membrane Electrode Assembly System” with support from the F. M. Becket Fellowship. He is a third-year PhD student in the Department of Chemical and Biomolecular Engineering at Georgia Institute of Technology. Professors Marta C. Hatzell and Andrew J. Medford supervise his research, which focuses on advancing sustainable technologies and developing decentralized fertilizers through electro/photochemical approaches. Po-Wei has received a Georgia Sea Grant Graduate Traineeship (2019), and he was selected as a Nakatani RIES Mentor (2022). The author of 11 publications (h-index 6), he has one patent pending. Po-Wei completed his BS in Chemical Engineering at National Cheng Kung University in 2017.
H. H. Uhlig Fellowship
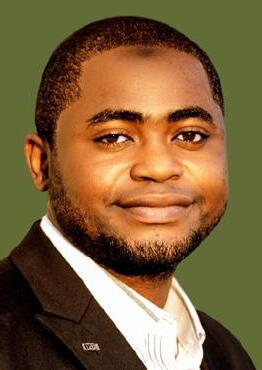
Ridwan Ayinla received the H. H. Uhlig Fellowship for his project, “Investigating the Critical Role of Cationic Species in BiomassBased All-Green Flexible Supercapacitors.” He is a PhD student in the Department of Sustainable Bioproducts at Mississippi State University under the supervision of Prof. El Barbary Hassan. His doctoral research focuses on synergizing electrochemical processes and imaging microscopy to develop and understand emerging green batteries and supercapacitors from biomass. His waste-towealth approach aligns the renewable energy transition initiative of the US Department of Energy with the clean environment goal of the US Department of Agriculture.
SOCIETY NEWS SOCIETY NEWS
The Nigerian-born researcher obtained his BT summa cum laude in Pure and Applied Physics at the Ladoke Akintola University of Technology. He completed an MS at the Universiti of Teknologi PETRONAS, then a second MS at Mississippi State University (MSU). Ridwan has published in several peer-reviewed journals and received local and international awards that include the Petroleum Development Trust Fund Scholarship, Nigerian Senate Kwara Class of Honors Award, Universiti Teknologi PETRONAS Graduate Award, and MSU Rising Star in Chemistry Award.
The Society thanks the 2024 Summer Fellowship Subcommittee members:
• Peter Mascher, Subcommittee Chair, McMaster University
• Oumaïma Gharbi, Centre national de la recherche scientifique (CNRS) – Sorbonne Université
• Junsoo Han, Sorbonne Université
• Joey Kish, McMaster University
• Patrik Schmutz, Eidgenössische Materialprüfungs-und Forschungsanstalt (EMPA)
• Kody Wolfe, Ohio University
Staff News
Meet ECS’s Section and Chapter Engagement Specialist
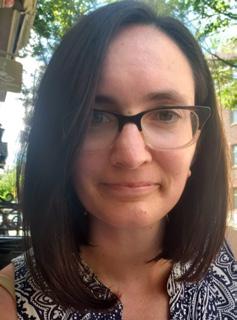
Maggie Hohenadel joined ECS in May as our new Section and Chapter Engagement Specialist. Maggie has many years of experience in association management, particularly with chapters and membership. Most recently, she managed the student chapter program at another STEM society. She will be working directly with the sections and student chapters to support and grow these programs. ECS Executive Director and CEO Chris Jannuzzi says, “Maggie brings a wealth of technical society chapter development experience to this position and will be a great complement to the Engagement group.”
Shannon Reed, ECS Senior Director of Engagement, adds “Maggie’s experience in managing 200 student chapters at her previous society is a great addition to the ECS team with our rapidly expanding student chapter program. I am confident in her ability to excel as a member of the team and provide the critical services to our grassroots leadership pipelines of sections and student chapter members.”
Maggie is excited to join ECS and is looking forward to getting to know the membership better and to the opportunity to further connect the electrochemistry community. In her free time, she enjoys reading (and always appreciates book recommendations) and taking art classes.
ECS Promotes Three Team Members for Outstanding Effort
On July 30, ECS Executive Director and CEO Chris Jannuzzi shared the news that John Lewis, Adrian Plummer, and Shannon Reed have received promotions in recognition of their outstanding work for the Society. Their new titles are:
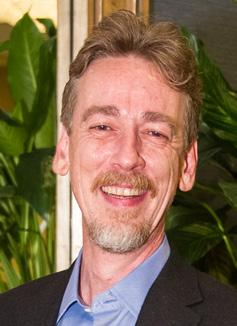
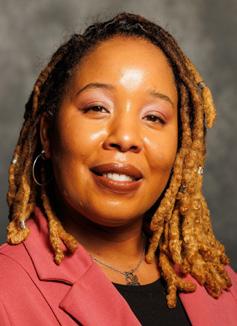
The term “community” was dropped from Shannon’s title to reflect the fact that Shannon and his department have internal as well as external engagement activities.
In his announcement of the promotions, Chris said, “I am exceedingly happy about these promotions. They are as well-deserved
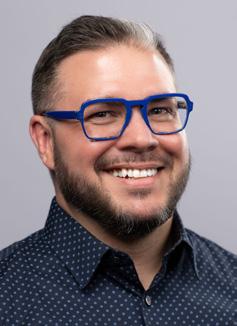
as any I have ever made. As individuals and as a leadership team John, Adrian, and Shannon have made enormous contributions to advancing the ECS mission. It is in recognition of these contributions that they have been promoted, so please join me in wishing John, Adrian, and Shannon all the best in their new positions.”
John Lewis Senior Director of Meetings
Adrian Plummer Senior Director of Publications
Shannon Reed Senior Director of Engagement
SOCIETY NEWS SOCIETY NEWS
ECS Division Contacts
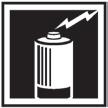
Battery
Brett Lucht, Chair
University of Rhode Island
Jie Xiao, Vice Chair
Jagjit Nanda, Secretary
Xiaolin Li, Treasurer
Doron Aurbach, Journals Editorial Board Representative
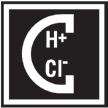
Corrosion
Dev Chidambaram, Chair
University of Nevada, Reno
Eiji Tada, Vice Chair
Rebecca Schaller, Secretary/Treasurer
Sannakaisa Virtanen, Journals Editorial Board Representative
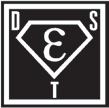
Dielectric Science and Technology
Sreeram Vaddiraju, Chair
Texas A&M University
Eva Kovacevic, Vice Chair
Zhi David Chen, Secretary
Thorsten Lill, Treasurer
Peter Mascher, Journals Editorial Board Representative
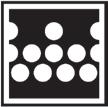
Electrodeposition
Luca Magagnin, Chair
Politecnico di Milano
Andreas Bund, Vice Chair
Rohan Akolkar, Secretary
Adriana Ispas, Treasurer
Takayuki Homma, Journals Editorial Board Representative
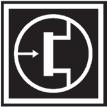
Electronics and Photonics
Qiliang Li, Chair
George Mason University
Vidhya Chakrapani, Vice Chair
Zia Karim, 2nd Vice Chair
Helmut Baumgart, Secretary
Travis Anderson, Treasurer
Khanna Aniruddh Jagdish, Journals Editorial Board Representative
Fan Ren, Journals Editorial Board Representative
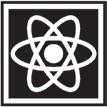
Energy Technology
Katherine Ayers, Chair
Nel Hydrogen
Minhua Shao, Vice Chair
Hui Xu, Secretary
Iryna Zenyuk, Treasurer
Minhua Shao, Journals Editorial Board Representative
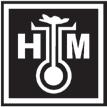
High-Temperature Energy, Materials, and Processes
Cortney Kreller, Chair
Los Alamos National Laboratory
Xingbo Liu, Vice Chair
Teruhisa Horita, Junior Vice Chair
Dong Ding, Secretary/Treasurer
Minhua Shao, Journals Editorial Board Representative
Industrial Electrochemistry and Electrochemical Engineering
Paul Kenis, Chair
University of Illinois at Urbana-Champaign
Elizabeth Biddinger, Vice Chair
Chockalingam Karuppaiah, Secretary/Treasurer
Paul Kenis, Journals Editorial Board Representative
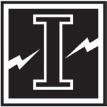
Luminescence and Display Materials
Eugeniusz Zych, Chair
Uniwersytet Wrocławski
Chong-Geng Ma, Vice Chair
Marco Bettinelli, Secretary/Treasurer
Won Bin Im, Journals Editorial Board Representative
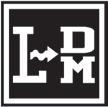
Nanocarbons
Jeff L. Blackburn, Chair
National Renewable Energy Laboratory
Ardemis Boghossian, Vice Chair
Yan Li, Secretary
Hiroshi Imahori, Treasurer
Dirk M. Guldi, Journals Editorial Board Representative
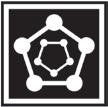
Organic and Biological Electrochemistry
Shelley Minteer, Chair
NSF Center for Synthetic Organic Electrochemistry
Jeffrey Halpern, 1st Vice Chair
Sabine Kuss, 2nd Vice Chair
Ariel Furst, Secretary/Treasurer
Janine Mauzeroll, Journals Editorial Board Representative
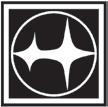
Physical and Analytical Electrochemistry
Stephen Paddison, Chair
University of Tennessee, Knoxville
Anne Co, Vice Chair
Svitlana Pylypenko, Secretary
Iwona Rutkowska, Treasurer
David Cliffel, Journals Editorial Board Representative
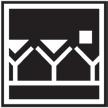
Sensor
Larry Nagahara, Chair
Johns Hopkins University
Praveen Kumar Sekhar, Vice Chair
Dong-Joo Kim, Secretary
Leyla Soleymani, Treasurer
Netz Arroyo, Journals Editorial Board Representative
Stefano Cinti, Journals Editorial Board Representative
ECS Industrial Electrochemistry and Electrochemical Engineering Division
At the 245th ECS meeting in San Francisco, the IE&EE Division conducted an outreach event for students from Cupertino High School and Alsion Montessori Middle and High School. The students learned the fundamentals of green hydrogen generation and its use in powering transportation. The highlight of the event was a race with model fuel cell cars using hydrogen generated from solar cells, built and raced by the students.
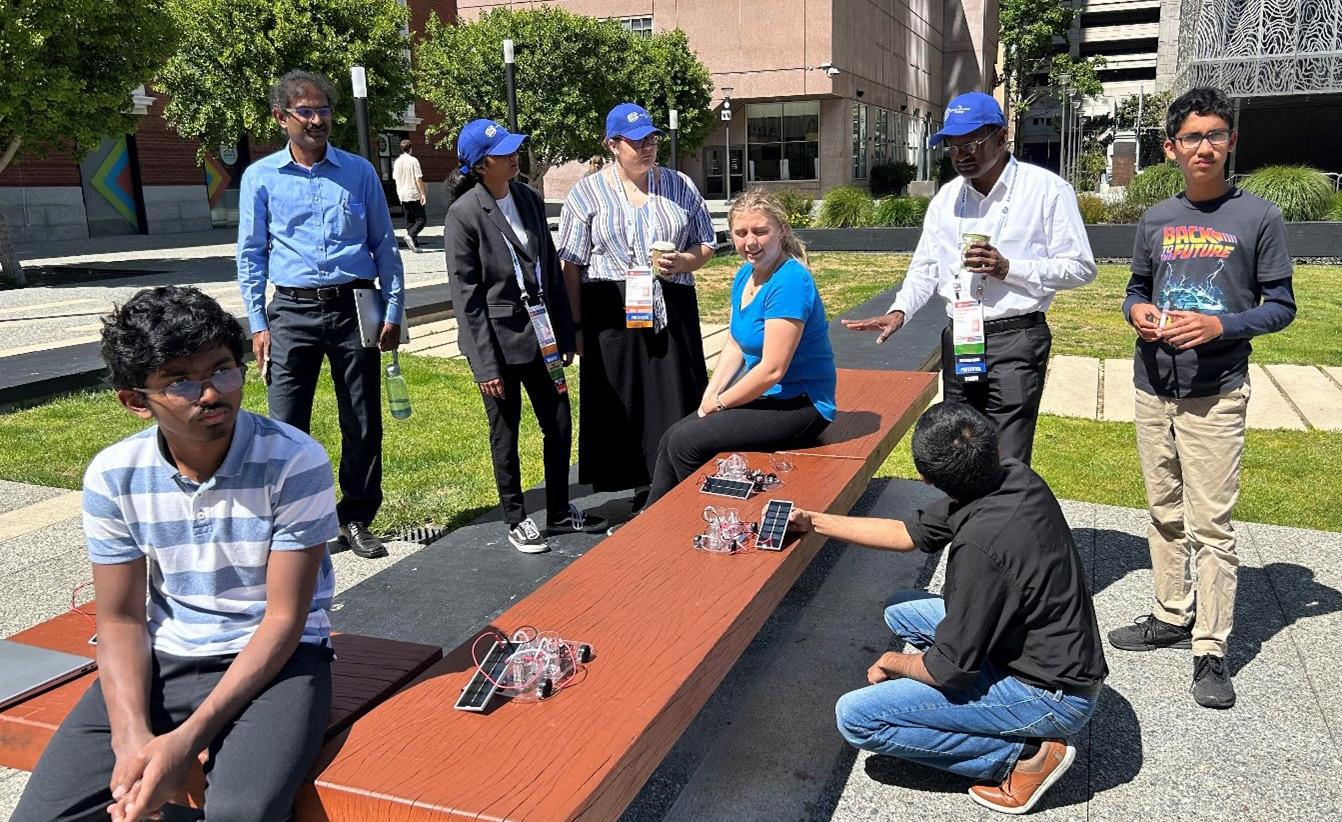
Creating Powerful Partnerships
ECS welcomes its newest institutional partner. The organization listed below is a great addition to the ECS Institutional Partnership Program.
R Initial Energy Science &Technology Co., Ltd (IEST) is a high-tech company focusing on R&D and production of lithium-ion battery testing instruments. With a multi-disciplinary research team covering from material science to physics, chemistry, electrochemistry, optics, machinery, electronics, computer science, and artificial intelligence, it is committed to providing leading testing solutions and services for the global new energy industry.
To learn more, visit the IEST website at www.iestbattery.com
NEXT ISSUE OF IN THE
The winter 2024 issue of Interface will feature the Dielectric Science and Technology Division (DS&T). Founded in 1945, the DS&T Division’s topical areas of interest (TIAs) encompass the theoretical and experimental aspects of inorganic and organic dielectric materials, including electrical, physical, optical, and chemical properties. The theme of the issue will be the future of semiconductor research, development, and manufacturing, and will cover topics that include:
Become an Institutional Partner
• Levels of partnership
• Institutional Partners
• Contact sponsorship@electrochem.org for more information.
• Perspectives on workforce training for chip manufacturing
• Novel strategies for chip design and a look at their future
You will also find highlights from PRiME 2024, the latest from the ECS President, reports from summer fellows, features favorites like EChem Education, Tech Highlights, and Looking at Patent Law, and the latest news about people, students, and the Society.
Chockkalingam (Chock) Karuppaiah (second from the left) and the ECS IE&EE Division built model fuel cell cars using hydrogen generated from solar cells with middle and high school students at the 245th ECS Meeting in San Francisco.
Websites of Note
Selected for you
by Alice H. Suroviec
Software Carpentry
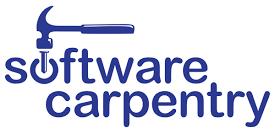
Software Carpentry has a large library of lesson materials available for researchers who would like to learn how do basic research computing. Data collection and analysis is a critical part of science, but most scientists are never taught how to build, use, and validate software well. Software Carpentry’s stated goal is to teach those skills with free lessons so scientists can spend less time wrestling with software and more time doing useful research. Their core topics include structured programming in Python, R, and MATLAB.
https://software-carpentry.org
Battery Fire Safety

The UL Fire Safety Research Institute advances fire safety knowledge to address a variety of risks and emerging dangers. Although fires involving lithium-ion battery–powered products are rare, this website provides a variety of resources to learn about Li-ion safety, thermal runaway, and how to manage Li-ion fires.
https://batteryfiresafety.org
Battery University
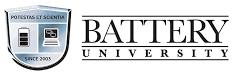
Battery University™ is a free educational website that offers battery information to engineers, educators, media, students, and battery users alike. Their tutorials evaluate the advantages and limitations of battery chemistries, advise on best battery choice, and suggest ways to extend battery life. The information is compiled from specifications and independent test laboratories as well as crowdsourcing.
https://software-carpentry.org/
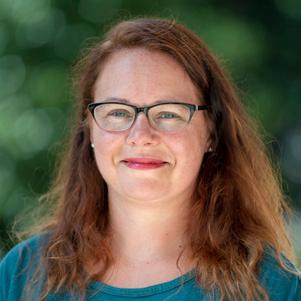
About the Author
Alice Suroviec is a Professor of Bioanalytical Chemistry and Dean of the School of Mathematical and Natural Sciences at Berry College. She earned a BS in Chemistry from Allegheny College in 2000. She received her PhD from Virginia Tech in 2005 under the direction of Dr. Mark R. Anderson. Her research focuses on enzymatically modified electrodes for use as biosensors. She is a Fellow of the Electrochemical Society and Associate Editor of the PAE Technical Division for the Journal of the Electrochemical Society. She welcomes feedback from the ECS community.


https://orcid.org/0000-0002-9252-2468
UPCOMING ECS SPONSORED MEETINGS
ECS and its divisions and sections sponsor meetings and symposia of interest to the technical audience ECS serves, as well as ECS biannual meetings and ECS satellite conferences. A partial list of upcoming sponsored meetings follows. Consult the ECS website for a complete list.
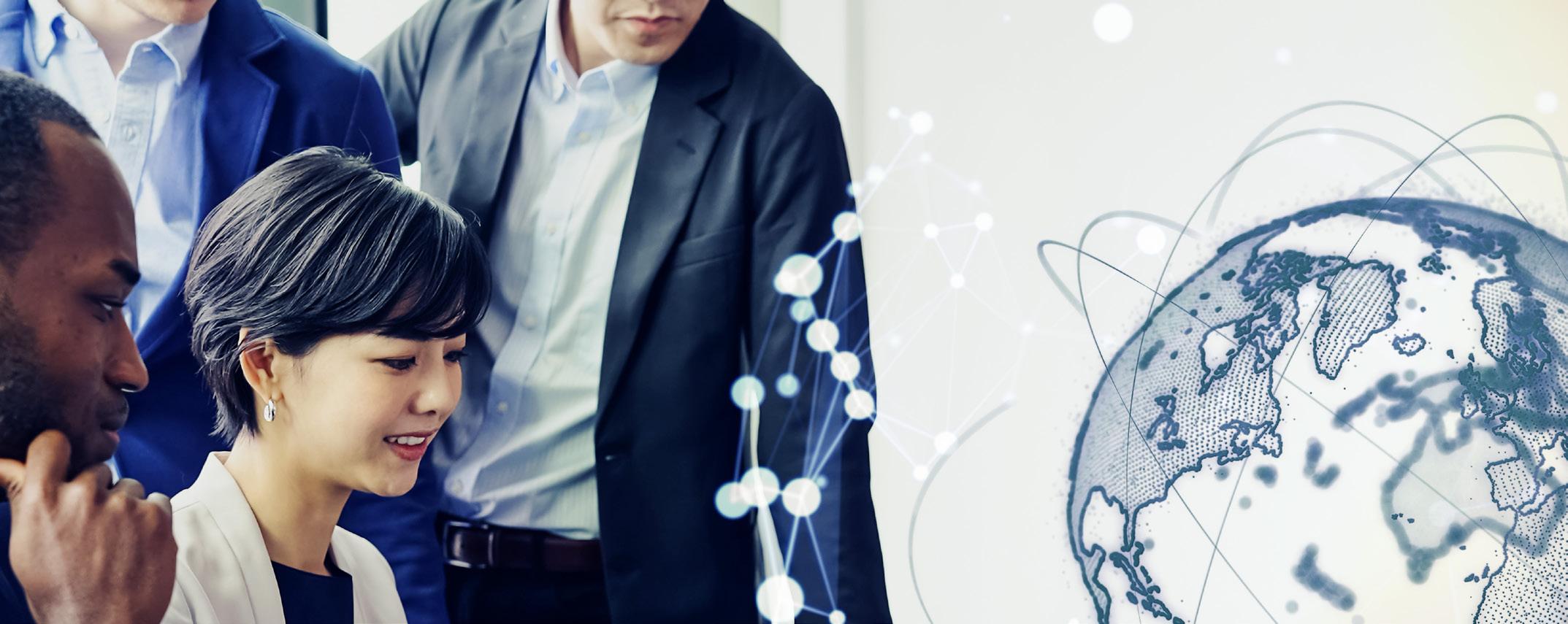
2025
19th International Symposium on Solid Oxide Fuel Cells (SOFC-XIX)
July 13–18, 2025 | Stockholm, Sweden
The Brewery Conference Center
To request ECS sponsorship of your technical event or to learn what ECS sponsorship can do for your meeting, contact ecs@electrochem.org.
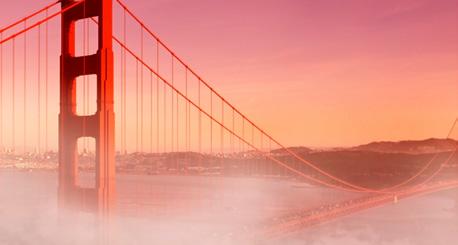
FHighlights of the 245th ECS Meeting
SAN FRANCISCO, CA, USA • May 26–30, 2024
rom May 26 to 30, 2024, the 245th ECS Meeting convened in San Francisco, with more than 2,829 attendees plus 33 companions representing 68 countries—making it one of ECS’s largest spring meetings. The meeting encompassed 49 symposia with 439 sessions. A total of 3,022 abstracts were accepted with 2,294 oral talks, 701 posters, and 27 digital presentations. Of these, student abstracts numbered 1,240 (842 presented orally and 388 posters). The meeting featured 575 invited talks and 25 ECS award and keynote talks.
San Francisco is home to the Golden Gate Bridge, the perfect symbol for the meeting, as ECS is the bridge uniting meeting participants with electrochemists and solid state scientists from around the world, all gathering in one place to connect, share ideas, and build a better world through their science.
ECS Member Reception
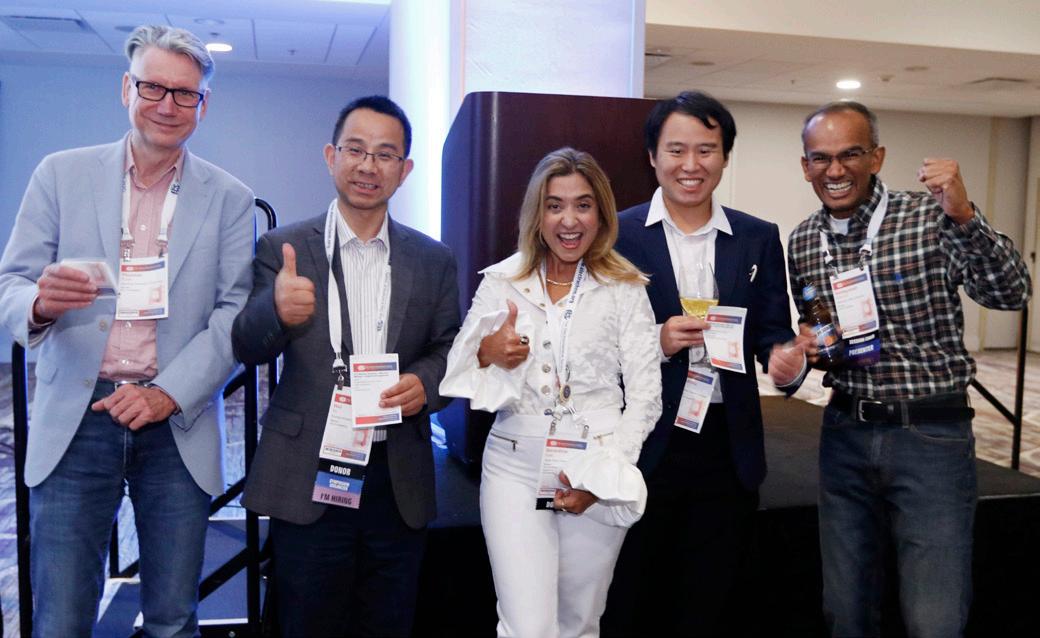
More than 400 ECS members came together for the ECS Member Reception. The sold-out event preceding the Opening Reception brought together colleagues old and new for networking, hanging out, and enjoying a raffle, food, and open bar. These especially lucky ECS members received prize giveaways as part of attending the event:
• 5-night Hotel Stay, 247th ECS Meeting, Montréal, Canada: Sadagopan Krishnan, Oklahoma State University
• Lifetime Membership: Simiao Niu, Rutgers University
• PRiME 2024 Meeting Registration: Thomas Turek, Technische Universität Clausthal
• $250 Amazon Gift Card: Ching-Hsiu Chung, University of Illinois Urbana-Champaign
• $250 Amazon Gift Card: Hui Xu, Envision Energy USA
Opening Reception
The 245th ECS Meeting Opening Reception was a wonderful opportunity to reconnect and forge new connections at the outset of the meeting. Almost 1,000 attendees enjoyed food and an open bar. Expert instructors led merengue dance classes, making the event even livelier!
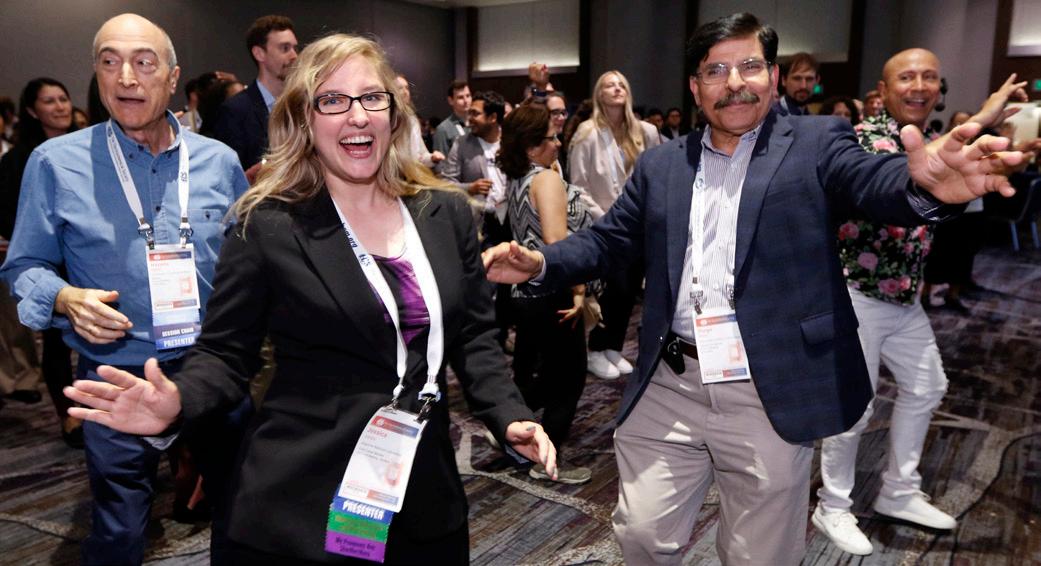
ECS Awards and Recognition Ceremony
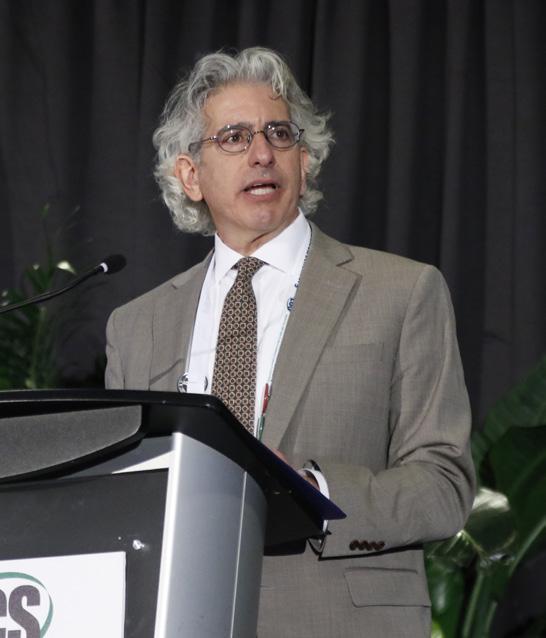
ECS Executive Director and CEO Christopher J. Jannuzzi delivered the meeting’s opening remarks. He welcomed event participants attending in person and via live video stream to the 245th ECS Meeting in San Francisco. Chris gave an overview of the week ahead and thanked general meeting sponsors, symposia sponsors, exhibitors, meeting attendees, digital participants, volunteers, and staff. He noted the critical support provided by ECS divisions and sections through their travel funding programs for students and early career attendees.
Chris stressed Accelerate, Champion, Engage, and Empower— keywords describing the Society as its mission moves forward to advance theory and practice at the forefront of solid state science and technology, and allied subjects. He announced that membership was exceptionally strong with 8,875 members, well beyond ECS’s pre-pandemic membership levels. Student membership is the largest it has ever been, with 2,690 student members. In 2023, ECS published 1,783 journal articles with almost 10 million downloads. ECS accelerates scientific discoveries on many fronts, including launching a new training program to upskill workers in new battery technologies. Chris thanked meeting sponsors and welcomed ECS’s Institutional Partners. He also noted the importance of support from ECS’s sections and divisions.
Chris introduced ECS President Gerardine (Gerri) Botte who took the floor, welcoming attendees and live-streaming participants,
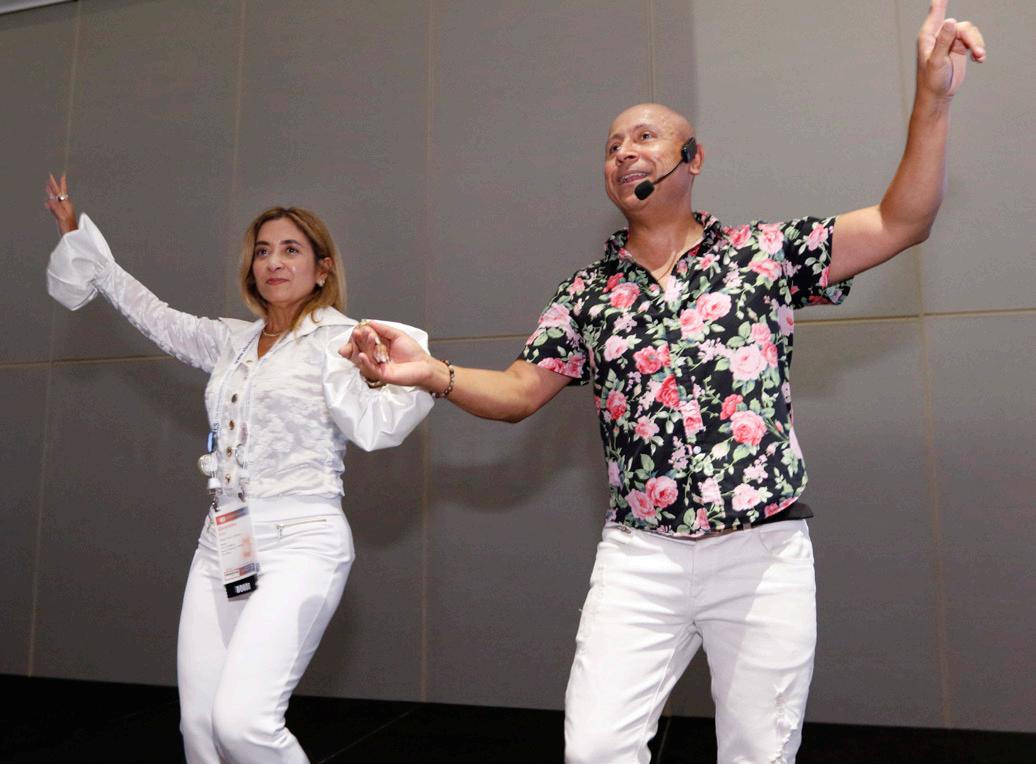
Attendees took merengue classes with President Gerri Botte, dancing the night away at the Opening Reception
Raffle winners celebrate with ECS President Gerardine (Gerri) Botte
From left to right: Thomas Turek, Hui Xu, Gerardine Botte, Ching-Hsiu Chung, and Sadagopan Krishnan All meeting photos: PaulSakuma.com Photography
ECS Executive Director and CEO Christopher J. Jannuzzi addresses attendees at the ECS Awards and Recognition event.
and thanking meeting supporters, attendees, and staff. She described the progress on major initiatives launched during her presidency: the ECS Presidential Ad Hoc Committee on Sustainability to CHAMPION the research and technologies needed for a sustainable future, and the Presidential Ad Hoc Committee on Diversity, Equity, Inclusion, and Engagement—a bold initiative to ENGAGE in a diverse and inclusive community. Before handing over the reins to the new president, she thanked the members of the ECS Board of Directors for their close collaboration and support.
Gerri opened the Awards and Recognition Ceremony celebrating the EMPOWERING accomplishments of community members who advance the Society’s mission. She congratulated division and section award winners and encouraged attendance at their award talks during the meeting.
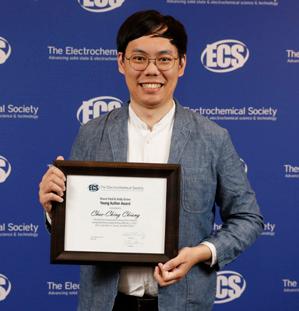
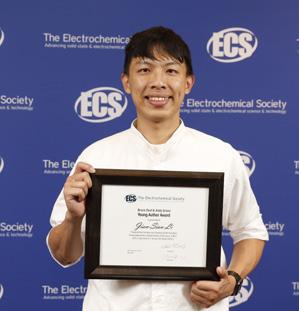
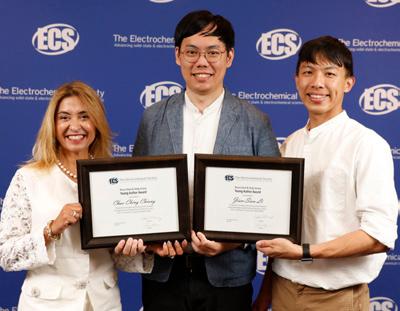
The ECS Young Author Awards are given annually to young authors for the best papers published in the Journal of The Electrochemical Society (JES) and the Journal of Solid State Science and Technology (JSS) by a young author or co-authors in the preceding volume year. Gerri congratulated Chao-Ching Chiang and Jian-Sian Li of the University of Florida for winning the Bruce Deal & Andy Grove Young Author Award for the best paper published in JSS, “Threshold Ion Energies and Cleaning of Etch Residues During Inductively Coupled Etching of NiO/Ga2O3 in BCI3.”
Gerri introduced incoming ECS President Colm O’Dwyer, who presented the 2024 Leadership Circle Awards recognizing and thanking our Institutional Partners in the year that member institutions reach significant anniversary milestones. Colm thanked these institutions for their continued support and engagement:
• EL-CELL GmbH for 10 years of institutional partnership. Sebastian Müller and Bernhard Bugenhagen accepted the award on behalf of EL-CELL.
• Ford Motor Corporation for 10 years of institutional partnership. Brian Roberts accepted the award on behalf of Ford.
• Ion Power, Inc. for 10 years of institutional partnership. Stephen, Wendy, and Winifred Grot accepted the award on behalf of Ion Power.
• Metrohm USA, Inc. for 10 years of institutional partnership. Hobbie Turley accepted the award on behalf of Metrohm.
• Pacific Northwest National Laboratory for five years of institutional partnership. Dr. Jie Xiao accepted the award on behalf of PNNL.
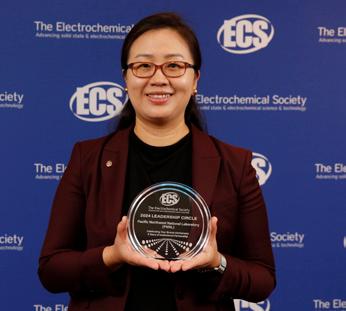
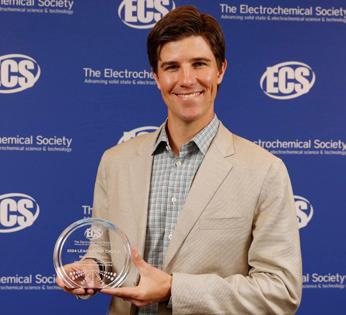
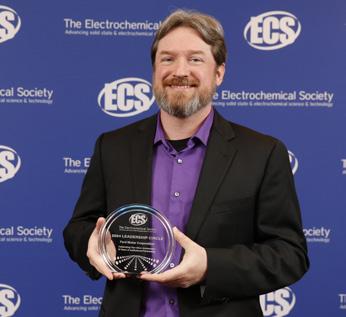
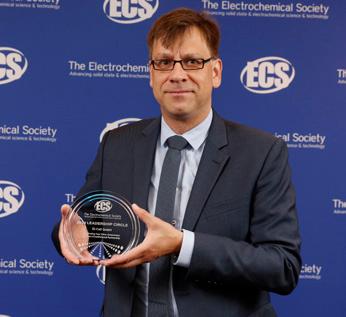
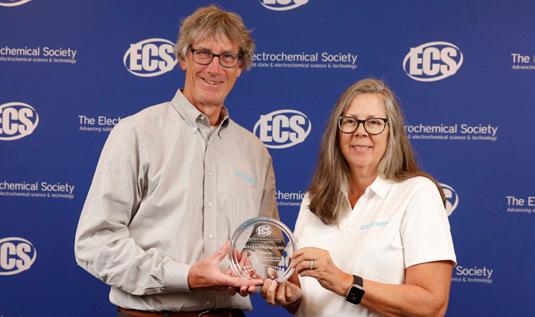
Colm thanked and recognized the past ECS division chairs whose two-year terms ended recently, for their oversight of the divisions and stewardship at the Society level by serving on the Board of Directors:
• Sean Bishop, Past Chair, H-TEMP Division, 2021–2023
• Vimal Chaitanya, Past Chair, Dielectric Science and Technology Division, 2018–2020
• Ajit Khosla, Past Chair, Sensor Division, 2018–2020
• William Mustain, Past Chair, Energy Technology Division, 2021–2023
Colm introduced members of the Class of 2023 Fellows of The Electrochemical Society who had not been acknowledged at prior meetings:
• Martin Bazant, Massachusetts Institute of Technology, for contributions to electrochemical physics and engineering.
• Ajit Khosla, Xidian University and Yamagata University, for his seminal contributions to, and international leadership of, sensor science and technology.
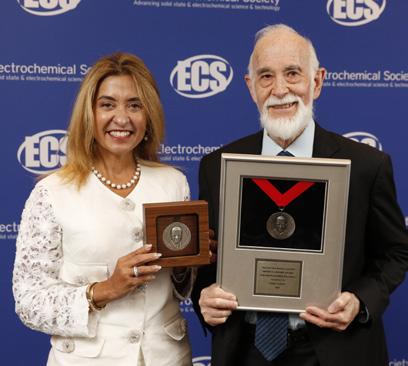
Gerri then returned to the floor to introduce the ECS Society Award winners. Prof. Uziel Landau, Case Western Reserve University, received the 2024 Henry B. Linford Award for Distinguished Teaching in recognition of four decades of excellence in teaching and inspiring students about the fundamentals of electrochemical engineering and the application of those fundamentals to solving industryrelevant electrochemical technology problems. The award, which recognizes excellence in teaching in subject areas of interest to the Society, was established in 1981 in honor of Henry B. Linford, Distinguished Professor of Chemical Engineering at Columbia University, known for his work and research in electroplating and corrosion of metals. With a Society history dating back to 1936, Dr. Linford served as ECS Secretary for 10 years and President from 1961 to 1962.
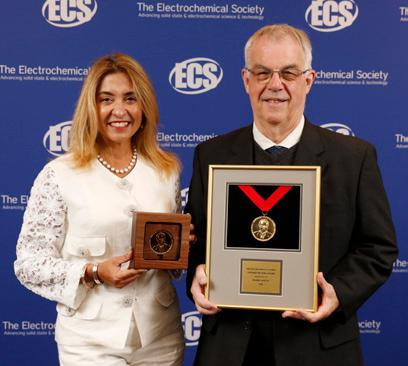
Prof. Landau’s award talk, “Overcoming Challenges in Teaching Electrochemical Engineering,” described the difficulties in creating a curriculum addressing the multi-disciplinarity of electrochemistry, which requires some knowledge of chemistry, material science, transport, kinetics, and process design. Electrochemical engineering courses typically draw students from different disciplines (e.g., chemical engineering, chemistry, material science, biomedical engineering) with different backgrounds and preparation. Practicing engineers attending electrochemical engineering short courses typically have different backgrounds, experience levels, and interests. The course Prof. Landau developed started with ionic conduction and transport, then moved on to electrochemical thermodynamics, overpotentials, current distributions, multi-components and alloy deposition, transient effects, and scaling. One class was devoted to discussing batteries and fuel-cells, electroplating, electrowinning and electrorefining, electrolytic processes, and corrosion. Discussing the fundamentals, issues, and major challenges for each was emphasized. Comprehensive case studies fostered critical thinking and discussion, and integrated the material taught. Prof. Landau acknowledged his debt to his mentor Charles Tobias and thanked his students and his friends and colleagues at Case Western Reserve University.
Prof. Daniel Lincot, Chimie ParisTech – PSL, was awarded the 2024 Vittorio de Nora Award
(continued on next page)
President Botte presents the Bruce Deal & Andy Grove Young Author Award to Ching Chiang and Jian-Sian Li, University of Florida.
2024 Leadership Circle Award winners
Uziel Landau, Case Western Reserve University, accepts the 2024 Henry B. Linford Award for Distinguished Teaching from ECS President Botte
Daniel Lincot, Chimie ParisTech – PSL, accepts the 2024 Vittorio de Nora Award from President Botte
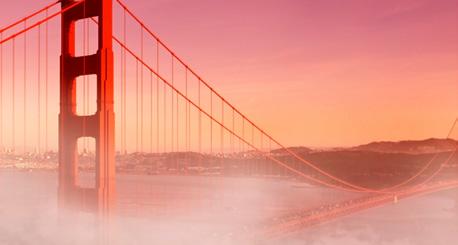
(continued from previous page)
for his distinguished contributions to the field of semiconductor electrochemistry and industrial-scale implementation in thinfilm solar cells and breakthrough innovations in electrodeposited cadmium telluride (CdTe), copper indium gallium di-selenide, chemical deposition of cadmium sulfide, and electrodeposition of zinc oxide window layers. Established in 1971, the award recognizes distinguished contributions to the field of electrochemical engineering and technology. It is named in honor of Vittorio de Nora, the renowned electrochemistry researcher known internationally for his many contributions to industry, particularly in the manufacture of chlorine and development of dimensionally stable electrodes which revolutionized the electrochemical and electrometallurgical industries.
Prof. Lincot’s award talk, “When Solid State Semiconductor Physics and Devices Meet Photoelectrochemistry: From Growth and Interfacial Processes to Photovoltaics,” explained how his research on photovoltaic solar energy since 1978 has led to innovations in the field of thin-film processes and materials. His vision of the fundamental and underestimated interest of solution growth methods (electrochemistry or CBD) for the development of high-quality functional materials, particularly for photovoltaic conversion, guided his entire scientific approach to date. In 1980, he discovered electrochemical deposition of CdTe, simplifying the process and leading to significant findings, including the synthesis of a new phase, CdTe2, and epitaxial growth. This work expanded into electrochemical synthesis of other semiconductors, such as ZnO and CuInGaSe2 (CIGS), contributing to advances in photovoltaics and leading to the creation of a dedicated laboratory and successful start-ups. His ongoing research emphasizes the importance of solution growth methods, which has been validated by the success of halogenated perovskite cells.
President Botte concluded the ECS Awards and Recognition Ceremony by recognizing ECS members celebrating milestone anniversaries as part of the ECS community. Their names appeared on the Ribbon Wall in the Registration area.
ECS Plenary Lecture
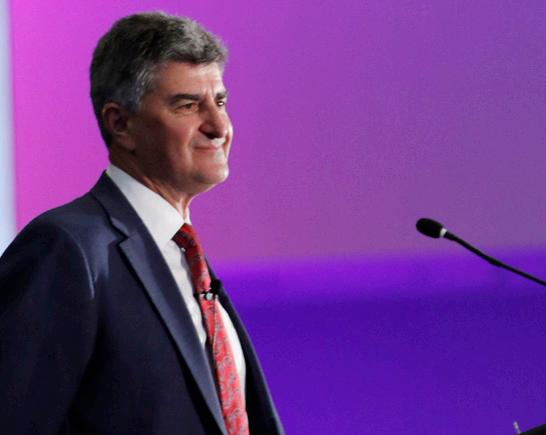
University,
Gerri introduced and highlighted the career accomplishments of Yury Gogotsi, who presented the ECS Lecture, a highlight of all ECS meetings. He is the distinguished University Professor and Charles T. and Ruth M. Bach Endowed Chair in the Department of Materials Science and Engineering at Drexel University. He also serves as Director of the A. J. Drexel Nanomaterials Institute.
Together with his students and colleagues, Prof. Gogotsi has made principal contributions to the development of materials for electrochemical capacitors and other energy storage devices; discovered MXenes and polygonal nanotubes (graphite polyhedral crystals); demonstrated the tuning of structure and porosity of carbide-derived carbons; and developed new processes for the synthesis, surface modification, and purification of nanotubes and nanodiamonds. He received his MS (1984) and PhD (1986) from Kyiv Polytechnic Institute and a DSc from the National Academy of Sciences of Ukraine in 1995. A Fellow of The Electrochemistry Society, Prof. Gogotsi’s h-index is over 200, and he has been recognized as a Highly Cited Researcher in Materials Science and Chemistry and a Citations Laureate in Physics by Clarivate Analytics (Web of Science).
In “What MXenes Can Do for Efficient Generation, Storage, and Conversion of Electrical Energy,” Prof. Gogotsi described the carbides and nitrides of transition metals known as MXenes, the fastest-growing family of 2D materials and probably the largest family of inorganic materials discovered in the 21st century. The versatile chemistry of the MXene family renders their properties tunable for a large variety of practical applications such as redox energy storage, especially when high rate and power are needed. This, along with other advantageous properties, makes the MXene family promising candidates for energy storage and related electrochemical applications. MXenes can also function as conductive supports for noble metal and other catalysts due to their unique and beneficial catalytic and electrocatalytic properties. Electrochemical and ionotronic control of the physical properties of MXenes allows the development of electrochemical actuators, modulation of infrared and microwave shielding, and many other innovative applications. Their use in plasmonics, electrochromic coatings, electrochemical sensors, epidermal and implantable electronics, water purification and desalination, and other fields, is equally exciting. This large and quickly growing family of 2D materials has the potential to impact many fields, which are just beginning to be understood and explored. Many important discoveries are expected in the future, ranging from the synthesis of MXenes that have not yet been reported and verifying their theoretically predicted properties, to exploring new applications.
Francis D’Souza, ECS 3rd Vice President, moderated the ensuing question and answer period with Prof. Gogotsi. Chris Jannuzzi concluded the session by encouraging attendees to visit the Exhibit Hall and participate actively in the 245th ECS Meeting and to join ECS for PRiME 2024 in Honolulu in October 2024.
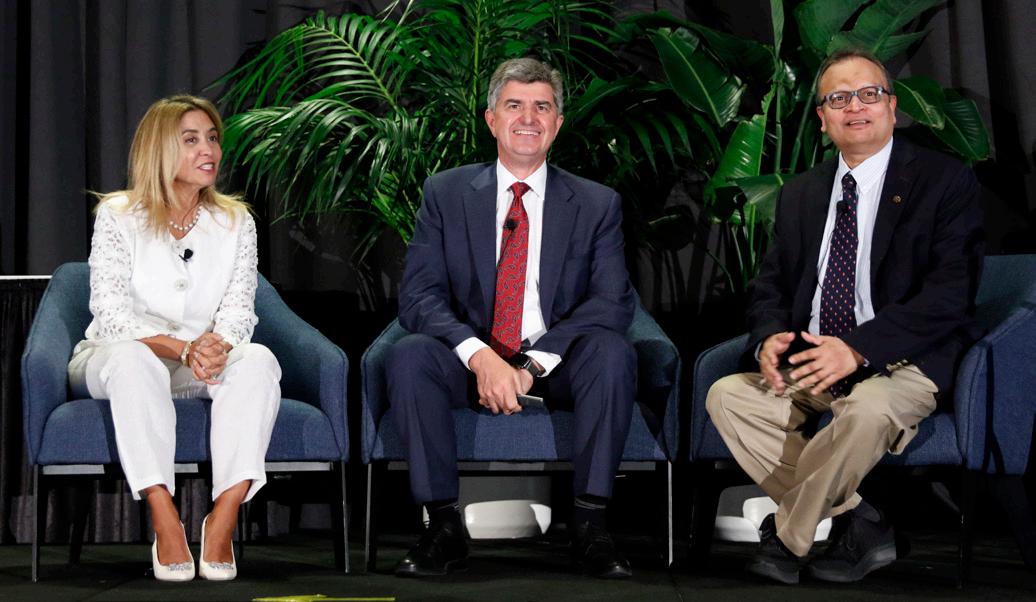
Division Awards
During the meeting, 12 division awards (including four student awards) were presented. Learn more about the award winners in the online meeting program.
• Battery Division Postdoctoral Associate Research Award
Sponsored by MTI Corporation and the Jiang Family Foundation: Xiang Gao, University of North Carolina at Charlotte
• Dielectric Science & Technology Thomas D. Callinan Award: Peter Mascher, McMaster University
• Electronics and Photonics Division Award: Travis Anderson, US Naval Research Laboratory
• Energy Technology Division Graduate Student Award
Sponsored by BioLogic: Noor Ul Hassan, National Renewable Energy Laboratory (NREL), Chemistry and Nanoscience Center
• Energy Technology Division Graduate Student Award
Sponsored by BioLogic: Maha Yusuf, Princeton University
• Energy Technology Division Research Award: William Mustain, University of South Carolina
ECS Lecture speaker Yury Gogotsi, A. J. Drexel Nanomaterials Institute and Department of Materials Science and Engineering, Drexel
takes the stage.
Francis D’Souza, ECS 3rd Vice President, moderates the Q&A session with the ECS Lecture speaker Yury Gogotsi, alongside ECS President Botte
• Energy Technology Division Supramaniam Srinivasan Young Investigator Award: Nikolay Kornienko, Institut für Anorganische Chemie, Universität Bonn
• Industrial Electrochemistry and Electrochemical Engineering Division H. H. Dow Memorial Student Achievement Award: Yirui Zhang, Massachusetts Institute of Technology
• Nanocarbons Division Richard E. Smalley Research Award: R. Bruce Weisman, Rice University
• Nanocarbons Division SES Young Investigator Award: Delphine Bouilly, Université de Montréal
• Sensor Division Early Career Award: Stefano Cinti, Università degli Studi di Napoli Federico II
• Sensor Division Student Research Award: Yaoli Zhao, University at Buffalo
245th Z01—General Student Poster Session
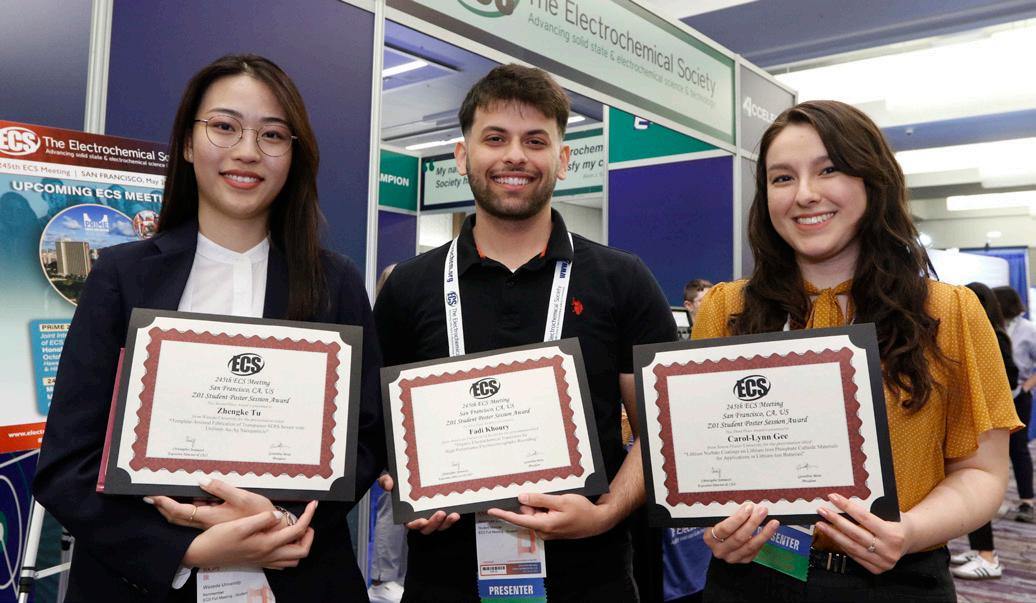
More than 200 posters were submitted to the General Student Poster Session. The session’s award winners are:
1st Prize: $1,500 award
Fadi Khoury, American University of Beirut, “Organic Electrochemical Transistors for High-Performance Electrocorticography Recording”
2nd Prize: $1,000 award
Zhengke Tu, Waseda University, “Template-Assisted Fabrication of Transparent SERS Sensor with Uniform Au-Ag Nanoparticle Electrodeposition”
3rd Prize: $500 award
Carol-Lynn Gee, Simon Fraser University, “Lithium Niobate Coatings on Lithium Iron Phosphate Cathode Materials for Applications in Lithium-Ion Batteries”
ECS thanks the Society members who served as reviewers for the 245th ECS Meeting Z01 General Student Poster Session.
In-person judges:
• Damilola A. Daramola, Northeastern University
• David Hall, Universitetet i Stavanger
• Adam Lovett, University College London
• Maureen Tang, Drexel University
• Roseanne Warren, University of Utah
Virtual judges:
• Sarah Berlinger, Lawrence Berkeley National Lab
• Marco Bettinelli, Università degli Studi di Verona
• Alanah Fitch, Loyola University Chicago
• Ellie Honarvar, Ford Motor Company
• Maria Inman, Faraday Technology Inc.
• Ahamed Ishrad, Stanford University
• Luca Magagnin, Politecnico di Milano
• Christian Malapit, Northwestern University
• Milad Navaei, Georgia Institute of Technology
• Joseph Quinn, Pacific Northwest National Lab
• Leah Rynearson, University of Rhode Island
• Neelakandan Santhosh, Jožef Stefan Institute
• Eiji Tada, Tokyo Institute of Technology
• Sreeram Vaddiraju, Texas A&M University
• Yasushi Katayama, Keio University
ECS thanks Alice Suroviec for organizing the Z01 General Student Poster Awards symposium.
Symposia Best Presentation and Poster Awards
Several 245th ECS Meeting symposia presented muchappreciated awards for best posters, presentations, and papers. The symposia organizers thank the sponsors who generously supported these awards.
A05—Battery Student Slam 8
Poster Award: $250 award Danwon Lee, Seoul National University
Poster Award: $250 award Jessica Ruby Gallawa, Colorado State University
Poster Award: $250 award Mengchen Liu, University of California San Diego
Poster Award: $250 award Peng Wang, Florida State University
Poster Award: $250 award Toshan Wickramanayake, Queen Mary University of London
B01-B09—Carbon Nanostructures and Devices
1st Place: $300 award Arslan Umer, The University of Texas at Dallas
2nd Place: $200 award Tanisha Davidson, Purdue University Fort Wayne
2nd Place: $200 award Jaina Sarris Wollowitz, Weill Cornell Medicine, Cornell University
3rd Place: $140 award Asmaa Heiba, The American University in Cairo
3rd Place: $140 award Nemanja Gavrilov, University of Belgrade
3rd Place: $100 award Hannah Dewey, North Carolina State University
D01—Chemical Mechanical Polishing 17
Student Best Paper – Closing Session
1st Place: $150 award A. Sadri Mofakham, Clarkson University
1st Place: $150 award K. Park, Sungkyunkwan University
2nd Place: $100 award E. M. McDonnell, Lewis University
2nd Place: $100 award P. M. Smith, Lewis University
3rd Place: $100 award Manhyup Han, Hanyang University
H01—Wide-Bandgap Semiconductor Materials and Devices 25
Poster Winner: $125 award
Student Presentation Award: $125 award
Student Presentation Award: $125 award
Student Presentation Award: $125 award
Nuwayyir Alshammari, George Mason University
Hsiao-Hsuan Wan, University of Florida
Hsiao-Hsuan Wan, University of Florida
Jian-Sian Li, University of Florida
Linhai Guo, Chinese Academy of Sciences
(continued on next page)
245th Z01 General Student Poster Session Award Winners (from left to right) Zhengke Tu, Fadi Khoury, and Carol-Lynn Gee show off their certificates.
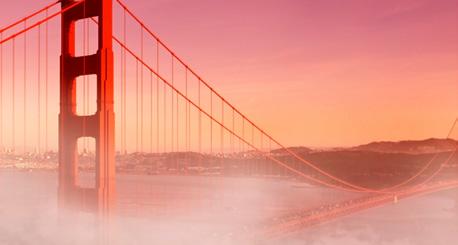
(continued from previous page)
L01—Physical and Analytical Electrochemistry, Electrocatalysis, and Photoelectrochemistry
General Session Award:
$750 award
General Session Award:
$750 award
General Session Award:
$750 award
Juliette Pelletier, École Polytechnique
Montréal
Nathanael Ramos, University of Colorado
Amira Ben Abderrahmane, Institut Européen des Membranes
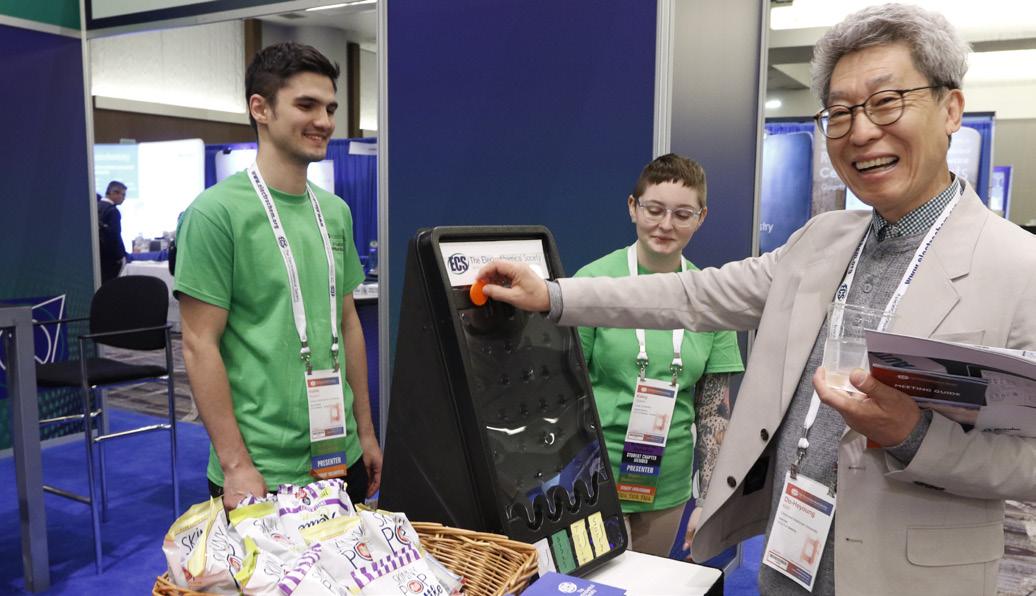
Lucky visitors to the ECS Exhibit Booth won raffle prizes:
• Complimentary ECS Life Membership: Leon Wickert, RuhrUniversität Bochum
• Free ECS Meeting Registration: Yin-Chen Lin, University of California, Santa Barbara
Student Mixer
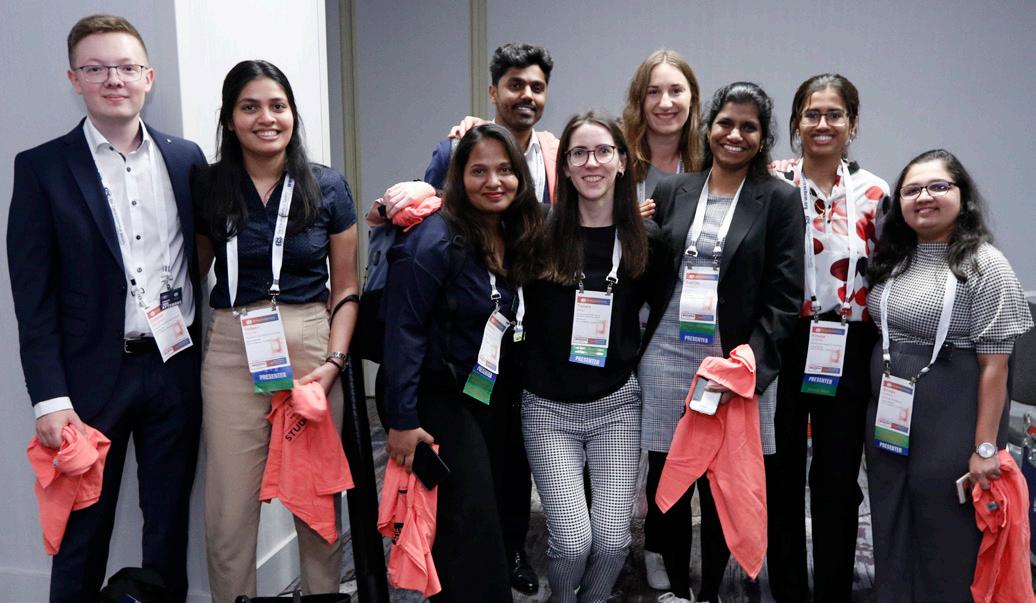
Almost 200 students and early career professionals attended the Student Mixer, mingling in a relaxed setting while enjoying light hors d’oeuvres and refreshments. Everyone received a Student Mixer t-shirt. One lucky ECS Student Chapter won t-shirts branded with their new chapter logo for all their members. Another institution without a chapter also won t-shirts encouraging forming a chapter! Special thanks to Pine Research and Scribner for supporting our younger members and sponsoring the event.
Annual Society Business Meeting and Luncheon
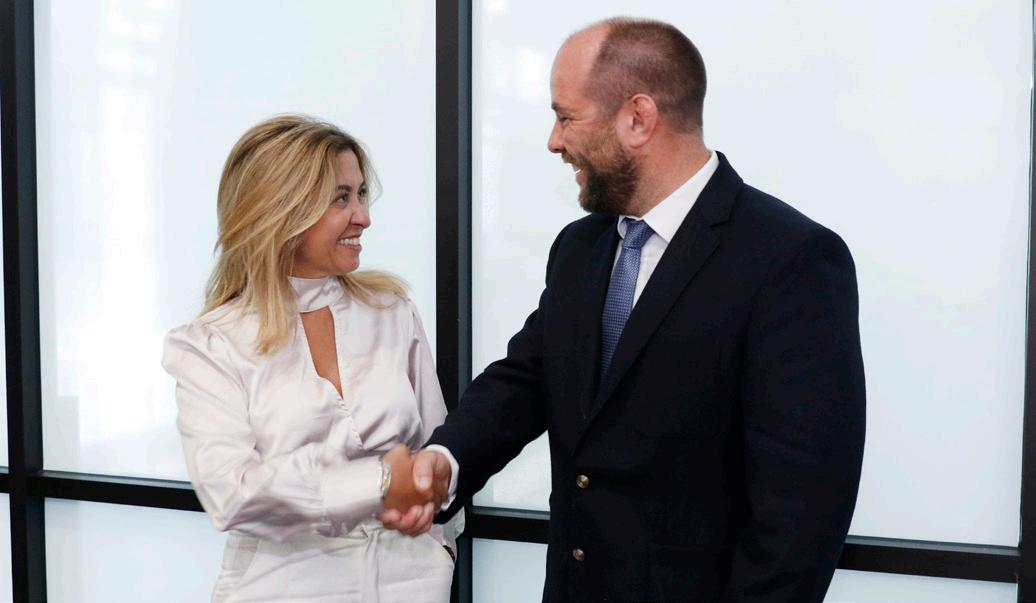
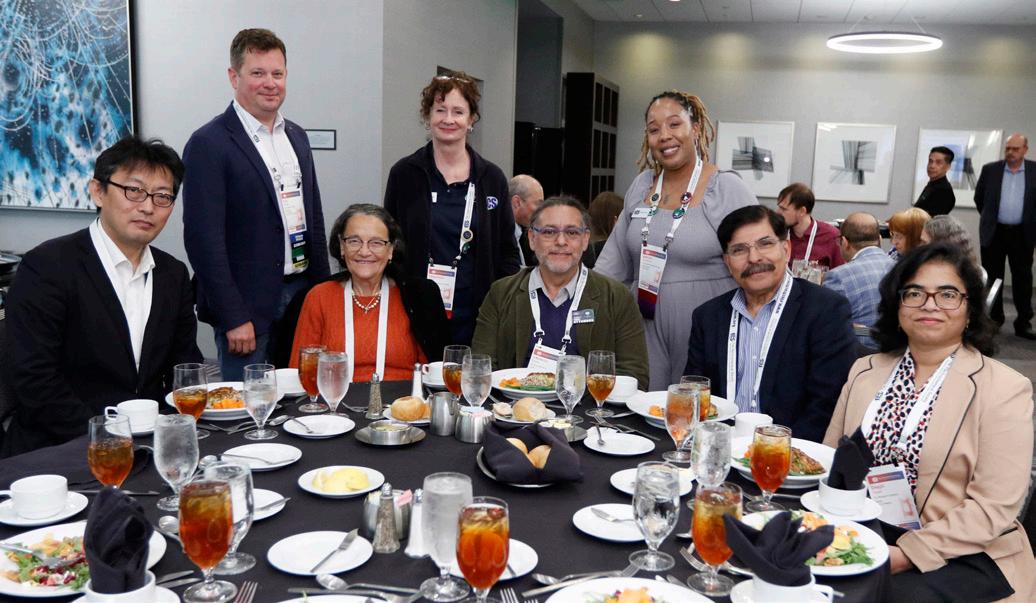
The Annual Society Business Meeting and Luncheon (ASBM) took place on May 28. Over a plated lunch, attendees heard the Society’s senior leaders review the successes and challenges of 2023 and preview exciting plans for the coming year.
ECS Executive Director and CEO Christopher Jannuzzi welcomed attendees, thanked the general meeting sponsors, and introduced ECS President Gerri Botte. After pointing out Ralph White, her mentor and academic “father” in the audience, Gerri described her goals and vision as ECS President were to build on past and recent successes to inspire a broader awareness and understanding of electrochemical technologies’ critical role in making positive change in our world and solving the planet’s grand challenges. The Presidential Ad Hoc Committee on Sustainability under Vice President Jim Fenton’s direction was formed and the “ECS Toward Sustainability” initiative was launched. The newly formed ECS Presidential Ad Hoc Committee on Diversity, Equity, Inclusion, and Engagement chaired by division chair Elizabeth Biddinger is focusing on recommending mechanisms for ensuring diverse representation in the Society’s governance structure; creating a grant and recognition program to engage ECS community members from minority-serving institutions and groups traditionally underrepresented within the society; and drafting recommendations for increasing Diversity, Equity, Inclusion, and Engagement within the Society. Gerri witnessed firsthand our community’s great ENGAGEMENT with the Society, which she is certain our new leadership will continue to embrace and expand.
Gerri formally opened the meeting and then Secretary Marca Doeff delivered her report. She started by introducing the new ECS officers: Colm O’Dwyer, ECS President, 2024–2025 term; Robert Savinell, 3rd Vice President, 2024–2025 term; and Gessie Brisard, ECS Secretary, 2024–2028 term.
The 2023 243rd and 244th ECS Meetings in Boston and Gothenburg resulted in total meeting attendees of 6,281 from 65 countries; 94 symposia; 6,347 abstracts; and $246,000 awarded in
Meeting attendees stop by the ECS Booth to try their luck in the ECS raffle.
Student attendees enjoy the bustling ambiance of the Student Mixer, a perfect atmosphere for reconnecting with old friends and making new ones!
ECS Exhibit Booth Raffle
ECS President Gerri Botte welcomes incoming President Colm O’Dwyer
Annual Society Business Meeting and Luncheon attendees learn about the past year’s successes and plans for the Society’s future.
speaker support through symposia funding. ECS sponsored two meetings in 2023 (18th International Symposium on Solid Oxide Fuel Cells and StorageX International Symposium series). Upcoming meetings were reviewed.
Marca outlined changes in membership from 2022 to 2023: a 12.2 percent increase in the total number of members with more than 8,800 current individual members; 15.5 percent increase in student members; 10.6 percent increase in student chapters amounting to a total of 136—with 11 new chapters seeking approval at the Board meeting later in the week; an 8.5 percent increase in institutional partners, bringing the total to 51, the most ever in the Society’s history.
Fellowships and grants awarded in the past year were: $100,705 awarded by ECS divisions for biannual meeting travel grants; two ECS Toyota Fellows each received $50,000 for a total of $100,000; four ECS Summer Fellows each received $5,000 for a total of $20,000; and one ECS Colin Garfield Fink Summer Fellow received $5,000.
Publications reached new highs with 9,015,249 articles and abstracts downloaded in 2023—a 12 percent increase over the previous year and a nearly 300 percent increase since moving to IOP in 2020; 1,763 journal articles were published in the 2023 volume year; and of the articles published in 2023, 614 articles (35 percent) were open access.
ECS Treasurer Elizabeth (Lisa) Podlaha-Murphy reported that 2023 was a strong financial year, primarily due to the investment portfolio. Revenue in 2023 was $8M with the majority (89 percent) coming from three program areas: Meetings (48 percent), Publications (34 percent), and Membership (7 percent). While expenses amounted to $8.3M, the value of Society assets continues to grow and has nearly returned to pre-pandemic levels.
Chris described critical new initiatives that the Society is undertaking. Colm shared his goal to use his presidency to elevate our STEM education and outreach by ensuring the creation of innovative programs like our battery workforce development and upskilling education courses.
Gerri then closed the meeting.
Notable Special Events
Electrochemistry in Action: Fuel Cell Model Car Race
The model car outreach event demonstrated how renewable energy can be harnessed to generate green hydrogen and how this hydrogen can be used for propulsion. Through these practical applications, students were introduced to the fundamental principles of electrochemistry.
Meet the Editors
Attendees had the valuable opportunity to connect with esteemed ECS journal editors Rohan Alkokar (JES and ECSA), Trisha Andrew (ECSSP), Netz Arroyo (ECSSP), Stefano Cinti (JES), Taylor Garrick (JES), and Peter Mascher (JSS) at the ECS booth. The editors answered questions about journal processes, discussed editorial opportunities, and shared their vision for the growth of ECS Publications.
Women in Green Hydrogen Breakfast
Women working in the green hydrogen sector gathered for a free networking event designed to foster diversity and visibility in the field. This event brought female scientists together and encouraged the amplification of their voices and contributions in the industry.
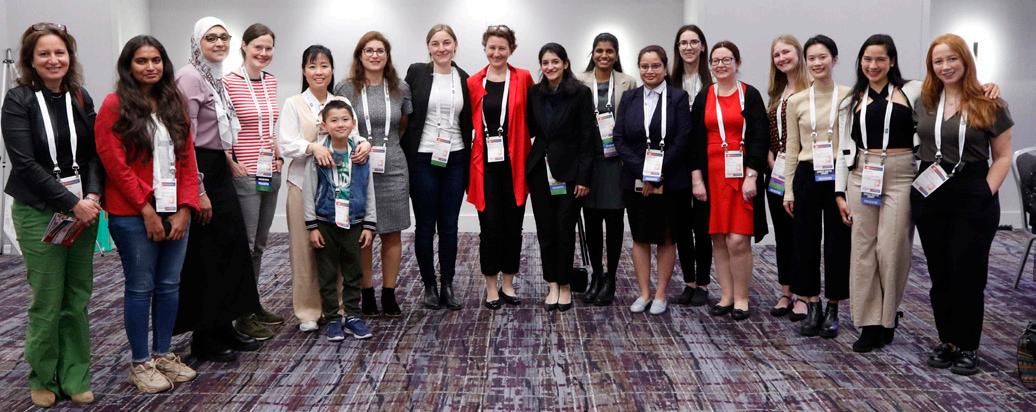
Sponsors and Exhibitors
ECS applauds the meeting sponsors and exhibitors whose support and participation contributed directly to the meeting’s success.
Thank you for developing the tools and equipment driving scientific advancement, sharing your innovations with the electrochemical and solid state communities, and providing generous support for the 245th ECS Meeting!
245th ECS Meeting – General Meeting
Sponsors
Thank you to the 245th ECS Meeting sponsors!
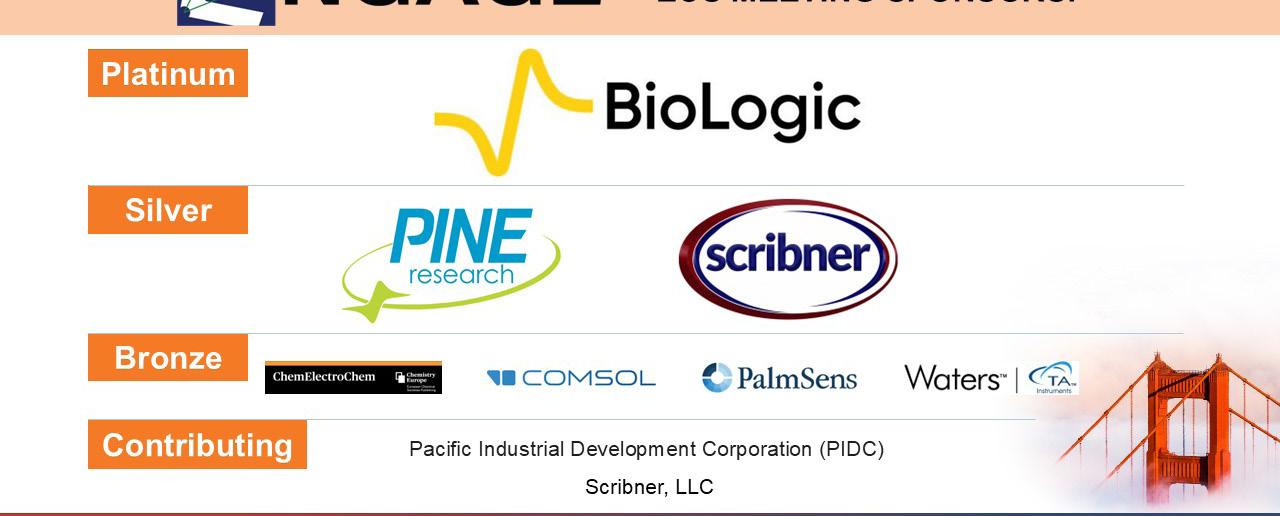
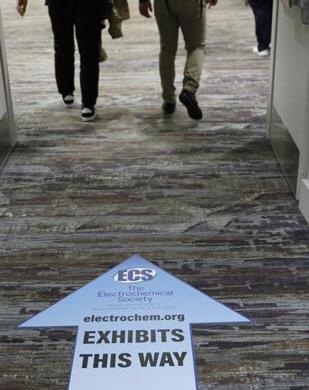
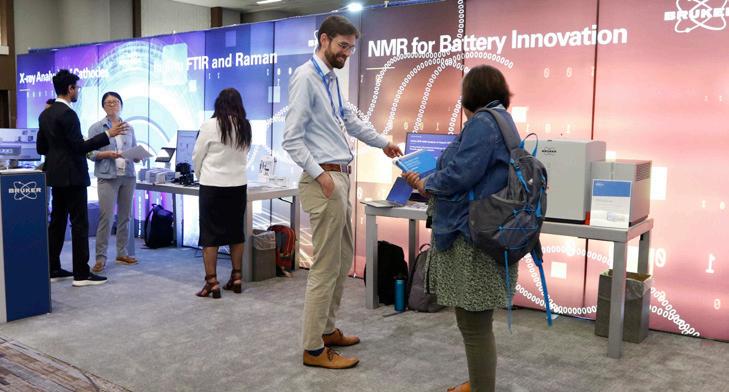
Thank you to our exhibitors!
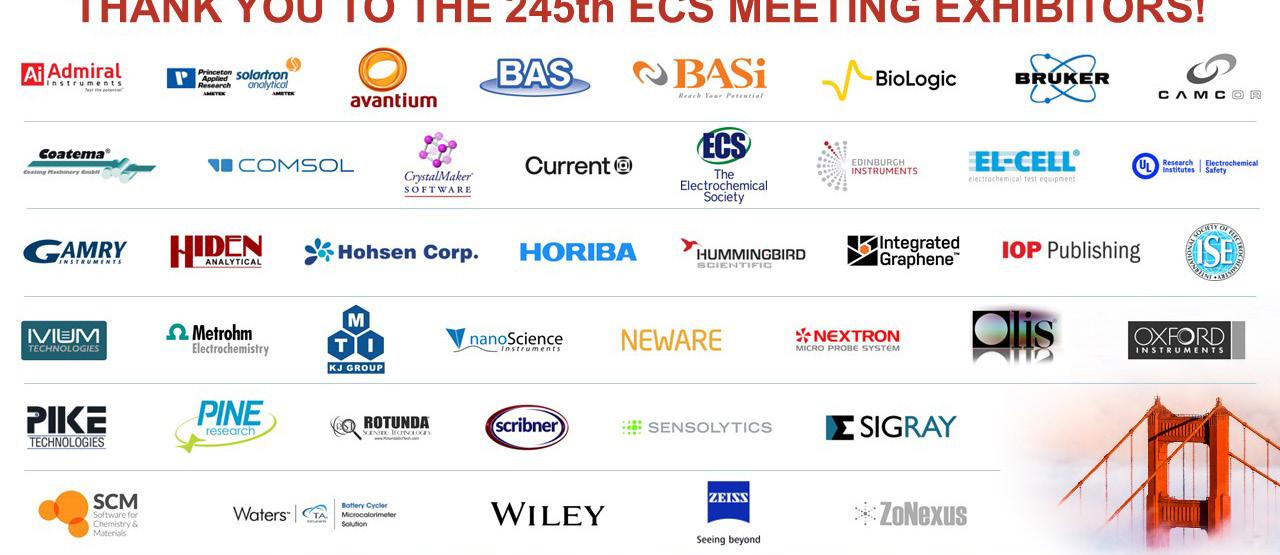
245th ECS Meeting – Symposia
Sponsors
Thank you to the 245th ECS Meeting symposia sponsors!
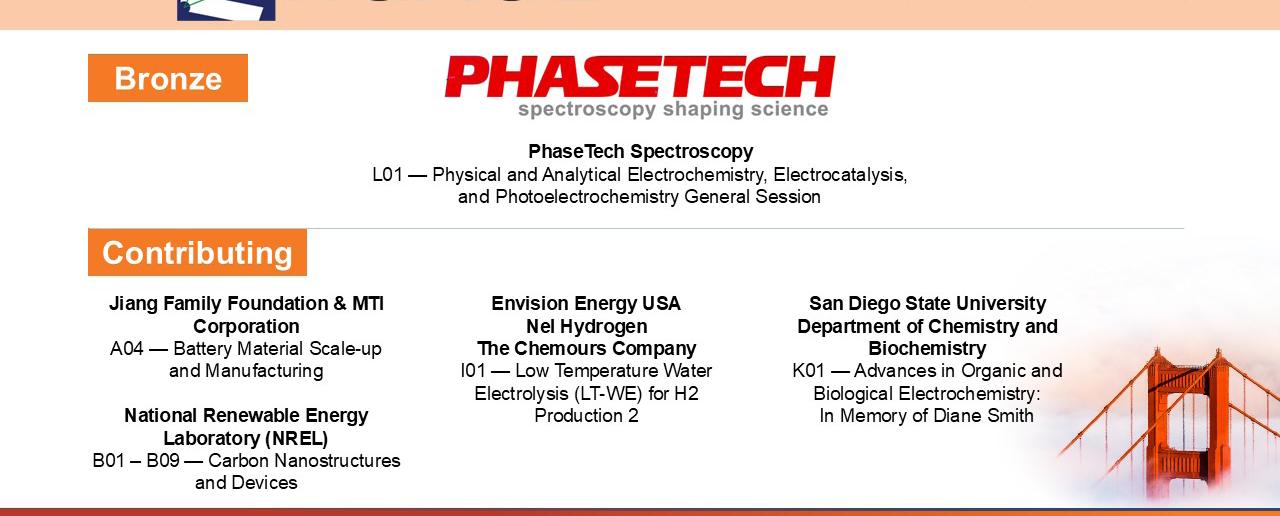
Attendees gather for the Women in Green Hydrogen Breakfast
Meeting attendees visited with more than 40 industry leaders at the Exhibit Hall
245th ECS Meeting – Exhibitors
ARE
842 oral student talks
2,294 total oral talks
1,240 student abstracts
575 invited talks
3,022 total abstracts
388 student posters
701 total posters
25 ECS award & keynote talks
439 sessions
48 symposia
68 countries represented
245th ECS MEETING BY THE NUMBERS
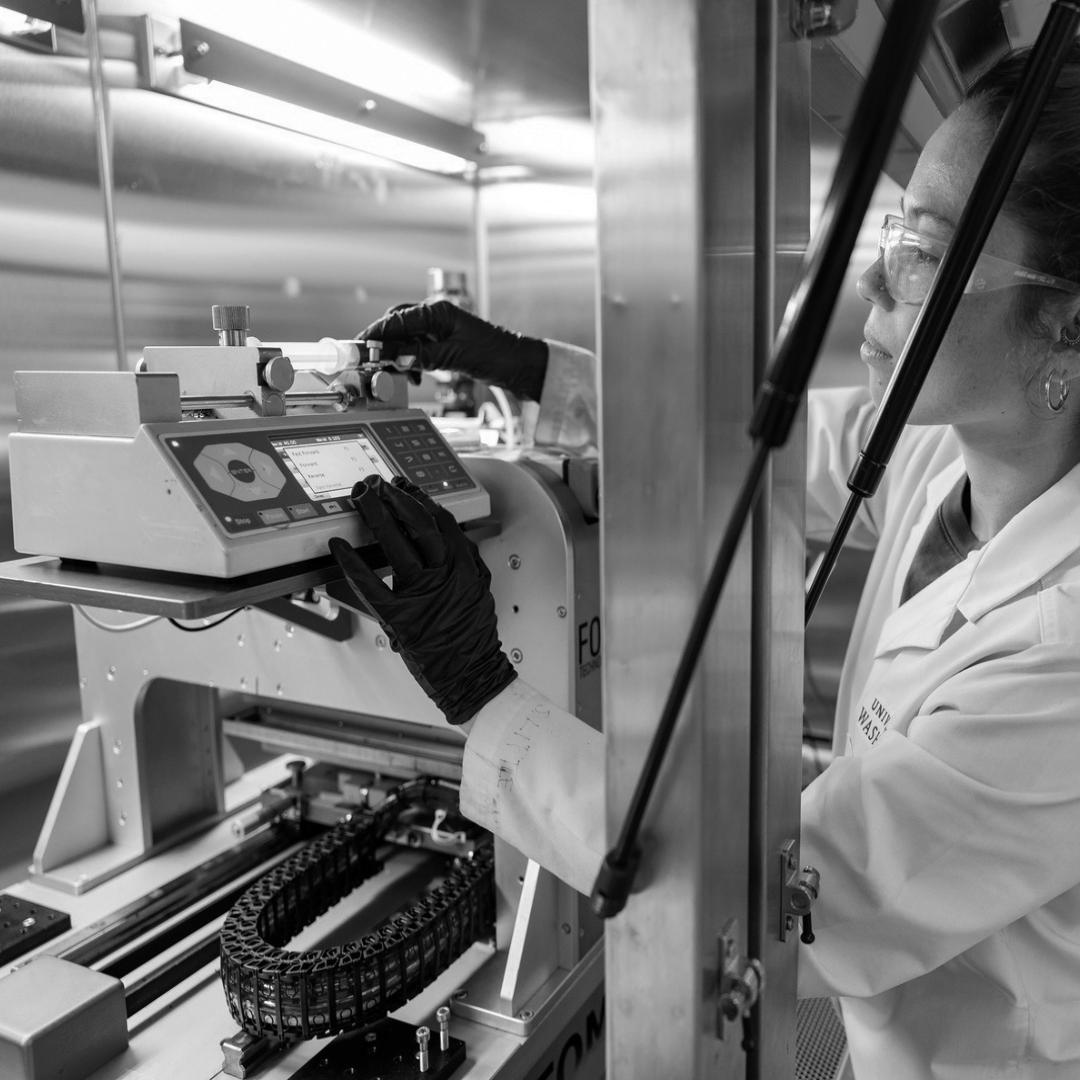
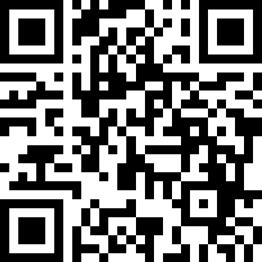
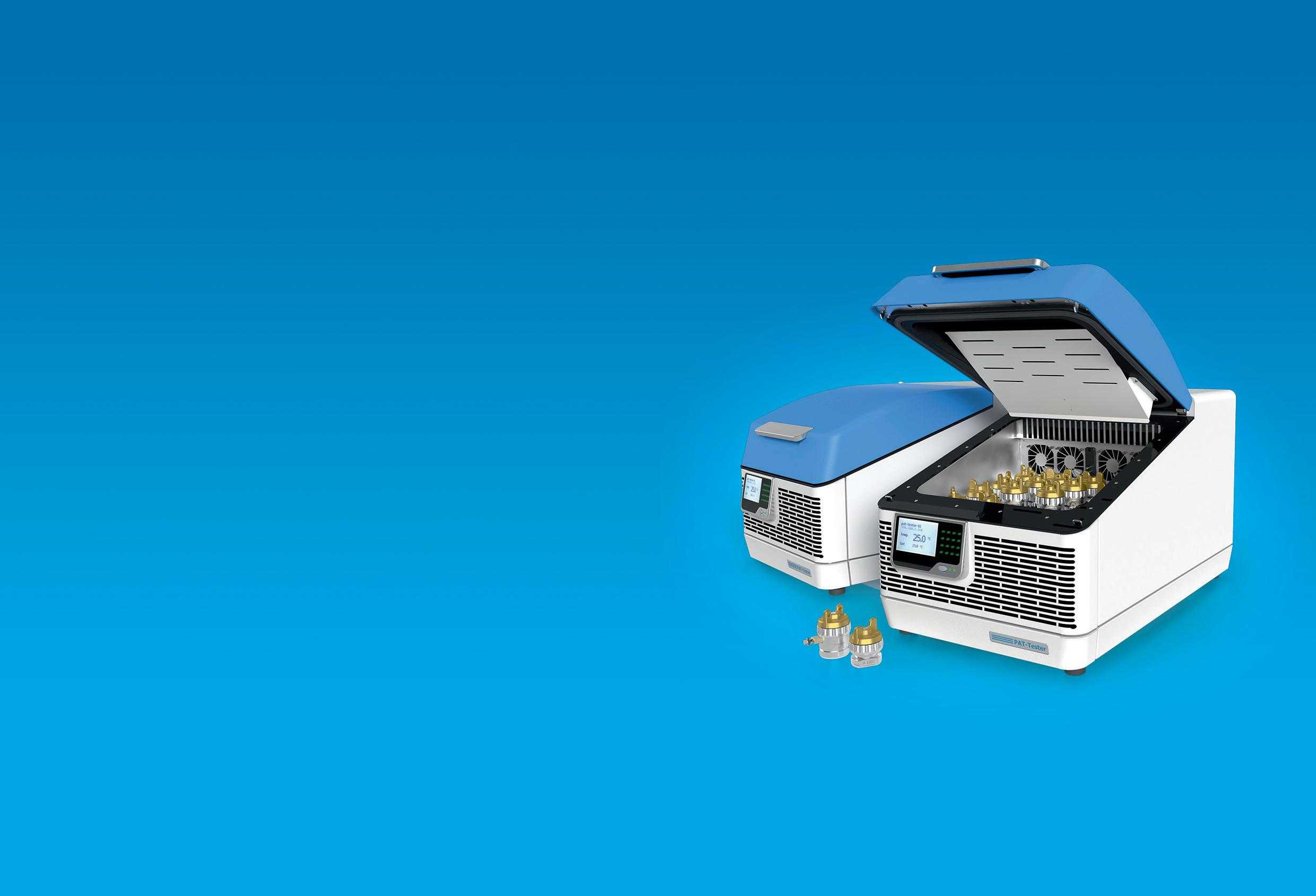
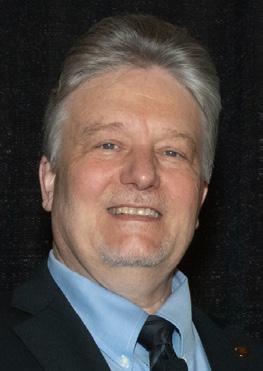
Ion Storage Systems Selected for SCALEUP Award
The US Advanced Research Projects Agency-Energy (ARPA-E) has selected Ion Storage Systems (ION), a startup founded by Eric D. Wachsman, for a SCALEUP award. The SCALEUP (Seeding Critical Advances for Leading Energy technologies with Untapped Potential) award provides major new funding to previous ARPA-E awardees that have successfully de-risked their technology and established a viable route to commercial deployment. At $20,000,000 it’s the largest award ARPA-E provides. ION will support domestic
manufacturing of next generation solid-state lithium-metal batteries and accelerate commercialization of the technology into the electric vehicle market.
ION is commercializing next-generation, high power-density, solid-state lithium metal batteries based on ceramic electrolyte manufacturing for large-scale, low-cost battery production. ION is a spinoff company of the University of Maryland’s Maryland Energy Innovation Institute (MEI), directed by Dr. Wachsman.
ARPA-E advances high-potential, high-impact energy technologies that are too early for private-sector investment. ARPA-E awardees are unique because they are developing entirely new ways to generate, store, and use energy.
How to Make the Transition to Green Energy Truly Sustainable: Solve the Corrosion Problems First This Time!
The transition to a net-zero energy system by 2050, in line with Paris Climate Targets, requires a dramatic shift away from fossil fuels and the ramp-up of new, renewable energy resources, including wind, solar, nuclear, hydro, and biomass. There are many challenges to achieving this goal, among them access to critical mineral resources and their supply chains, scale-up and deployment, and infrastructure investment. One often-overlooked but critical factor is the stability and reliability of these new technologies—the inherent corrosion and degradation of these materials systems affects efficiency, safety, and recyclability.
Corrosion is the (electro)chemical degradation of materials, and for many of the new and low-carbon technologies it is a critical barrier to their successful application at scale. For example, batteries, fuel cells, and electrolyzers have highly unstable interfaces—the corrosion of electrodes, current collectors, and electrocatalysts determines the device efficiency and lifetime. Corrosion also dramatically impacts safety and cost, and is the main concern in the ongoing question of how
to deal with long-term nuclear waste storage for low-carbon nuclear fission energy generation. And nuclear fusion will only be realized if we first address a whole raft of corrosion challenges. Hydrogen will be essential in green technologies for energy storage, transport, and hard-to-decarbonize industries such as steelmaking. However, hydrogen causes metals to embrittle and crack—a phenomenon that is still not well understood.
In July, some of the world’s leading corrosion scientists converged at the ECS-sponsored Gordon Research Conference on Aqueous Corrosion in a small college in New Hampshire to discuss these issues. Leading global researchers working across different new energy technologies discussed new and old challenges, and shared insights into promising models and tools to study and mitigate corrosion.
The cost of corrosion to industrial economies stands at around 3% of GDP—we have an opportunity as we transition our infrastructure and systems to remove this enormous burden, but only if we act now to ensure we embed corrosion science into the design and development of new technologies. In many countries, corrosion and electrochemical science are not even taught in most engineering and science university classes. It is, therefore, not surprising that corrosion is often an afterthought in new technology development, while it should be on the front line. Corrosion scientists are ready to contribute to the energy transition before the financial and environmental costs of corrosion means intervention is needed—let’s make industry 4.0 truly sustainable.
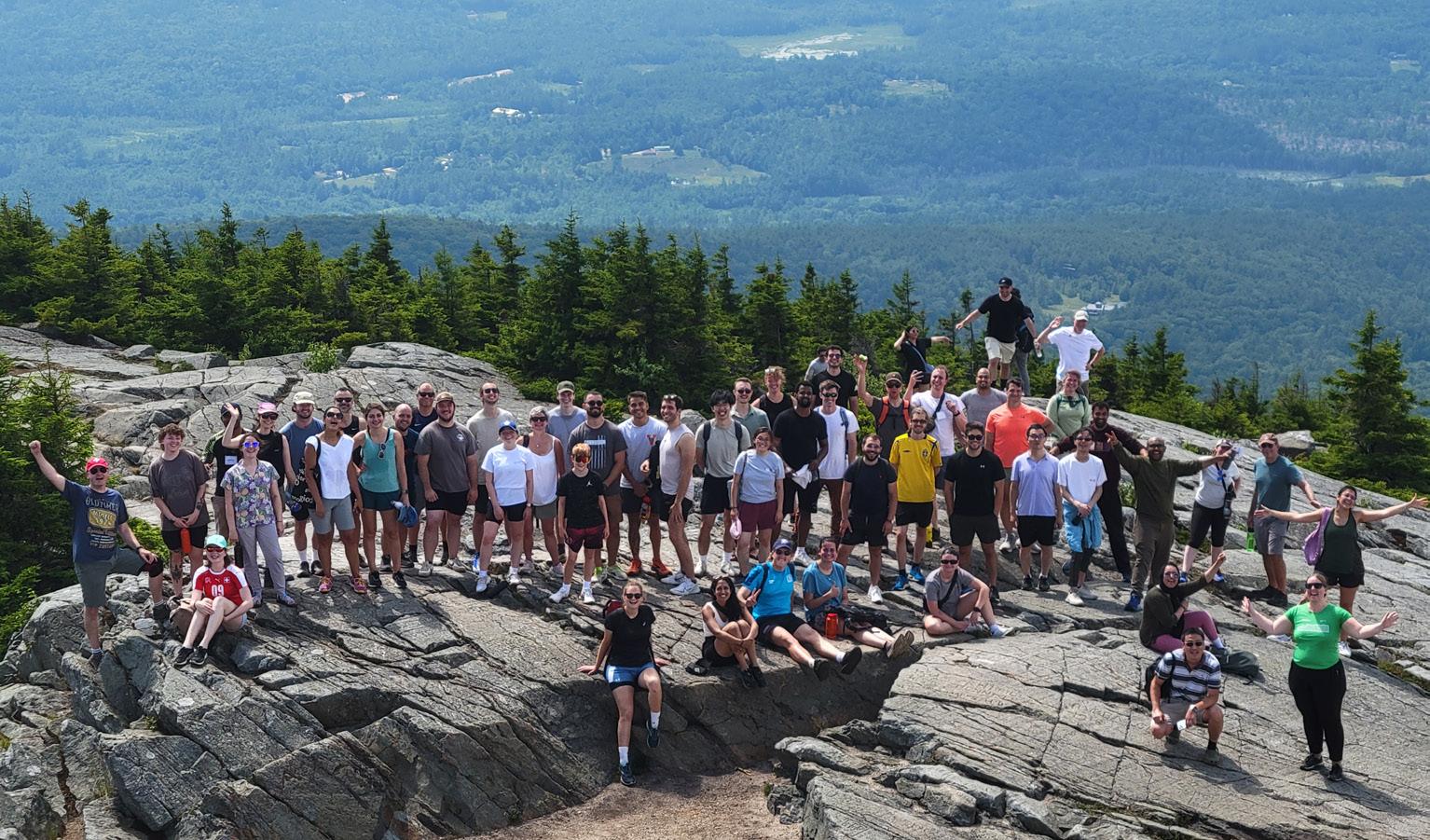
Contributed by Mary P. Ryan (conference chair), Jenifer S. Locke and Arjan Mol (conference cochairs), and Yolanda Hedberg
Participants in the 2024 Aqueous Corrosion Gordon Research Conference
8th Baltic Electrochemistry Conference: Finding New Inspiration 2
The eighth conference in the Baltic Electrochemistry series took place in Tartu, Estonia, from April 14 to 17, 2024. The Institute of Chemistry at the University of Tartu hosted “Finding New Inspiration 2” (BEChem 2024) in collaboration with Elektrokeemia Selts, the Estonian electrochemistry society. BEChem 2024 welcomed 181 delegates from 22 countries.
Estonia, a small country in the Baltic region of Northern Europe, is bordered by the Gulf of Finland to the north, the Baltic Sea to the west, Latvia to the south, and Lake Peipus to the east. Sweden and Finland are across the Baltic Sea. The city of Tartu is called Estonia’s spiritual capital. Locals describe a special Tartu spirit (Tartu vaim) created by the “time-stands-still” feel of its wooden houses and stately buildings, and the beauty of its parks and riverfront. Established in 1632, the University of Tartu is Estonia’s oldest and largest higher education institution.
History
The Institute of Chemistry at the University of Tartu has organized electrochemistry conferences since 1966. Scientists from all over the former Soviet Union attended and highly praised the first nine symposia, titled “Electrical Double Layer and Adsorption at Solid Interfaces.” After Estonia’s re-independence, the conference series was updated and renamed “The Baltic Electrochemistry Conference” since it was attended by scientists from all around the Baltic Sea, as well as from the UK, US, Canada, France, Italy, Japan, and other countries. The first seven conferences were:
June 6–18, 1996 Kääriku,Estonia
October 6–12, 1999 Palanga, Lithuania
April 23–26, 2003 Gdansk-Sobieszewo, Poland
March 13–16, 2005 Greifswald, Germany
March 4–5, 2008 .............. Tartu, Estonia
June 15–17, 2016 Helsinki, Finland
November 4–7, 2018 Tartu, Estonia
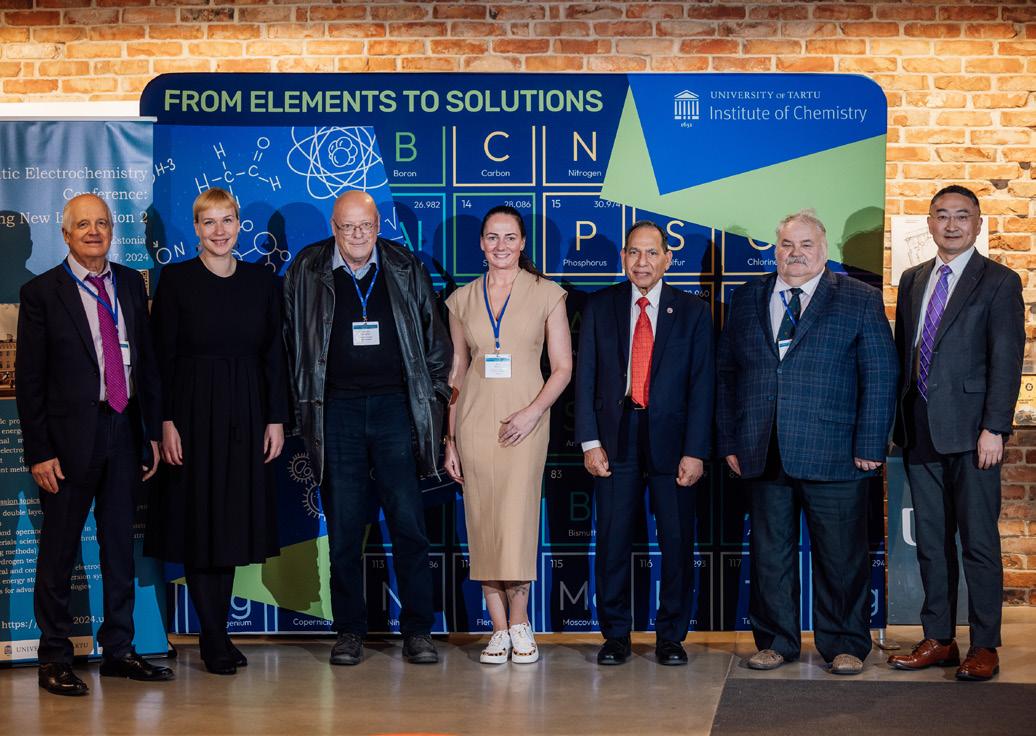
World-renowned scientists in physical and electrochemistry who participated in BEChem
included (from
to
Prof. Alexei Kornyshev, Assoc. Prof. Liis Siinor, Prof. Richard Compton, Dr. Piret Pikma, Dr. Shubash C. Singhal, Prof. Enn Lust, Prof. Xiao-Dong Zhou Photo: Maanus
BEChem 2024
The scientific program of BEChem 2024 focused on sustainable energetics; the application of operando and computational methods for developing interfacial electrochemistry; and finding new employment for prominent electrochemical measurement methods. Estonian electrochemical companies were highlighted, and a special session reviewed developments in science-based industry toward sustainable energy solutions. We were pleased to have seven keynote speakers, all highly acknowledged scientists in their respective fields:
• Prof. Alexei A. Kornyshev
• Prof. Elena Baranova
• Assoc. Prof. Svitlana Pylypenko
• Prof. Enn Lust
• Dr. Monika Hartl
• Dr. Rasmus Palm
• Dr. Subhash C. Singhal
The BEChem 2024 Organizing Committee —Assoc. Prof. Liis Siinor, Dr. Piret Pikma, and Prof. Enn Lust—extends its deepest gratitude to our sponsors for their generous support. Their contributions enabled us to hold a successful BEChem 2024, for which we are profoundly thankful.
ECS support is greatly appreciated. We hope you continue to be part of the Baltic Electrochemistry Conference in the future, as the event’s success and relatively high attendance proved the importance of organizing smaller scientific conferences targeted to a specific region.
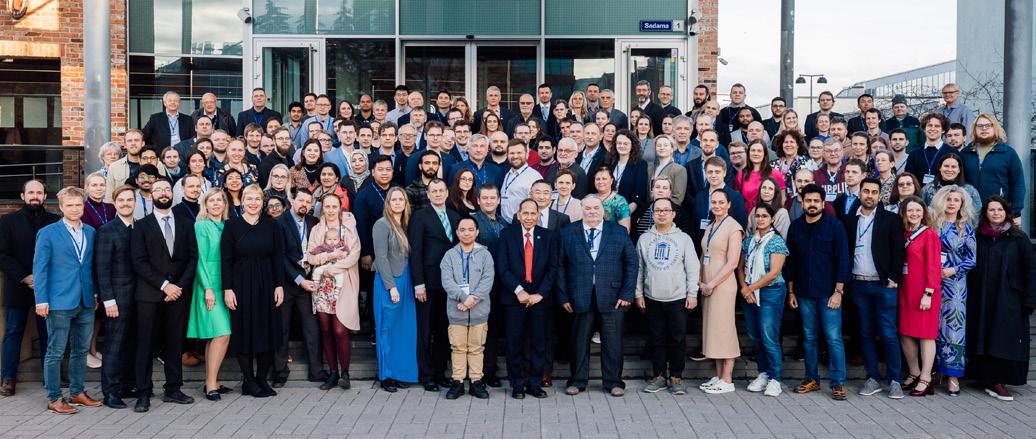
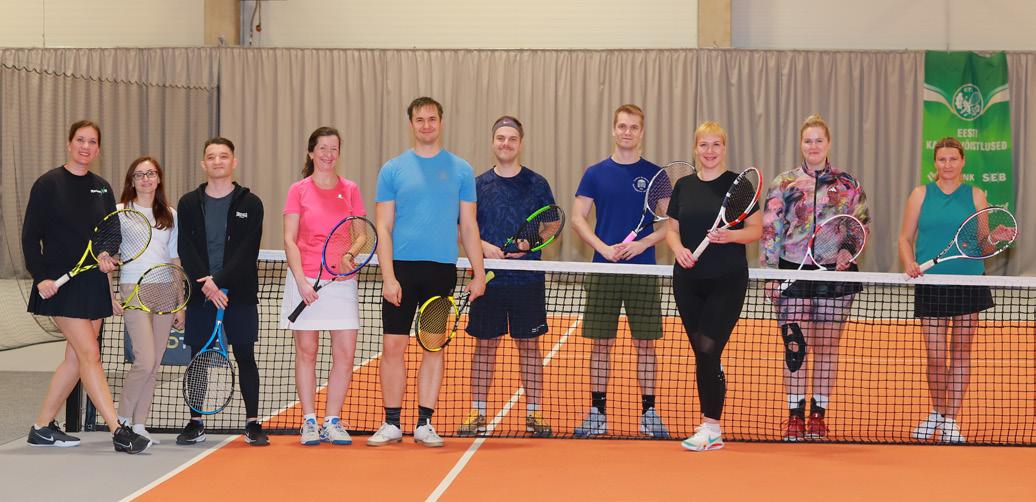
2024
left
right):
Kullamaa
Delegates to the 8th Baltic Electrochemistry Conference gathered in Tartu, Estonia. Photo: Maanus Kullamaa
A tennis tournament took place as part of the 8th Baltic Electrochemistry Conference.
In Memoriam ...
Dennis H. Evans
1939–2024
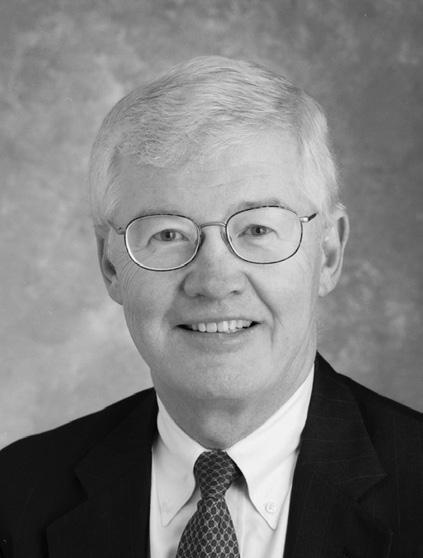
It is with great sadness that I learned of the passing of my friend and mentor Dennis Evans on March 26, 2024. I sat down to write this tribute many times, but the appropriate words to describe Dennis and his impact on my life and that of many others were difficult to conjure. Each time I tried, more and more memories came to mind and I ended up spending more time thinking about all that he did for me and those who studied with him than writing this. It is these memories that finally gave me the inspiration and opportunity to remember the many good times that describe the influence and impact that Dennis had on me and on many others.
I met Dennis in 1983 when I was investigating which graduate school to attend. Little did I know at the time that I had just met someone who would play a central role in my life for the next 40+ years. Arriving at Wisconsin in the fall of 1983, I quickly joined Dennis’ research laboratory. Dennis’ laboratories at the time were at the forefront of using digital/computer simulation to fit electrochemical data to extract heterogeneous kinetic parameters. This was a time of great change in instrumental laboratories as the early 1980’s coincided with the advent of the personal computer. At the time the Evans laboratory was in the process of migrating these simulations to personal computers. This was my first insight into Dennis and his adaptability/embracing of new ideas and technologies—and this was one of the important lessons that I took from Dennis.
Dennis encouraged his students to be independent and allowed projects to grow with the student. In my case, he indulged my interest in combining spectroscopy and interfacial electrochemistry. Together, we bult a system using (the then relatively newly discovered) surface enhanced Raman spectroscopy to study the structure of molecules at an interface under potential bias. Dennis embodied being a lifelong learner, being curious, and not being intimidated to try new things— another of the life lessons that Dennis provided.
I presented this work as a poster at a conference in Lake Tahoe— my first conference presentation; to say I was nervous would be an understatement. I wanted everything to go well, and to make Dennis proud. The night before the presentation, Dennis, Mary Wirth, my wife, and I met to go over the poster and then have dinner. During dinner, my wife asked Dennis what she should call him. To say I was mortified would be an understatement. For context, the students and post-docs in the laboratory all called him Dr. Evans. When we were feeling cheeky, and not to his face, we called him DHE—his initials and the way he signed documents that we sent to the group. He never told us not to call him Dennis; we just called him Dr. Evans automatically out of respect to him, and perhaps because we all were a little in awe of him. In response to my wife’s question, he told her to call him Dennis. In hindsight, he was tacitly giving me permission to also call him Dennis; however, this was a step too large for me to take at the time because my respect and admiration for him were too great. So for the rest of the conference (and the entirety of my graduate studies), my wife called him Dennis, and I called him Dr. Evans. It probably wasn’t until I had been promoted and achieved tenure that I became slightly, but never totally, comfortable calling Dennis anything other than Dr. Evans. As I began my independent career, Dennis Evans was a person who was always there to support me and to serve as a person upon whom to model my career. One thing that
Dennis was remarkable at was seeing subtle aspects of experimental data and realizing what was important and what was not. When fitting simulations to experimental data, Dennis was a master at suggesting small changes to parameters to better fit the data. It was this attention to the importance of small details that stuck with me the most, and that I tried to emulate with my research students. In this way, he pushed us away from the obvious and out of our comfort zones, and encouraged us to try new things and to embrace the unknown.
The curiosity to try new things, and to see subtle features and explore them was something that he instilled in his students. This was a lesson that extended beyond science and into our everyday lives as well. I tried sushi the first time with Dennis, I had steak tartare with Dennis, and (of course) I enjoyed some of the most fabulous wines with Dennis and Mary. Once, when I was a relatively young assistant professor, Dennis invited me to join him and Mary for dinner one night at an Electrochemical Society meeting. I accepted because an opportunity to spend time with Dennis was always fun and enlightening, but I was also nervous about the potential size of the wine tab (something that Dennis and Mary were somewhat known for). Fortunately, before the dinner Dennis told me that they would be ordering some wonderful (read, expensive) wines and that he would take care of the wine tab. As always, Dennis was encouraging me to expand my horizons while simultaneously providing an easy landing. To this day, I think of Dennis whenever I am enjoying a glass of wine
When he relocated to Purdue, my wife and I visited Dennis and Mary in West Lafayette once when we were in Indiana. As always with Dennis and Mary, there was wonderful food and plenty of good wine. We reminisced about times past and people we knew. As the evening came to a close, we thanked them for a wonderful evening, and as I was leaving their home, I said good night to Mary and to my friend and mentor, Dr. Evans.
Dennis—Thank you for everything that you did for me, for all that you did for the many students that you mentored and (by extension) all that you did for the people whom your students mentored, and for your contributions to the organic electrochemistry and physical electrochemistry communities. My professional life and personal life are so much fuller from your influence. I will miss you, but the lessons that you taught remain with me for the rest of my life.
This tribute was contributed by Mark Anderson
Dr. Evans was born March 28, 1939 just outside of Grinnell, Iowa. His formal education began in a one-room schoolhouse in rural Iowa and concluded with his PhD in chemistry at Harvard. He stayed at Harvard as Instructor in the chemistry department for two years, and then he joined the chemistry faculty at University of WisconsinMadison where he rose through the ranks to become the Bascom & Meloche Professor of Chemistry. A summer graduate fellowship there is endowed in his honor by his former graduate student, Dr. Michael D. Ryan, Emeritus Professor of Marquette University. Dr. Evans was also Professor of Chemistry at the Universities of Delaware and Arizona, and Research Professor at Purdue University. Dr. Evans held one of the longest continuously renewed research grants from the National Science Foundation, spanning from 1968 to 2011, ending only with his retirement. He joined ECS in 1983 and was a longtime member of the OBE Division. He was named a Fellow of the Electrochemical Society in 1998 and received the OBE Division Manuel Baizer Award in 2004. He was an Emeritus Member, qualifying and receiving that membership in 2018. He also served on the Editorial Advisory Committee and several award review subcommittees.
In Memoriam ...
Luby Romankiw
1931–2024
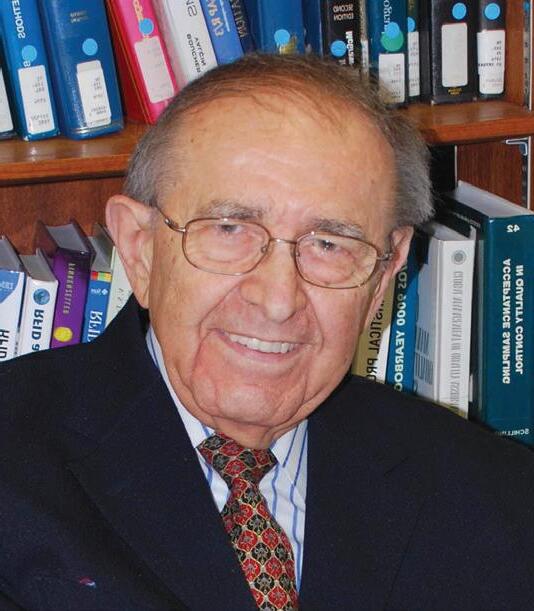
Dr. Lubomyr (Luby) Taras Romankiw was born on April 17, 1931, in Zhovkva, Ukraine, and passed away on June 27, 2024, in New Jersey, United States. He transformed electrodeposition from an art to a scientific discipline and put it onto the map as a platform technology for the development of many technologies in the computer and microelectronic industry. A worldrenowned electrochemical engineer, material scientist, engineer, and inventor, Luby received his BS in Chemical Engineering from the University of Alberta in 1955, and his MSc and PhD in Metallurgy and Materials from MIT in 1962. He joined IBM Research as a research staff member in 1962, later he was made a director of the Electrochemical Technology department, and was an IBM Fellow from 1986 until his retirement in 2018. He is listed as the inventor or co-inventor on more than 90 US patents, authored more than 150 articles, wrote 6 book chapters, and edited numerous volumes of technical symposia.
Luby is best known for his invention of magnetic thin film heads, which has been a critical enabler for mainframe servers and personal computers and has remained largely unchanged for decades as the storage density in a hard disc drive has exponentially increased. By the mid-1960s, magnetic drum storage was approaching its limit as the hand-made and assembled copper-wired heads were becoming too large to be extendible to smaller size and scalable to higher areal density storage.
Luby had three key inventions,1 the inductive transducer for the writing, the magneto resistive sensor for the reading, and the integrated thin film heads with the dual functionality. These inventions are accompanied by extensive fundamental studies on the electrodeposited magnetic thin film materials as well as the invention of tools and processes to manufacture them. Through-mask electroplating is one of the key processes invented by Luby,2 originally enabling the integration of photolithography with electroplating to define the copper coils and magnetic yoke in the three-dimensional structure of thin film heads. This concept has later been extended by adopting X-ray lithography into a LIGA process for the fabrication of MEMS and NEMS. In addition to the device design and integration scheme, another challenge by that time was to define and fabricate the magnetic material with the desired permeability, moment, coercivity, and anisotropy, using a manufacturable approach. Electrodeposition was therefore introduced by Luby for the fabrication of microelectronic devices with the invention of the paddle cell.3
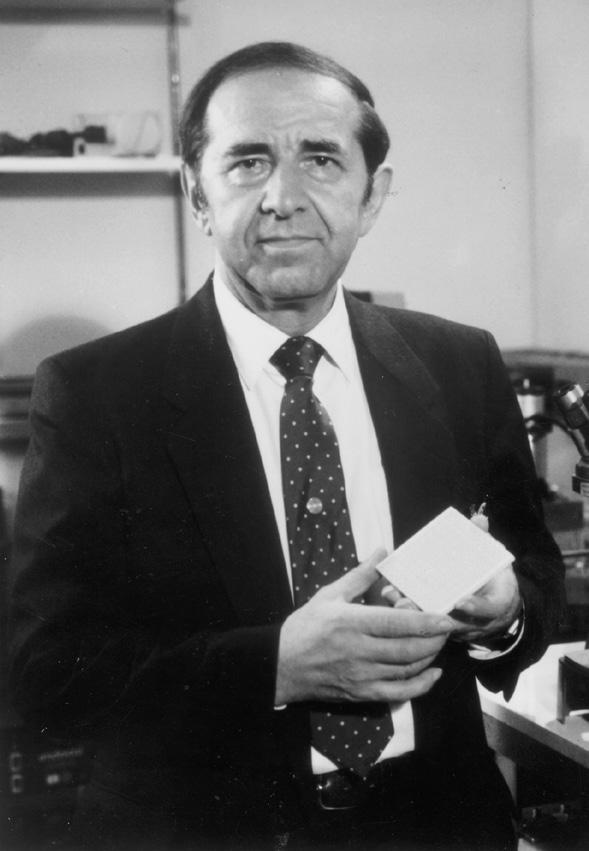
A reciprocating paddle movement results in a uniform and well controlled agitation, enabling the fabrication of permalloy, a superior soft magnetic alloy with 80% Ni and 20% Fe, with precise compositional control across a large area. The paddle cell allowed for the application of an exter-
nal magnetic field during electrodeposition, which, in conjunction with a thermal treatment process in a magnetic field, defined the easy axis of the permalloy with respect to the yoke shape,4 finally enabling the proper functioning of the thin film heads.
Luby continued to lead the research and development effort of magnetic storage for decades, including the electrodeposition of ternary alloys, nanostructured magnetic materials, and magnetic MEMS. In addition, his inventions and technical contributions go way beyond magnetic storage and magnetic devices.5 To name a few, electrodeposition, electroless deposition, electrochemical etching, and other electrochemical processes have been extended to the fabrication of flip-chip interconnects, copper interconnects, and onchip power converters, as well as thin film solar panels.
Luby was always a strong advocate for collaboration between industry and academia. He visited many universities and promoted collaboration on research projects in academia on topics related to problems in the electronics and computer industries. By doing that, he established strong connections with university professors and supported the education and training of younger generations of scientists and engineers.
A long-time ECS member, Luby joined the Society in 1965 and made tremendous contributions to the Society throughout his life. He organized numerous symposia and edited nine volumes of ECS symposia proceedings on topics that include Magnetic Materials and Processes, Devices for Storage and MEMS, and Electrochemistry in Electronics. He was named an ECS fellow in 1990 and an ECS honorary member in 2003. He received the ECS Electrodeposition Division Research Award in 1984, and the Vittorio De Nora Award in 1994. He received the Society of Chemical Industry Perkin Gold Medal in 1993 and the IEEE Morris N. Liebmann Memorial Award in 1994. He was inducted into the National Inventors Hall of Fame in 2012 and was elected as a foreign member of the National Academy of Engineering of the United States in 2014. Luby was also active in various organizations outside his technical profession, most notably serving as the Chief Scout of the Ukraine National Scout Organization (Plast), where he promoted leadership development of Ukrainian youth both in Ukraine and abroad. This was an outstanding honor as Lubomyr was only the 3rd person to have been elected Ukrainian Chief Scout within the past 112 years. His love of youth and his desire to instill strong leadership, ethical, and moral attributes in them is evident and reflected by their great love and respect for him. In 2020 Luby was awarded The Order of Prince Yaroslav the Wise, a Ukrainian award for distingished service in the fields of science, culture, education, health, and other state, charitable, and humanitarian matters in Ukraine.
His breakthrough thinking and approach to solving problems was contagious. He inspired us and many other colleagues to do our best scientific work. He was also the kindest mentor with his time, patience, encouragement, and leadership. Every meeting and interaction with Luby resulted in new ideas for research or new inventions. He has left behind a tremendous legacy.
This remembrance was contributed by Qiang Huang and Lili Deligianni
References
1. US patents 3,840,898; 3,908,194; and 4,295,173.
2. US patents 4,281,057; 4,315,985; and 3,853,715
3. US patents 3,652,442; and 5,312,532.
4. US patents 4,102,756; and 4,003,768
5. Patents by Inventor Lubomyr T. Romankiw, at JUSTIA patents.
Dr. Romankiw holding a ceramic wafer with the thin film heads he invented.
In
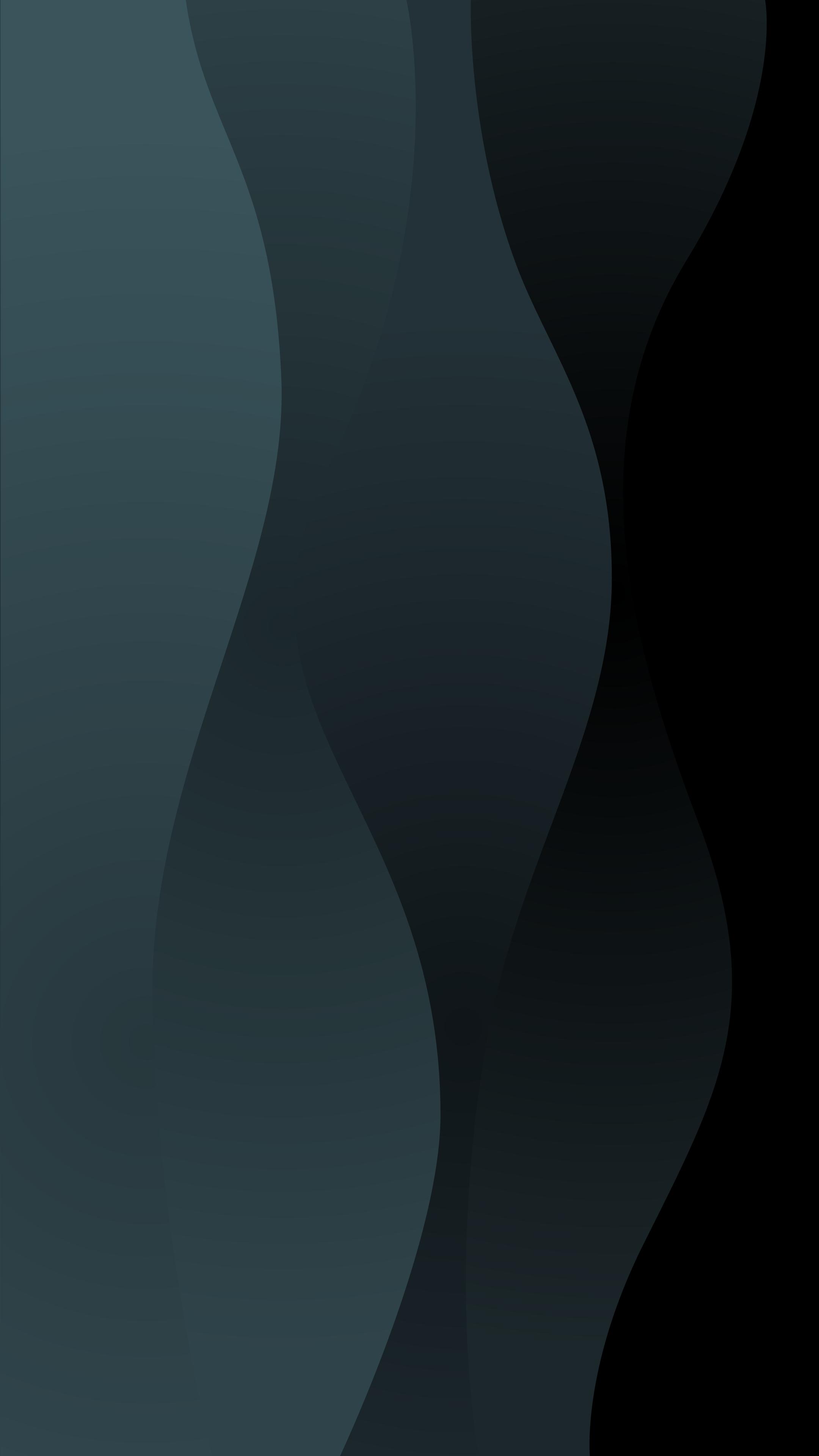
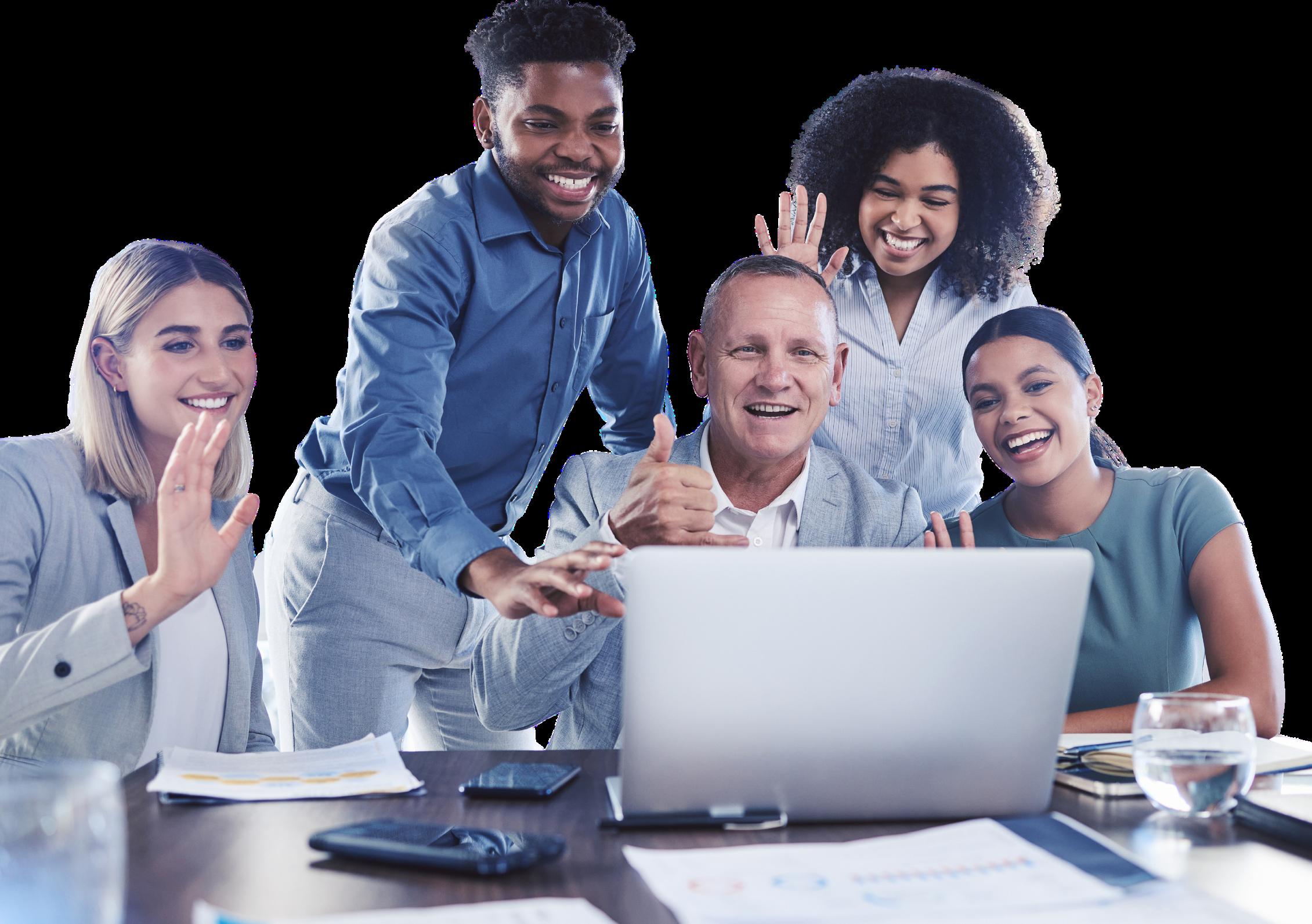
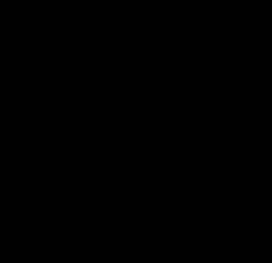
Looking at Patent Law
Patenting an Invention for Improved Electrochemical Machining by Combining Magnetic Field Waveforms, Ultrasonic Motion, and Pulsed Electric Field Waveforms: A Case Study
...Overcoming an Obviousness-based Prior Art Rejection
by E. Jennings Taylor and Maria Inman
In this installment of the ‟Looking at Patent Lawˮ articles, we present a case study of a patented invention for an improved electrochemical machining process based on combining magnetic field waveforms, ultrasonic motion, and pulsed electric field waveforms. The subject invention aligns with the technical interests of several divisions of The Electrochemical Society (ECS), including Corrosion (CORR), Industrial Electrochemistry and Electrochemical Engineering (IE&EE), and Physical and Analytical Electrochemistry (PAE). The case illustrates overcoming prior art obviousness rejections by incorporating dependent claim limitations and a limitation from the specification into the main independent claim. In addition, the case illustrates an inventor-initiated exception to avoid the 18-month publication requirement by the United States Patent and Trademark Office (USPTO). The case also introduces ECS members to an emerging technology of interest to industry.
Recall from our previous article,1 the prosecution history of a patent application is publicly available in the file wrapper available at the USPTO Patent Center.2 With the USPTO Patent Center system as the primary source of information for this case study, we illustrate the prosecution “events” encountered during the examination of US Patent No. 10,357,839; “Method for Electrochemical Machining using Sympathetic Waveform Interactions”.3 The ‘839 patent issued on July 23, 2019 with inventor Curtis Bradley. Dr. Bradley is currently a Senior Roboticist at General Electric Global Research Center in Schenectady, NY. Dr. Bradley received his MS in Electrical and Electronics Engineering and PhD in Mechanical Engineering from Rensselaer Polytechnic Institute.
The ‘839 patent abstract generally describes the invention as follows:
“A system and method for combining the effects of magnetic field waveforms, ultrasonic motion, and pulsed electric field waveforms in an electrolytic cell creates a sympathetic interaction among the three waveforms that improves the electrochemical machining process. Precisely controlling the coupled effects of simultaneously applying at least two of the three waveforms to the electrochemical cell improves the metal removal rate of the process and the surface finish of the resulting piece. The coupled effects are termed a sympathetic interaction as they can be damped or amplified by modifying
the phasing, frequency, orientation, or amplitude of the waveforms. In an electrochemical machining process aided by an external magnetic field and periodic ultrasonic relative motion between the tool and working piece, improvements are realized by utilizing for at least two of the magnetic field waveform, electric waveform, and ultrasonic waveform, a common frequency and a chosen phase lag between the two waveforms.”
Artificial Intelligence (AI) generated summaries of the ‘839 patent using Patsnap software are:
Technical Problem Summary
“The technical problem addressed in this patent is to develop an improved system and method for electrochemical machining that combines the effects of multiple technologies, including pulsed current, ultrasonic actuation, and magnetic fields, to achieve better results than each technology alone.”
Benefit Summary
“The present invention is a system and process for improving electrochemical machining. It involves using an electrolyte solution containing ions and a special electric field waveform, a magnetic field waveform, and an ultrasonic waveform to (continued on next page)
Taylor and Inman
(continued from previous page)
generate a force on the ions in the solution. This force acts on the surface of the metallic workpiece and the electrically conductive tool, resulting in a more precise and efficient electrochemical machining process. The invention provides a unique way to control the electrochemical machining process that allows for improved accuracy and productivity.”
(Author note: Patsnap is one of numerous commercially available patent analysis software packages.)
Patent Applications
This article summarizes the prosecution history of US Utility Patent Application No. 15/290,258 leading to US Patent No. 10,357,839. In Table I, we list the patent applications related to Patent Application No. 15/570,848. US Provisional Patent Application Nos. 62/238,940, 62/238,951, and 62/238,959 were all filed on October 8, 2015. Recall, a claim or claims are not required in a provisional patent application4 and a provisional patent application expires one year from its filing date.5 US Utility Patent Application No. 15,290,258 was filed on October 11, 2016. The utility patent application was filed within one year of the filing date of the provisional patent application. Note, if the one-year date falls on a weekend or holiday, the one-year date is extended to the next weekday. Consequently, the priority date of the US utility patent application is the filing date of the US provisional patent applications; October 8, 2015.6 The inventor team used the provisional patent application as a placeholder while additional experimental activities progressed. Although it is somewhat unusual to file three US provisional patent applications on the same day, the inventor’s patent attorney filed separate provisional applications covering magnetic field/electric field interactions, ultrasonic actuation/electric field interactions, and magnetic field/ultrasonic actuation interactions. The US Utility Patent Application combined the three provisional patent applications with magnetic field/ultrasonic actuation/electric field interactions. A detailed discussion of the costs/ benefits of this approach are beyond the scope of this article. Simply stated, the applicant filed three provisional patent applications on the separate interactions to establish an actual-reduction-to-practice date as each separate advance or milestone was demonstrated. In addition, including all three of the interactions if only two were beneficial could be viewed by the USPTO as overly burdensome by including interactions which are not effective. The primary takeaway is that a utility patent application may claim priority to multiple provisional patent applications.
Background and Detailed Description of the Invention
The “BACKGROUND” section of the patent application describes problems in the state of the art related to electrochemical machining. Specifically,
“Electrochemical machining (ECM) is a non-mechanical process for removing metal at an atomic level in an electrolytic
cell. ECM is useful for machining hard, high strength, wear resistance metals that are difficult and expensive to shape using traditional methods. In addition, ECM is also useful to machine irregular shapes such as dies and molds which do not respond well to traditional machining methods. The electrolytic cell in an ECM process consists of a tool electrode (cathode) and a work piece electrode (anode) that are separated by an interelectrode gap (IEG)… In conventional ECM processes, the tool electrode is advanced towards the work piece electrode while an electric current passes through the electrolyte flowing through the IEG. This causes dissolution of the surface metal ions from the work piece into the electrolyte solution. Over time, accumulation of waste material, heat and exhaustion of the electrolytes in the IEG leads to unfavorable kinetics and reaction stoichiometry in the electrolytic cell. To remedy this, the electrolytic solution is flowed through the cell to remove the remove waste product and heat away from the IEG while supplying unreactive ions to maintain the electrically conductive path…a variety of technologies may be incorporated to improve the ECM process. One such method is to vary the current through the IEG. Pulsed ECM (PECM) processes employ a pulsed direct current (DC) electric field across the IEG to improve surface finish. Ultrasonic actuation of the tool or work piece may also be incorporated to improve the ECM process. By speeding the metal hydroxide byproduct out the inter-electrode gap through mixing action which helps overcome the usual mass transport limitations the material removal rate and current efficiency of the process is improved…Magnetic fields introduced in the electrochemical cell improve the conditions within the cell as well. When augmenting electrochemical cells, magnetic fields are typically generated from permanent magnets with changes in the magnetic field created using rotary motion of the magnets or work piece. When electromagnets are employed, they typically function using direct current (DC) or at very low frequency alternating current (AC).”
The “BACKGROUND” section concludes with a “need” statement,
“While it is currently known to combine two or more of these technologies to further improve the machining process, these efforts have primarily been focused on combining the individual effects without regard for any synergistic effects which may be realized. Accordingly, there is a need for an improved system and method for electrochemical machining.”
The “DETAILED DESCRIPTION” section of the patent application further describes the electrochemical machining invention as
“Provided herein is a system and method for combining the effects of an external magnetic field, ultrasonic motion and a pulsed electric field in an electrolytic cell to create sympathetic interaction among the waveforms to improve the electrochemical machining process. Precisely coordinating
Table I. Patent Applications Associated with the ‘839 US Patent.
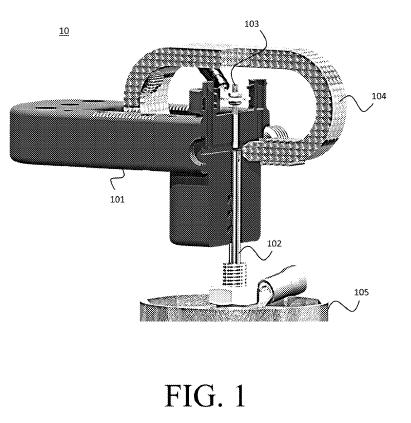
Fig. 1. field waveforms, ultrasonic motion, and pulsed electric field waveforms in an electrolytic cell to create a sympathetic interaction, in accordance with one illustrative embodiment of the invention…The system comprises a flow cell assembly 10 further comprising, a flow cell 101, a work piece (the anode) 102, a tool (the cathode) 103, and electric field generator, an electromagnet C-core 104, and an ultrasonic transducer 105
the coupled effects of simultaneously applying at least two of the three technologies, defined by an electric field waveform, a magnetic field waveform and an ultrasonic waveform, to the electrochemical cell improves the metal removal rate of the process and the surface finish of the resulting piece.
The coupled effects are termed a sympathetic interaction as they can be damped or amplified by modifying the phasing, frequency, orientation or amplitude of the waveforms.”
Several figures from the patent application further illustrate the subject invention.
The patent application included two independent claims and eighteen dependent claims. Independent Claim 1 and Dependent Claims 2, 3, 4, and 7 are reproduced herein.
1. An electrochemical machining technique comprising: a. interposing an electrolyte solution comprising ions through an interelectrode gap wherein the surface of a metallic work piece and an electrically conductive tool is exposed to the electrolyte solution in the interelectrode gap, b. generating a force on the ions in the electrolyte solution by simultaneously applying i. an electric field defined by an electric field waveform across the interelectrode gap, ii. an external magnetic field defined by a magnetic field waveform to the electrolyte solution, and iii. a relative ultrasonic actuation defined by an ultrasonic waveform to the electrically conductive tool wherein at least two of the electric field waveforms, the magnetic field waveform and the ultrasonic waveform, are coupled in a predetermined sympathetic interaction.
2. The technique of Claim 1 wherein at least two of the electric field waveform, the magnetic field waveform, and the ultrasonic waveform have the same frequency.
3. The technique of Claim 1 wherein at least two of the electric field waveform, the magnetic field waveform, and the ultrasonic waveform are coupled by having a predetermined phase difference.
(continued on next page)
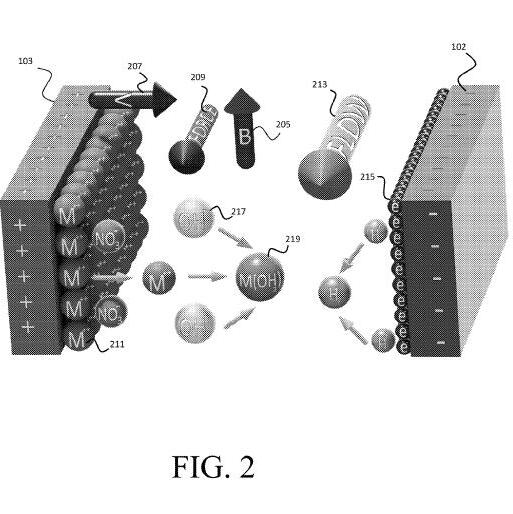
Fig. 2. An illustration of an anodic dissolution cell used in electrochemical machining, in accordance with an illustrative embodiment of the invention. An electric field is generated between the two charged electrodes, an anode by arrow which points in the direction of the magnetic field. The motion of ultrasonic actuation is represented by shown in FIG. 2, the ultrasonic velocity and electric field are parallel to one another, and both are orthogonal to the magnetic field. The resulting Lorentz force is generically represented by arrow 209, where the actual force would have a specific magnitude and direction unique to and centered on each ion. Metal ions 211 form part of the EDL [electrical double layer] on the anode 102. Electrolyte flow through the machining gap between the anode 102 and the cathode 103 is represented by arrow 213 Electrons 215 form part of the cathode 103. A typical anodic dissolution reaction is illustrated in FIG. 2 showing a metal ion 211 combined with hydroxide molecules 217 to form metal hydroxide molecules 219
3 from the ‘839 Patent Illustrating the Electrochemical Machining Invention.
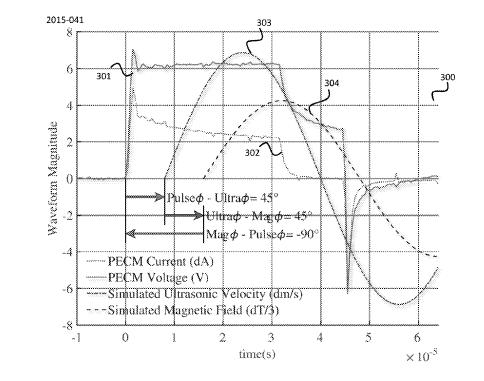
Fig. 3. An illustration of the PECM [pulse electrochemical machining] voltage waveform across the electrodes, the current waveform flowing into the cathode, the magnetic field waveform and the ultrasonic motion waveform, in accordance with one illustrative embodiment of the invention. For ECM processes in which sympathetic interactions were achieved by controlling the electric field waveform 301 and one or more of the magnetic field waveform 304 and the ultrasonic waveform 303, an electric field waveform 301, as represented by the waveform for the voltage across the electrodes, having an asymmetrical bi-polar electric pulse was generated. As shown in FIG. 3, the anodic duty cycle of the electric field waveform 301 is approximately 50% and the cathodic duty cycle is approximately 2% with a delay of 20% from the anodic pulse. For testing purposes, the rising edge anodic current pulse was set to be at a time zero when the phase shift of the electric field waveform 301 was at zero degrees. A ninety (90) degree shift in the phase of the electric field waveform 301 delays voltage across the cell by 16 microseconds.
Figure
Taylor and Inman
(continued from previous page)
4. The technique of Claim 1 wherein the electric field waveform and the ultrasonic waveform are coupled in a predetermined sympathetic interaction.
7. The technique of Claim 4 wherein the electric field waveform is a bipolar pulsed waveform.
In addition to the specification, the applicant submitted the filing fee and an inventor declaration signed by the inventor with the patent application.7 The declaration included an assertion by the inventor stating,
“As a below named inventor, I hereby declare that: …The below-identified application was made or authorized to be made by me.
I believe I am the original inventor or an original joint inventor of a claimed invention in the application… I hereby state I have reviewed and understand the contents of the above identified specification, including the claims, as amended by any amendment referred to above. I acknowledge the duty to disclose information which is material to patentability as defined in Title 37, Code of Federal Regulations, Section 1.56.”
The declaration included an acknowledgement that the inventor was aware of the penalties for a false statement,8
“I hereby declare that all statements made herein of my own knowledge are true and that all statements made on information and belief are believed to be true; and further that these statements were made with the knowledge that willful false statements and the like so made are punishable by fine or imprisonment of not more than five (5) years, or both, under 18 U.S.C. 1001 and that such willful false statements may jeopardize the validity of the application or any patent issued therefrom.”
Importantly, the “named inventor” must be correctly represented on a US patent application.9 Specifically, inclusion of a colleague as a co-inventor who did not participate in the conception of the invention is known as a misjoinder and may invalidate an otherwise valid patent. Similarly, exclusion of a co-inventor who participated in the conception is known as a nonjoinder and may invalidate an otherwise valid patent. If an inventor is erroneously omitted or erroneously included as an inventor, the misjoinder/nonjoinder may be corrected and the patent remains valid.10
Establishing and Maintaining a Filing Date
To establish a filing date, a utility patent application must include
1) Specification11
“…a written description of the invention, and the manner and process for making it…to enable any person skilled in the art…to make and use [the invention] …”
2) Minimum of one claim12
“…particularly pointing out… the subject matter…as the invention…”
3) Drawings13
“…where necessary for understanding the subject matter…to be patented…”
To maintain the filing date, the following additional criteria are required
1) Filing fee in accordance with the current USPTO fee schedule14
2) Inventor oath or declaration asserting15
a. The patent application was authorized by the inventor(s),
b. The inventor(s) believe he/she is the original inventor or they are the original joint inventors.
As summarized above, the specification included a background and summary of the invention describing various embodiments of the invention. The patent application also included drawings illustrating the “elements” of the subject invention. The utility patent application contained claims directed toward the electrochemical method along with the filing fee and the inventor oath.
The filing of the patent application and associated documents met the met the requirements to both establish and maintain a filing date and thereby avoided being abandoned. On October 21, 2016, the USPTO issued a filing receipt and assigned the patent application no. 15/290,258.
Inventor Assignment and Power of Attorney
The inventor was employed by GE Global Research at the time of the invention. Because the inventor was Principal Investigator on a US Army contact awarded to GE Global Research, the patent application was assigned to the US Government as Represented by the Secretary of the Army. The patent application included a statement asserting the “rights of the government,” specifically
“The inventions described herein may be manufactured and used by or for the United States Government for governmental purposes without payment of any royalties.”
The patent application included a statement that the applicant appointed patent attorneys/agents employed by the US Army to prosecute the patent application at the USPTO.
Information Disclosure Statement
The applicants submitted an “Information Disclosure Statement” (IDS) to the USPTO after filing the patent application and prior to the first office action addressing patentability. The IDS included prior art references, including those of the inventors as required by the “Duty of Candor.” The “Duty of Candor” requires that the inventor(s) submit an IDS within a reasonable time of submission of the patent application disclosing16
“…to the Office [USPTO] all information known to that individual to be material to patentability…”
The “Duty of Candor” is specific to any existing claim and requires that the IDS be continually updated while the claim is pending. The “Duty of Candor” ceases only when the claim is allowed and the patent issue fee is paid.
The “Duty of Candor” extends to any individual associated with the filing of the patent application including
1) Inventor(s),
2) Patent Counsel, or
3) Persons who are substantially involved in the preparation or prosecution of the patent application.
Substantial involvement in the preparation of the patent application could include technical assistants, collaborators, or colleagues. Substantial involvement would generally not extend to clerical workers. Furthermore, the inclusion of a reference in an IDS17
“…is not taken as an admission that the reference is prior art against the claims.”
If a finding of a violation of the “Duty of Candor” resulting in “inequitable conduct” regarding any claim in a patent is determined, then all the claims of the subject patent are rendered invalid.18 Finally,
in spite of the requirement of the “Duty of Candor,” the applicant is cautioned to not “bury” the examiner with a long list of non-material references in hopes that the examiner will not notice the relevant material references.19 The specific guidance from the USPTO is to20
“…avoid the submission of long lists of documents if it can be avoided…If a long list is submitted, highlight those documents which have been specifically brought to the applicant’s attention and/or are known to be of most significance.”
Publication
The USPTO began publishing patent applications 18 months after their priority date for patent applications filed on or after November 29, 2000. Because the subject patent application was filed after this date, it would normally be published 18 months after its priority date of October 8, 2015. An exception to the 18-month publication rule is if the applicant states that the patent application will not be filed21
“…in another country, or under a multilateral international agreement, that requires publication of application 18 months after filing…”
The applicant requested a nonpublication exception on October 11, 2016 with the patent application filing documents.
Non-Final Office Actions
On September 12, 2018, the USPTO issued a non-final office action (NF-OA) rejecting all the claims in the patent application as being “obvious” in view of the prior art22
“A patent may not be obtained… if the differences between the subject matter sought to be patented and the prior art are such that the subject matter as a whole would have been obvious at the time the invention was made to a person having ordinary skill in the art...”
The patent application was not rejected for lack of “novelty,” which is23
“…the invention was patented or described in a printed publication in this or a foreign country or in public use or on sale in this country, more than one year prior to the date of application for patent in the United States.”
The obviousness rejections were based on multiple prior art references and can be challenging to overcome. Specifically, the obvious rejections were based on three prior art patents, one cited by the applicant and two patents identified in the patent examiner’s search. The three patents were used in combination to reject the independent claims as well as the limitations included in the dependent claims. Generally, obviousness rejections are overcome by adding limitations from dependent claims or from the specification to the rejected claims.24
Applicant Response/Allowance of Patent Application
On December 12, 2018, the applicant submitted amended claims and arguments to the USPTO with the intent of overcoming the obviousness rejections. The applicant added the limitations of Dependent Claims 2, 3, 4, and 7 into Claim 1. In addition to the dependent claim limitations, the applicant included that the intent of the sympathetic interaction is to induce a “Lorenz” force on the ions of the electrolyte solution. In essence, the inventors copied the limitations of Dependent Claims 2, 3, 4, and 7 along with the Lorenz force into Independent Claim 1. This narrowed or limited the breadth of Independent Claim 1 and with the subject limitations overcame the obviousness rejections. The applicant cited the paragraphs in the
patent application enabling the Lorenz force addition. The amended Claim 1 is reproduced herein with the inserted text depicted in [brackets].
1. An electrochemical machining technique comprising:
a. interposing an electrolyte solution comprising ions through an interelectrode gap wherein the surface of a metallic work piece and an electrically conductive tool is exposed to the electrolyte solution in the interelectrode gap,
b. generating a [Lorentz] force on the ions in the electrolyte solution by simultaneously applying i. an electric field defined by [bi-polar pulsed] electric field waveform across the interelectrode gap, ii. an external magnetic field defined by a magnetic field waveform to the electrolyte solution, and iii. a relative ultrasonic actuation defined by an ultrasonic [velocity] waveform to the electrically conductive tool wherein at least two of the [bi-polar pulsed] electric field waveform, the magnetic field waveform, and the ultrasonic [velocity] waveform are coupled in a predetermined sympathetic interaction [to influence the Lorentz force on the ions].
On March 8, 2019, the USPTO issued a notice of allowance. The amended Independent Claim 1 allowed the applicants to overcome the “obviousness” rejections. After payment of the issue fees, the patent application issued as US Patent No. 10,357,839 on July 23, 2019.
Summary
In this installment of the “Looking at Patent Law” series, we present a case study of the prosecution of US Patent No. 10,357,839; “Method for Electrochemical Machining using Sympathetic Waveform Interactions.” The ‘839 patent issued on July 23, 2019 with inventor Curtis Bradley. The assignee of the patent at time of issue was The United States of America as Represented by the Secretary of the Army. The case study begins with a brief synopsis of the background of the invention followed by 1) summary of several drawings and the specification of the invention, 2) inventor assignment and power of attorney designations, 3) submission of the Invention Disclosure Statement (IDS) and associated Duty of Candor, 4) summary of the non-final office action rejecting the patent application for obviousness, and 5) applicant response and allowance of the patent application. The case study illustrates the use of adding limitations from the dependent claims and the specification to the independent claim to overcome an obviousness rejection. In addition, the case illustrates an applicant-initiated nonpublication exception to the 18-month publication rule. With this case study, we hope to de-mystify the patent prosecution process and better prepare electrochemical and solid-state scientists, engineers, and technologists to interact with their patent counsel regarding their inventions.
©The Electrochemical Society. DOI: 10.1149/2.F0X243IF
About the Authors
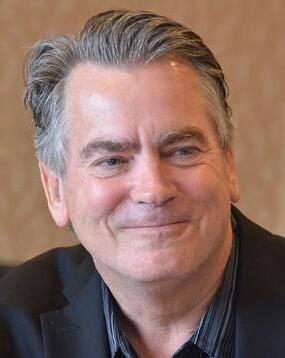
E. Jennings Taylor, Founder of Faraday Technology, Inc.
Research Interest: Founder of Faraday Technology, Inc., a small business focused on developing innovative electrochemical processes and technologies based on pulse and pulse reverse electrolytic principles. Until his recent retirement, Taylor led Faraday’s patent and commercialization strategy and negotiated (continued on next page)
Taylor and Inman
(continued from previous page)
numerous patents via field-of-use licenses as well as patent sales. Taylor continues to serve as Strategic Advisor to Faraday as well as other clients. He is admitted to practice before the United States Patent & Trademark Office (USPTO) in patents cases as a patent agent (Registration No. 53,676). He is a Member of the American Intellectual Property Law Association (AIPLA).
Pubs & Patents: Numerous technical pubs and presentations, inventor on approximately 100 patents/patent application.
Work with ECS: Member for 42 years, ECS Fellow.
4. 35 U.S.C. §111(b)(2) Provisional Application/Claim.
5. 35 U.S.C. §111(b)(5) Provisional Application/Abandonment.
6. 35 U.S.C. §119(a) Benefit of Earlier Filing Date; Right of Priority.
7. 37 CFR 1.63 Inventor’s Oath or Declaration.
8. 18 U.S.C. §1001Statements or Entries Generally.
9. E. J. Taylor and M. Inman, Electrochem. Soc. Interface, 26(2), 45 (2017)
10. Manual of Patent Examination Procedure (MPEP) §1481.02 Correction of Named Inventor.
11. 35 U.S.C. §112(a) Specification/In General.
12. 35 U.S.C. §112(b) Specification/Conclusion.


Website: http://www.faradaytechnology.com/ https://orcid.org/0000-0002-3410-0267
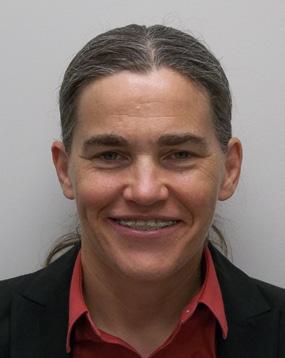
Maria Inman, Vice President, Faraday Technology, Inc.
Education:
Work Experience: Dr. Inman manages Faraday Technology’s pulse and pulse reverse research project portfolio and business development activities.
Pubs + Patents: >108 publications and 7 patents.
Work with ECS: Member for 27 years, Chair of the IE&EE Division.
13. 35 U.S.C. §113 Drawings.
14. https://www.uspto.gov/learning-and-resources/fees-andpayment/uspto-fee-schedule#Patent%20Fees
15. 35 U.S.C. §115(b)(1)(2) Inventor’s Oath or Declaration/ Required Statements.
16. 37 CFR §1.56(a) Duty to Disclose Information Material to Patentability.
17. Riverwood Int’l Corp. v. R.A. Jones & Co., 324 F.3d 1346, 135455, 66 USPQ2d 1331, 1337-38 (Fed Cir. 2003).
18. Manual of Patent Examination Procedure (MPEP) §2016 Fraud, Inequitable Conduct, or Violation of Duty of Disclosure Affects All Claims
19. R. B. Taylor, Mich Telecom & Tech Law Rev, 19, 99 (2012).


Website: http://www.faradaytechnology.com/. https://orcid.org/0000-0003-2560-8410
References
1. E. J. Taylor and M. Inman, Electrochem Soc Interface 26(4), 57 (2017).
2. Patent Center
3. C. Bradley “Method for Electrochemical Machining using Sympathetic Waveform Interactions” US Patent No. 10,357,839 issued July 23, 2019.
20. Manual of Patent Examination Procedure (MPEP) §2004.13 Aids to Comply with Duty of Disclosure.
21. 35 U.S.C. §122(b)(2)(B)(i) Confidential Status of Applications; Publication of Patent Applications.
22. 35 U.S.C. §103(a) (pre-AIA) Conditions for Patentability; Nonobvious Subject Matter.
23. 35 U.S.C. §102(b) (pre-AIA) Conditions for Patentability; Novelty and Loss of Right to Patent.
24. E. J. Taylor and M. Inman, Electrochem Soc Interface, 26(3), 39 (2017)
2024 ECS INSTITUTIONAL PARTNERS
BENEFACTOR PATRON
BioLogic (16)*
Duracell US Operations, Inc. (67)
Gamry Instruments (17)
Gelest Inc. (15)
Hydro-Québec (17)
PalmSens BV (1)
Pine Research Instrumentation (18)
SPONSORING
BASi (9)
Center for Solar Energy and Hydrogen Research Baden-Württemberg (ZSW) (20)
Central Electrochemical Research Institute (31)
Corteva Agriscience (2)
DLR – Institute of Engineering Thermodynamics (16)
EL-CELL GmbH (10)
Electrosynthesis Company, Inc. (28)
Ford Motor Company (10)
GS Yuasa International Ltd. (44)
Honda R&D Co., Ltd. (17)
Medtronic, Inc. (44)
Nel Hydrogen (2)
Nissan Motor Co., Ltd. (17)
NSF Center for Synthetic Organic Electrochemistry (3)
Pacific Northwest National Laboratory (PNNL) (5)
Panasonic Energy Corporation (29)
Permascand AB (21)
Plug Power, Inc. (3)
Teledyne Energy Systems, Inc. (25)
UL Research Institutes (3)
Energizer Battery (79)
Faraday Technology, Inc. (18)
GE Global Research Center (72)
Lawrence Berkeley National Laboratory (LBNL) (20)
Scribner, LLC (28)
Toyota Research Institute of North America (TRINA) (16)
SUSTAINING
Current Chemicals (2)
General Motors Holdings LLC (72)
Giner, Inc. (38)
Initial Energy Science & Technology Co., Ltd (IEST) (1)
Ion Power, Inc. (10)
Kanto Chemical Co., Inc. (12)
Los Alamos National Laboratory (LANL) (16)
Metrohm USA, Inc. (10)
Microsoft Corporation (7)
Occidental Chemical Corporation (82)
Sandia National Laboratories (48)
Sensolytics GmbH (1)
Sherwin-Williams (3)
Spectro Inlets ApS (2)
Technic, Inc. (28)
United Mineral & Chemical Corporation (3)
Western Digital GK Corporation (10)
Westlake Corporation (29)
Help us continue the vital work of ECS by joining as an institutional partner today.
To renew, join, or discuss institutional partnership options please contact Anna Olsen, Sr. Manager, Corporate Programs, sponsorship@electrochem.org
Residual
NMP and Its Impacts on Performance of Lithium-Ion Cells
N-methyl-2-pyrrolidone (NMP) is a common industrial solvent that is often used when slurry casting Li-ion battery electrodes because it dissolves the binder PVDF. A research team at Dalhousie University has studied the effects of trace amounts of residual NMP in battery cathodes. They examined commercially produced pouch cells with cathode active materials LiMn0.8Fe0.2PO4 (LMFP) and NMC532 and found that there was measurable residual NMP in the LMFP cells but not the NMC532 cells. This was due to the high surface area of LMFP (26 m2/g) as opposed to NMC532 (<1 m2/g), which results in more difficult drying. Subsequent experiments, in which electrolyte was purposefully spiked with NMP to assess the effect on performance, showed that NMP impacts cycling of NMC532 cells. In small amounts, NMP had no obvious effect on capacity retention, but increased the voltage polarization. It was also found that NMP can react with electrolyte additives. In cells with a typical electrolyte formulation with additives vinylene carbonate (VC) and ethylene sulfate (DTD), NMP was seen to decompose DTD, thereby changing the electrolyte chemistry. These results demonstrate that care must be taken to drive off NMP during battery fabrication.
From: M. Yue, S. Azam, N. Zhang, et al., J. Electrochem. Soc., 171, 050515 (2024).
Turn-Off Fluorescent Sensor for Metal Ions Quantifies Corrosion in an Organic Solvent Assessment and prediction of corrosion of metals in situ in non-aqueous solutions can be challenging due to the low conductivity of the solution. To determine corrosion rates in these types of solutions, researchers at Case Western Reserve University recently investigated the use of fluorescent sensors in quantifying corrosion of AISI 1045 steel and aluminum in an ethanol-based solution. Fluorescent sensors have broad use in biological and environmental sensing applications and have been used sparingly for corrosion detection, such as through the incorporation of these compounds into coatings. For corrosion detection in this manner, they have mostly served as an “onoff” switch, tied to enhanced fluorescence associated with oxidation and early-stage corrosion occurring under the coating. In this work, researchers used Phen GreenSK (PGSK) as the fluorescence indicator and correlated the fluorescence response of PGSK with electrochemically determined corrosion rates (10% water was added to the ethanol mixture to ensure the necessary conductivity). Early results demonstrated the promise of this technique; however, future work should include a more in-depth investigation into potential interactions
TECH HIGHLIGHTS TECH HIGHLIGHTS
between chemical species. Enhanced instrumentation could further decrease the concentration of the fluorescent compound needed for corrosion detection.
From: L. Liu, Z. Pfaffenberger, M. Siegel, et al., J. Electrochem. Soc., 171 051502 (2024).
A Redox-Electrodialysis Model with Zero Fitting Parameters: Insights into Process Limitations, Design, and Material Interventions
A multitude of factors has led to water scarcity and the desalination of water has become a critical need. While reverse osmosis–based systems can support large plants, electrochemical-based desalination helps reduce the energy consumption for distributed systems. Further reduction in energy consumption can be achieved with redox electrodialysis. A research team at The Pennsylvania State University has carried out both experimental and model development work for a redox electrodialysis system. The model developed covers both thermodynamic and kinetic aspects, without any fitting parameters. The study helped authors to conclude that ion depletion in the diluate stream led to higher resistance, and hence the energy consumption. With this insight, the team implemented and confirmed the effectiveness of ion exchange resin wafers to reduce the ionic resistance in the diluate stream and found an 18% reduction in energy consumption. In addition, the model helped analyze and optimize the stack configuration for lowering energy consumption, with multiple cells in series being more energy efficient than a single large cell. The developed and experimentally validated model provides a tool for practitioners to carry out design tradeoff and technoeconomic analyses.
From: T. C. Hudak, C. A. Gorski, and C. G. Arges, J. Electrochem. Soc., 171, 053502 (2024).
A Highly Synergistic Dual-Cathode Electro-Fenton Process with SelfRegulation of pH and Coating Stability for the Effective Degradation of Refractory Pollutants
In an electro-Fenton process where Fe2+ and H2O2 are obtained from electrolysis, two main challenges affect the radical generation efficiency. The first is the stringent requirement of the solution pH. The other is the competition for active sites on the same electrode between the oxygen reduction (to generate H2O2) and the H2O2 activation reactions. Researchers from Hebei University of Environmental Engineering of China recently reported the use of a dualcathode design to address these challenges. In their system, the authors utilized a carbon cloth anode to oxidize water to form oxygen. The electrons harvested were distributed to two cathodes— one of biochar-coated steel to reduce oxygen to H2O2; and the other of FeOCl/carbon-cloth to regenerate Fe2+ from
Fe3+. The competition for electrode active sites mentioned earlier was thus avoided. In addition, the pH of the whole solution was found to self adjust to a stable benign range of 3.0–4.5, even if the starting pH of the solution ranged from 3 to 9. Compared with the corresponding single cathode setups using the same electrode materials, the dual-cathode system showed much better efficiency in degrading several common organic refractory pollutants.
From: J. Zhao, J. Zhang, Z. An, et al., J. Electrochem. Soc., 171, 046507 (2024)
Electrochemical Supercapacitor Application of Electrochemically Produced Chlorine-Doped Graphene Oxide with Cobalt Sulfide-Based Compounds Produced from Recycling of Spent Li-Ion Batteries
With depleting natural resources and increasing costs, cobalt has been identified as a critical raw material (CRM) by many global organizations, including the European Union. As the use of Li-ion batteries has increased in recent decades, so too has the number of spent cells that are disposed of every year. The extraction of Co from spent Li-ion cells is a promising approach to address supply chain shortages of this CRM. To this end, researchers from Yıldız Technical University and Çankırı Karatekin University have recently reported a novel method to extract Co from spent commercial Li-ion batteries and repurpose the Co into a cobalt sulfidebased compound. This material was then processed into a composite with chlorinedoped graphene oxide to form a positive electrode material for supercapacitors. The resulting supercapacitors demonstrated an impressive areal capacitance of 101 mF cm−2 at a scan rate of 10 mV s−1. This report offers valuable insights into a potentially scalable pathway toward the extraction of Co from spent Li-ion cells and demonstrates the application of the recovered Co as a supercapacitor material.
From: S. Yasa, B. Birol, and M. Gencten, J. Solid State Sci. Technol , 13, 041007 (2024).
Tech Highlights was prepared by Joshua Gallaway of Northeastern University, Mara Schindelholz of Sandia National Laboratories, David McNulty of University of Limerick, Zenghe Liu of Abbott Diabetes Care, Chock Karuppaiah of Vetri Labs and Ohmium International, and Donald Pile of EnPower, Inc. Each article highlighted here is available free online. Go to the online version of Tech Highlights in each issue of Interface, and click on the article summary to take you to the full-text version of the article.
Battery Safety
by Paul R. Shearing and Thomas P. Barrera
When we engage in discussions about lithium-ion batteries (LIBs), one of the most common questions asked by our colleagues, friends, and family is “Are lithium-ion batteries safe?” The answer to this important question is, for the individual, “Yes.” Despite the ubiquity of LIBs in our daily lives, the failure rates of these batteries are sufficiently low such that the chances of a failure affecting an individual using a battery for its intended purpose are vanishingly small. However, there is no room for complacency, as over 25 years of field experience has shown that there are inherent safety risks associated with LIB technologies. These are commonly related to in-service abuse by consumers, LIBs manufactured with poor quality and/or poor manufacturing controls, and many other factors.
Before the commercialization of LIB technology, the traditional electrochemical science community perceived battery safety as a user-driven requirement. That is, battery users had the primary responsibility of ensuring that cells and batteries were safely integrated, stored, and operated within the battery manufacturers’ specification requirements. However, lessons-learned emanating from root-cause analyses of multiple catastrophic LIB-related industry system safety incidents has shown that battery safety not only includes user-induced abuse avoidance, but also includes adopting a rigorous safe-by-design approach to the design and manufacture of LIBs. More recently, the increasing quantity of marketed counterfeit and substandard Li-ion cells and batteries has created a new safety risk to the LIB user community. The increase in counterfeit and substandard batteries is expected to continue as the global manufacturing base evolves, supply chain participants become more diverse, and consumer demand for LIBs grows.
Motivated by the global visibility of the 2013 Boeing Co. 787 Dreamliner™ commercial passenger aircraft LIB safety incidents, the electrochemical and solid-state science community has prioritized fundamental research and development into developing new materials, safety devices, manufacturing protocols, and advanced test methods which mitigate the likelihood and consequences of LIB safety hazards. For over 20 years, the bi-annual ECS meetings have supported Battery Division safety-related symposia focused on topics such as battery abuse tolerance, failure modes, reliability, and characterization. During this period, a variety of safety hazards associated with new rechargeable and non-rechargeable lithium battery technologies have been characterized over a wide range of operating conditions. Nevertheless, catastrophic LIB failures continue to proliferate in virtually all known applications, including the commercial portable electronics, electrified transportation, gridenergy storage, marine, and aviation industries. Tensioned against the sheer volume of batteries now in operation, the fraction of batteries failing in the field remains low; however, with the rapid uptake of batteries in increasingly demanding applications, exercising diligence in their continued safe deployment is mandatory. As new high energy density batteries emerge, and new sectors and geographies adopt them, batteries will also play a central role in the pursuit of net-zero emissions. To ensure this is achieved safely, our ECS community must continue to collaborate with industry to continue fundamental and applied research into safer battery technologies. In addition, the growing widespread commercialization of high specific energy lithium battery technologies has increased the need for heightened user awareness of the inherent safety risks of LIBs.
This issue of Interface features invited contributions from internationally recognized LIB safety subject matter experts from industry and academia. The first article, “Insights into Thermal Runaway of Cylindrical Lithium-ion Batteries by Internal Temperature Sensors,” discusses LIB thermal runaway safety hazards in terms
of characterizing the thermal characteristics of new commercially available lithium-ion cell designs. The authors propose a novel means to conduct in-situ measurements of nickel-rich 21700 lithiumion cell temperature gradients using embedded temperature sensors and advanced accelerating rate calorimetry techniques. These data are used to better understand the effects of lithium-ion cell aging on safety in support of second-life LIB needs. The second contribution, “Achieving Passive Thermal Runaway Propagation Resistance in Liion Battery Packs,” presents a recently developed set of key design guidelines intended to minimize catastrophic cell-to-cell thermal runaway propagation risk in multi-cell LIB packs. Manufactured from cylindrical 18650 or 21700 cells, these new guidelines were derived from qualified and deployed propagation-resistant LIB designs supporting crewed NASA spacecraft and other safetycritical applications. The authors also share key lessons learned from design approaches, manufacturing methods, testing protocols, and modeling techniques used to qualify propagation-resistant LIBs. The third contribution, “Modeling of Li-ion Battery Thermal Runaway Insights into Modeling and Prediction,” recognizes the complex nature of lithium-ion cell thermal runaway characteristics in terms of developing predictive modeling methodologies. The fundamentals of physics-based and reduced-order models are introduced and described with special attention to quantifying key modeling parameters for predicting cell temperature during thermal runaway. The authors propose modeling pathways toward utilizing machine learning and artificial intelligence to further modeling efficacy. The fourth paper, “A Multi-scale Framework for Advancing Battery Safety Through Early Calorimetric Analysis of Materials and Components,” is a novel strategy intended to identify material-level characteristics during the early stages of new cell design development. Early characterization of key cell-level material properties is proposed as an approach to mitigate safety hazards prior to cell-level scale-up. The final paper in our collection “High-Speed Imaging for Investigating Battery Failure Mechanisms” provides an overview of the latest developments using ultra-high-speed X-ray techniques to visualize the thermal runaway process, revealing new insights into the internal structure of batteries during the aggressive failure process.
We hope that the collection of articles in this issue will serve as a touchstone for the battery safety community, revealing new insight into this important field of research.
©The Electrochemical Society. DOI: 10.1149/2.F06243IF
About the Authors
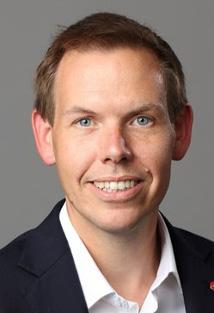
Paul R. Shearing, Professor of Sustainable Energy Engineering at the Department of Engineering Science and Director of the ZERO Institute at Oxford University
Education: Masters in Chemical Engineering (Birmingham University), PhD (Imperial College London, UCL)
Research Interests: Cover a broad range of electrochemical engineering themes with a particular interest in the characterization and understanding of materials for batteries, fuel cells, and other energy applications.
Work Experience: Chair in Sustainable Energy Engineering, University of Oxford (2023–), Professor in Chemical Engineering, UCL (2012–23), Royal Academy of Engineering Chair in Emerging Battery Technologies (2018–)
Pubs + Patents: >450 peer reviewed scientific papers, 1 patent
(continued on next page)
Shearing and Barrera (continued from previous page)
Research Interests: Electrochemical engineering, Clean energy sources, Physical electrochemistry
Work Experience: President and owner of LIB-X Consulting, where he provides engineering, expert witness, and educational services in the broad area of LIB power systems. Previously, Tom was a Technical Fellow for The Boeing Co. where he led multidisciplinary teams in the end-to-end development of advanced electrical power and battery energy storage systems for high-value government and commercial spacecraft applications.


Awards: Royal Academy of Engineering Chair in Emerging Battery Technologies. Founding investigator of The Faraday Institution, the UK’s independent institute for electrochemical energy storage research where he leads the LiSTAR and Safebatt research programs. 2022 Royal Academy of Engineering Silver Medal. Work with ECS: Member since 2009 Websites: www.zero.ox.ac.uk https://eng.ox.ac.uk/people/paul-shearing/ https://orcid.org/0000-0002-1387-9531
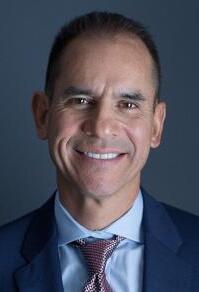
Thomas P. Barrera, President, LithiumIon Battery (LIB)-X Consulting Education: BS in chemical engineering (cum laude) and BA in mathematics-economics (UCSB), MS in industrial engineering (Northwestern University), PhD in chemical engineering (UCLA, under the direction of Ken Nobe), postdoctoral research fellow in materials science and engineering (UCLA, under the supervision of Bruce Dunn)
Pubs + Patents: Editor-in-chief and contributor, Spacecraft Lithium-Ion Battery Power Systems, John Wiley & Sons (IEEE Press, 2023); 3 US patents in aviation battery safety; >50 combined publications and conference presentations.
Awards: AIAA Associate Fellow.
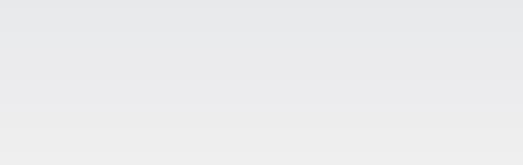
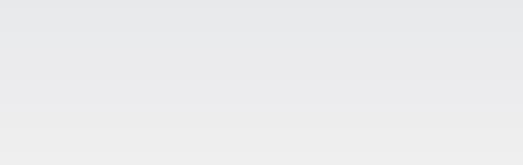
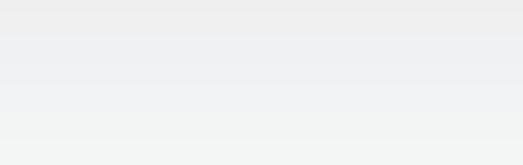
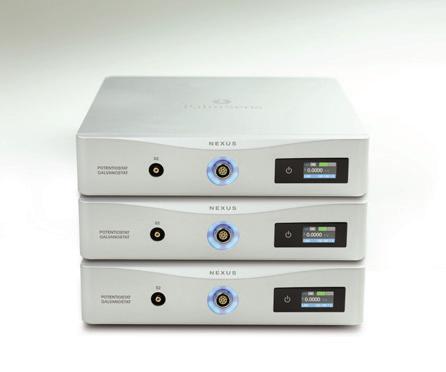
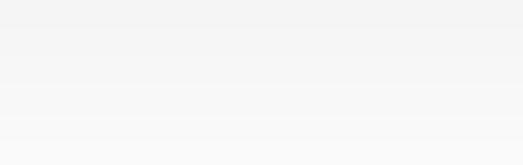
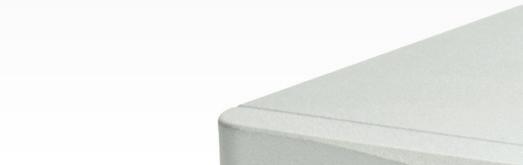
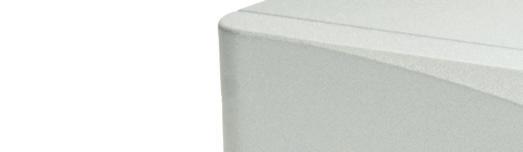
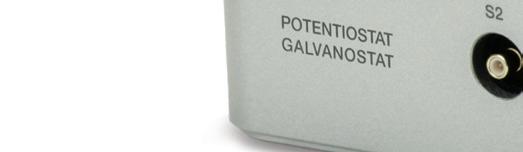
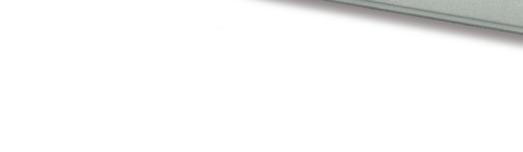
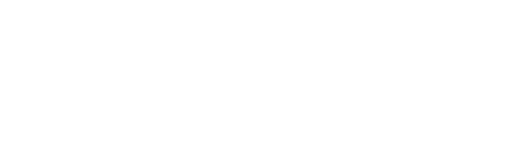
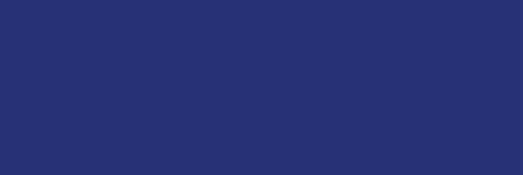
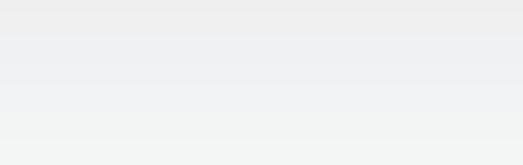
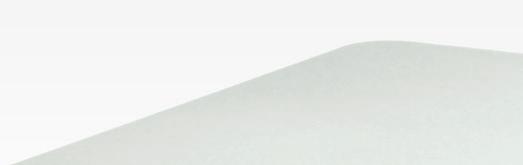
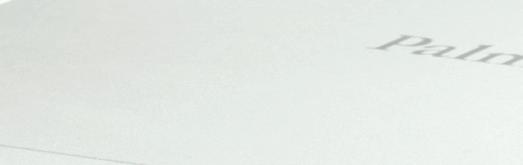
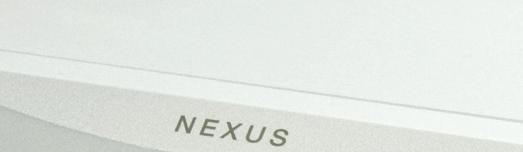
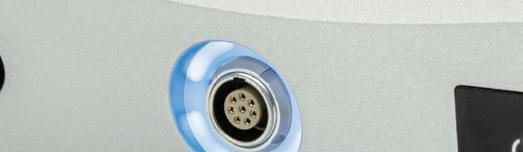
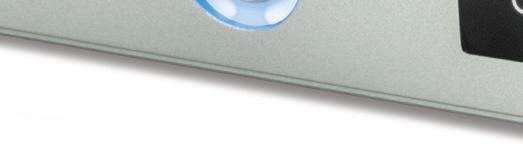
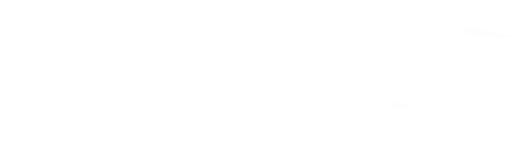
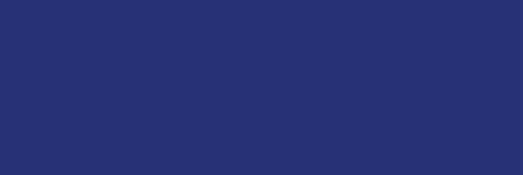
Work with ECS: Life Member; Member-at-Large, Battery Division; Institutional Engagement Committee; Symposia Lead, Battery Safety and Failure Modes (234th, 235th, & 244th meetings). Website: https://www.libxconsult.com/ https://orcid.org/0000-0001-8311-1889


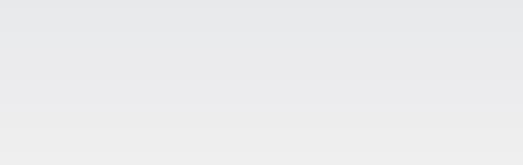
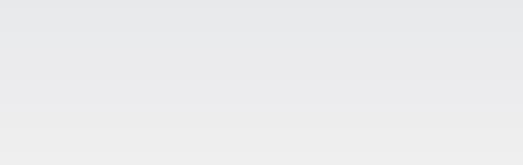
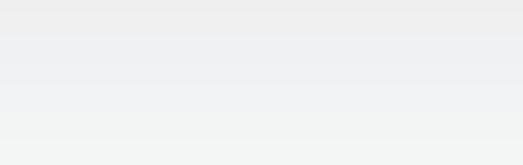
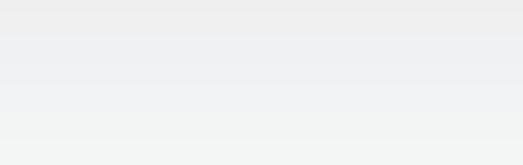
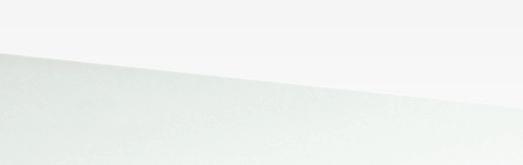
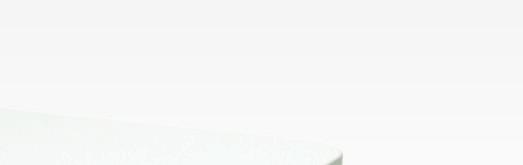
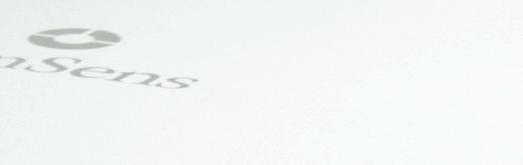
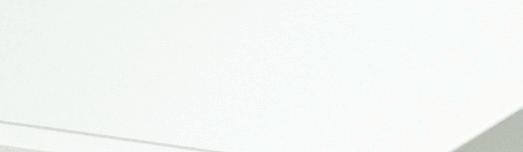
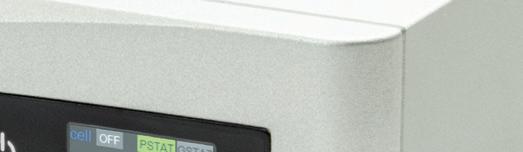
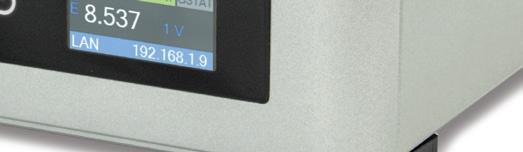
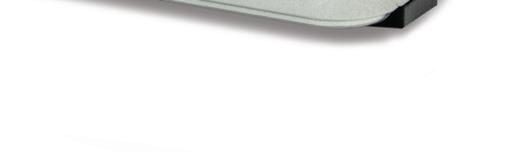
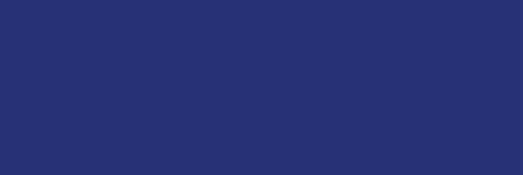
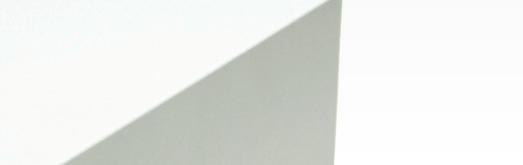
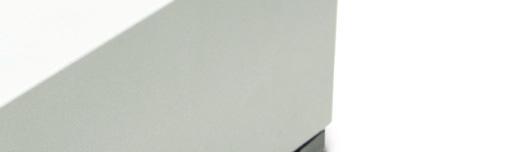
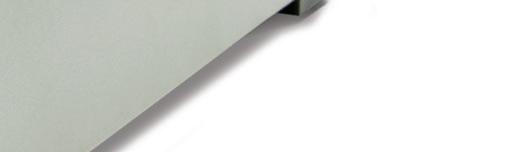
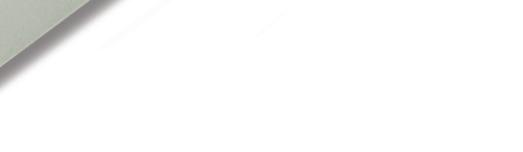













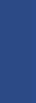
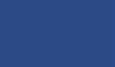
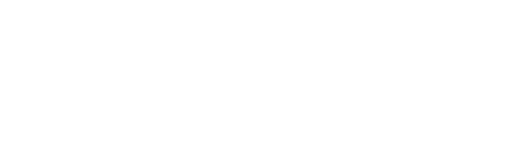




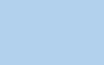

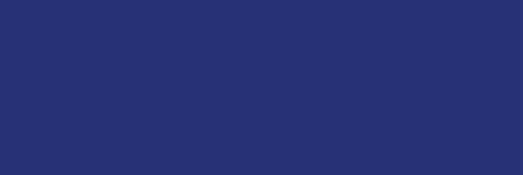
Insights into Thermal Runaway of Cylindrical Lithium-ion Batteries by Internal Temperature Sensors
by Max Feinauer, Markus Hölzle, and Thomas Waldmann
Li-ion batteries (LIBs), recognized by the Nobel Prize awarded to John B. Goodenough, M. Stanley Whittingham, and Akira Yoshino in 2019,1 are the backbone of everyday electronic devices such as smartphones, laptops, electric vehicles, and grid-scale energy storage systems.2 Advantages of LIBs over other battery chemistries are their high energy density, specific energy, fast-charging capability, high cell voltage, low self-discharge rate, and long cycle life.
State-of-the-art commercial off-the-shelf cells typically contain graphite/silicon anodes, Ni-rich cathodes, a polyolefin separator, and a carbonate-based organic electrolyte with LiPF6 as conductive salt. As for all electrochemical energy storage devices, safety is a requirement also for LIBs.3–16 The total energy content of LIBs consists of the stored electrochemical energy, which varies with the state-of-charge (SOC) in addition to the chemical combustion energy of its components—both of which are relevant to safety.17,18
At the cell level, commercial 18650 and 21700 cylindrical cells contain the following safety features in their cap: (i) a current interrupt device (CID), which in addition acts as a burst disc, and (ii) a positive temperature coefficient (PTC) resistor—both of which are often at the positive terminal of the cell (see Fig. 1b).5, 19 When the pressure inside a cell due to gas-producing side reactions exceeds a threshold, the CID opens and interrupts the current followed by venting of the cell, releasing the gas into the environment.11, 19 Additionally, LIB cells often utilize a shutdown separator, which prevents ionic conduction at increased temperatures.9 At the battery system level, propagation of thermal runaway (TR) from cell-to-cell has to be considered. TR propagation from cell-to-cell can be mitigated by optimized system design13 and by thermal propagation barriers that are added in between individual cells.20
If LIB cells are used beyond their specified limits (i.e., under abuse conditions), the involved materials will become thermally unstable, leading to a self-heating of the cell, which may ultimately result in a TR condition. Therefore, it is of utmost importance to fully characterize all safety-relevant issues in LIB cells and detect them as early as possible.16 A commonly used empirical method for testing the thermal safety of LIB is accelerating rate calorimetry (ARC).3–11 In ARC tests, the temperature of the calorimeter is increased (typically in steps of 5 °C) until a defined self-heating rate (> 0.02 °C min-1) of the cell is detected that arises from internal exothermic reactions. Subsequently, the calorimeter follows the cell temperature providing quasi-adiabatic conditions. This implies that the cell is unable to dissipate the generated heat into its environment and is instead forcing the cell into TR. In addition to monitoring the temperature, the cell voltage is also recorded throughout the ARC test. As the ARC experiment progresses, different safety-relevant features occur, including venting, separator melting, and finally TR, where the maximum temperature occurs.3,4,10 Sensors positioned outside the cell—for example, ultrasonic transducers, strain gauges, mass-spectrometry, or an audio recorder—in combination with ARC provide valuable insights into TR characteristics.3–5
A particular focus of interest is the temperature within LIB cells.14,15,21–25 Different groups approached the measurement of cell internal temperatures by thermocouples both during standard operations and under abusive conditions.21,22,24–28 Parameters that influence the maximum cell temperature during discharge are time,21,25,29–33 C-rate,21,25,29–33 cell volume/surface ratio,25,31 electrode thickness,25 and tab design.32,33 During non-abusive discharge, the temperature inside the cells is typically higher compared to the cell
surface.21,23–25,30,32,34,35 For instance, a high-power 18650-type cell showed maximum temperatures of 66 °C on the cell surface and 92 °C inside the cell during a continuous constant-current discharge at 16 C (24 A).21 In this example,21 these high temperatures did not lead to a safety-critical event, because the generated heat could be dissipated to the cell environment. However, there is a lack of systematic measurements of internal cell temperature during TR in ARC tests. In addition, most existing approaches incorporate sensors during cell manufacturing only, which limits investigations to custom-built pilot cells. By contrast, the approach used in this work is applicable to both commercial and custom-built cells.
Commercially available state-of-the-art high-energy 4.85 Ah 21700-type LIB cells with graphite/silicon anodes and Ni-rich cathodes in ARC experiments at various SOCs were studied. The cells were equipped with an internal and an external temperature sensor for operando measurements during TR. The aim of this work was to gain insights into TR behavior and the corresponding temperature gradients. Based on the measured data, an outlook on the application of this technique to aged cells is discussed.
Implementation of Temperature Sensors in Commercial Cells
Fig. 1(a) illustrates the methodology of equipping a cylindrical cell with an internal thermocouple. For safety reasons, all the following steps were carried out on a fully discharged cell at the lower cut-off voltage given in the manufacturer’s data sheet (2.5 V). In the initial step, a laser is employed to create a hole in the center of the bottom of the cell housing. To prevent the temperature of the cell in the vicinity of the laser beam from reaching a critical level, the temperature is permanently controlled. Alignment of the laser focal plane with the surface of the cell housing is of paramount importance. Alignment prevents the electrodes inside the cell from being affected by the laser since the laser beam diverges below the focal point (Fig. 1(a)). Additional advantages of the laser over conventional drilling22 are that the laser does not produce metal chips and no metallic drill is introduced into the cell. Both of these can act as foreign objects and debris (FOD), which could potentially cause internal cell short circuits and corresponding safety issues. In the second step, a Type K thermocouple is inserted and the hole is sealed by a high-temperature resistant epoxy resin (Loctite EA 9497), followed by curing for 24 h (Fig. 1(a)). To prevent moisture from entering the cell, the procedure is performed in a dry-room environment. With this method it is possible to introduce thermocouples into existing commercial off-the-shelf cells and in pilot cells using controlled and reproducible processes and procedures.
Figure 1(b) shows an X-ray computed tomography (CT) crosssection of the commercial 21700 cell, overlaid with the sensor positions. The internal temperature sensor (Tint) is positioned within the cylindrical hole in the middle of the jellyroll, which is typically empty as a consequence of the technical winding process of the jellyroll. The external thermocouple is fixed at mid-height of the cylinder on the cell surface by a polyimide tape and additionally fixed with a steel sheet holder around the cell during the ARC test. For cells with and without sensor, a comparison of the voltage curves for charging and discharging at 0.5 C, as well as the maximum temperature on the cell surface (see below), did not reveal any significant differences.
(continued on next page)
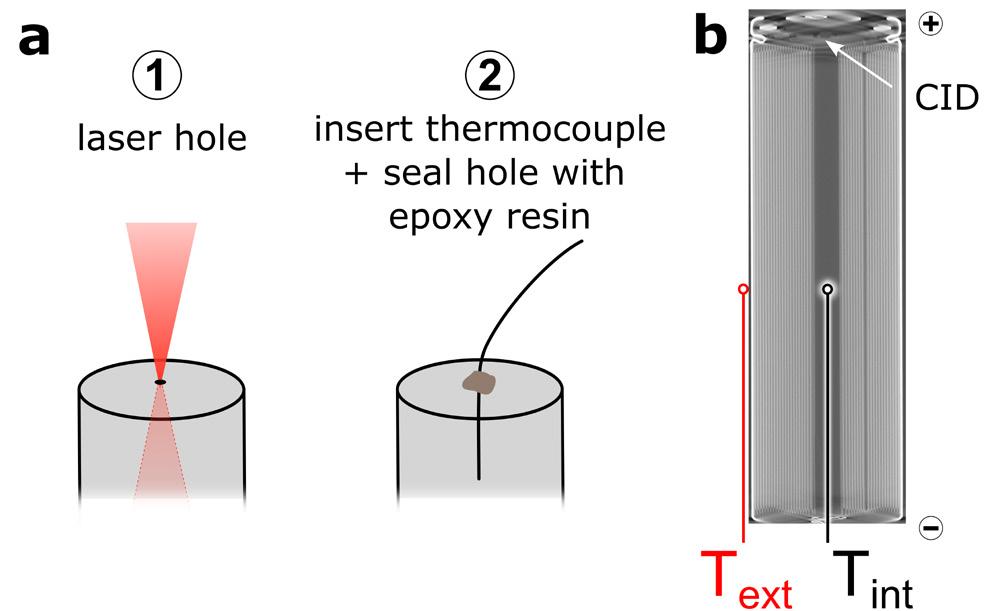
Fig. 1. Implementation of an internal temperature sensor in a cylindrical cell. (a) First, a hole is created in the middle of the bottom of the cell, then a Type K thermocouple is inserted and the hole is closed by epoxy resin. (b) X-ray CT cross-section of a commercial 21700 cell with superimposed internal and external sensor positions. The cell is 70 mm high and 21 mm in diameter.
Thermal Runaway Experiments with Internal Temperature Sensors
Fig. 2(a) shows an exemplary ARC measurement with an internal and external temperature sensor. Before the ARC experiment, the commercial 21700 cell was fully charged to 100 % SOC (4.2 V). In the beginning (until t ≈ 15 h), the internally and externally measured temperatures are very similar due to low heating rates in ARC experiments that lead to an almost thermodynamic equilibrium-like situation. An exception is observed at the marked positions in Fig. 2(a) for the venting (Fig. 2(b)) and the maximum temperature during TR (Fig. 2(c)), where the cell moves away from equilibrium. Notably, the temperature changes inside the cell are in both cases significantly higher than on the cell surface. Furthermore, the typical safety characteristic temperatures during an ARC test, such as the onset of self-heating, venting, and onset of TR, did not show any significant differences for cells with or without an incorporated temperature sensor, supporting the applicability of our method. The cell voltage in Fig. 2(a) remains almost constant until the CID triggering. Following the interruption of the current flow within the cell, the cell voltage is no longer measurable externally. However, during the venting process, the cell briefly reconnects, allowing for the measurement of the cell voltage, as evidenced by the brief voltage peak observed during venting.
At elevated temperatures, the organic carbonate-based electrolyte evaporates and decomposition reactions produce additional gas.3,4 The increasing cell internal pressure11 leads to the interruption of the current flow and venting in the cap of cylindrical cells as a predetermined breaking point. The gas streaming out and the evaporation of the remaining organic electrolyte causes a reduction in temperature due to the Joule-Thomson effect (see Fig. 2(b)). Interestingly, in the example shown, the drop in external temperature is only 2.1 °C, whereas the temperature drop inside the cell is as high as 20.3 °C. Furthermore, the minimum temperature is detected at an earlier point inside the cell compared to the cell surface (∆tvent = 56 s).
Further increases in temperature under quasi-adiabatic conditions in the ARC experiment forces the cell into TR. The maximum temperatures during the TR are shown in Fig. 2(c). While the maximum temperature on the cell surface, Tmax,ext, increases to 537 °C, the temperature inside the cell, Tmax,int, increases to over 1,000 °C. This is in good agreement with the results of Xu et al.36 Furthermore, the maximum temperature occurs earlier inside the cell compared to the cell surface (Δtmax = 10 s). It is notable that Δtmax was observed to be shorter than Δtvent, given that TR processes are inherently faster than venting.
The maximum temperature differences at any given time between the inside and outside of the cells are 20.1 °C and 699.2 °C during venting and TR, respectively. Given a cell diameter of 21 mm, the internal and external temperature sensors are separated by 10.5 mm. This translates to maximum temperature gradients of 1.9 °C mm-1 and 66.6 °C mm-1 for venting and TR, respectively. These values can be compared with radial temperature gradients measured in commercial 18650-type cells (18 mm cell diameter), where maximum values in the order of ~4 °C mm-1 were reported for discharging at high C-rates.21,24,25 Finally, it should be noted that the radial temperature gradient during venting is in a similar range (but pointing in the opposite direction)
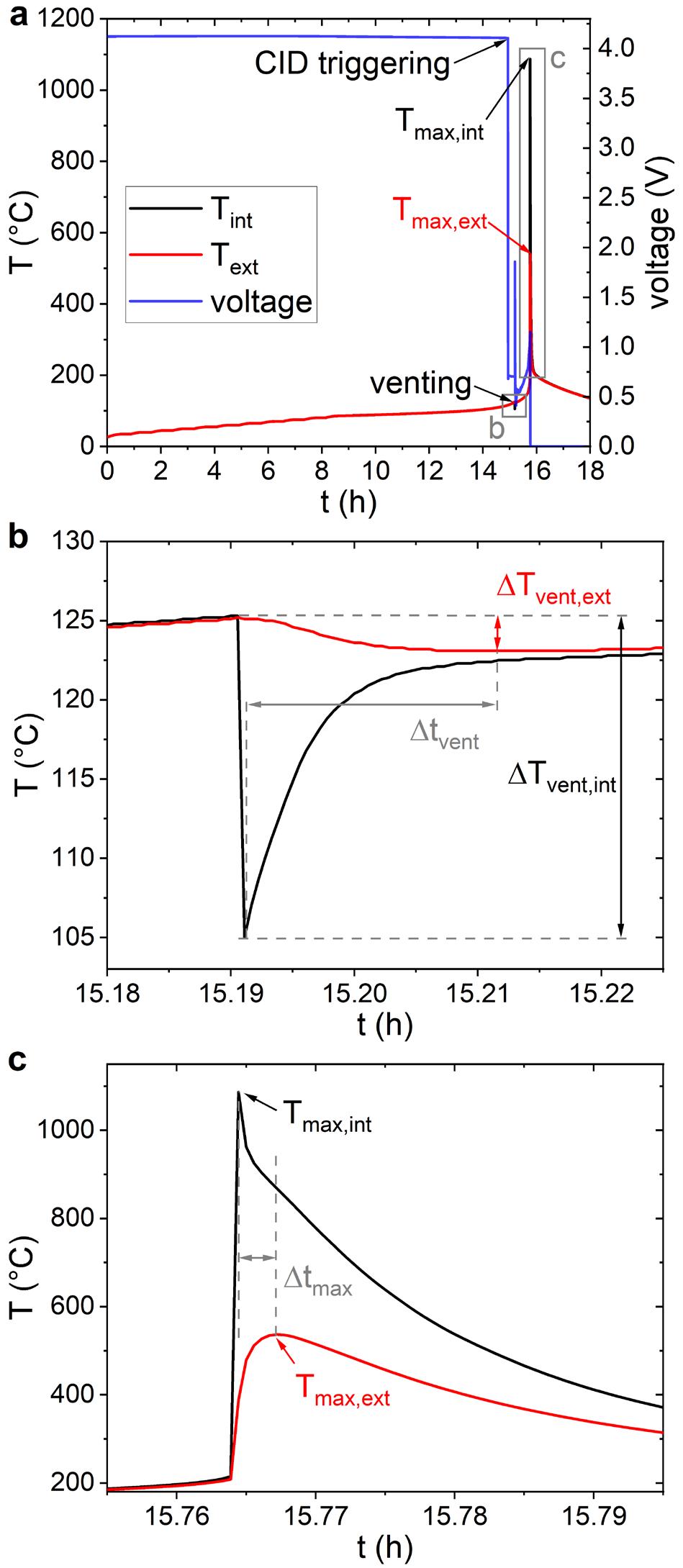
Fig. 2. Exemplary ARC measurement with internal and external temperature sensor for a cell at 100 % SOC. (a) Internal and external temperatures are mostly very similar except at the marked positions for (b) venting and (c) maximum temperature during TR. (a) Additionally, the cell voltage is plotted, which is used to detect the CID triggering and venting.
as high-rate discharge (> 10 C); however, it is an order of magnitude higher during TR.
Fig. 3 shows an evaluation of the maximum temperatures for cells charged to different SOCs before the ARC experiment. Tmax,ext is in a similar range for cells with and without an internal sensor, indicating a similar behavior of both. At 10 % SOC, Tmax,int and Tmax,ext are similar. By contrast, for increasing SOCs the internal and external temperatures start to diverge. While Tmax,ext remains in a similar range of 500–700 °C, Tmax,int increases more rapidly with increasing SOC.
Summary and Outlook
We presented a new method to implement thermocouples into cylindrical LIB cells for operando measurements. As an example, high-energy commercial 21700-type cells (4.85 Ah nominal capacity) with graphite/silicon anodes and Ni-rich cathodes were studied in ARC experiments at different SOCs.
Our results show that the magnitude of temperature changes during safety relevant events of commercial high-energy 21700 LIB cells is much higher inside cells when compared to the cell external surface. During venting, the temperature decrease due to evaporation of electrolyte and the Joule-Thomson effect is on the order of 2–3 °C on the cell surface, whereas it is on the order of approximately 20 °C inside the cell. Most interestingly, the internal temperature sensor detects venting and Tmax earlier by 1–3 min and ~10 s, respectively.
The maximum temperature during TR varies between 500 °C and 700 °C on the housing of the tested cell type over the range of 10 % to 100 % SOC. At 10 % SOC, the maximum temperatures inside and on the outer surface of the cell housing are similar. As soon as SOC and thus the electrochemical energy of the cell increases, the temperature inside the cells rises to greater than 1,000 °C at 100 % SOC, while the cell surface temperature remains in the range of 500–700 °C. This is due to the poor thermal conductivity perpendicular to the electrode and separator layers,37 limiting the heat transfer to the cell housing during the fast TR process. In the tested cells, this can result in temperature gradients of up to ~66.6 °C mm-1, which is one order of magnitude higher than during discharge at a high C-rate (e.g., 10 C) under normal operation.
For 18650-type cells, a similar order of magnitude of the temperatures due to the similar heating behavior under non-abusive conditions30 and due to the similar volume/surface ratio of both formats (21700: 4.57, 18650: 3.95)31 is expected. In larger format cells (such as 46800 cylindrical cells or prismatic cells), it is likely that such effects are magnified due to their higher volume/surface ratios.31 Conversely, these effects may not be as significant in smaller format cells (such as CR2032 coin cells). Comparing high-power and high-energy optimized cylindrical cells of the same format, it is likely that higher radial temperature gradients occur during TR for the high-energy cells
as they tend to have thicker electrode coatings which reduce thermal conductivity. However, this is counteracted by the trend of more windings in the jelly roll and therefore more separator layers for the high-power cells.
The method of implementing temperature sensors is likely to be applicable to all cylindrical cells, provided they do not contain a mandrel in the middle of the jelly roll. The presence of a mandrel would hinder the insertion of the thermocouple in the middle of the cell. In particular, the method may also be applied to commercial offthe-shelf cells without having to change the production process, which would not be possible in most cases. The advantage of using a laser to prepare the hole in the cell housing for sensor insertion is that it does not create metal chips and no metallic drill is required, both of which increase the risk of FOD entering the cell. However, in contrast to other methods, our approach allows for the operando measurement of cell temperature during laboratory safety tests, which enables an increased understanding of how Li-ion cylindrical cells behave under TR conditions.
To increase sustainability and reduce LIB costs and market dependence on critical raw materials (such as lithium, cobalt, manganese, and nickel), aged LIBs can be re-used in second-life applications before recycling. The safety of aged LIBs in ARC tests depends on the main aging mechanism that the cells have experienced.3–5,38–40 A severe aging mechanism is the deposition of lithium metal on anodes as a competitive reaction to lithium intercalation into graphite.39 Thermodynamically, deposition of lithium is favored for anode potentials < 0 V vs. Li/Li+ (i.e., during charging at low temperatures and at high C-rates).39,41 While lithium plating on the anodes can lead to a decreased safety level, 3–5,38–40 growth of the solid-electrolyte interface (SEI) can increase the safety properties in ARC tests.4,5 We would like to point out that the decreased safety in the case of Li plating in ARC experiments is not due to Li dendrites producing internal short circuits between the electrodes, but due to exothermic reactions of lithium with electrolyte.39 In contrast to internal short circuits, which can only happen during charging, exothermic reactions are additionally relevant during discharging and even during rest times. Cell internal sensors are a valuable operando tool for gaining more knowledge about the TR behavior of LIB cells and will be utilized in the future to study cells with different aging mechanisms. This will help to increase the safety in second-life applications of LIBs on a knowledge-based approach.
Acknowledgements
Funding of the projects RollBatt (03XP0245A) in the ProZell cluster and AnaLiBa (03XP0347C) in the AQua cluster by the German Federal Ministry of Education and Research (BMBF) and project management by Projektträger Jülich (PtJ) are gratefully acknowledged. © The Electrochemical Society. DOI:10.1149/2.F07243IF
About the Authors
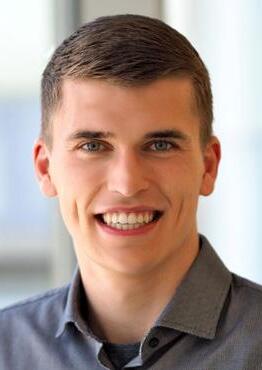
Max Feinauer, PhD student, Zentrum für Sonnenenergie- und WasserstoffForschung (ZSW)
Education: BSc in physics (Ulm University), MSc in physics (Karlsruhe Institute of Technology)
Research Interests: Aging mechanisms in Liion batteries with a special focus on temperature, extension of battery life based on knowledge of underlying mechanisms, interactions of aging and safety of Li-ion batteries
Work Experience: Master student at Institute for Data Processing and Electronics (IPE) at KIT with focus on battery systems, PhD student at ZSW in the team Post-Mortem Analysis and Aging Mechanisms of Thomas Waldmann (since 2021)
Fig. 3. Internal and external maximum temperatures during ARC tests with commercial 21700 cells at different SOC for cells with and without internal temperature sensor. (continued on next page)

Pubs + Patents: 6 peer-reviewed articles, 4 presentations, 14 posters, ECS pub affiliations: 5 Website: https://www.zsw-bw.de/ https://orcid.org/0000-0003-2483-8257

Feinauer et al.
(continued from previous page)
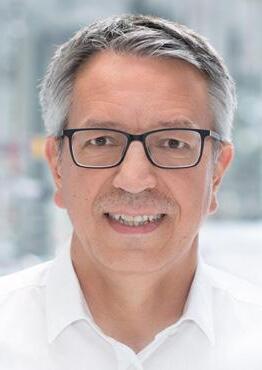
Markus Hölzle, Board Member and R&D Manager, ZSW
Education: Diploma in Chemistry (Ulm University), PhD in Electrochemistry, Dr. rer. nat., Department of Electrochemistry (Ulm University). Supervisor: Prof. Dr. D.M. Kolb
Research Interests: Energy materials and their applications, Cathode materials for Lithium-ion batteries, Precious and base metals catalysts, Hydrogen generation and use
Work Experience: Various R&D, marketing, and management positions within BASF Group in Germany, USA, and Japan. Focus on performance inorganics (1996–2020), Current position: Board member and R&D management of approx. 200 scientists in the field of battery, fuel-cells and hydrogen at ZSW (from 2020)
Work with ECS: Responsible for membership of ZSW institute, Guiding ECS student chapter at ZSW institute
Awards: 3rd Poster Award, Batterieforum Deutschland (2024)
Pubs + Patents: 33 patents and patent applications, 26 peer-reviewed articles and 30 posters
8. E. P. Roth and C. J. Orendorff, Electrochem Soc Interface, 21, 45 (2012)
9. D. Doughty and E. P. Roth, Electrochem Soc Interface, 21, 37 (2012).
10. S. Ohneseit, P. Finster, C. Floras, N. Lubenau, N. Uhlmann, H. J. Seifert, and C. Ziebert, Batteries, 9, 237 (2023)
11. B. Lei, W. Zhao, C. Ziebert, N. Uhlmann, M. Rohde, and H. Seifert, Batteries, 3, 14 (2017)
12. Y. Preger, L. Torres-Castro, T. Rauhala, and J. Jeevarajan, J Electrochem Soc, 169, 030507 (2022).
13. T. P. Barrera, J. R. Bond, M. Bradley, R. Gitzendanner, E. C. Darcy, M. Armstrong, and C.-Y. Wang, Electrochem Soc Interface, 31, 69 (2022)
14. R. Srinivasan, P. A. Demirev, and B. G. Carkhuff, J Power Sources, 405, 30 (2018)
15. R. Srinivasan, B. G. Carkhuff, M. H. Butler, and A. C. Baisden, Electrochimica Acta, 56, 6198 (2011)
16. R. Srinivasan, P. A. Demirev, and B. G. Carkhuff, J Electrochem Soc, 169, 020522 (2022)
17. J. Lamb, L. Torres-Castro, J. C. Hewson, R. C. Shurtz, and Y. Preger, J Electrochem Soc, 168, 060516 (2021)
18. T. Joshi, S. Azam, C. Lopez, S. Kinyon, and J. Jeevarajan, J Electrochem Soc, 167, 140547 (2020).


Website: https://www.zsw-bw.de/ https://orcid.org/0009-0004-8278-1089
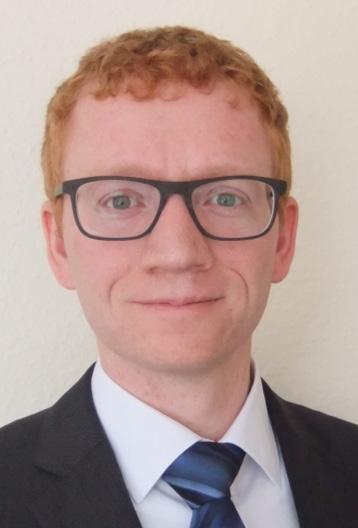
Thomas Waldmann, Team Leader, PostMortem Analysis & Aging Mechanisms, ZSW and Principal Investigator, Helmholtz Institute for Electrochemical Energy Storage
Education: Diploma in Chemistry (Ulm University), PhD in Surface Chemistry, Dr. rer. nat., (Ulm University, Institute of Surface Science and Catalysis), Supervisor: Prof. Dr. R. J. Behm
Research Interests: Development of physico-chemical methods for material characterization, Material characterization of Li-ion and Na-ion batteries, Extension of battery life based on knowledge of underlying mechanisms, Li deposition/plating on anodes and fastcharging of Li-ion batteries, Interactions of aging and safety of Li-ion batteries
Work Experience: Previous positions include Scientific Project Manager at ZSW (2011–2017), currently team leader, PI, and Privatdozent
Work with ECS: Partner institute representative, Peer-reviewer for JES
Awards: 1st Poster Award, session A05, 236th ECS meeting (2019); 1st Poster Award, Advanced Battery Power (2021); 3rd Poster Award, Batterieforum Deutschland (2024)
19. S. Oishi, T. Abe, T. Nagaura, and M. Watanabe, (1990).
20. B. Bausch, S. Frankl, D. Becher, F. Menz, T. Baier, M. Bauer, O. Böse, and M. Hölzle, J Energy Storage, 61, 106841 (2023).
21. T. Waldmann and M. Wohlfahrt-Mehrens, ECS Electrochem Lett, 4, A1 (2015)
22. L. Sun, W. Sun, and F. You, Appl Energy, 271, 115243 (2020)
23. G. Zhang, L. Cao, S. Ge, C.-Y. Wang, C. E. Shaffer, and C. D. Rahn, J Electrochem Soc, 161, A1499 (2014).
24. T. Waldmann, S. Gorse, T. Samtleben, G. Schneider, V. Knoblauch, and M. Wohlfahrt-Mehrens, J Electrochem Soc, 161, A1742 (2014)
25. T. Waldmann, G. Bisle, B.-I. Hogg, S. Stumpp, M. A. Danzer, M. Kasper, P. Axmann, and M. Wohlfahrt-Mehrens, J Electrochem Soc, 162, A921 (2015)
26. Q. Yuan, F. Zhao, W. Wang, Y. Zhao, Z. Liang, and D. Yan, Electrochimica Acta, 178, 682 (2015)
27. M. S. K. Mutyala, J. Zhao, J. Li, H. Pan, C. Yuan, and X. Li, J Power Sources, 260, 43 (2014)
28. D. Anthony, D. Wong, D. Wetz, and A. Jain, Int J Heat Mass Transf, 111, 223 (2017)
29. J. B. Quinn, T. Waldmann, K. Richter, M. Kasper, and M. Wohlfahrt-Mehrens, J Electrochem Soc, 165, A3284 (2018).
30. T. Waldmann, R.-G. Scurtu, K. Richter, and M. WohlfahrtMehrens, J Power Sources, 472, 228614 (2020)
31. T. Waldmann, S. Rössler, M. Blessing, R. Schäfer, R.-G. Scurtu, W. Braunwarth, and M. Wohlfahrt-Mehrens, J Electrochem Soc, 168, 090519 (2021)
32. T. Waldmann, G. Geramifard, and M. Wohlfahrt-Mehrens, J Energy Storage, 5, 163 (2016)


Pubs + Patents: 58 peer-reviewed articles, 81 presentations, 89 posters, h-index: 28, ECS pub affiliations: 35 Websites: https://www.zsw-bw.de; https://hiu-batteries.de https://orcid.org/0000-0003-3761-1668
References
1. M. M. Doeff, Electrochem Soc Interface, 28, 8 (2019).
2. Y. Ding, Z. P. Cano, A. Yu, J. Lu, and Z. Chen, Electrochem Energy Rev, 2, 1 (2019).
3. A. A. Abd‐El‐Latif, P. Sichler, M. Kasper, T. Waldmann, and M. Wohlfahrt‐Mehrens, Batter Supercaps, 4, 1135 (2021)
4. M. Feinauer, A. A. Abd-El-Latif, P. Sichler, A. Aracil Regalado, M. Wohlfahrt-Mehrens, and T. Waldmann, J Power Sources, 570, 233046 (2023)
5. T. Waldmann, J. B. Quinn, K. Richter, M. Kasper, A. Tost, A. Klein, and M. Wohlfahrt-Mehrens, J Electrochem Soc, 164, A3154 (2017).
6. M. N. Richard and J. R. Dahn, J Electrochem Soc, 146, 2068 (1999).
7. E. P. Roth and D. H. Doughty, J Power Sources, 128, 308 (2004)
33. T. Waldmann, R.-G. Scurtu, D. Brändle, and M. WohlfahrtMehrens, Energy Technol, 2200583 (2022)
34. X. Zhang, Electrochimica Acta, 56, 1246 (2011)
35. S. V. Erhard, P. J. Osswald, J. Wilhelm, A. Rheinfeld, S. Kosch, and A. Jossen, J Electrochem Soc, 162, A2707 (2015)
36. C. Xu, X. Feng, W. Huang, Y. Duan, T. Chen, S. Gao, L. Lu, F. Jiang, and M. Ouyang, J Energy Storage, 31, 101670 (2020)
37. M. Feinauer, N. Uhlmann, C. Ziebert, and T. Blank, Batteries, 8, 177 (2022)
38. M. Fleischhammer, T. Waldmann, G. Bisle, B.-I. Hogg, and M. Wohlfahrt-Mehrens, J Power Sources, 274, 432 (2015)
39. T. Waldmann, B.-I. Hogg, and M. Wohlfahrt-Mehrens, J Power Sources, 384, 107 (2018).
40. T. Waldmann and M. Wohlfahrt-Mehrens, Electrochimica Acta, 230, 454 (2017).
41. M. Weiss, R. Ruess, J. Kasnatscheew, Y. Levartovsky, N. R. Levy, P. Minnmann, L. Stolz, T. Waldmann, M. Wohlfahrt‐Mehrens, D. Aurbach, M. Winter, Y. Ein‐Eli, and J. Janek, Adv Energy Mater, 2101126 (2021).
Achieving Passive Thermal Runaway Propagation Resistance in Li-ion Battery Packs
by Alexander Quinn, Jacob Darst, Matthew Keyser, Paul T. Coman, Thomas P. Barrera,
Paul R. Shearing, David Petrushenko, Donal P. Finegan, Eric Darcy
Because of their high specific and volumetric energy density, lithium-ion cells are popular in energy storage systems with strict mass and volume restrictions. Such applications include electric vehicles, power tools, consumer electronics, all-electric aircraft, as well as high-reliability military and crewed spacecraft. Next-generation rechargeable lithium cell designs are characterized by higher specific energy which further increases the severity of thermal runaway (TR) safety hazards. Even with carefully controlled high-quality manufacturing processes, TR can occur unpredictably due to latent defects inside the cells causing internal short circuits.1-3 This work elaborates on the lessons learned from cell and battery pack safety research for crewed spacecraft applications.
TR is the most energetic battery system safety hazard. TR begins with excessive cell heat generation that cannot be adequately dissipated, leading to a cascade of exothermic reactions involving electrode and electrolyte materials.4 These reactions generate gases which pressurize the cell. Together, elevated temperatures and pressure often burst the cell housing,5 causing violent ejection of hot solids, molten metals, vapors, and highly toxic gases.6,7 Further, combustible ejected material (such as H2 gas and vaporized organic material) can ignite, exacerbating the energy release.8,9 Cell chemistry,9 material quantity, state of charge (SOC),10 and aging history11 largely determine the energy and materials released during TR. Thus, while higher capacity chemistries and elevated cell voltages increase the energy density of battery packs, they also lower the TR onset temperatures which increases energy release.6,8,9,12 Crushes, penetrations, and external shorts can induce TR,13-17 typically driving numerous cells into TR simultaneously. Such events are complex and challenging to mitigate, often requiring information about the environment of the battery (e.g., the location of the battery within an EV) to design adequate safety measures. Single-cell TR, on the other hand, can be managed at the pack level.
Cell quality varies with manufacturer practices and cell design. A critical, yet insufficient, task for safe battery assemblies is to assess cell quality and to employ prevention measures which minimize the probability of cell defects entering assemblies. Such measures include assessing cell design maturity, verifying that production history is absent of cell-quality recalls, performing cell production line audits for defect mitigation, validating uniform performance of received lots, examining cell quality with X-ray computed tomography (CT), and tear-down examinations.47 Further, 100% of cells used in a battery assembly can be nondestructively screened, particularly to identify subtle soft shorts.48 Nevertheless, it is imperative to assume that these prevention efforts will not eliminate 100% of defects nor prevent single-cell TR due to them. This necessitates and motivates passive propagation resistant (PPR) battery design features for critical applications such as crewed spacecraft.
In this perspective, we expand upon five general design guidelines developed by NASA to significantly reduce TR propagation risk and hazards in multi-cell lithium-ion battery systems used in crewed spaceflight missions.18-25 These battery pack design guidelines are:
1. Reduce risk of sidewall and spin groove ruptures in cells
2. Provide adequate spacing and heat sinking for cells
3. Protect adjacent cells from ejected material during TR
4. Electrically isolate the TR cell
5. Prevent flames and sparks from exiting the battery enclosure
Following these guidelines, a passive propagation architecture tolerant of a single-cell TR event can be achieved without major compromise to battery performance, and with only a ~20% increase in parasitic mass burden compared to non-TR tolerant lightweight designs.23 Our findings are presented in the context of complementary cell TR calorimetry, with high speed radiography, relevant cell sidewall rupture characterization, blast plate testing, and simulation to guide battery design and its PPR test articles for validation.26
Characterization of Cell TR Response
The internal location of TR initiation is often unpredictable but correlates to the cell failure mode and the consequent risks.27 A spectrum of risk scenarios should be considered in battery pack engineering (Fig. 1(a)). High temperature and pressure during TR may stress cell features, some of which are prone to failure. Vents placed on the top, bottom, or both ends of a cell are intended to relieve excess cell pressure, release electrolyte before it can combust, and provide a dedicated ejection path for TR products.27,28 For example, in Fig. 1(b) the top vent bursting was accompanied by material ejection. Sometimes, material is expelled from both vents (Fig. 1(d)). However, vents are not a guaranteed egress path. In an identical cell (Fig. 1(c)), the vents remained intact, with most of the material contained. Some cells, including those with vents, fail on their side walls (Fig. 1 (e)-(j)). Thermal imaging of various cells (Fig. 1 (f)-(i)) shows that material can eject in random directions. High energy density cells are more prone to sidewall breaches due to increased energy generation during TR and thinner cell casings used to reduce parasitic mass.12,27 These observations suggest that 18650 and 21700 cells require optimization to release ejecta only at the vents, necessitating pack-level safety features that address the unpredictable directionality of ejecta.
Supporting Structures for Cells
The cell support, a structure designed to mechanically support cells during expected mechanical stresses (e.g., shocks, vibrations), is frequently also the principal component of battery thermal management. Its design is critical to achieving Guideline 1 (prevent sidewall and spin groove ruptures) and Guideline 2 (provide adequate spacing and heat sink for cells). Battery packs often have cells arranged in parallel (spatially) making adjacent cells prone to sidewall and spin groove breaches, subjecting adjacent cells to significantly more heat transfer than conduction alone.
Without adequate sidewall and spin groove support, TR can easily propagate between cells in designs which otherwise effectively suppress TR propagation. For instance, the vaporizing heat sink in (continued on next page)
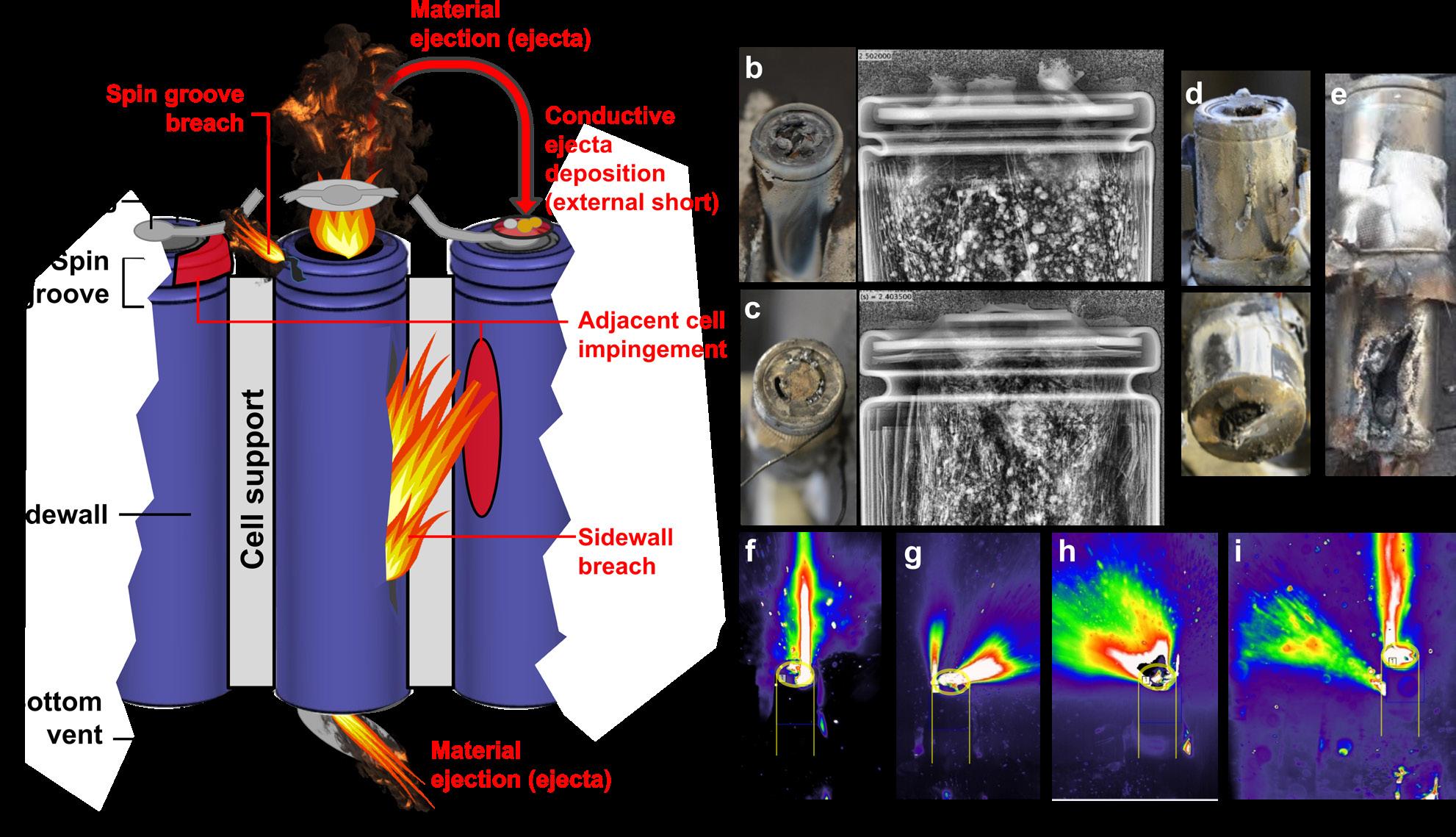
failure modes as result of single-cell TR. (a) Schematic of cell-level failure modes and their implications in a battery. (b) Cell picture and corresponding radiograph after TR showing material expulsion at top vent where the top button melted. (c) TR example where the top button remained intact with the cell containing most of the material. (d) Both top and bottom vents with expelled materials. (e) An example of a sidewall breach. (f-i) Thermal imaging of gas and material ejection. (f) An example of upward ejecta expulsion. (g) Compromise of spin groove and sideward ejection. (h) Broad sideward ejection. (i) Sidewall breach and upward ejection.
Fig. 2(a), consisting of water contained within a wicking material, was tolerant to TR unless a sidewall breach occurred. Metallic supports (e.g., the aluminum heat sink of Fig. 2 (a) can protect adjacent cells effectively by decreasing the chance of sidewall rupture, preventing direct ejecta impingement, and dissipating heat. In Fig. 2 (b)-(c), the aluminum heat sink contained a sidewall breach and prevented TR, yet adjacent cells were still impacted by material ejected above the heat sink. This highlights the necessity of well-designed capture plates (temperature-resistant, electronically insulating structures) which protect cell spin grooves without compromising vent performance (allowing room for crimp unfolding and header release).
Conductive heat sinks can accommodate narrow cell spacing to improve volumetric energy density. For example, hexagonally arranged cells in an aluminum heat sink with as little as 0.5 mm spacing between cells can meet TR propagation requirements. Thermal mass, conductivity, and contact resistances determine the efficacy of a heat sink in preventing the propagation of TR.29 In some designs, a low thermal interface resistance between the cell and cell support is desired to rapidly transfer heat from the cell.30 In other designs, higher thermal resistance may be desired to more slowly dissipate excessive heat that may damage adjacent cells. For instance, in certain designs studied with computational modeling, insulating gaps (of air or mica) between cells and the heat sink decrease adjacent cell temperatures (Fig. 2(c)).31,32 Modeling generally accelerates safe battery design by quantifying heat dissipation during nominal operation and TR. However, assumptions about heat generation rates and modes of heat transfer should be scrutinized and tested. For instance, while air gaps are effective thermal resistors, volumetric expansion of TR cells can decrease cell-support gaps and accelerate conductive heat transfer. Altogether, the locality and intensity of heat generation rates, heat sink-cell interfaces, and heat sink material are necessary considerations to satisfy Guideline 2 (provide adequate cell spacing and heat sinking).
Insulating cell support designs can also mitigate TR propagation, but they are limited in thermal dissipation capability during operation. A 2 mm air gap was previously suggested to prevent propagation of TR from cell to cell.33 Unfortunately, we found that the lack of cell heat sinking can lead to TR propagation of adjacent cells through thermal conduction in nickel bussing. Based on these results, we studied a design with 4 mm cell spacing, without any heat sinking except through bussing, and without any cell can wall supports. In this design, propagation occurred due to sidewall rupture events.25 Air gaps offer no protection to adjacent cells from sidewall and spin groove breaches. Thus, air gap-based cell supports are suited only for batteries with relatively low discharge rates which reliably eject material through their vent(s). We observed that cells with a rated capacity > 2.8 Ah were significantly more likely to breach their sidewalls than those with ≤ 2.6 Ah capacity, which consistently released materials through vents. Perhaps improved, lightweight, temperature-resistant, and high-strength insulating materials can be used to prevent sidewall impingement (Fig. 2 (d)) at the expense of additional mass. A compromise between using a metallic heat sink and one without support material is to use metal sleeves to contain sidewall breaches (Fig. 2 (e)). In the right image of Fig. 2 e, a singlecell sidewall breach is contained within the region covered by a 230 μm thick steel sleeve. Similarly, thicker but less-dense carbon-fiber sleeves have shown promise for sidewall rupture mitigation.24
Cell spacing is closely related to the thermal management system of choice, which in turn depends on the intended battery application.17,34,35 While active thermal management methods generally have superior cooling capabilities compared to passive ones, passive systems are simpler, less expensive, lighter weight, and are preferred when active cooling is not needed. Passive TR propagation is a necessity for passive or active heat sinks, given that active thermal management systems are likely to be ineffective at dissipating the heat fluxes of TR.29
Fig. 1. Cell-level
Electrical Danger Mitigation
External and internal shorts release stored energy as heat— potentially leading to TR. External shorts may occur when battery wiring or insulating features are compromised, such as through heating or mechanical forces. TR events increase the odds of developing shorts. Ejecta contains conductive materials which may deposit on electrical pathways, shorting single or multiple cells. The spatial arrangement of cells and electrical connections (including the seriesparallel strategy) can strongly influence the probability and hazard of shorting events due to ejecta deposits. The severity of the short is determined by the impedance of the short and the available power to the short. Low-impedance shorts across the battery terminals, for instance, can result in catastrophic high-current situations that may drive additional TR, whereas high-impedance shorts may lead to slow battery discharge which generates insufficient heat to drive TR but nevertheless, is undesirable.37,38 Guideline 3 (protect cells from ejected material during TR) involves electrical isolation of cells from one another and managing the flow path of ejecta. Guideline 4 (electrically isolate the TR cell) suggests backup protection is necessary when internal or external shorts develop.
Bussing is preferred to wire for electrical connections because of its low profile, static positioning, and easy installation. Wiring required for instrumentation should be routed to avoid vents and other regions of high susceptibility to ejecta impingement and tethered or guided by grooves to maintain its position. Wiring may be coated in high-temperature insulation material to protect against ejecta impingement. Fuses (Guideline 4) halt certain external short circuits or shunt currents before they overheat additional cells and drive them into TR. Cells in parallel are especially susceptible to current shunting: an open-circuit voltage (OCV) drop in a failed cell will sink current from parallel cells. Fuses prevent this. Cell fuses are most effective near the cell terminal with the lowest propensity for ejected TR material, which is the negative terminal in most current cell designs. Otherwise, conductive TR ejecta may reclose the circuit, rendering the fuse ineffective. The resistance incurred by fuses is important to consider for normal operation. By employing a series-parallel topology, entire series strings can be isolated with fewer fuses, enabling lower pack resistance and thus higher battery power. In some designs, clocking and the orientation of the tabs/fuses
(continued on next page)
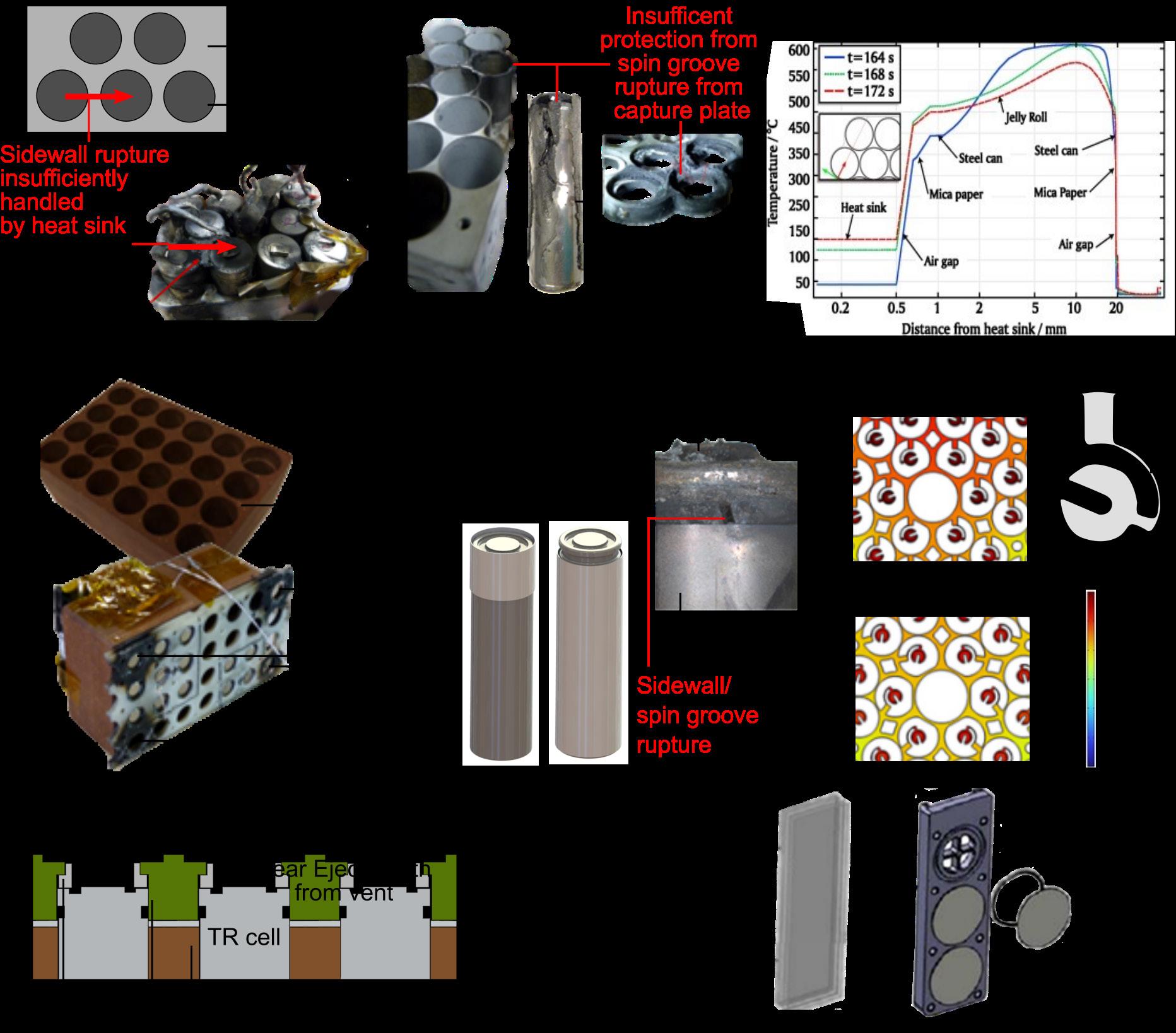
Fig. 2. Pack-level TR propagation mitigation measures. (a) Sidewall breach in vaporizing heat sink–caused TR propagation. (b) Metallic heat sink contains a sidewall rupture, but adjacent cells are damaged due to ejected material. (c) Computational modeling highlighting temperature gradients from TR cell to heat sink.32 (d) Rigid insulating cell support effectively mitigates sidewall breach of cells. (e) Steel tubes used to provide sidewall support. (f) Fuse integrated into Ni bussing, showing the clocking that favors a smooth current path between the cells and the pack terminals. Computational modeling guides design of efficient bussing.36 (g,h) Vent pipe structure directs ejecta away from cell. Tortuous path, baffles, steel mesh, prior to a waterproof, gas permeable membrane in battery housing limits escape of flames, particulate, and sparks.
can significantly influence the resistance of the bussing, especially at high current (Fig. 2(f)).36
Protecting Adjacent Cells from TR Ejecta
Once the TR ejecta exit path from the cell is controlled (Guideline 1), with only top and bottom venting of ejecta, the ejecta must be encouraged to disperse widely in thermally and mechanically strong channels to protect adjacent cells (Guideline 3). Blast plates, composites of layered thin-metal and insulating materials, protect cells that would otherwise be in the line of sight of the vented ejecta. Ideally, blast plate tests should show that the plates are not perforated by ejecta nor that ejecta accumulates next to the TR cell.23 Triggering cells in these blast plate tests by heat rather than by nail-penetration results in higher ejecta momenta and thus is a more conservative test.23 The gap distance between the vents and the blast plate (the nearest surface that the ejecta is directed at) is also crucial. A gap increase from 5 mm to 8 mm was found necessary in scaling up from 18650 to 21700 cell designs.23
Battery Housing for Protecting Against Flames and Ejected Material
Guideline 5 (prevent flames and sparks from exiting the battery), is intended to limit damage external to the battery, minimize injury, and decrease the combustion intensity. In most cases, complete containment of TR is not feasible: a completely contained TR event is an explosion hazard. In an open system, gases and particles exit the enclosure through the path of least resistance,39 but the flowing material should be treated prior to departure. Tortuous and/or filtered vent paths to the battery exterior can suppress flames and particulate ejection (Fig. 2 (g)-(h)) A clear egress path for the ejecta gasses and particulate also minimizes unfavorable ejecta deposits on battery components. When implementing such restrictions to flow, it is necessary to seal battery enclosures to facilitate flow only through intended paths. The amount of gas generated by a single cell can be estimated from calorimetry data and, with the free-volume space of the battery, can be used to estimate the mechanical loads on the battery housing due to the elevated pressure within enclosed battery packs. Note that proper housing will still leave users vulnerable to toxic gases.
Simulation Guides TR Propagation Testing
Mathematical modeling has played a critical role in guiding numerous battery and TR propagation test designs. Fractional thermal runaway calorimetry (FTRC) data was used to extract heatgeneration profiles for use in models which predict the trigger cell temperature during thermal runaway events both as stand-alone cells and within a pack.31, 32, 39-42 Electrochemical models based on reaction kinetics, incorporating calorimetric profiles from experimental heat measurements, can predict voltage profiles and temperature dynamics during normal charge and discharge cycles.43 Charge-conservation equations coupled with thermal models have been instrumental in reducing bussing resistance and optimizing its geometry, while electrical-thermal models have guided bussing design, minimizing parasitic mass.36 The thermal runaway model has identified worst-case scenario trigger cell locations, guiding placement of thermocouples based on predicted thermal gradients. Advanced 3D and reduced 2D models have been used to optimize cell spacing, cell-battery interfaces, and identify insulating layers at interfaces. These models were anchored to experimental results, guided initial designs, and informed design iterations based on experimental data.40
Field-relevant TR Propagation Testing
The battery operating environment and the potential failure mechanisms determine the critical failure modes to test for compliance with battery safety requirements. Preventing TR propagation is increasingly required among validation and safety tests. True internal short-circuit tests have been recognized as a necessity for accurate quantification of short-circuit failure modes and their effects.44
Battery-level TR testing involves placing a designated “trigger” cell into a specific location within a “flight-like” battery, instrumenting the battery and enclosure, driving the trigger cell to TR, observing the time and space-resolved temperature profiles throughout the TR process, and assessing the damage to battery components and individual cells. Several options for triggering single-cell TR include nail penetration, heat, overcharge, and implanting an internal shorting device in a cell.45 All these methods have drawbacks. Overcharge requires electrically isolating the trigger cell and the TR response happens at irrelevant states-of-charge. Nail penetration perforates the cell enclosure and pins down the jelly roll, and thus does not capture certain relevant TR ejecta responses. Heating single cells is difficult to perform without biasing the thermal response of adjacent cells. Cell manufacturers are not universally willing to implant the on-demand wax shorting device invented by NREL and NASA.3 However, this device reliably generates single point cell internal shorts leading to TR when exposed to > 57 °C. Moreover, when the device is located 3 winds from the outside of the jelly roll, the resulting initiating TR event is more likely to induce a spin groove rupture in the same orientation as the device. Thus, aligning the cell such that the internal short circuiting device faces adjacent cells. Test protocols can take advantage of utilizing multiple initiation methods for conservative test programs.
Generally, TR propagation testing should involve driving single cells to TR in a variety of scenarios, including those in which the battery is subject to its most extreme environments or can result in the greatest consequences. Trigger cell location options to consider are those with the fewest and the most adjacent cells, those closest to high voltage differences between cells, and those closest to the flamearresting vent port of the battery. The test campaign should include credible worst-case temperatures, pressures, and SOC conditions. The design of the PPR test unit(s) does not need to be a full-size replica of the final battery design, but it must include all the salient features. Such features include the mechanical, thermal, and electrical batterycell interfaces, TR ejecta paths, and battery enclosure pressurerelease/vent ports. The capacity and energy of the test-unit batteries should be determined prior to starting the single-cell TR PPR test campaign. After an individual or a series of test runs, the battery capacity/energy should be determined to assess the health of the cells adjacent to the TR cell. Our current criteria for success is no cell-tocell TR propagation and no more than 5% performance degradation in the adjacent cells versus pre-test performance. If the 5% criterion is not met, then batteries are disassembled for in-depth assessment (visual inspection, capacity/energy cycling) of individual adjacent cells. If > 1 adjacent cells are found to have degraded in capacity by >5%, then twice as many PPR test runs with no propagation are required to gain confidence in the lower PPR margins of the battery design. Full details for the PPR verification requirements for human spacecraft batteries are in a NASA memorandum.46
Summary
Cell-to-cell TR propagation tolerance and optimal battery function are highly dependent on the cell designs used in the battery pack. The identified relevant cell failure scenarios from these studies directly inform battery development. Modeling, subscale testing, and incremental full-scale battery testing follow to develop a battery that is tolerant to TR and meets application requirements. The five guidelines for designing safe, TR-propagation resistant, and riskreduced batteries are described in detail with examples throughout this perspective and are summarized here:
1. Reduce risk of sidewall and spin groove ruptures in cells. In most pack designs, direct structural support should be used to suppress or contain sidewall and spin groove ruptures.
2. Provide adequate cell spacing and heat sinking for cells. Cell spacing influences heat dissipation and ejecta containment. Proper spacing is a function of the cell used, discharge/charge rates, interstitial material, and the expected failure modes. The goal is to space cells such that heat is sufficiently dissipated during both normal battery operation and TR.
3. Protect adjacent cells from hot TR ejecta and allow ejecta to disperse broadly. The hot ejected material from a TR cell can have very destructive momentum against design features that protect adjacent cells. The channeling of a single cell TR ejecta with high temperature materials, sufficient gaps, and blast plates that don’t perforate are design considerations.
4. Individually fuse cells or series strings of cells to reduce shorting risk. Cell internal shorts are external shorts to adjacent parallel cells. TR ejecta is electrically conductive and can induce circulating currents. Both reduce margins against achieving PPR. Fuses or fusible links are a reliable passive implementation.
5. Prevent flames and sparks from exiting the battery enclosure using flame-arresting features. While propagation is curtailed using Guidelines 1–4, a single-cell TR event can cause flames to exit an improperly designed battery enclosure. First, there must be adequate paths to the environment external to the battery and adequate void volume between each cell. At the battery-environment interfaces, flames and sparks should be suppressed with tortuous and flame-arresting venting features.
Acknowledgements
This work was supported by NASA, the US Navy, and the Advanced Projects Research Agency for Energy (ARPA-E) under the EVs4ALL program. The National Renewable Energy Laboratory is operated by Alliance for Sustainable Energy under Contract No. DE-AC36-08GO28308 for the US Department of Energy. The views expressed in the article do not necessarily represent the views of the DOE or the US Government. The US Government retains and the publisher, by accepting the article for publication, acknowledges that the US Government retains a nonexclusive, paid-up, irrevocable, worldwide license to publish or reproduce the published form of this work, or allow others to do so, for US Government purposes.
©The Electrochemical Society. DOI: 10.1149/2.F08243IF
About the Authors
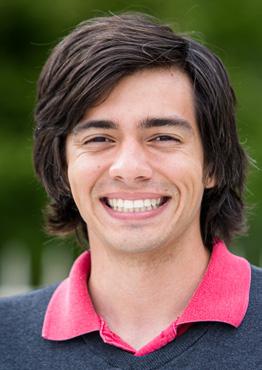
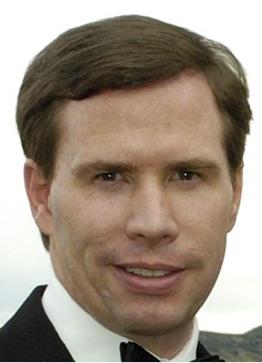
Matthew Keyser, Manager, National Renewable Energy Laboratory (NREL)
Education: BS in Mechanical Engineering (University of Colorado)
Research Interests: Energy storage, Electric vehicles, Battery safety
Work Experience: Matt Keyser leads the electrochemical energy storage group at NREL. During Keyser’s tenure (30+ years) at NREL, he has focused on growing the energy storage laboratory facilities from its humble beginnings to over 6,000 ft2 of laboratory space that enables electrochemical material fabrication, safety analysis and characterization, as well as expanding NREL’s one-of-a-kind and world-class thermal characterization laboratories.
Awards: Four R&D100 awards (1996, 2001, 2013, 2017), 2017 NASAInnovation of the Year Runner-up, 2002 MIT Top 100 Innovators under theAge of 35, Governor’sAward for High Impact Research (2012)
Pubs + Patents: >90 publications, 8 patents
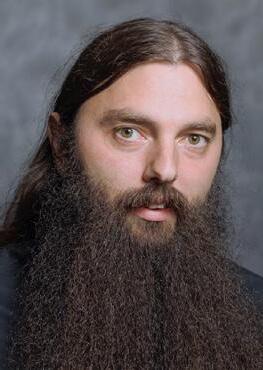
Paul T. Coman, Associate Research Professor, Chemical Engineering, University of South Carolina
Education: PhD in Applied Mathematical
Modeling (University of Southern Denmark)
Research Interests: Li-ion batteries, Battery safety, Electrochemical modeling
Work Experience: Post-doctoral Fellow, Assistant and Associate Research Professor at the University of South Carolina working with mathematical modeling of Li-ion batteries
Work with ECS: ECS Member
Awards: Electrochemical Society (ECS) Battery Division
Postdoctoral Associate Research Award
Alexander H. Quinn, PhD Candidate, MIT
Education: BS in Chemical Engineering (Texas A&M University)
Research Interests: Flow electrochemistry, Liion batteries, Battery safety
Work Experience: Intern at National Renewable Energy Laboratory, Intern at NASA
Johnson Space Center
Work with ECS: ECS Member


Pubs + Patents: 7 papers https://orcid.org/0000-0002-4101-034X
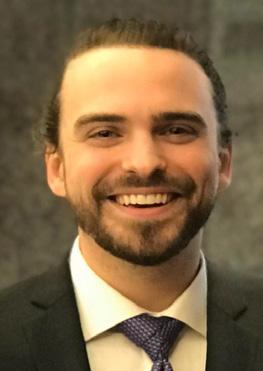
Jacob Darst, Engineer, NASA Johnson Space Center
Education: MEng in Mechanical Engineering (Texas A&M University)
Research Interests: Battery design, Li-ion batteries, Battery safety Awards: NASA Gold Medal, NASA group directors achievement award
Pubs + Patents: 5 publications, 2 patents


Pubs + Patents: > 20 papers https://orcid.org/0000-0003-2876-7441
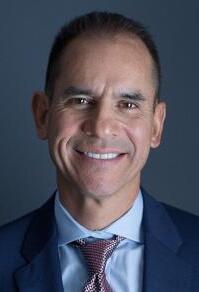
Thomas P. Barrera, President, LithiumIon Battery (LIB)-X Consulting
Education: PhD in chemical engineering (UCLA, under the direction of Ken Nobe), postdoctoral research fellow in materials science and engineering (UCLA, under the supervision of Bruce Dunn). MS in industrial engineering (Northwestern University) and BS in chemical engineering (cum laude) and BA in mathematics-economics (UCSB).
Research Interests: Electrochemical engineering, Clean energy sources, Physical electrochemistry
Work Experience: President and owner of LIB-X Consulting where he provides engineering, expert witness, and educational services in the broad area of lithium-ion battery power systems. Previously, Tom was a Technical Fellow for The Boeing Co. where he led multidisciplinary teams in the end-to-end development of advanced electrical power and battery energy storage systems for high-value government and commercial spacecraft applications.
Pubs + Patents: Editor-in-chief and contributor, Spacecraft Lithium-Ion Battery Power Systems, John Wiley & Sons, IEEE Press, 2023; 3 US patents in aviation battery safety; >50 combined publications and conference presentations.
Awards: AIAA Associate Fellow.
Work with ECS: Life Member; Member-at-Large, Battery Division; Institutional Engagement Committee; Symposia Lead, Battery Safety and Failure Modes (234th, 235th, & 244th meetings).


Website: https://www.libxconsult.com/ https://orcid.org/0000-0001-8311-1889
(continued on next page)
(continued from previous page)
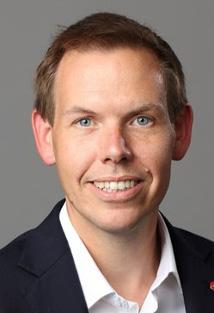
Paul R. Shearing, Professor of Sustainable Energy Engineering at the Department of Engineering Science and Director of the ZERO Institute at Oxford University
Education: Masters in Chemical Engineering (Birmingham University), PhD (Imperial College London, UCL)
Research Interests: Cover a broad range of electrochemical engineering themes with a particular interest in the characterization and understanding of materials for batteries, fuel cells, and other energy applications.
Work Experience: Chair in Sustainable Energy Engineering, University of Oxford (2023–), Professor in Chemical Engineering, UCL (2012–23), Royal Academy of Engineering Chair in Emerging Battery Technologies (2018–)
Pubs + Patents: >450 peer reviewed scientific papers, 1 patent
Awards: Royal Academy of Engineering Chair in Emerging Battery Technologies. Founding investigator of The Faraday Institution, the UK’s independent institute for electrochemical energy storage research where he leads the LiSTAR and Safebatt research programs. 2022 Royal Academy of Engineering Silver Medal.
Work with ECS: Member since 2009
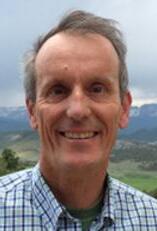
Eric Darcy, Battery Technical Discipline
Lead, NASA Johnson Space Center
Education: PhD in Chemical Engineering (University of Houston)
Research Interests: Battery design, Li-ion batteries, Battery safety
Work Experience: 37-year career at NASA in the areas of battery design, verification, and safety assessments for the rigors of manned spacecraft applications. As Battery Technical Discipline Lead at NASA-JSC, his main objective has been the development of safe, while high performing, battery systems with a deep focus on understanding, preventing, and mitigating latent defects that could lead to Awards: NASA invention of the year 2023, lifetime achievement award by NAATBatt Pubs + Patents: >10 publications, >2 patents Website: https://www.nasa.gov/people/dr-eric-darcy-nesc-academybiography/
References
1. B. Liu, Y. Jia, J. Li, S. Yin, C. Yuan, Z. Hu, L. Wang, Y. Li and J. Xu, J Mater Chem A, 6, 21475 (2018)
2. M. Zhang, J. Du, L. Liu, A. Stefanopoulou, J. Siegel, L. Lu, X. He, X. Xie and M. Ouyang, J Electrochem Soc, 164, A3038 (2017)


Websites: www.zero.ox.ac.uk https://eng.ox.ac.uk/people/paul-shearing/ https://orcid.org/0000-0002-1387-9531
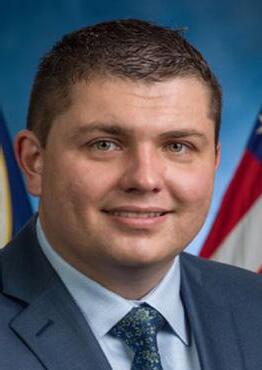
David Petrushenko, Engineer, NASA Johnson Space Center
Education: PhD in Chemical Engineering (University of South Carolina)
Research Interests: Battery design, Li-ion batteries, Battery safety
Work Experience: Engineer at NASA Johnson Space Center
Awards: 2023 R&D 100 Award (FABIA: Fieldable Atomic Beam Isotopic Analyzer)
Pubs + Patents: 7 papers
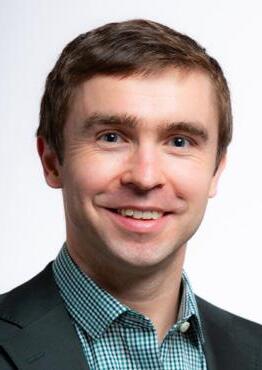
Donal P. Finegan, Researcher IVChemical Engineering, NREL
Education: PhD in Chemical Engineering (University College London)
Research Interests: Characterization and diagnostics of energy materials, Li-ion batteries, Safety
Work Experience: Donal Finegan has seven years of experience working on energy storage technologies at the National Renewable Energy Laboratory. Finegan has led several projects focused on battery safety, scaling advanced manufacturing methods for Li-ion batteries, and characterizing degradation mechanisms of energy storage materials. He leads NREL’s X-ray computed tomography laboratory. Work with ECS: Member.
Awards: The European Synchrotron Young Scientist Award (2020), NASA Johnson Space Center Directors Group Achievement Award (2019), Umicore Materials Technology Award (2018)
Pubs + Patents: >100 publications, 4 patents
3. D. P. Finegan, E. Darcy, M. Keyser, B. Tjaden, T. M. Heenan, R. Jervis, J. J. Bailey, R. Malik, N. T. Vo and O. V. Magdysyuk, Energ Environ Sci, 10, 1377 (2017).
4. J. Lamb, C. J. Orendorff, E. P. Roth, and J. Langendorf, J Electrochem Soc, 162, A2131 (2015).
5. D. P. Finegan, E. Darcy, M. Keyser, B. Tjaden, T. M. Heenan, R. Jervis, J. J. Bailey, N. T. Vo, O. V. Magdysyuk and M. Drakopoulos, Adv Sci, 5, 1700369 (2018).
6. P. Ribière, S. Grugeon, M. Morcrette, S. Boyanov, S. Laruelle and G. Marlair, Energ Environ Sci, 5, 5271 (2012).
7. F. Larsson, S. Bertilsson, M. Furlani, I. Albinsson and B.-E. Mellander, J Power Sources, 373, 220 (2018).
8. S. Koch, A. Fill and K. P. Birke, J Power Sources, 398, 106 (2018).
9. A. W. Golubkov, D. Fuchs, J. Wagner, H. Wiltsche, C. Stangl, G. Fauler, G. Voitic, A. Thaler and V. Hacker, RSC Adv, 4, 3633 (2014).
10. A. W. Golubkov, S. Scheikl, R. Planteu, G. Voitic, H. Wiltsche, C. Stangl, G. Fauler, A. Thaler and V. Hacker, RSC Adv, 5, 57171 (2015).
11. X. Feng, D. Ren, S. Zhang, X. He, L. Wang and M. Ouyang, Int J Electrochem Sci, 14, 44 (2019).
12. S. Yayathi, W. Walker, D. Doughty and H. Ardebili, J Power Sources, 329, 197 (2016)
13. D. P. Finegan, B. Tjaden, T. M. Heenan, R. Jervis, M. Di Michiel, A. Rack, G. Hinds, D. J. Brett and P. R. Shearing, J Electrochem Soc, 164, A3285 (2017).
14. T. Yokoshima, D. Mukoyama, F. Maeda, T. Osaka, K. Takazawa, S. Egusa, S. Naoi, S. Ishikura and K. Yamamoto, J Power Sources, 393, 67 (2018).
15. E. Sahraei, J. Campbell and T. Wierzbicki, J Power Sources, 220, 360 (2012).
16. E. Sahraei, E. Bosco, B. Dixon and B. Lai, J Power Sources, 319, 56 (2016).


Website: https://www.nrel.gov/research/staff/donal-finegan.html https://orcid.org/0000-0003-4633-560X
17. S. Arora, W. Shen and A. Kapoor, Renew Sustain Energy Rev, 60, 1319 (2016)
18. E. Darcy, J. Darst, W. Walker, D. Finegan and P. Shearing, Driving Design Factors for Safe, High Power Batteries for Space Applications, in Advanced Automotive Battery Conference (2018).
S. L. Rickman, R. J. Christie, R. E. White, B. L. Drolen, M. Navarro and P. T. Coman, in 46th International Conference on
V. Ruiz, A. Pfrang, A. Kriston, N. Omar, P. Van den Bossche and Underwriters Laboratories, UL 9540 Energy Storage Systems
E. Castro, NASA-JSC Memo EP-19-001: Interpretation Memo for the Battery TR Propagation Requirements in JSC 20793 Rev JSC-20793 Rev D, Crewed Space Vehicle Battery Safety
E. Darcy, B. Strangways, C. McCoy, and T. Adams, Detecting Subtle Soft Shorts in Li-ion Cells, NASA Aerospace Battery

247th ECS Meeting Montréal, Canada
May 18-22, 2025
Palais des Congrès de Montréal
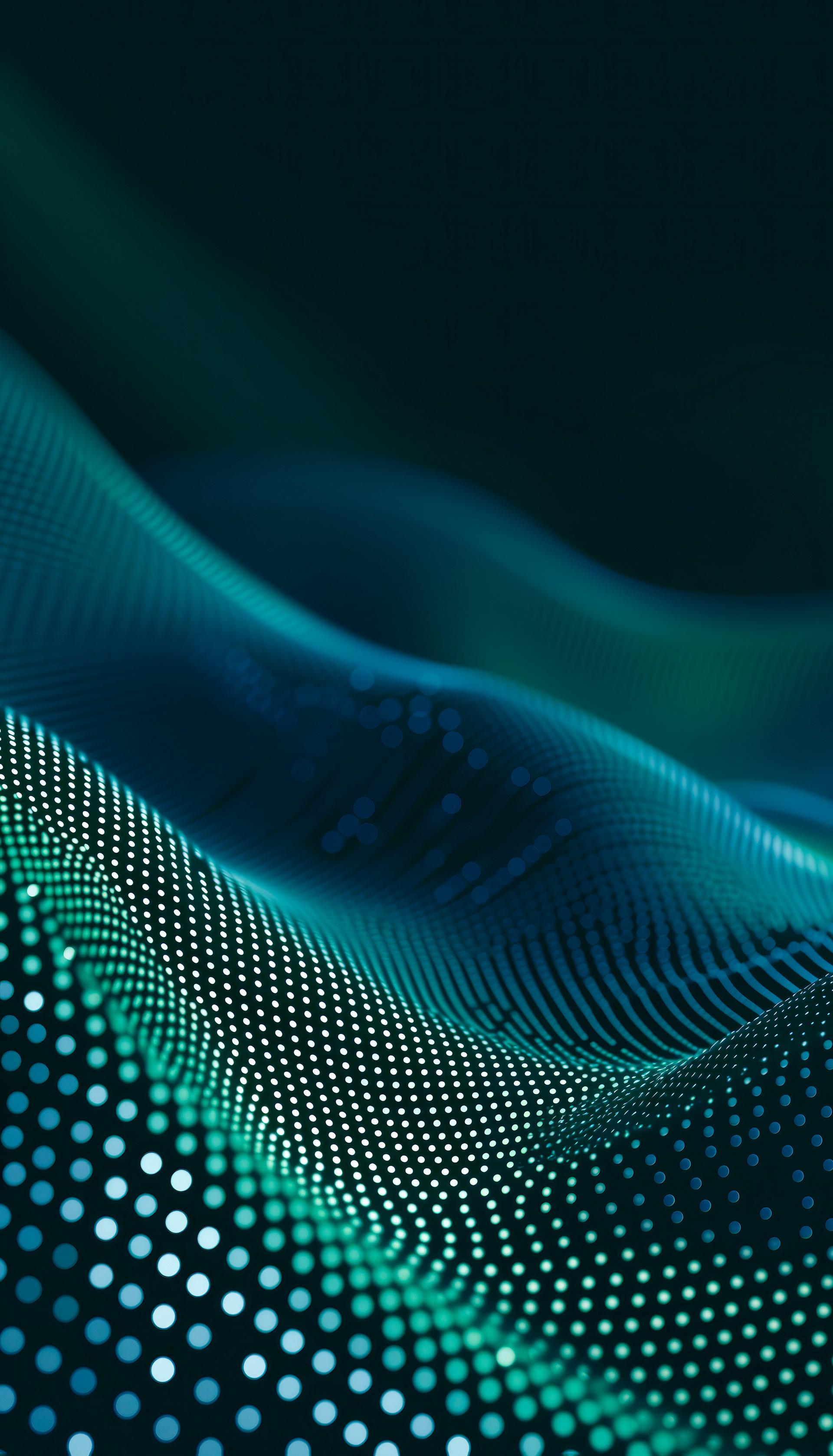
Abstracts due December 6th
Focus Issue on Trends and Biohybrid Approaches in Microbial Electrochemical Systems
The Journal of The Electrochemical Society is publishing a collection of papers on trends and biohybrid approaches in microbial electrochemical systems.
Papers sought on:
• Innovative applications of microbial electrochemical systems such as the application for self-powered biosensing, bioelectrosynthesis, and biosolar cells
• Novel techniques for the study and characterization of microbial electrochemical systems
Guest Editors
Olja Simoska, University of South Carolina
Matteo Grattieri, Università degli studi di Bari
Ariel Furst, Massachusetts Institute of Technology
Jeffrey Halpern, University of New Hampshire
Sanela Martic, Trent University
Technical Editor
Janine Mauzeroll, McGill University
Editor-in-Chief
Robert Savinell, Case Western Reserve University
Submission Deadline EXTENDED: November 30, 2024
Modeling of Li-ion Battery Thermal Runaway: Insights into Modeling and Prediction
by Paul T. Coman, Andrew Weng, Jason Ostanek, Eric Darcy, Donal P. Finegan, Ralph E. White
Complexities of Thermal Runaway
Lithium-ion battery safety has become one of the most discussed topics in recent years, not only as a subject of debate but also as a mandatory requirement driven by industry standards for deploying high-reliability battery power systems across all market applications. As more batteries enter the market in various applications, there is a strong incentive to improve safety and to mitigate associated consumer concerns to accelerate the adoption of electric vehicles (EVs) and other devices. The cornerstone of battery safety lies in understanding and mitigating thermal runaway (TR)—a failure mode characterized by a rapid, self-heating, and uncontrollable increase in temperature and pressure within a battery cell. This can lead to the venting of toxic gases, fires, or explosions, posing severe risks to both users and manufacturers.1,2 Determining whether a Li-ion battery meets industry safety requirements, or assessing the severity of a TR event, requires advanced knowledge of TR phenomena and relevant experimentation.
Despite advances in battery technology, the chaotic nature of TR makes it a complex phenomenon to model and, more critically, to predict its impact on battery pack design. Variability in manufacturing processes, material properties, and usage conditions means that even cells from the same batch can exhibit significantly different characteristics during TR.3-6 This unpredictability is compounded by the fact that TR is influenced by a multitude of interconnected factors, including electrochemical reactions, thermal dynamics, electrical configuration, and mechanical stresses.
Understanding the complexity of TR requires sophisticated experimentation, empirical data, and robust modeling and simulation approaches that capture the phenomenon’s essential features. Physicsbased mathematical models are often indispensable for elucidating TR mechanisms. However, they must balance detailed accuracy with computational efficiency, often requiring assumptions, mathematical constructs, and simplifications, especially for battery packs. Celllevel TR understanding is insufficient; as batteries scale to modules and packs, the risk of TR propagation escalates. Therefore, modeling must extend to the pack level, incorporating cell interactions and the overall thermal management system. Ensuring battery pack safety is critical, as a single cell failure can trigger a cascade of exothermic decomposition reactions, potentially leading to pack failure.
The most significant challenge, however, remains the accurate prediction of TR events both in single cells and battery packs. Future research must focus on refining these models and developing advanced predictive tools that integrate probabilistic methods. Machine learning (ML) and artificial intelligence (AI) also offer promising data-driven avenues for enhancing predictive capabilities.7
This article discusses the dual challenges of modeling and predicting TR in li-ion batteries. It explores the current challenges of TR modeling, the methods for progressing from single cells to battery packs, and future directions involving the use of probabilistic methods and ML/AI to tackle this multifaceted issue. Accurate prediction of TR events remains a major challenge, especially because of the cellto-cell variability, but also due to parametrization, which requires complex experimentation or exhaustive experimental data for training data-driven ML models. Continued research and innovation in modeling and predictive techniques are essential for ensuring safer and more reliable battery systems.
Physics-Based Modeling Approaches
TR models often include detailed representations of the battery’s internal reactions and structural changes in a full-order modeling (FOM) setting using different models, including mechanical models; electrochemical, electrical, thermal, and models that include TR propagation; fire propagation, reactive flow, pressure change models; mechanical response; and decomposition reactions.8 These reactions involve the decomposition of the SEI (solid-electrolyte interphase) layer, anode, cathode, electrolyte, salt, binder, and other materials within the battery which can react with the electrolyte or with the other gases generated during TR.9,10 These reactions can be modeled and used to calculate the thermal energy within a cell or pack, but such an approach often lacks mechanical or gas flow, for example. Some advanced models include thermodynamic equations to calculate the amount of gas vented from the cell and to measure the mass of each battery component to provide important parameters for analyzing TR numerically.11 High-speed tomography has shown that TR can lead to the collapse of the electrodes and the structure, as well as the melting of the current-collecting materials, which also indicates that mechanical effects need to be included.12 These insights are vital for creating accurate and representative numerical models. Accounting for all the reactions and phenomena in a model is an extremely complex process which is not practical with current computational capabilities. Such a model requires many measured parameters and knowledge of the reactions that occur for each chemistry of interest.13 A typical physics-based model used for predicting single-cell TR behavior can be described with the equations in Table I.
As can be seen from Table I, the model describes the reactions that occur inside a cell during TR, but even this basic model requires at least 15 parameters that require complex experimental data. For instance, accelerating rate calorimetry (ARC) or differential rate calorimetry (DSC) is needed to determine the activation energy (the E-terms in the Table) and pre-exponential factor (the A-terms) for each cell under different conditions. This comprehensive physics-based modeling can lead to accurate temperature predictions, but it lacks some critical phenomena that are essential for understanding the real dynamics of TR.
Another key aspect of TR that needs to be incorporated is the venting of gas and ejecta. Venting plays a significant role in the overall TR process, as it involves the release of gases and materials from the cell, impacting the thermal, electrical, and mechanical behavior of the battery, but, in this specific case, adds at least 12 extra parameters that need experimentation, as seen in Table II.
But venting is also a complex phenomenon, which can be divided into two assumptions: 1) considering vapor-liquid equilibrium (VLE) assumption,15 and 2) considering conditions of non-equilibrium. In the latter approach,16 a non-equilibrium model using porous drying theory can be introduced. This evaporation process is described by the constant rate drying period (CRDP) and the decaying rate drying period (DRDP). This is important because evaporative cooling affects the time between venting and thermal runaway.17 Gas is also generated from decomposition reactions. The gas generation rate can be modeled in several ways. Simple constant rate assumptions are commonly employed, while other approaches include an analogy where heat (continued on next page)
(continued from previous page)
Table I. Set of equations describing the decomposition reactions modeled in typical physics-based models.10,11,14
generation and gas generation are proportional. For example, in Refs.11,15 it was assumed that the electrolyte decomposition reaction rate is proportional to the rate of electrolyte evaporation. The authors in Ref.16 made a similar assumption for the vent gas mixture.
Models can incorporate the venting of the electrolyte by setting up burst conditions for the trigger pressure of the battery relief mechanism, simulating venting. This approach includes the partial ejection of the jelly roll, where the amount of ejecta is measured and incorporated into the model.
The processes involved in the electrolyte are complex and include evaporation, boiling, and venting, which can be accompanied by explosions of ejecta. In terms of boiling and evaporation, knowledge about the electrolyte composition is also required, because it influences the energy output.
For modeling the electrolyte, mixtures with specific compositions need to be known, and venting is modeled by assuming the electrolyte
behaves as an ideal gas flowing isentropically through an orifice, which opens at a critical pressure, but might be assumed to be a real gas during the VLE phase. The pressure and temperature during venting are defined by equations derived from the energy balance in a reversible system with compressible flow of an ideal gas passing through an orifice.
Additionally, another challenge is combustion outside the cell,18,19 which, in fact, can impact the TR behavior inside the cell. By incorporating gas venting, electrolyte thermodynamics, and combustion, the models can provide a more comprehensive understanding of TR and help identify critical areas for improving battery safety. An example of modeling prediction using a comprehensive physics-based model using the venting equations can be seen in Fig. 1.
Such a model can predict the heat dynamics that can be observed by measuring the temperature of the housing of a cell and can provide
a typical physics-based
Table II. Equations describing the reactions modeled in
model with venting.10,11,14
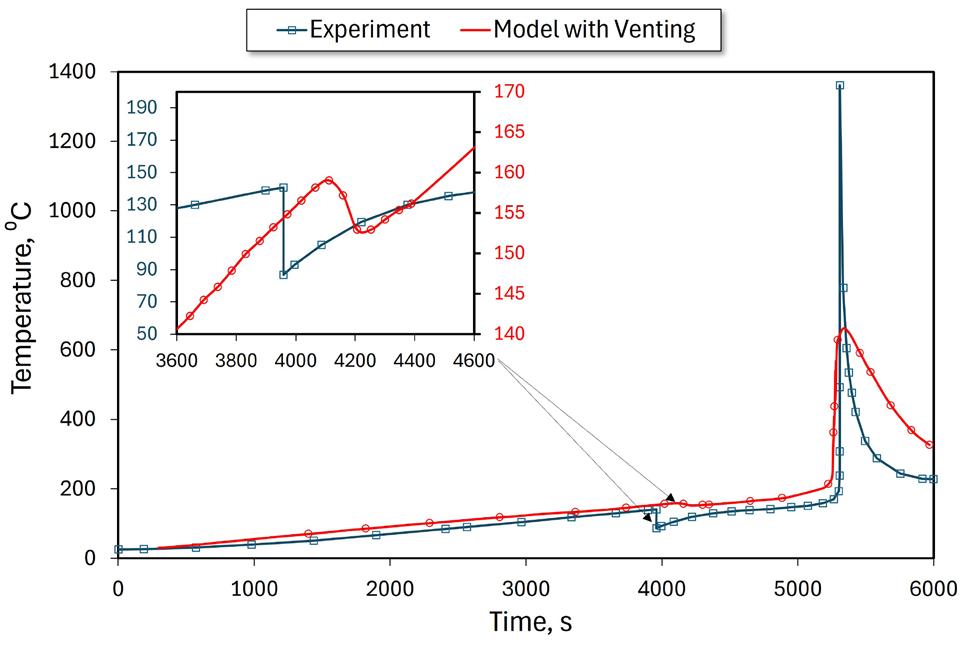
Fig. 1. Comparison between modeling predictions using a comprehensive physics-based model and experimental data. Incorporating venting equations also captures the Joule-Thomson/evaporative cooling effect in the pre-onset phase.11
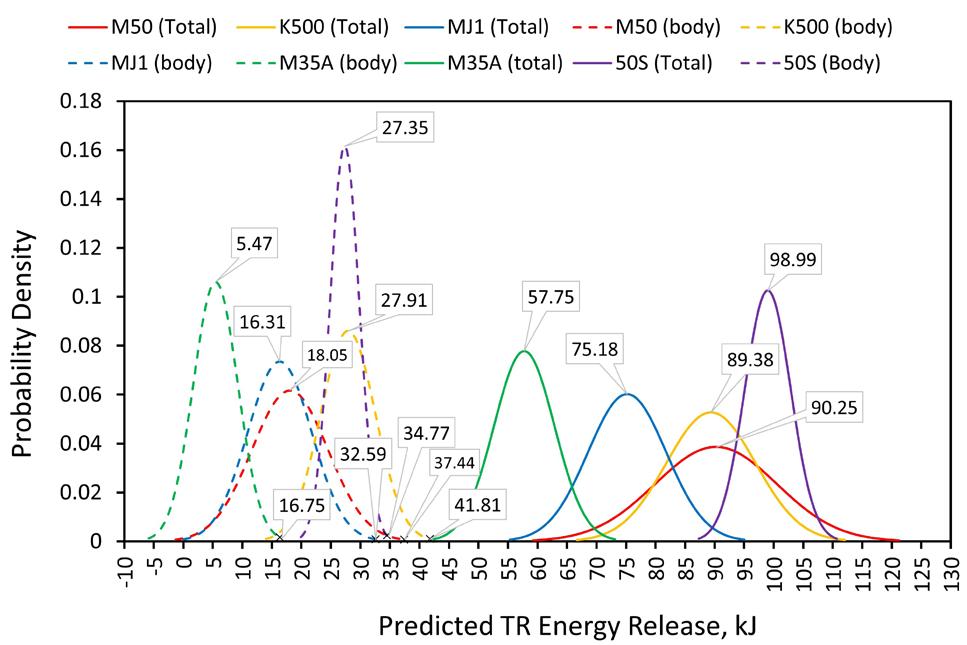
Fig. 2. Distribution of energy released during TR through the cell body vs. total, for the same type of 18650-format cells and chemistry calculated using a fractional thermal runaway calorimeter (FTRC).21
the energy released during various exothermic and endothermic reactions, including the gas-throttling Joule-Thomson/evaporative cooling effect20 that might occur before the TR onset point.
Although these FOMs offer a high fidelity in calculating temperatures and energy, they often require simplifications to manage computational efficiency while retaining essential details, while they also require a large number of parameters. Despite the complexity and the need for numerous parameters, these TR models can still be used to assist in the design of safe battery packs and to analyze various geometric designs. However, they cannot be used for prediction in their current form. Importantly, even when a physics-based model is set in place for a certain Li-ion chemistry, during TR, each cell yields different energy outputs even if they come from the same batch of cells. Consequently, there is a large distribution in terms of energy output during TR (Fig. 2).
Given the importance of ensuring battery safety, especially at the pack level, continuous research and development in TR modeling is crucial. Experimental data is essential for validating these models and for ensuring that they accurately reflect real-world behavior.
Reduced-order Thermal Runaway Models
One of the methods to model TR in battery packs is to find ways to reduce the computational burden. Reduced-order models (ROMs) are crucial for integrating detailed multi-scale simulations into practical engineering applications. These models aim to simplify the complex
behaviors captured in multi-scale simulations while retaining the essential characteristics needed for accurate predictions. By reducing the number of parameters and computational requirements, ROMs provide a more efficient way to analyze TR and support the rapid prototyping and evaluation of battery systems, even when analyzing complex phenomena like the venting of gases and expansion.22 They are instrumental in making advanced modeling techniques accessible for real-world applications, ensuring timely and cost-effective safety assessments. Some of the most common reduced-order methods, especially for battery packs, is to consider either reducing the thermal model and coupling with the electrochemical model23 or reducing the number of equations by coupling decomposition reactions24 (Fig. 3).
In Fig. 3, it can be seen that some of the reactions can be nested into a so-called efficiency factor, which greatly reduces the number of parameters that need to be fitted, estimated (for example by knowing the cell capacity), or measured. Another common reduction method is to downscale a model from a 3D to a 2D model. In Fig. 4, it can be seen that a 3D-2D reduced order model can still predict the temperature measured experimentally without much loss in terms of precision for a single cell.
However, when scaling from cells to packs, additional parameters are added to the model, associated with the cell dimensions, heat transfer coefficients, and others, which adds more uncertainty in modeling. Using 3D or 2D FEA reduced models is the most desirable approach for analyzing battery packs, due to the presence of pack design piece-part electrical, mechanical, and thermal components, etc. However, a physics-based FOM is almost impossible because there are so many unknowns and because of cell-to-cell variability. A recent reducing order approach, where the 3D thermal model was reduced to a 2D model with a heat-generation profile generated by empirical observation (Fig. 5(a) and (b)) and combined with an efficiency factor.
Adding an efficiency factor is a good approach to assist the design of battery packs, as seen when comparing simulation with experimental data (Fig. 5(c)). Modeling TR in lithium-ion batteries can be approached through physics-based models, reduced-order models, or multi-scale models, each with its own set of advantages and challenges. Physics-based models provide a detailed understanding of the electrochemical and thermal processes involved, while reducedorder models offer computational efficiency by simplifying complex interactions. Multi-scale models integrate phenomena across different scales to provide a comprehensive view of TR dynamics.
Accurately Predicting TR: What’s Needed and Can AI Help?
Despite significant efforts in TR modeling, accurate TR predictions remain elusive. The main impediment to accurate predictions is the inherently stochastic nature of TR. Even cells from the same
(continued on next page)
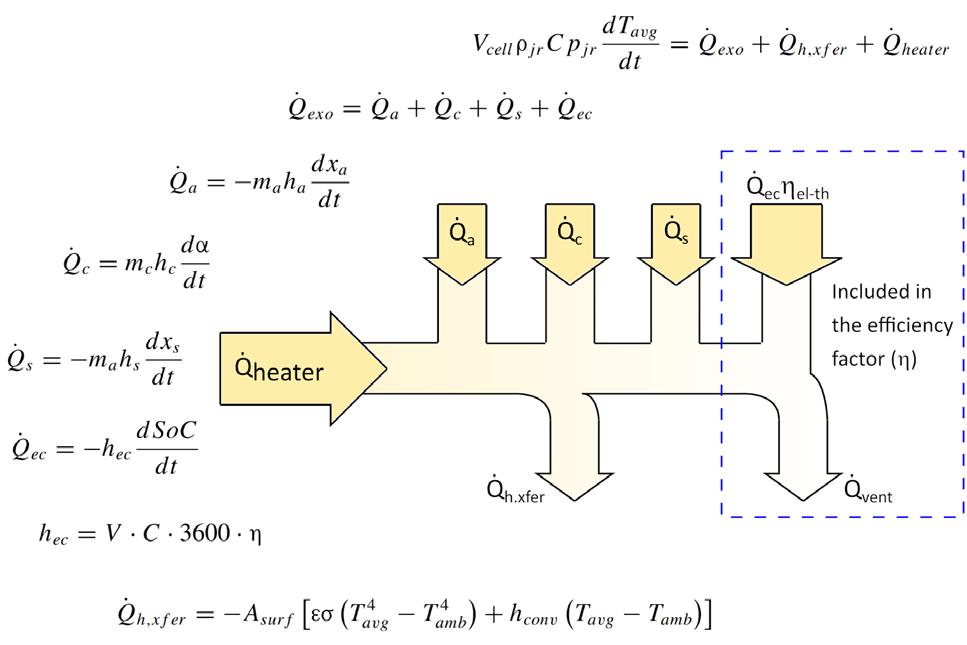
Fig. 3. ROM by subtracting the energy due to venting of electrolyte and ejecta from the total electrochemical energy and introducing the efficiency factor (η).24
(continued from previous page)
(a) (b)
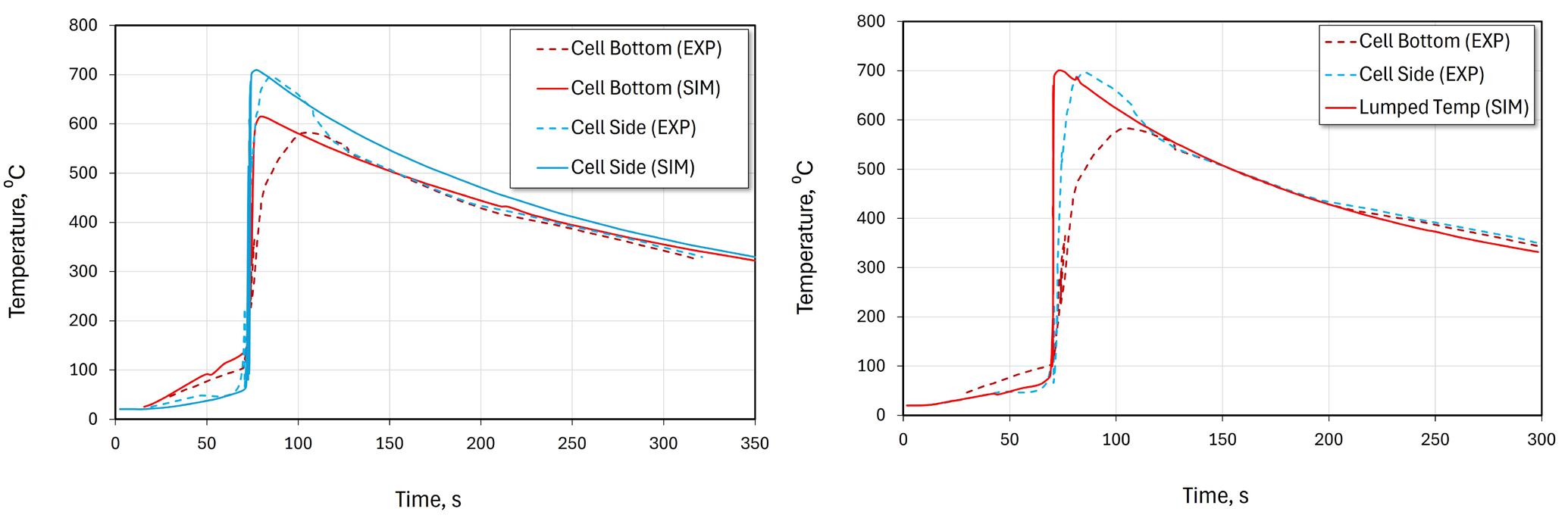
4. A 3D-2D reduced-order model which can fit experimental data (temperature measured on the side of the 18650-format cell steel can and at the bottom) without the burden of an FOM.24
production batch from the same manufacturer with the same dimensions, same chemistry, and same capacities can exhibit different TR characteristics. For example, Fig. 6 shows the variability in total energy released through the cell casing for a variety of cell types. For some cell types, the energy released can vary from 2% to 30%, highlighting the extremely stochastic nature of TR. This high degree of variability in TR propagation is an inherent attribute of the system which exhibits high sensitivity to initial conditions.
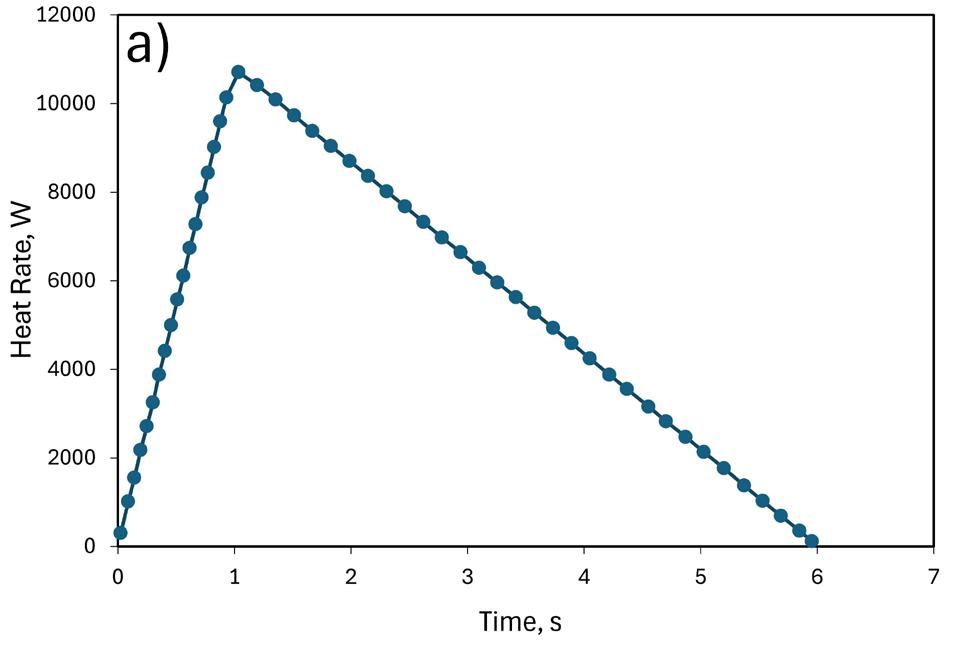
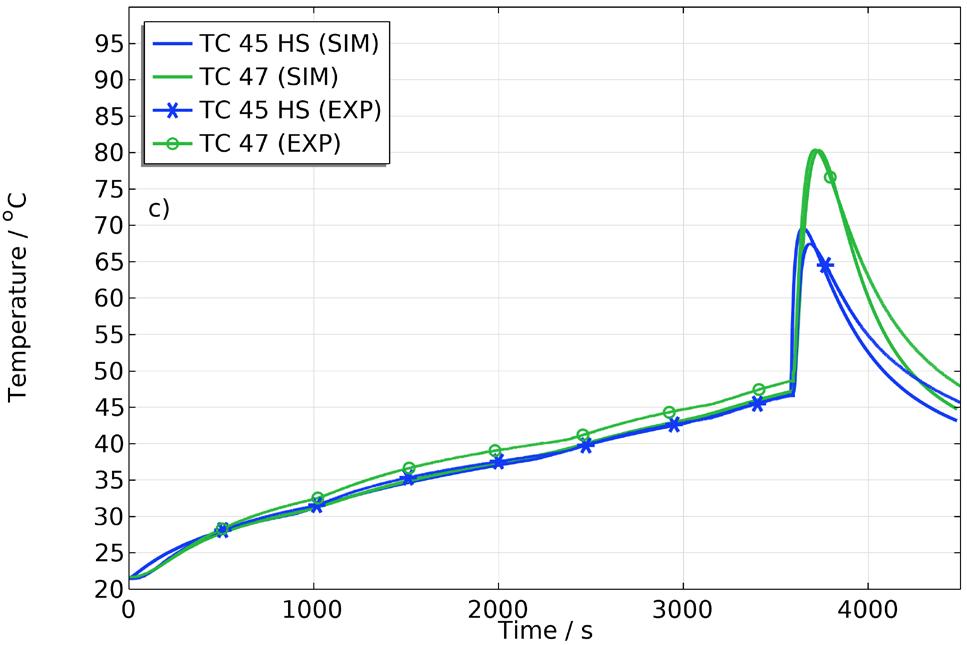
The stochastic nature of TR calls for the use of more probabilistic frameworks for TR prediction. Existing prediction methods tend to be deterministic and thus only predict a single TR outcome. This predicted outcome is not necessarily “wrong,” but it can represent only one outcome when a range of different outcomes are possible. For physics-based TR models, a future approach could be to combine existing deterministic simulations with Monte Carlo simulation approaches in which the model is repeatedly run to generate a
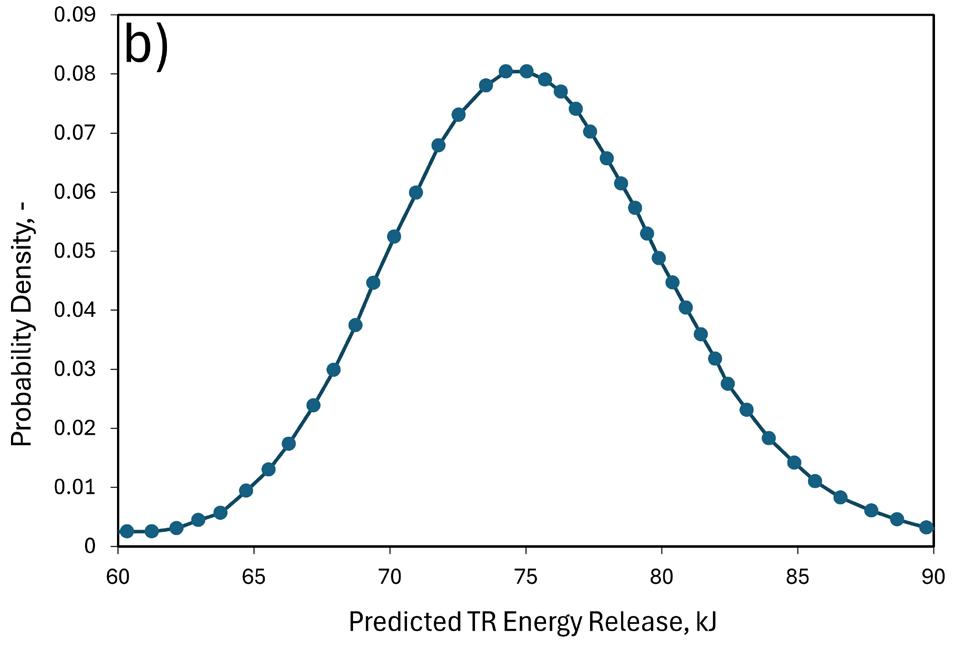
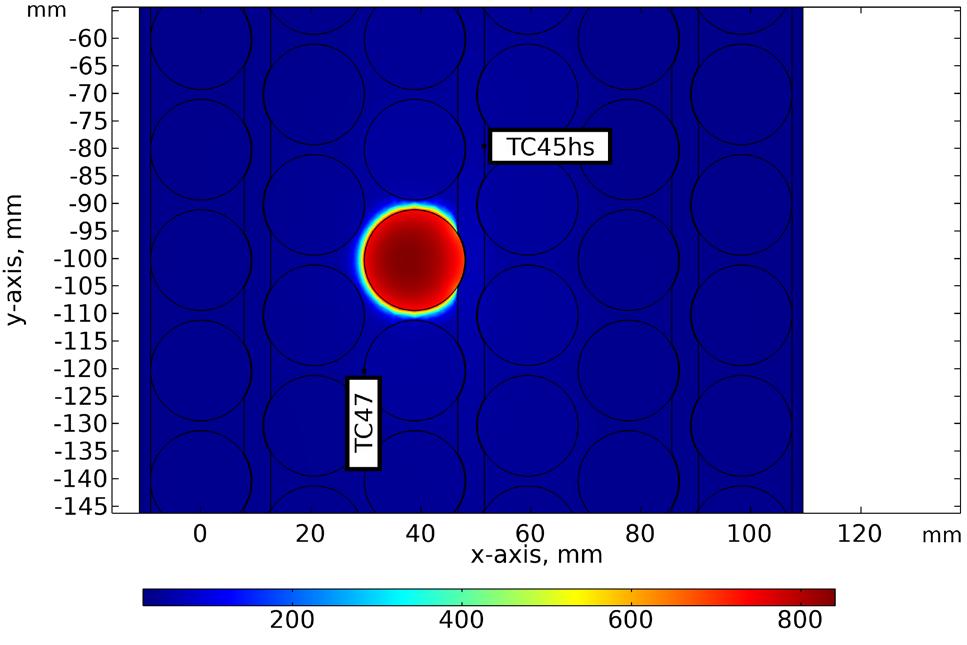
Fig.
Fig. 5. The results from the ROM depicting: (a) the empirically observed heat generation profile, (b) the thermal runaway energy (TR) released through the single-cell cans, and (c) left and right, the comparison between the modeling predictions and experimental data for a battery pack.25
(c)
(a) (b)
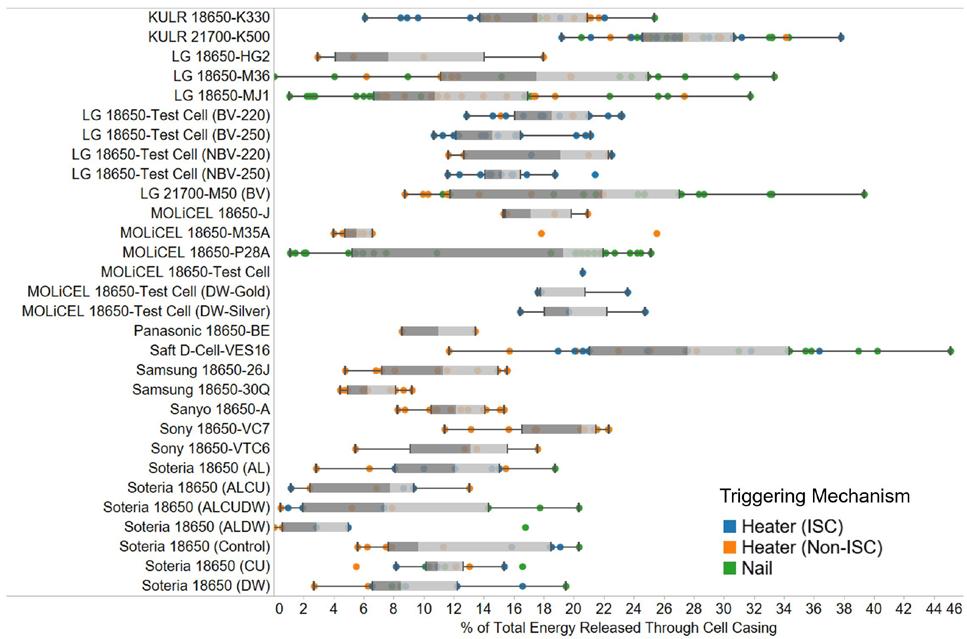
Fig. 6. Variability in total energy released through cell casing. Dataset adapted from the Battery Failure Databank (NREL).3
distribution of outcomes. Within each run, model parameters, such as those listed in Table I and Table II, are randomly sampled based on distributions of values that are either measured or assumed. It is not yet clear how many runs are necessary to accurately capture the failure distributions of typical commercial cells. When computation time becomes a bottleneck, ROMs can be used in place of FOMs. Thus, fast and accurate ROMs of TR may play an important role in enabling stochastic TR predictions.
AI/ML models, specifically artificial neural network (ANN) models, may still play a role in enabling accurate TR predictions, though many challenges remain. ANN models provide some inherent representation of stochasticity since the nodes in the layer can be interpreted as a probability (e.g., of TR occurrence). ANN models are also fundamentally data-driven without requiring knowledge of the complex, often intractable, multi-domain physics of TR. Recently, physics-informed neural networks (PINNs)26 which combine the advantages of both types of models27,28 have also been proposed. As of today, the literature on ANN models for TR prediction applications focuses on prediction of battery heat generation,29 temperature estimation,30,31 and surrogate modeling for FEM simulations.32 These methods show the feasibility of physical-state predictions without the need to construct a physical model. However, the accuracy of such models when applied beyond the immediate dataset they were trained and tested on remains unclear.
Ultimately, the availability of reliable experimental TR data is the biggest impediment to building and validating any kind of TR model. Large amounts of experimental data are especially needed for AI/ML approaches whose accuracy and generalizability largely depend on the quality and volume of data used to train the model. Comparatively, AI/ML methods need the most data, followed by FOMs, followed by ROMs. The stochastic nature of TR further means that even more measurements are needed to resolve distributions of outcomes. Given the difficulty of TR experiments and the existing sparsity of data, community efforts are needed to build and curate large TR datasets for model training and validation. The NREL/NASA Battery Failure Databank3 is the first effort of its kind and should be viewed as a promising beginning to an effort that must be continued and expanded.
Summary
Modeling TR in lithium-ion batteries can be approached through physics-based models, reduced-order models, or multi-scale models, each with its own set of advantages and challenges. Physics-based models provide a detailed understanding of the electrochemical and thermal processes involved, while reduced-order models offer computational efficiency by simplifying complex interactions. Multiscale models integrate phenomena across different scales to provide a comprehensive view of TR dynamics. Despite these advancements, accurate prediction of TR events remains a major challenge due to the chaotic and highly variable nature of the phenomena.
Future research must continue to refine these models and explore the integration of probabilistic approaches and AI/ML to enhance predictive capabilities. These advanced techniques hold the potential to significantly improve battery safety and reliability. However, predicting the dynamics of TR in a battery pack from the beginning remains challenging and requires a large volume of data for both individual cells and packs. The continuous development of probabilistic approaches and AI/ML models, combined with comprehensive data collection and real-time monitoring, is essential for overcoming these challenges and ensuring the safe and efficient operation of lithium-ion batteries.
©The Electrochemical Society. DOI: 10.1149/2.F09243IF
About the Authors
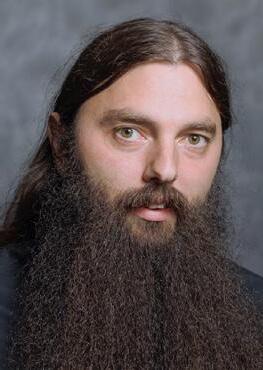
Paul T. Coman, Associate Research Professor, Chemical Engineering, University of South Carolina
Education: PhD in Applied Mathematical
Modeling (University of Southern Denmark)
Research Interests: Li-ion batteries, Battery safety, Electrochemical modeling
Work Experience: Post-doctoral Fellow, Assistant and Associate Research Professor at the University of South Carolina working with mathematical modeling of Li-ion batteries. Over the years, Dr. Coman has guided the design of safe battery packs for NASA.
Work with ECS: ECS Member
Awards: Electrochemical Society (ECS) Battery Division
Postdoctoral Associate Research Award
Pubs + Patents: > 20 papers https://orcid.org/0000-0003-2876-7441


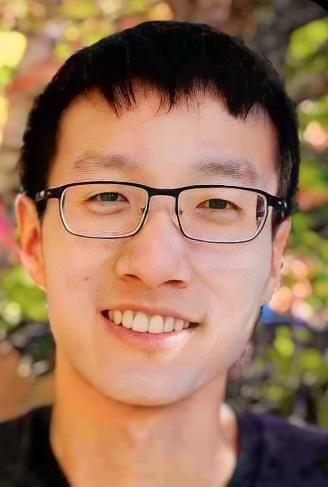
Andrew Weng, Postdoctoral Fellow, Chemical Engineering, University of South Carolina
Education: PhD (University of Michigan)
Research Interests: Li-ion batteries, Electrochemical modeling
Work Experience: Senior Cell Research Engineer, Tesla (2017–2021), Cell Test Engineer, Tesla (2014–2017)
Work with ECS: ECS Member
Awards: S. M. and Benjamin Wu Fellowship in Manufacturing, University of Michigan (2023), Dwight F. Benton Fellowship and Forrest Student Fellowship, University of Michigan (2020)
Pubs + Patents: > 15 papers
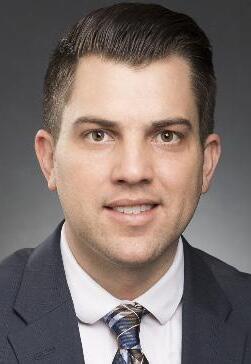
Jason Ostanek, Associate Professor, School of Engineering Technology, Purdue University
Education: PhD in Mechanical Engineering (Penn State)
Research Interests: Li-ion safety, Thermal runaway, Venting, Combustion, Heat transfer
Work Experience: Assistant Professor, Purdue University 2017–2023, Research Scientist, Naval Surface Warfare Center Philadelphia Division 2012–2017
Work with ECS: ECS Member
Awards: Purdue Teaching Academy’s Award for Exceptional Teaching and Instructional Support during the COVID-19 Pandemic (2020), SOET Outstanding Faculty in Engagement (2020), SOET Outstanding Faculty in Discovery (2019), First Annual NAVSE Commander’s Award for Innovation (2015)
Pubs + Patents: > 30 papers
Website: https://polytechnic.purdue.edu/facilities/appliedthermofluids-laboratory https://orcid.org/0000-0002-7724-3110


(continued on next page)
(continued from previous page)
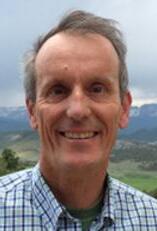
Eric Darcy, Battery Technical Discipline
Lead, NASA Johnson Space Center
Education: PhD in Chemical Engineering (University of Houston)
Research Interests: Battery design, Li-ion batteries, Battery safety
Work Experience: 37-year career at NASA in the areas of battery design, verification, and safety assessments for the rigors of manned spacecraft applications. As Battery Technical Discipline Lead at NASA-JSC, his main objective has been the development of safe, while high performing, battery systems with a deep focus on understanding, preventing, and mitigating latent defects that could lead to catastrophic cell internal short circuits.
Awards: NASA invention of the year 2023, lifetime achievement award by NAATBatt
Pubs + Patents: >10 publications, >2 patents
Website: https://www.nasa.gov/people/dr-eric-darcy-nesc-academybiography/
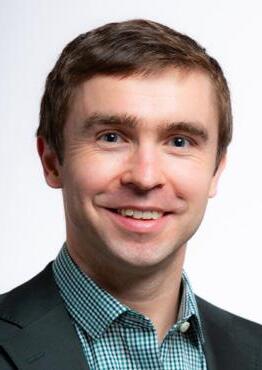
Donal Finegan, Researcher IV-Chemical
Engineering, National Renewable Energy Laboratory (NREL)
Education: PhD in Chemical Engineering (University College London)
Research Interests: Characterization and diagnostics of energy materials, Li-ion batteries, Safety
Work Experience: Donal Finegan has seven years of experience working on energy storage technologies at the National Renewable Energy Laboratory. Finegan has led several projects focused on battery safety, scaling advanced manufacturing methods for Li-ion batteries, and characterizing degradation mechanisms of energy storage materials. He leads NREL’s X-ray computed tomography laboratory.
Work with ECS: Member.
Awards: The European Synchrotron Young Scientist Award (2020), NASA Johnson Space Center Directors Group Achievement Award (2019), Umicore Materials Technology Award (2018)
Pubs + Patents: >100 publications, 4 patents
4. W. Q. Walker, J. J. Darst, D. P. Finegan, G. A. Bayles, K. L. Johnson, E. C. Darcy, and S. L. Rickman, J Power Sources, 415, 207 (2019).
5. D. P. Finegan, J. Darst, W. Walker, Q. Li, C. Yang, R. Jervis, T. M. M. Heenan, J. Hack, J. C. Thomas, A. Rack, et al., J Power Sources, 417, 29 (2019).
6. D. P. Finegan, E. Darcy, M. Keyser, B. Tjaden, T. M. M. Heenan, R. Jervis, J. J. Bailey, R. Malik, N. T. Vo, O. V. Magdysyuk, et al., Energy Environ Sci, 10, 1377 (2017)
7. A. Li, J. Weng, A. C. Y. Yuen, W. Wang, H. Liu, E. W. M. Lee, J. Wang, S. Kook, and G. H. Yeoh, J Energy Storage, 60, 106688 (2023)
8. G. Wang, P. Ping, D. Kong, R. Peng, X. He, Y. Zhang, X. Dai, and J. Wen, Innovation, 5, 100624 (2024).
9. R. Spotnitz and J. Franklin, J Electrochem Soc, 113, 81 (2003)
10. T. D. Hatchard, D. D. MacNeil, A. Basu, and J. R. Dahn, J Electrochem Soc, 148, A755 (2001)
11. P. T. Coman, S. Rayman, and R. E. White, J Power Sources, 307, 56 (2016)
12. D. P. Finegan, M. Scheel, J. B. Robinson, B. Tjaden, I. Hunt, T. J. Mason, J. Millichamp, M. Di Michiel, G. J. Offer, and P. R. Shearing, Nat Commun, 6, 1 (2015)
13. A. W. Golubkov, D. Fuchs, J. Wagner, H. Wiltsche, C. Stangl, G. Fauler, G. Voitic, A. Thaler, and V. Hacker, RSC Adv, 4, 3633 (2014)
14. G. Kim, A. Pesaran, and R. Spotnitz, J Power Sources, 170, 476 (2007).
15. P. T. Coman, S. Mátéfi-Tempfli, C. T. Veje, R. E. White, P. T. Coman, C. T. Veje, and R. E. White, J Power Sources, 164, 1858 (2017).
16. J. K. Ostanek, W. Li, P. P. Mukherjee, K. R. Crompton, and C. Hacker, Appl Energy, 268, 114972 (2020).
17. M. Parhizi, K. R. Crompton, and J. Ostanek, in ASME International Mechanical Engineering Congress and Exposition, Proceedings (IMECE), vol. 8A-2021 (2021).
18. F. A. Yravedra and Z. Li, Electr J, 34, 106887 (2021).
19. J. T. Kim, J. Y. Choi, S. Kang, N. G. Han, and D. K. Kim, J Energy Storage, 60 (2023)
20. B. D. Horbaniuc, in Encyclopedia of Energy, vol. 5, p. 261–289, Elsevier (2004).
21. D. Petrushenko, P. Coman, J. Trillo, B. Esparza, R. White, E. Darcy, J. L. Moyar, J. R. Izzo, T. E. Adams, and J. H. Fontaine, in NASA Aerospace Battery Workshop, Huntsville, AL (2023).


Website: https://www.nrel.gov/research/staff/donal-finegan.html https://orcid.org/0000-0003-4633-560X
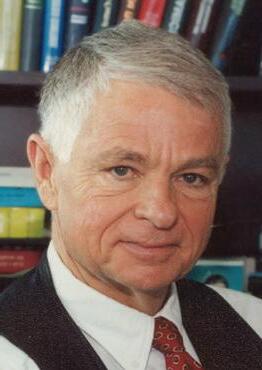
Ralph E. White, Professor, University of South Carolina
Education: PhD in Chemical Engineering (University of California, Berkeley)
Research Interests: Fuel cells, Batteries, Electrodeposition, Corrosion, Numerical methods
Work Experience: Assistant Professor (1977–1981), Associate Professor (1981–1985), Professor (1985–1993) of Chemical Engineering, Texas A&M University
Work with ECS: ECS Fellow
Awards: ECS Olin Palladium Award (2013) Vittorio de Nora Award (2016) Henry B. Linford Award (2018)
22. V. Tran, J. B. Siegel, and A. G. Stefanopoulou, J Electrochem Soc, 171, 060507 (2024).
23. C. Xu, H. Wang, F. Jiang, X. Feng, L. Lu, C. Jin, F. Zhang, W. Huang, M. Zhang, and M. Ouyang, Energy, 268, 126646 (2023)
24. P. T. Coman, E. C. Darcy, C. T. Veje, and R. E. White, J Electrochem Soc, 164, A1 (2017).
25. P. T. Coman, E. C. Darcy, and R. E. White, J Electrochem Soc, 169, 040516 (2022)
26. S. W. Kim, E. Kwak, J.-H. Kim, K.-Y. Oh, and S. Lee, J Energy Storage, 60, 106654 (2023).
27. D. P. Finegan, J. Zhu, X. Feng, M. Keyser, M. Ulmefors, W. Li, M. Z. Bazant, and S. J. Cooper, Joule, 5, 316 (2021).
28. M. Raissi, P. Perdikaris, and G. E. Karniadakis, J Comput Phys, 378, 686 (2019)
29. S. Arora, W. Shen, and A. Kapoor, Comput Chem Eng, 101, 81 (2017)


Pubs + Patents: >300 publications https://orcid.org/0000-0003-3889-8568
References
1. F. Larsson, P. Andersson, P. Blomqvist, and B. E. Mellander, Sci Rep, 7, 10018 (2017)
2. P. J. Bugryniec, E. G. Resendiz, S. M. Nwophoke, S. Khanna, C. James, and S. F. Brown, J Energy Storage, 87, 111288 (2024)
3. D. P. Finegan, J. Billman, J. Darst, et al., J Power Sources, 597, 234106 (2024).
30. M. Wang, W. Hu, Y. Jiang, F. Su, and Z. Fang, Int J Energy Res, 45, 13681 (2021)
31. J. Kleiner, M. Stuckenberger, L. Komsiyska, and C. Endisch, J Energy Storage, 39, 102588 (2021)
32. F. Kolodziejczyk, B. Mortazavi, T. Rabczuk, and X. Zhuang, Int J Heat Mass Transf, 172 (2021)
A Multi-scale Framework for Advancing Battery Safety Through Early Calorimetric Analysis of Materials and Components
by Alex Bates, Loraine Torres-Castro, Paul Albertus, Partha P. Mukherjee, Judith Jeevarajan, Nathan Johnson, Bhuvsmita Bhargava, Zixuan Wang, Taiwo Ogundipe, Bairav S. Vishnugopi, Avijit Karmakar, Wan Si Tang
Batteries are the cornerstone of the global shift toward electrification, powering applications from passenger transportation, like electric vehicles (EVs), to load shifting of renewable energy generation at the grid level. The demand for batteries is accelerating, and is projected to nearly quadruple by 2030.1 In response to increasing battery demands, in particular demand for longer range EVs, higher energy density and specific energy alternatives to conventional Liion battery technologies are being researched.2 However, higher energy density directly correlates with an increased potential for higher peak temperatures on failure.3 In addition, safety evaluation of conventional and new battery technologies generally occurs late in development, often at the commercialization stage when mandated by stakeholders or industry standards. This approach can be costly and challenging as it may require a change to manufacturing processes, a re-design of the battery, and even a change in chemistry to meet safety requirements. This is critical; scale-up efforts alone can take tens to hundreds of millions of dollars and >5 years to achieve.4 Safety risks discovered after commercialization can significantly exacerbate those figures. Furthermore, safety is still a major risk with already commercialized Li-ion batteries. These risks hinder adoption, slow commercialization, and when they become issues, the outcome may result in recalls, fires, injuries, and even fatalities. Providing additional levers to proactively mitigate safety risk at the materials and components scale (e.g., composite cathode) will lead to considerable time and money savings in the long run.
New technologies, such as solid state batteries (SSBs) and Na-ion cells, are often assumed to be safe even before any work has gone into validating safety. This assumption, along with the requirement for higher capacity cells in current safety standards, often results in the deferment of safety evaluation until late in development. In recent years, it has become evident that some newer technologies are more hazardous (i.e., higher maximum temperature, faster heating rate, toxic gas generation, etc.) than they were previously believed to be. For example, Na-ion is currently being marketed as a safer alternative to Li-ion. However, even with its lower energy density and specific energy, recent studies show that Na-ion exhibits similar safety hazards to, and in some cases more severe outcomes than, commercially available Li-ion cells.5-8 A more established example of cells being pushed to commercialization before safety was well understood comes from Li-metal anode cells in the late 1970s through the early 1990s, with companies like Exxon, Duracell, Moli Energy, and Toshiba.9 These cells suffered devastating blows due to safety events that occurred after cell commercialization. In the case of Moli Energy, large recalls occurred after several fire incidents that caused minor burns to users.10 Other companies experienced similar setbacks resulting in the loss of millions of investment funds and years of research. That is not to say that the research investments were without benefit; however, the trajectory of the research might have been significantly different had safety been considered earlier in the technology’s development process.
The current approach to cell safety has the notable downside of missing the opportunity to address problems at the materials and components scales. For instance, if a certain formulation of cathode
material is found to have more exothermic reactivity with other cell components, changes can be made early in development to alleviate that hazard. However, once the manufacturing process has been established, and performance metrics achieved with a specific cathode formulation, changes become far more costly and time consuming. At that point, the most likely solution to safety risk at the cell level becomes an engineering response (e.g., current interrupt device (CID)) that does not solve the core of the problem; it merely applies a bandage with the hope of mitigating severe outcomes. In addition, pouch cell/battery engineering solutions are limited by the nature of the casing. As a result, pack and module solutions must be utilized, further removing the mitigation from the core problem.
In some cases, researchers do make efforts to examine and understand potential safety outcomes for specific failure modes that could lead to thermal runaway. However, they typically use coin cells or single-layer pouch cells for this purpose. Unfortunately, the capacity of these cells is too low to provide meaningful results due to noncommercial ratios and current density. For instance, the ratios among components often differ from commercial-sized cells, such as the ratio of casing to active material. When that ratio is high, internally generated heat dissipates quickly, and the temperature needed to sustain reaction propagation within the cell is not achieved, making the cell appear safer than it is.11, 12 In addition, new technologies may have failure modes not previously encountered, requiring new testing protocols. To improve the understanding of safety at an early stage of development, and with relevance to the safety response of multilayer, commercial-ready cells, a new safety strategy is needed.
A New Strategy for Materials and Components Scale Safety Evaluation
In this work, a new strategy for the safety evaluation of prospective cell technologies is discussed. At present, there is a high level of certainty in safety outcomes only at a later stage of development (the multi-layer battery in Fig. 1) conceptualized by the solid red arrow in Fig. 1. The overriding goal of a new safety evaluation strategy is to improve understanding with respect to safety at an earlier stage of development, which will lead to safer outcomes after commercialization. This is conceptualized by the color gradient arrow in Fig. 1. This Multi-Scale Framework for Safety Evaluation, or simply “Multi-Scale Framework,” leverages calorimetry, gas analysis, and characterization results for materials and components, while retaining carefully defined ratios, to quantify interactions, heat releases, onset temperatures, and generated gas compositions. The output parameters are then applied as inputs to a model that, through refinement and validation with known data sets, allows for the prediction of expected multi-layer cell safety outcomes. Subsequent iteration of the Multi-Scale Framework enhances certainty in the safety profile of a cell and provide avenues for achieving improved safety outcomes on large-format cells. Here, safety profile refers to the overall landscape of safety for a given cell including onset temperature, max temperature, released gas composition, etc.
(continued on next page)
(continued from previous page)
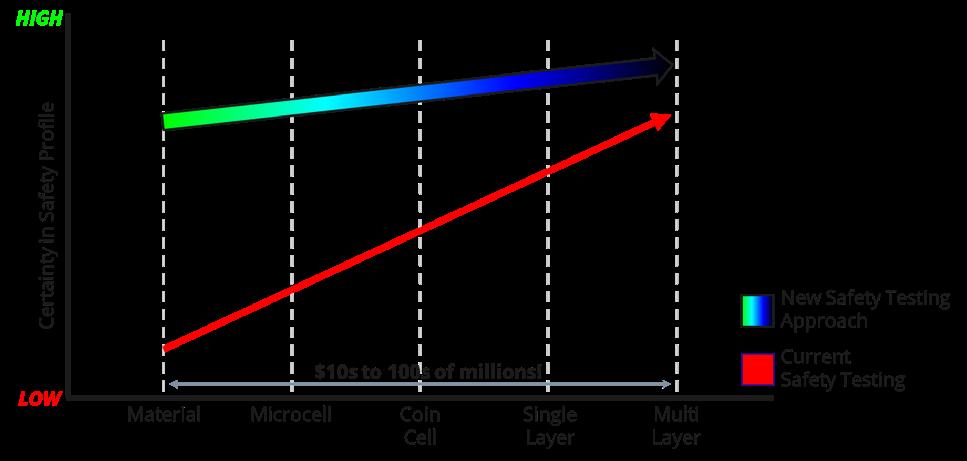
In summary, by using the Multi-Scale Framework approach, a new cell technology can be evaluated early in development, and, using the gathered data, the safety response predicted at larger scales. The Multi-Scale Framework is summarized in Fig. 2.
Materials and Components Scale Safety Profiling: An Experimental Approach
The first stage of the Multi-Scale Framework involves conducting a comprehensive Failure Mode Effects Analysis (FMEA). This analysis aims to thoroughly delineate the potential failure modes and to seek non-standard failure modes associated with new technologies. Once potential failure modes are identified, the next step is to analyze their effects. This involves describing each failure mode in detail and determining its consequences. For instance, a failure mode like thermal runaway could lead to severe outcomes such as fire or explosion, while electrode degradation might result in reduced battery performance over time. Both immediate and long-term effects need to be considered to fully understand the impact of each failure mode and guide design of targeted experiments and materials characterization efforts. As research progresses through the Multi-Scale Framework, it is expected that the FMEA will change, resulting in experimental modifications to address new concerns. The goal of the experiments and materials characterization is to understand which internal reactions contribute to significant exothermic events. To obtain this information, the Multi-Scale Framework starts with calorimetry. Differential scanning calorimetry (DSC) is a technique in which a sample chamber, containing two sample containers, is heated at a controlled ramp rate, on the order of 1-10 °C/min. The temperature of both containers is measured and any difference is attributed to endothermic heat flow if Tsample < Treference or exothermic heat flow if Tsample > Treference. This results in a collection of heat flows that are associated with changes in the target sample, such as phase changes,
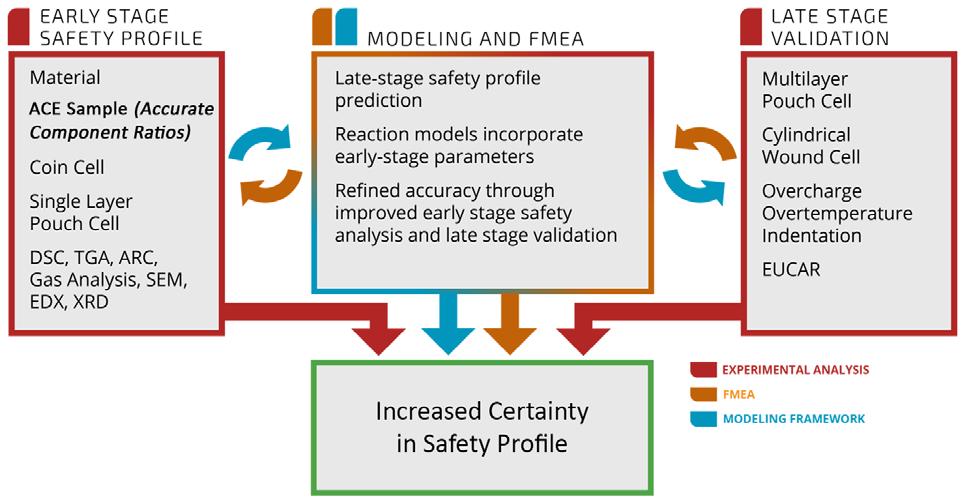
degradation, and reactions. For combinations of components, heat flows associated with additional interactions are also recorded. Examination of datasets produced by DSC provides insight into the temperatures at which reactions are taking place, the magnitude of their associated heat flows, and how different materials interact with each other.
The unique use of DSC in the Multi-Scale Framework described herein is the anode, cathode, and electrolyte (ACE) sample assembly (Fig. 3). An ACE sample contains, at minimum, the anode, cathode, and electrolyte used in a functioning electrochemical cell, but is not limited to only those components. For example, a conventional Li-ion battery contains a polymer separator that provides electrical isolation between electrodes, a space to contain electrolyte, and a pathway for ion transport. This polymer separator should be included in the DSC test as it has been shown to interact with other materials at high temperatures, impacting heat flow and available reactants. For the ACE sample, the components should be the same as, or representative of, what is in the chemistry/technology being evaluated, and in the correct ratios (e.g., a balanced negative to positive capacity ratio). Additionally, all components should be placed in the ACE sample in the form factor used in a battery (i.e., all components should be cell stack layers instead of powders). In addition, the upper temperature for calorimetry in this Multi-Scale Framework is about 500 °C, while it is often only 300 °C in studies that focus only on onset temperatures. The higher stop temperature of 500 °C allows for understanding of reactions between components that don’t decompose until higher temperatures, like electrode binders, and are important to understanding the hazards of thermal runaway. Higher temperature exothermic reactions can contribute significantly to peak temperatures during thermal runaway. Similar work has been explored previously by our team in Johnson et al. and by others.13-15
The preparation of components for ACE sample assembly and DSC experiments can have a significant impact on the heat flow signature of the sample. A very precise and targeted method must be adhered to for each sample run. To prepare electrodes for DSC experiments, cells should be assembled using the same electrolyte, separator, current collector, etc. that will be used in the target chemistry/technology. This can be tricky due to the unique nature of some cells and the ever-evolving materials and components used in advanced and beyond Li-ion technologies. After cell assembly, a minimum of three cycles at target operating conditions should be carried out to ensure proper cell function. Typically, it is advised to run at least one set (i.e., single components, combinations, and ACE samples) of DSC experiments, with repeats, using samples at 100% state-of-charge (SOC), which is often the most reactive state. There are some exceptions to this; for instance, electrodes that are fabricated in their 100% SOC state may be more reactive in their 0% SOC state (e.g., a conversion cathode like S in certain environments). Testing of single components and combinations aids in delineation of reaction
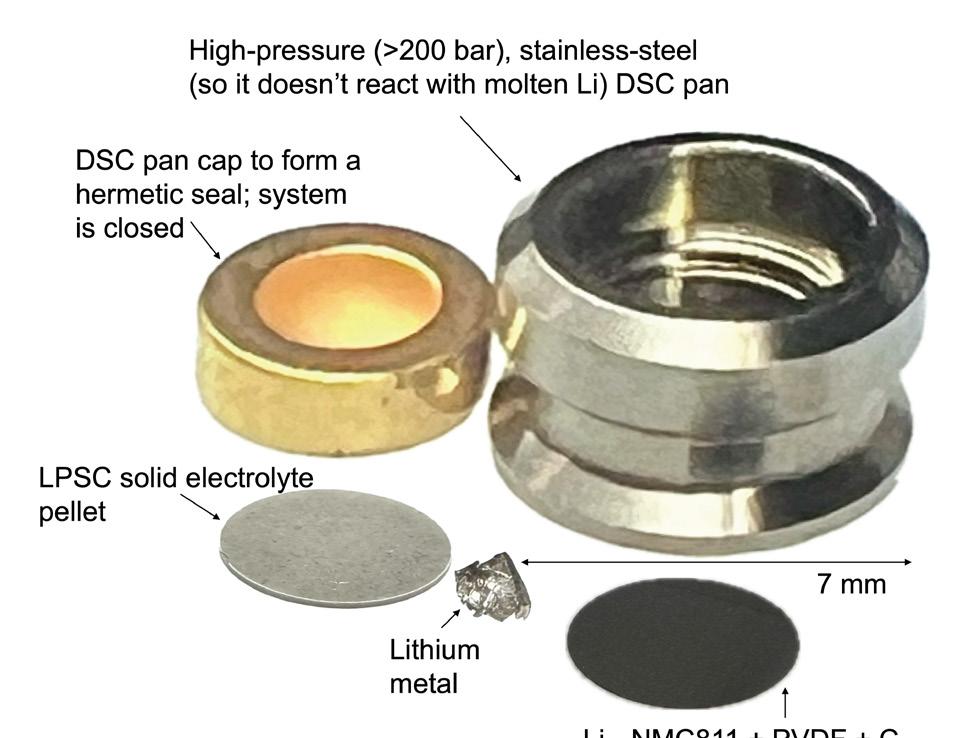
Fig. 1. Conceptual graphic for increasing certainty of safety outcomes at an early stage of development using the Multi-Scale Framework for Safety Evaluation.
Fig. 2. The Multi-Scale Framework for Safety Evaluation: an iterative methodology to improve safety understanding at an early stage of development.
Fig. 3. A high-pressure differential scanning calorimetry (DSC) sample pan with required battery cell components, in appropriate ratios, to assemble an anode, cathode, electrolyte (ACE) sample (LPSC = Li6PS5Cl).
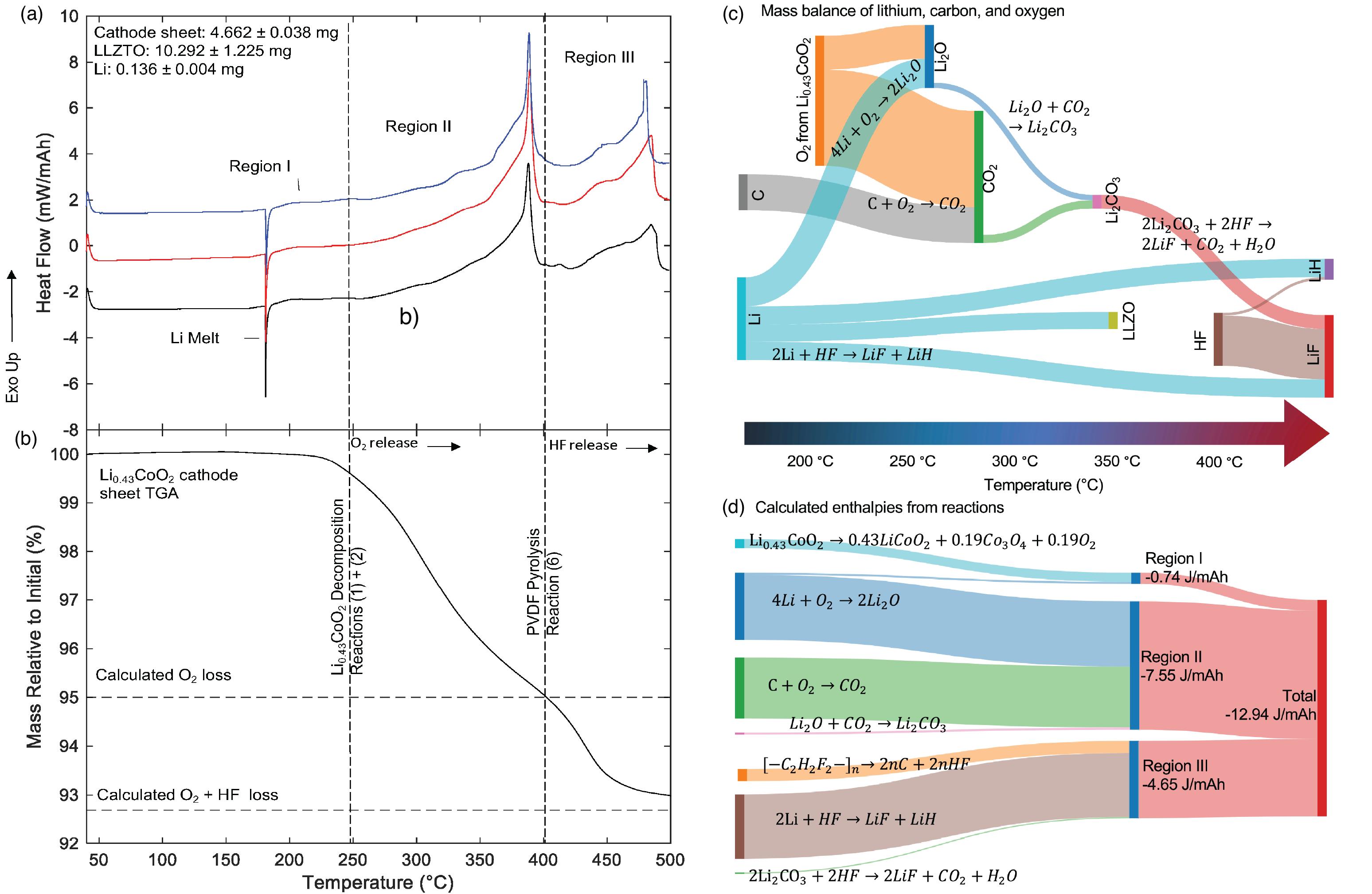
Fig. 4. Example of the experimental part of the Multi-Scale Framework which includes (a) differential scanning calorimetry (DSC) of anode, cathode, electrolyte (ACE) samples; (b) thermogravimetric analysis (TGA) of the cathode component; and (c) and (d) thermodynamic analysis of reaction pathways to pinpoint critical reactions and their heat flows (LLZTO = Li6.4La3Zr1.4Ta0.6O12). Reprinted with permission from N. B. Johnson, B. Bhargava, J. Chang, S. Zaman, W. Schubert and P. Albertus, ACS Applied Materials & Interfaces, 15, 57134 (2023). Copyright 2023 American Chemical Society.
pathways and crosstalk phenomena. To obtain charged materials, after cycling, and at 100% SOC, a cell is disassembled and the cell components harvested. In some cases, components are rinsed in a strategically selected solvent to remove electrolyte salt and prevent increased salt content during the DSC experiments. The components are then dried and cut to the internal diameter of the DSC pan, while ensuring the proper cathode-to-anode capacity ratio is maintained. Finally, components are stacked into the sample pan in the same orientation as their electrochemical cell target and, if utilized, liquid electrolyte is reintroduced. The ratio of electrolyte to anode, cathode, and separator volume is another critical ratio. Electrolyte is often a very reactive cell component; as such, the mass ratio of electrolyte to electrodes must be carefully maintained, or the heat contributions from the electrolyte will be over/underestimated. These controls aid in obtaining repeatable heat flows through multiple tests.
Carrying out DSC experiments on carefully constructed components, combinations, and ACE samples, combined with thermodynamic and kinetic knowledge of the reactions that may take place between the sample materials, provides a reasonable understanding of the reactions that will take place in a commercialized cell. Even so, the reactions that will take place in an ACE sample are not always easily understood. Complementary data can also be obtained by thermogravimetric analysis (TGA) using the same component preparation process as for DSC. TGA provides precise temperatures at which a gas-forming reaction or degradation step occurs by measuring the mass loss of the sample through a controlled heating rate. In addition, the TGA can be paired with mass spectrometry (MS) to characterize the outgoing gases. This information can help to validate or discern the exotherms seen
in DSC and give insight into the toxicity and/or flammability of the gases generated by the cell materials. This is a key consideration in some chemistries that have been suggested to release dangerous gas compounds. Beyond DSC and TGA, the Multi-Scale Framework employs materials characterization techniques (e.g., X-ray diffraction, X-ray photoelectron spectroscopy), where necessary, to aid in understanding and validating critical reaction pathways. These techniques can be invaluable to fill knowledge gaps in interpreting collected DSC/TGA data. It is possible to delineate thermochemical reaction pathways with reasonable confidence by utilizing the abovedescribed techniques, and while going to at least 500 °C in calorimetry Reactions that result in significant exothermic heat releases and the onset temperatures associated with those heat releases can then be identified. An example of the experimental approach of the MultiScale Framework is shown in Fig. 4(a–d).14
Fig. 4(a) shows three replicates of an ACE sample [Li | LLZTO | Li0.43CoO2+PVDF+C] in a DSC experiment at 2 °C/min to 500 °C. This sample and temperature range allow the measurement of several reaction regions and captures key behaviors such as Li melting, the initial release of O2 from Li0.43CoO2, the reaction of O2 with C and Li, PVDF breakdown, and the subsequent reaction of its products (HF) with the remaining Li. Fig. 4(a) includes the masses of each component (~1:1 N:P capacity ratio), and the replicates show that repeatable heat flows can be obtained with this method. Fig. 4(b) presents TGA results that provide additional quantitative insight into the reaction pathways taking place. In particular, the TGA results show the temperature at which O2 release from the Li0.43CoO2 cathode
(continued on next page)
(continued from previous page)
material begins, as well as the calculated mass loss, assuming the reaction goes to completion. It also shows the temperature at which PVDF breakdown occurs, and the total amount of mass loss for the completion of that breakdown reaction. Taken together, Fig. 4(a) and Fig. 4(b) provide complementary and quantitative information to inform the reaction pathways described in Fig. 4(c) and Fig. 4(d). To obtain the reaction pathways shown in Figs. 4(c) and (d), TGA data and known enthalpies of reaction are used in a heat and mass balance. The temperature-adjusted ∆Grxn was calculated to ensure all reactions were thermodynamically spontaneous. The extents of reaction, for possible reactions, were adjusted until the heat flow in each temperature region reasonably matched the DSC data, and the mass balance was satisfied. This chemistry is particularly advantageous because, in Region 2, there is one known principal oxidant (O2 gas) and two main reductants (C and Li), and the DSC heat flow at <400 °C can be used to determine the split between those reactions. Likewise, at >400 °C a second major oxidant is released from PVDF (HF), and the heat flow at >400 °C can be used to determine the extent of those reactions. A major conclusion from this analysis was that a significant portion of the O2 released from Li0.43CoO2 reacted with C rather than Li, and that seemingly minor cathode materials (C and PVDF) can play a major role in exothermic heat release. Through this example of the Multi-Scale Framework’s experimental approach, a collection of key data points were collected, designed to be used as inputs to the modeling approach.
Modeling to Create Multilayer Cell Safety Predictions
The next phase of the Multi-Scale Framework is to take materiallevel information determined from DSC, TGA, or accelerating rate calorimetry (ARC) experimental measurements (i.e., heat flow, onset temperature, temperature rise, etc.) as inputs to a physics-based celllevel thermal stability model.16-19 The temperature-dependent kinetics of the chemical reactions during thermal runaway with the associated heat release can be estimated based on kinetic expressions using a classical Arrhenius rate constant as long as the critical reaction parameters (i.e., activation energy, frequency factor, and reaction enthalpy) can be determined from calorimetric experiments. The kinetics of such exothermic reactions obtained from materials and components scale DSC/TGA/ARC signatures (e.g., Fig. 5(a)) can be combined to predict the net heat generation rate during the thermal runaway process in a typical large-format, production-ready cell. The developed cell-level model can be used to computationally predict large-scale safety outcomes under higher cell capacities, electrode active material loading, specific energy, crosstalk dynamics, abuse
scenarios (i.e., mechanical, electrochemical, or thermal), degradation, and operational parameters based on various applications. These factors are challenging to evaluate experimentally at higher scales. Crosstalk dynamics is a critical phenomenon in SSBs in which the mechanistic behavior of one electrode affects the response of the opposing electrode. Fig. 5(b) illustrates representative thermal stability maps for Li10SnP2S12 (LSPS)-based SSB cells constructed through the cell-level thermal runaway model. The map demonstrates that fsafety, representing the minimum fraction of specific energy that needs to be converted to heat to reach self-heating onset and cause thermal runaway, decreases with an increase in the specific energy of the SSB cell. Such modeling approaches will offer mechanistic material and cell design guidelines, play a pivotal role in the earlystage safety evaluation, and inform required changes to the FMEA, potentially uncovering new failure modes and thus reinforcing the feedback loop of the Multi-Scale Framework.
Conclusions and Outlook
New technologies often target higher energy densities and specific energies than the state-of-the-art Li-ion cell. Higher energy density and specific energy directly correlate with a greater potential for higher maximum temperature rise.2 That maximum temperature may or may not be realized in a production-ready cell, but the propensity for that realization should be better understood long before commercialization. The Multi-Scale Framework for Safety Evaluation presented here outlines an approach that utilizes a specified methodology for sample design and experiments at an early stage of development (materials and components) that, when combined with a thermodynamic understanding of material interactions and a validated modeling approach, provides a prediction, with higher certainty than previously achieved, of largescale safety outcomes. The Multi-Scale Framework’s application will bring safety assessments of batteries to the materials and components stage of development. By doing so, the community will have a better understanding of the risks and hazards associated with battery chemistries and technologies prior to expending large efforts on commercialization, thus enabling greater flexibility for reducing risks of commercialized cells by making informed decisions during cell development. The Multi-Scale Framework also allows for design of mitigation strategies earlier in development and throughout the development process. Ultimately, the framework must be validated with cells exceeding 2 Ah, as predictive models face limitations when translating from the materials scale to multi-layer cells. The behaviors and interactions observed at the materials scale often fail to account for the additional complexities inherent in larger systems, such as cell engineering, thermal management, mechanical stresses, and electrical connections. However, given the large number of new chemistries (e.g., Na-ion, variations of Li-metal, variations of SSB,
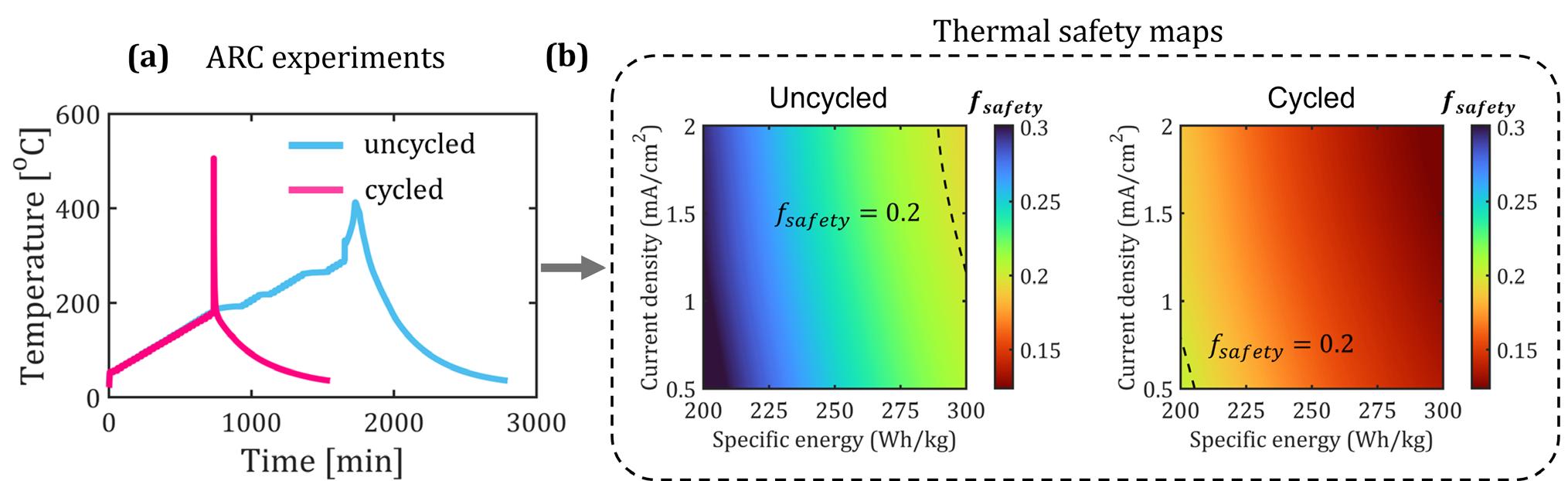
Fig. 4. (a) Representative thermal stability signatures from ARC experiments, (b) Modeling predictions that provide insight into the safety profile of advanced and beyond Li-ion batteries utilizing inputs from materials-scale calorimetry experiments. Reprinted with permission from B. S. Vishnugopi, M. T. Hasan, H. Zhou, and P. P. Mukherjee, ACS Energy Letters, 8, 398 (2023). Copyright 2022 American Chemical Society.
etc.) under development, the Multi-Scale Framework presented here will be critical for safety evaluation of prospective materials now and in the future.
This Multi-Scale Safety Framework is being employed in the EVs4ALL program, funded through the DOE’s ARPA-E. Stay tuned for our upcoming presentations and publications!
Acknowledgements
The information, data, or work presented herein was funded in part by the Advanced Research Projects Agency-Energy (ARPA-E), U.S. Department of Energy, under Award Number DE-AR0001722. The authors wish to thank Halle Cheeseman for his support of research advancing safety and reliability of advanced and beyond Li-ion battery technologies. Sandia National Laboratories is a multi-mission laboratory managed and operated by National Technology and Engineering Solutions of Sandia, LLC, a wholly owned subsidiary of Honeywell International, Inc., for the US Department of Energy’s National Nuclear Security Administration under contract DENA-0003525. This paper describes objective technical results and analysis. Any subjective views or opinions that might be expressed in the paper do not necessarily represent the views of the US Department of Energy or the United States Government.
© The Electrochemical Society. DOI:10.1149/2.F10243IF
About the Authors
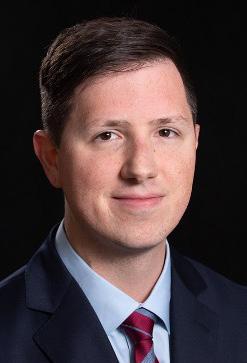
Alex Bates, Sr Member of the Technical Staff, Sandia National Laboratories
Education: BS, MEng, and PhD in Mechanical Engineering (University of Louisville)
Work Experience: Alex has been working in the fields of electrochemistry and energy storage for the past 15 years on technologies that include fuel cells, flow batteries, Li and Naion batteries, and solid-state batteries. In 2020, joined Sandia National Labs as a Postdoctoral Appointee and in 2022, transitioned to Senior Member of Technical Staff. His current work involves grid and vehicle energy storage safety and reliability with a focus on conventional, advanced, and beyond Li-ion chemistries from materials and cells to packs and modules.
Research Interests: Advanced and beyond Li-ion safety in grid and transportation applications; early detection and intervention methods to cell failure; early-stage evaluation of battery materials safety characteristics
Pubs + Patents: >25 publications, >10 invited presentations (domestic and international), 2 patents
Awards: Hsing Chuang Award for Excellence in Graduate Study, Mechanical Engineering Department Academic Achievement Awards
Work with ECS: Member for 3 years, Member of Battery Division, Lead Symposium Organizer for ECS247 in Montreal, Several chair positions at ECS conferences
of catastrophic thermal runaway. Leading the Battery Abuse Testing Laboratory, Loraine and her team concentrate on deciphering the mechanisms behind safety incidents and crafting mitigation strategies for both single-cell and system failure.
Research Interests: Battery safety science of Li-ion and beyond Li-ion technologies; early detection of failure and developing intervention method; developing new safety testing protocols
Pubs + Patents: >15 publications on battery safety, >20 invited presentations (domestic and international) on battery safety Awards: Women in Energy – DOE Energy Storage Grand Challenge (2023)
Work with ECS: Member for 12 years, Member of the Battery Division
Website: https://energy.sandia.gov/programs/energy-storage/staff/ loraine-torres-castro/ https://energy.sandia.gov/programs/energy-storage/safety-andreliability/ https://orcid.org/0000-0002-9267-8489


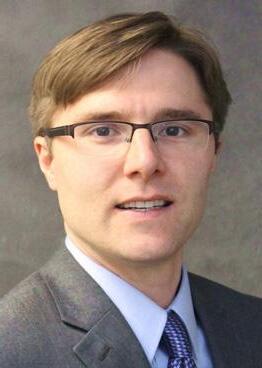
Paul Albertus, Associate Professor of Chemical and Biomolecular Engineering and Associate Director, Maryland Energy Innovation Institute, University of Maryland, College Park
Education: BSE in Chemical Engineering (Univ of Michigan), PhD in Chemical Engineering (Univ of California, Berkeley, under the direction of John Newman)
Work Experience: Following his PhD, Paul continued work on electrochemical systems as a Senior Research Engineer at the Bosch Research and Technology Center (2009–2013), and then transitioned to roles as a Fellow and Program Director at DOE ARPA-E (2014–2018). While at ARPA-E, Paul led the initiation of the IONICS (solid-state separators) and DAYS (long-duration electricity storage) programs, and oversaw a portfolio of >50 projects in numerous areas of electrochemical technologies. Research Interests: Electrochemical systems, with a focus on battery science and technology. Specific areas of current interest include early-stage evaluation of battery safety using calorimetry and other methods, electrochemical-mechanical coupling in solidstate electrochemical systems, and continuum-scale modeling of high-rate and high-energy primary and secondary batteries. Pubs + Patents: >30 peer-reviewed publications, >20 invited presentations, >25 issued patents, and has >6,000 citations of his work.
Awards: Norman Hackerman Electrochemical Society Young Author Award.
Work with ECS: Member since 2009, Session chair at numerous meetings Website: https://albertus.umd.edu https://orcid.org/0000-0003-0072-0529


Partha P. Mukherjee, Professor and University Faculty Scholar, Purdue University


Website: https://energy.sandia.gov/programs/energy-storage/staff/ alex-bates/ https://www.linkedin.com/in/alex-bates/
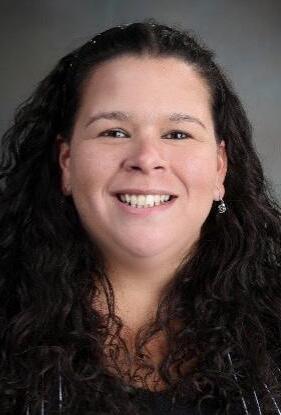
Loraine Torres-Castro, Battery Safety
Lead, Sandia National Laboratories
Education: BS in Physics and PhD in Chemical Physics (University of Puerto Rico-Rio Piedras) Work Experience: Loraine serves as the battery safety lead at Sandia National Labs, specializing in vehicle electrification and grid energy storage. Her work involves rigorous evaluation of batteries beyond manufacturer specifications, particularly assessing the risks
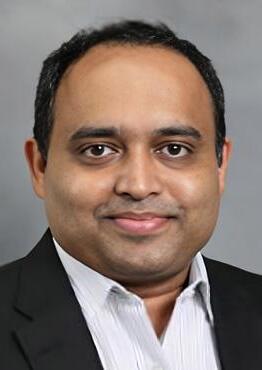
Education: PhD in Mechanical Engineering (Pennsylvania State University)
Work Experience: Prior appointments include Assistant Professor and Morris E. Foster Faculty Fellow of Mechanical Engineering at Texas A&M University (2012–2017), Staff Scientist at Oak Ridge National Laboratory (2009–2011), Director’s Research Fellow at Los Alamos National Laboratory (2008–2009), and Engineer at Fluent India (currently Ansys Inc., 1999–2003).
Research Interests: Mesoscale physics and stochastics of transport, electrochemistry, and materials interactions in energy storage and conversion.
(continued on next page)
(continued from previous page)
Pubs + Patents: >200 journal publications in energy storage and conversion, h-index: 60; invited presentations: >100 including at the US National Academy of Engineering Frontiers of Engineering symposium and Gordon Research Conference – Batteries
Awards: Scialog Fellows’ recognition for advanced energy storage; University Faculty Scholar and Faculty Excellence for Early Career Research awards from Purdue University; The Minerals, Metals & Materials Society Young Leaders Award
Work with ECS: Member since 2008 (before that a student member); Guest Co-Editor for three JES special; Symposium coorganizer; Founding Faculty Adviser for Purdue University ECS Student Chapter
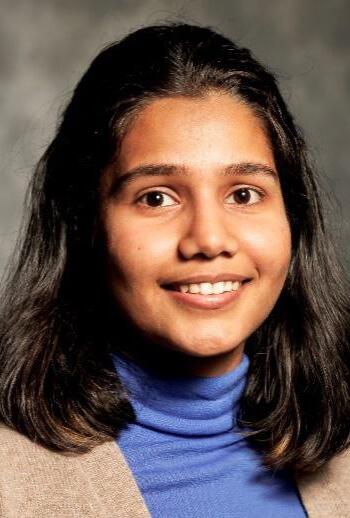
Bhuvsmita Bhargava, Graduate Student
Researcher, Department of Chemical and Biomolecular Engineering, University of Maryland, College Park
Education: BE in Chemical Engineering (BITS Pilani), MTech in Chemical Engineering (IIT Madras)
Research Interests: Battery safety analysis through calorimetry, electrochemicalmechanical coupling at interfaces in solid state batteries
Awards: Wells Energy Fellowship (2020)


Website: https://engineering.purdue.edu/ETSL/ https://orcid.org/0000-0001-7900-7261
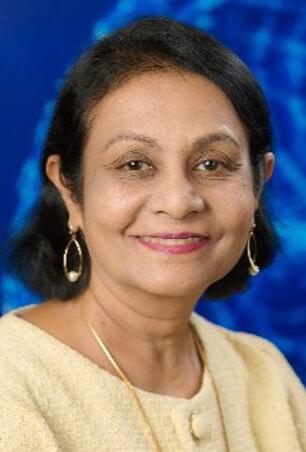
Judith Jeevarajan, Vice President and Executive Director of Electrochemical Safety Research Institute at UL Research Institutes
Education: PhD in Electrochemistry (University of Alabama, Tuscaloosa)
Research Interests: Energy storage performance and safety, battery safety, lithiumion safety
Brief Work Experience: Vice President and Executive Director of Electrochemical Safety Research Institute at UL Research Institutes with a focus on safety of energy storage systems, including batteries and novel energy storage chemistries.
Pubs + Patents: Several publications and presentations; three book chapters
Awards: Woman of Color – Leaders and Legends Award (2023); Woman Leader of the Year in Energy Storage (2020); AIAA Power Systems Award (2019); NASA NESC Engineering Excellence Award (2013)
Work with ECS: Member for 15 years
Website: https://ul.org/research/electrochemical-safety https://orcid.org/0000-0003-4843-7597


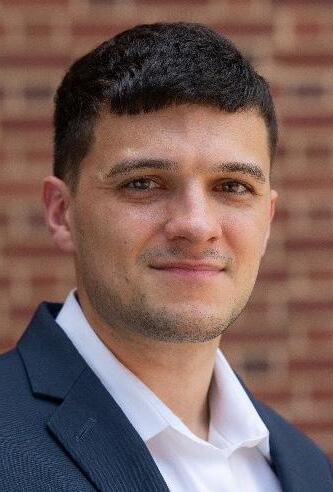
Nathan Johnson, Postdoctoral Appointee, Sandia National Laboratories
Education: PhD in Chemical Engineering (University of Maryland, College Park, under the direction of Paul Albertus)
Work Experience: Nathan’s doctoral work focused on assessing the safety of nextgeneration lithium metal solid-state batteries through a combination of benchtop experiments and finite element analysis modeling. Research Interests: Testing the safety and reliability of primary and secondary batteries for identifying failure mechanisms and hazards. Current research focuses are on establishing early-stage safety testing methods for next-generation battery materials.
Work with ECS: Nathan was the Vice-Chair of the University of Maryland ECS Student Chapter. He has presented numerous times at ECS conferences, and will be a session Co-Chair at the upcoming 2024 ECS PRiME.


Website: https://energy.sandia.gov/programs/energy-storage/safetyand-reliability/ https://orcid.org/0000-0002-1360-110X
Work with ECS: ECS member, Vice-chair of the ECS Student Chapter at the University of Maryland https://orcid.org/0009-0007-1268-6635


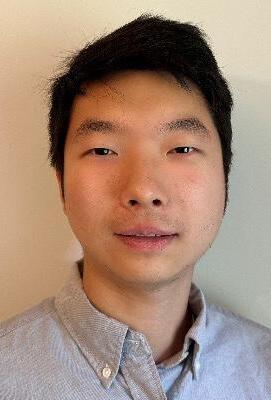
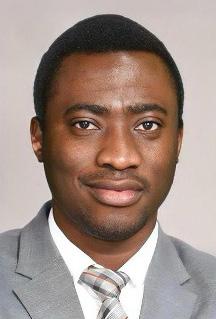
Zixuan Wang, Graduate Student Researcher, University of Maryland, College Park
Education: BE in Materials Science and Engineering (Zhejiang University)
Research Interest: Safety of solid-state battery systems https://orcid.org/0009-0004-8178-114X


Taiwo Ogundipe, Graduate Student Researcher, University of Maryland, College Park
Education: BSc in Chemical Engineering (Obafemi Awolowo University), MSc in Chemical Engineering (University of Chinese Academy of Sciences)
Work Experience: Research Assistant under Prof. Changfeng Yan at the Hydrogen Production and Utilization Lab at the Guangzhou Institute of Energy Conversion, Chinese Academy of Sciences. Worked on non-noble metal electrocatalysts and electrochemical cell designs for water splitting and fuel cells. Research Interests: Electrochemical systems, with a focus on battery safety. Specific area of current interest includes the evaluation of solid-state battery safety using calorimetry.
Pubs + Patents: >5 peer-reviewed publications, >2 invited presentations, and has >175 citations of his work.
Work with ECS: Member ECS student chapter at the University of Maryland https://orcid.org/0000-0002-9167-6254


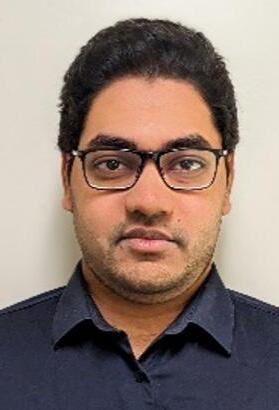
Bairav S. Vishnugopi, Research Scientist, Purdue University
Education: BE in Mechanical Engineering (Birla Institute of Technology and Science, Pilani), PhD in Mechanical Engineering (Purdue University)
Work Experience: Bairav has developed physics-based modeling and analytics frameworks to interrogate and predict the performance, degradation, and safety of lithiumion and next-generation batteries.
Research Interests: Energy storage, Next-generation batteries, Liion batteries, Thermal safety. Bairav’s research at Purdue University focuses on understanding the mechanistic origin and evolution of reactive-transport, chemo-mechanical, and thermo-electrochemical interactions in porous architectures and interfaces for energy storage and conversion.
Pubs + Patents: > 40 journal publications in different energy storage chemistries, including Li-ion batteries, alkali-metal batteries, and solid-state batteries for applications such as fast charging and electric aviation.
References
1. M. H. J. Fleischmann, E. Horetsky, D. Ibrahim, et al., Battery 2030: Resilient, Sustainable, and Circular, McKinsey & Company (2023).
2. J. Lamb, L. Torres-Castro, J. C. Hewson, R. C. Shurtz, and Y. Preger, J Electrochem Soc, 168, 060516 (2021).

Awards: IE&EE Division H. H. Dow Memorial Student Achievement Award, Outstanding Graduate Student Research Award and R. H. Kohr Graduate Student Fellowship (Purdue University) https://orcid.org/0009-0002-6357-9358

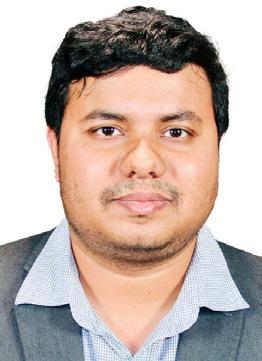
Avijit Karmakar, Postdoctoral Research Associate, Purdue University
Education: BE (Bengal Engineering and Science University), MTech (Indian Institute of Technology, Kanpur) and PhD in Mechanical Engineering (Illinois Institute of Technology)
Research Interests: Energy storage safety, Thermal management, Thermal system design, Multiphase flows, Bluff body hydrodynamics Awards: Trailblazers in Engineering Fellow and “Recognize, Reward and Retain top talent” (Purdue University)
3. A. M. Bates, Y. Preger, L. Torres-Castro, K. L. Harrison, S. J. Harris, and J. Hewson, Joule, 6, 742 (2022).
4. Volta Foundation, The Battery Report 2023 (2023).
5. Sandia National Laboratories, Sodium-ion battery safety research: Advancing the next generation of energy storage technology (2024).
6. D. R. Kannan, C. Lopez, J. Jeevarajan, NAATBatt Na/Zn Workshop, Houston, TX (2023).
7. D. R. Kannan, C. Lopez, V. Premnath, J. Jeevarajan, 2024 Energy Storage Safety and Reliability Forum, Richland, WA (2024).
8. G. W. Rachel Carter, C. Jacob, D. Hayman, Corey Love, Energy Storage Safety and Reliability Forum, Richland, WA (2024).


Website: https://sites.google.com/view/avijitkarmakar/home https://orcid.org/0000-0001-7904-1179
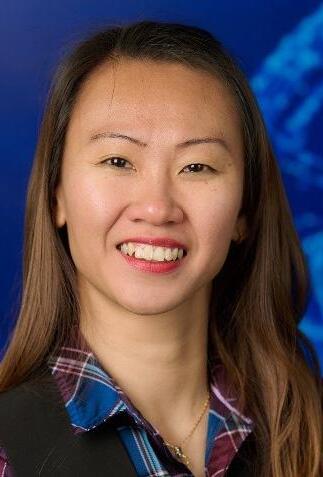
Wan Si Tang, Director of Research, Electrochemical Safety Research Institute, UL Research Institutes
Education: BS in Applied Chemistry (National University of Singapore), PhD and MS in Materials Science (University of Picardy Jules Verne), postdoctoral experiences at the Carnegie Institution for Science and the National Institute of Standards and Technology.
Work Experience: Wan Si has 15+ years of research, discovery, design, and development experience. Prior to her role at the ESRI, she was the Advanced Battery Manufacturing Team Leader and Materials Chemist at the Battery Innovation Center with proficiency in industry-standard advanced battery manufacturing processes.
Research Interests: Novel materials and new energy forms pertaining to practical and safer energy storage and conversion. Pubs + Patents: 30 publications, 1900+ citations, 40+ conferences, h-index=21
9. M. V. Reddy, A. Mauger, C. M. Julien, A. Paolella, and K. Zaghib, Materials, 13, 1884 (2020)
10. E. Jarratt, New lessons from the epic story of Moli Energy, the Canadian pioneer of rechargeable lithium battery technology, Electric Autonomy Canada (2020).
11. I. U. Mohsin, C. Ziebert, M. Rohde, and H. J. Seifert, J Electrochem Soc, 168, 050544 (2021)
12. H. Zhang, X. Wu, Z. Li, et al., Adv Energy Mater, 14, 2400397 (2024).
13. T. Inoue and K. Mukai, ACS Appl Mater Interfaces, 9, 1507 (2017)
14. N. B. Johnson, B. Bhargava, J. Chang, S. Zaman, W. Schubert, and P. Albertus, ACS Appl Mater Interfaces, 15, 57134 (2023).
15. A. M. Bates, P. Albertus, J. Jeevarajan, P. P. Mukherjee and L. Torres-Castro, ECS Meeting Abstracts, MA2023-02, 457 (2023).
16. B. S. Vishnugopi, M. T. Hasan, H. Zhou, and P. P. Mukherjee, ACS Energy Lett, 8, 398 (2023)
17. A. Karmakar, H. Zhou, B. S. Vishnugopi, J. A. Jeevarajan, and P. P. Mukherjee, J Electrochem Soc, 171, 010529 (2024)
18. H. Zhou, M. Parmananda, K. R. Crompton, M. P. Hladky, M. A. Dann, J. K. Ostanek, and P. P. Mukherjee, Energy Storage Mater, 44, 326 (2022)


Website: https://wansitang.wordpress.com/ https://orcid.org/0000-0002-7893-3025
19. N. Johnson and P. Albertus, J Electrochem Soc, 169, 060546 (2022)
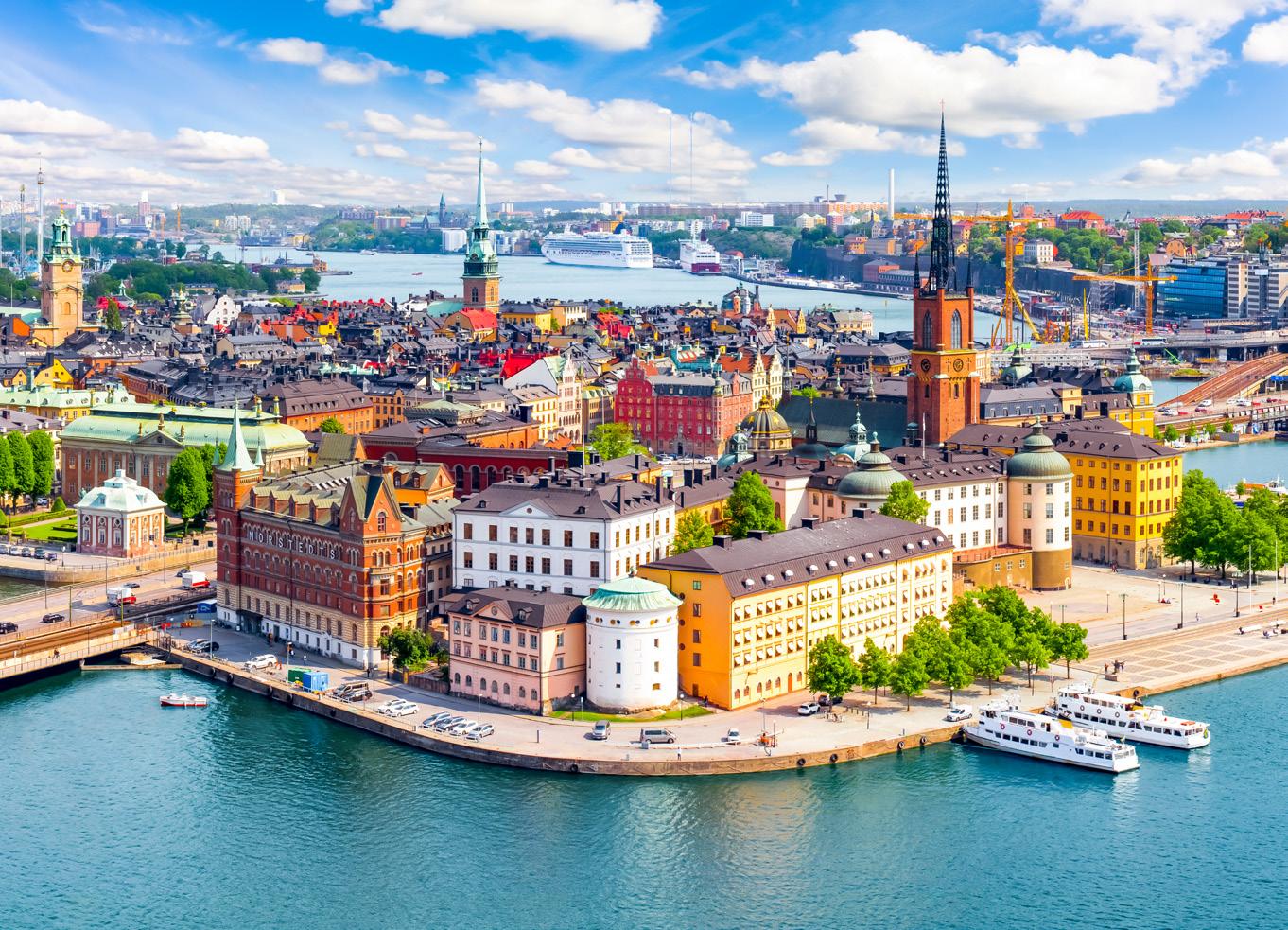
19th International Symposium on Solid Oxide Fuel Cells (SOFC-XIX)
Stockholm, Sweden
July 13-18, 2025
The Brewery Conference Center
www.electrochem.org/upcoming-meetings
The Electrochemical Society book series provides authoritative, detailed accounts on specific topics in electrochemistry and solid state science and technology. These titles are sponsored by ECS and published in cooperation with Wiley.
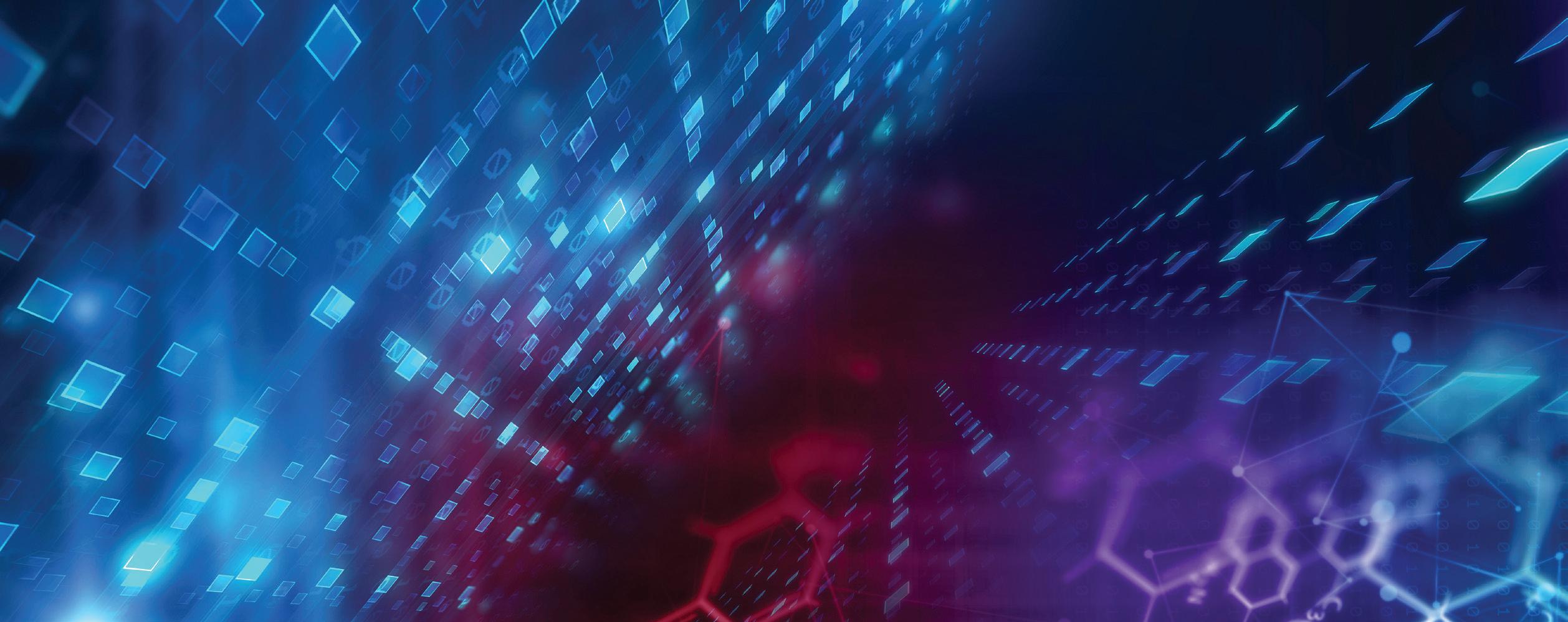
ECS members receive 20% off all Wiley books. ECS members use promo code ECS18 at checkout.
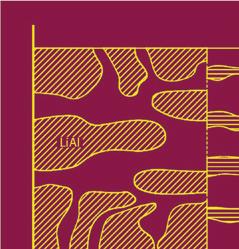
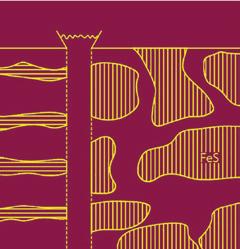

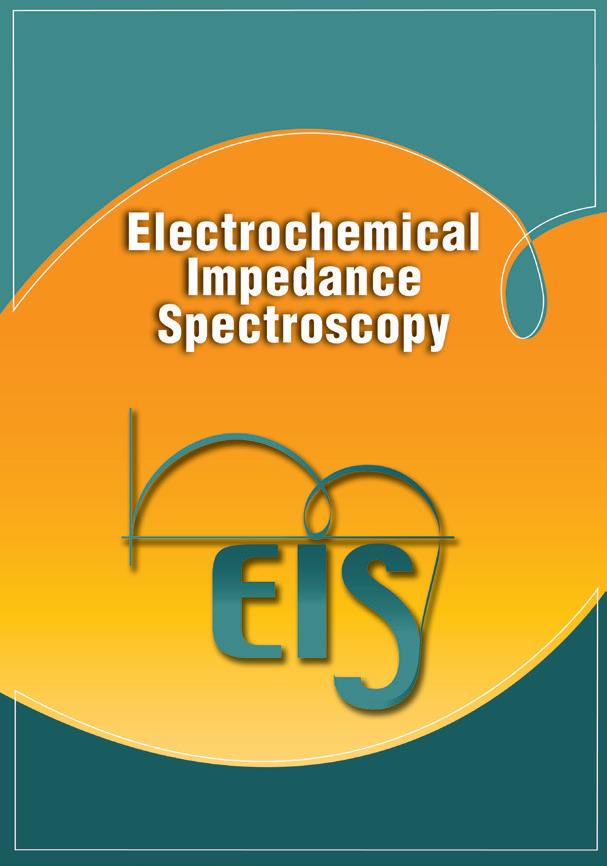
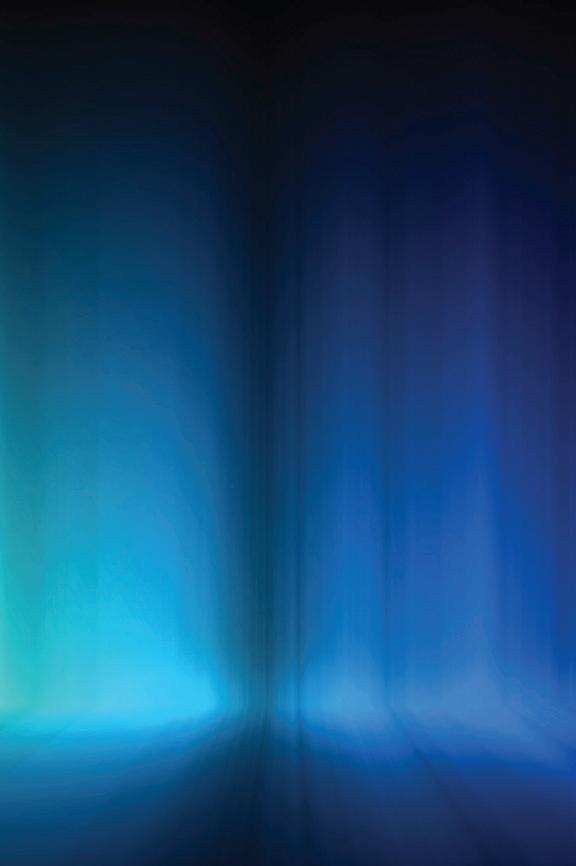
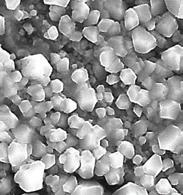

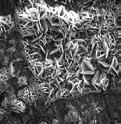

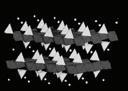

New
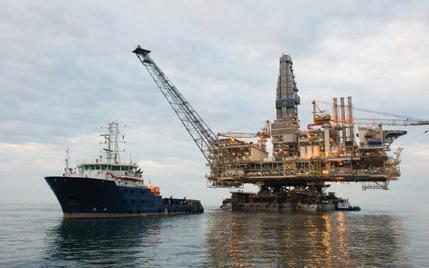
LaQue’s Handbook on Marine Corrosion, 2nd Edition
David A. Shifler
ISBN 978-1-119-78883-6 | July 2022
A practical, single-source reference on the unique nature of seawater as a corrosive environment. Explains practical corrosion control solutions via design, proper materials selection, and implementation of good corrosion control engineering practices.

INTERESTED IN PUBLISHING WITH US?
Please contact publications@electrochem.org to discuss your idea.
Christofer Leygraf Inger Odnevall Wallinder Johan Tidblad Thomas Graedel
High-Speed Imaging for Investigating Battery Failure Mechanisms
by Hamish T. Reid, Inez Kesuma, Mark Buckwell, James B. Robinson, Paul R. Shearing
As the EV and battery market continues to grow, understanding and mitigating battery thermal runaway remains a critically important area of research. In 2023, there were 270 reported battery fires in the UK, up 46% from the previous year.1 While electric transportation is generally very safe, and an EV is less likely to set alight than a traditional internal combustion car, clearly there is still room for improvement, particularly given the ubiquity of the device and as batteries are increasingly being explored in other, more demanding, sectors including aerospace applications.2,3
Under controlled experimental conditions, researchers have employed a range of different techniques to initiate battery fires and understand both how and why they occur. These can be broadly categorized under thermal, electrical, and mechanical methods.4 Mechanical abuse tests include nail penetration, crush, and vibrational testing, where an internal short-circuit is initiated from external damage. These techniques mimic what happens when, for example, an electric car undergoes a traffic collision. Electrical abuse includes overcharge and over discharge, where batteries are taken well outside their safe voltage operating limits. An example scenario of an overcharge condition is if the charge controllers, or the battery management system, on a device fail and supply current above 100% state-of-charge (SOC). Thermal abuse methods include heating a cell to thermal runaway or lowering the cell temperature sufficiently to initiate lithium dendrite formation and possibly cell internal shorting. Heating can initiate reactions between the chemicals within a battery and cause a domino effect of exothermic reactions leading to a thermal runaway condition. All these tests give important information about how a battery cell catastrophically fails under abuse conditions. However, recognizing the changes in the internal structure of the cell is vital to understanding the mechanisms of thermal runaway which enables the development of effective mitigation strategies. During the last decade, X-ray imaging has emerged as an important diagnostic tool for battery degradation, electrochemical performance, and safety diagnostics.5 Researchers use X-ray imaging to directly observe the internal changes in the cell during all stages of thermal runaway. Depending on the initiation method, it can sometimes take minutes, hours, or days for a cell to undergo failure.6–8 However, the actual final reactions that cause thermal runaway occur over fractions of a second. High-speed cameras, operating at up to 40,000 frames per second, can capture the interactions between electrodes at the final moments of thermal runaway. In this article, the basics of highspeed X-ray imaging and how this technique has been applied to investigating battery thermal runaway are discussed.
Introduction to X-ray Imaging
X-rays are a form of electromagnetic radiation with wavelengths in the range of 0.01 to 10 nanometres, placing them between ultraviolet light and gamma rays on the electromagnetic spectrum. The wavelengths of X-rays allow them to penetrate different materials to different extents, making them ideal for imaging applications. The ability of X-rays to pass through some materials while being absorbed by others is fundamental to X-ray imaging. Whether a material is opaque or transparent to X-rays is dependent on the material’s elemental composition, crystallographic configuration, and density.9,10
X-rays can be generated by several methods. In lab-based computed tomography (CT) sources, an X-ray tube source contains a cathode filament and a target anode, typically made using heavy metals such as W, Cu, or Mo. A voltage of approximately 60–300 keV is applied across the cathode and anode, and the potential difference causes electrons to travel from the filament toward the anode target. As the electrons approach the anode nuclei, they rapidly decelerate and produce Bremsstrahlung radiation to maintain conservation of energy. Increasing the voltage applied across the cathode and anode also increases the energy of the X-ray emissions. Furthermore, the collisions between the filament electrons and lower-orbital anode electrons also produce characteristic X-ray radiation. This produces a broad emission spectrum from the Bremsstrahlung radiation, with several sharp peaks from the characteristic photons.11
Synchrotron radiation sources generate X-rays by decelerating electrons in a particle accelerator ring. To conserve momentum, the rapid deceleration produces a “white beam” of radiation, with emissions across a broad range of wavelengths. Optics and filters are used to refine the beam to the required wavelength for the application. Synchrotrons have the key advantages of higher brilliance and coherence compared to a lab-based apparatus. Since their becoming a popular technique in the 1980s, non-destructive synchrotron X-ray imaging has developed for applications in biology, geology, material engineering, and beyond. In the context of high-speed imaging, the superior photon flux is useful for high frame-rate cameras. Lab-based sources often cannot achieve the minimum number of transmitted photons required for detectors for effective imaging and useful image resolutions, analogous to taking a photograph in a dark room without flash. Synchrotron beamlines also simply have more space to build around the beam path. Lab-based CT machines are enclosed in lead shielding to allow them to be placed wherever is convenient in lab, where space is often at a premium. The actual space to install an apparatus for safety testing around the sample is often >1 m3. At synchrotron facilities, researchers have the whole hutch (which is generally an order of magnitude larger) at their disposable to install machinery and specially designed enclosures to ensure the safety of the tests. When studying battery safety, there needs to be sufficient space for voltage and temperature measurements, hazardous material extraction, shielding from the force of the explosion, controllers for nail penetration/heaters/overcharging equipment, and other devices to monitor and perform experiments. These test requirements can be challenging to achieve in lab-based CT equipment.
In the context of batteries, X-ray imaging is a commonly used as a quality control technique by manufacturers and is applied at different scales. Electrode-level features, such as porosity and geometry, and cell-level features, such as number of windings or electrode misalignment, are screened as part of the production process.12 When investigating catastrophic cell failure, X-ray imaging is also useful for evaluating of safety mechanisms built into various cells in-situ. For example, most cylindrical cells (such as an 18650, 21700, or the more recent 4680 cell types) will typically be fitted with safety devices such as a positive temperature coefficient (PTC), current interruption device (CID), and/or a vent to release gases in the case of pressure build up in the cell. By directly imaging these safety devices during a failure event, researchers can better evaluate their operational characteristics which can be fed back into the product development cycle to build safer battery cell designs.13
(continued on next page)
(continued from previous page)
Challenges
While X-ray characterization for batteries and energy storage materials has matured in the last decade, there remain several persistent challenges in conducting battery failure experiments. A major hurdle is safety. Battery thermal runaway releases several hazardous chemicals such as HF, NiO, and carcinogenic particulates. Both the explosion caused by battery thermal runaway and the material that is released must be contained to protect people, equipment, and facilities. At the same time, any apparatus must have some degree of X-ray transparency to be suitable for imaging. This is typically achieved by using steel, which is highly attenuating to X-ray, as the main construction material, and aluminium, which is far less attenuating, as a window. However, aluminium has a melting point of 660°C, well below the surface temperatures achieved by some cells undergoing thermal runaway.14 The X-ray window must be properly maintained to prevent accidental release of hazardous materials or poor imaging conditions. To allow for sufficient airflow to remove gases and particulates, any failure apparatus must either be connected to a filtration system with some method of air ingress, or be treated as a pressure system, where the pressure can be released in a controlled environment. The latter can make use of solenoid valves, where the system is sealed during thermal runaway to prevent release of material, before the valves are opened for airflow in and extraction. Alternatively, filters are attached to the air intake, allowing the extraction system to run continuously.
Case Studies
Fractional Thermal Runaway Calorimetry
The fractional thermal runaway calorimeter (FTRC) was developed by NASA to quantitatively determine the distribution of energy into the surrounding area during battery thermal runaway.15 Rechargeable batteries are principally utilized in space applications to provide power to all spacecraft loads. In typical Li-ion batteries, oxygen can be liberated from the positive electrode during failure, making them potentially flammable even in a low-oxygen environment.16 The FTRC was introduced as a method for determining how best to
engineer spacecraft batteries to mitigate the effect of a battery thermal runaway safety hazard. The FTRC is designed to accommodate both heat and mechanical abuse tests. Aluminium is chosen to build the walls of the cell holder due to its low X-ray attenuation. This allows high-speed radiography to be performed in conjunction with the FTRC to analyze a range of cylindrical and pouch cells. The FTRC has also been vital in evaluating thermal runaway prevention devices.17,18
The data from the tests conducted in the FTRC characterizes the variation in thermal and mass ejection behaviors of cells. This is particularly important for battery designs that are required to safely vent ejecta emanating from a vented battery cell. Recently, Finegan et al. published the Battery Failure Databank, which contains the FTRC data of different cell variations.19 The databank includes more than 300 FTRC tests of Li-ion cells, many of them accompanying highspeed radiography at 2000–3000 fps. The FTRC plays a vital role in recognizing the difference in heat release of the ejected vs. the non-ejected mass remaining in the cell casing.20 Due to the FTRC design, researchers can separate the distinct heat output generated of each section. The three sources of mass are ejecta from the positive terminal, the negative terminal, and the non-ejected mass that remains in the cell. As visualized in Fig. 1, this level of detail provides insight into the relationship between the distinct heat release and the ejected mass of each section by fitting them as a linear equation against their masses.
The FTRC was developed based on a commonly used safety characterization technique: accelerating rate calorimetry (ARC). ARC is used to determine the heat required to initiate chemical reactions between battery cell components. In ARC, the cell is placed in an adiabatic chamber, and heated in sequential steps before selfheating reactions are detected. Once the cell begins self-heating, the calorimeter matches the chamber and cell temperatures to maintain an adiabatic environment. This temperature tracking process continues until the cell undergoes thermal runaway, or until the reactions stop, after which the calorimeter will return to applying heat. ARC is vital in determining the safe operating temperatures and storage conditions of battery cells.21 While the FTRC is effective at determining the heat output during thermal runaway, ARC is a complementary method that can determine the heat input required to initiate an explosive failure event. Together, the two techniques can paint a complete picture of how a cell goes from a safe state into thermal runaway.
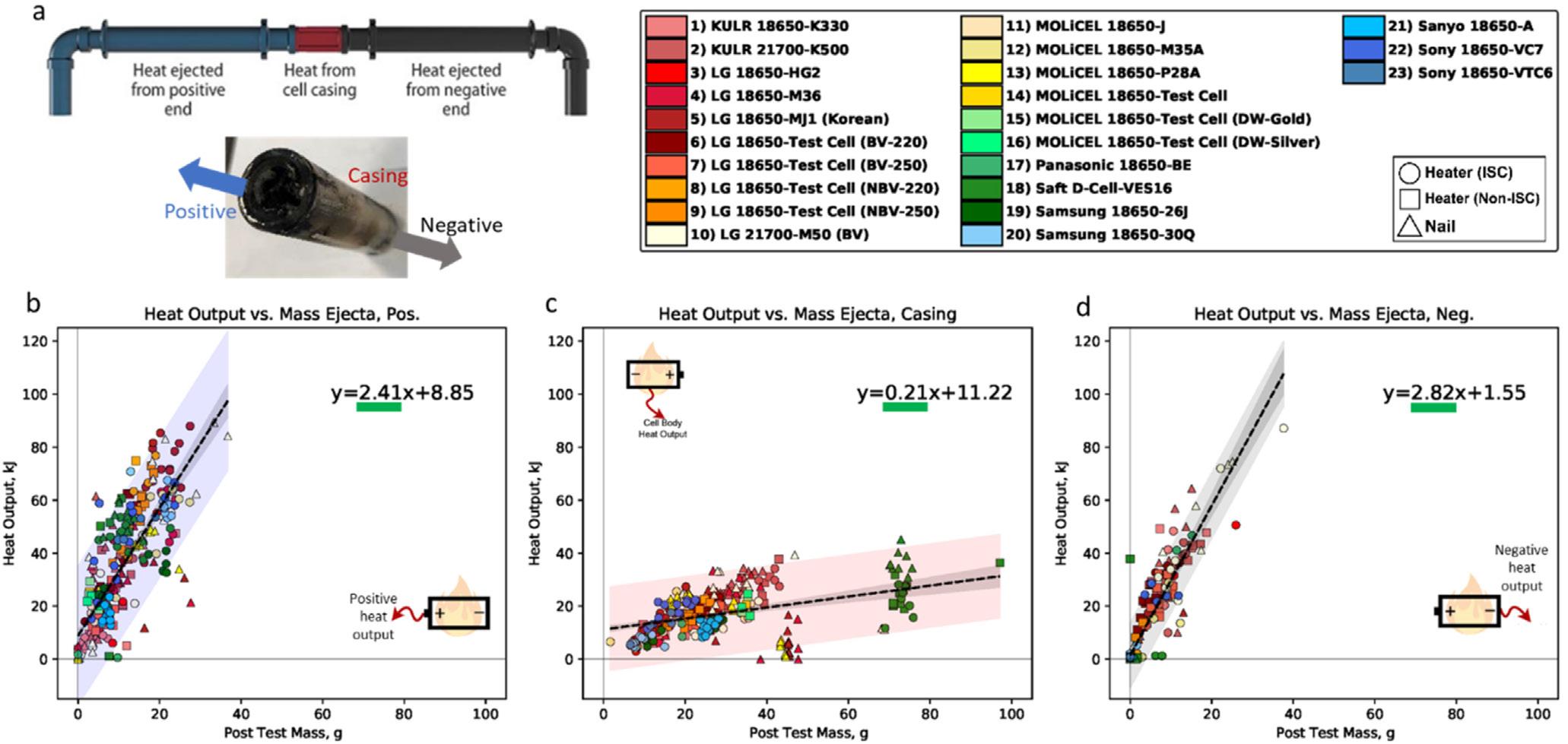
Reproduced with permission from Finegan et al.19
Fig. 1. (a) A visualization of the three sections. (b) Fractional heat output as a function of positive ejected mass, (c) non-ejected mass, (d) negative ejected mass. Due to a more oxygen rich environment, ejected mass released around > 10 times more heat (2.6 kJ/g) compared to non-ejected mass (0.21 kJ/g).
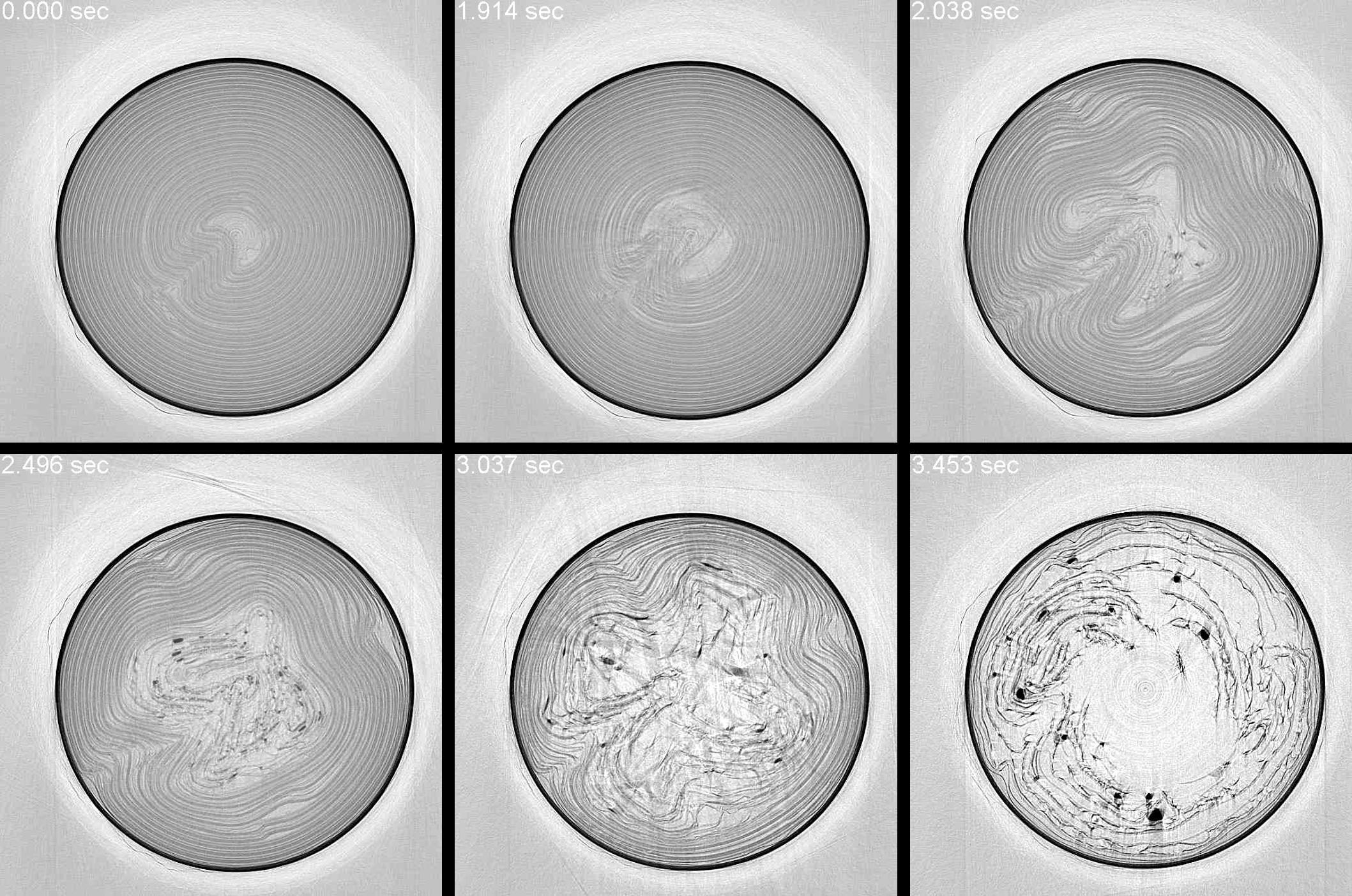
Fraunhofer Ernst Mach Institute (EMI) Chamber
While the FTRC is adept at quantifying the energy release of small cylindrical and pouch cells during thermal runaway, it’s limited to radiographic imaging of specific cell sizes. To study larger cells or packs and employ high-speed tomography, researchers at the ESRF, Fraunhofer EMI, and University College London have developed a chamber compatible with the ID19 beamline.22 Like the FTRC, the EMI chamber is fitted with a remote-controlled nail penetration device that can be used in conjunction with both radiography and tomography. Unlike the FTRC, the chamber typically uses a heat gun for overheating testing, whereas the FTRC employs heat cartridges for accurate recording of energy input.23 Heating cartridges are not suitable for use with tomography without a high-speed slip ring. The large volume of the chamber (dimensions 0.7 x 0.7 x 0.7 m) allows for greater flexibility in abuse testing of different cell or module designs, as well as a fire suppression system using inert gases to prevent damage to the internal components. To date, a range of samples have been investigated, from 200 mAh pouch cells to 22 Ah Tesla 4680 cells.
While the radiography capability of the battery-abuse chamber acts in a similar way to the FTRC, where the steel walls are fitted with an aluminium window, the abuse chamber also has a unique tomography capability. The brilliance and photon flux of the synchrotron beamline, coupled with high frame rate cameras, allows for the collection of tomographic projections as the cell undergoes thermal runaway. To successfully perform tomographic imaging, the cell needs to rotate quickly enough to achieve sufficient temporal resolution to effectively see the internal changes in the cell during thermal runaway. The chamber is fitted with a motor underneath it which is connected to the cell and rotates at 20 Hz. The motor and beamline instruments
are synchronized to ensure that the projections are evenly spaced. The opportunity to image batteries in four dimensions (i.e., x, y, z, and time) when they are failing provides unparalleled detail of the internal changes. For example, Fig. 2 shows an orthogonal slice of a cylindrical cell as it undergoes thermal runaway. The dark outer circle is the steel casing, and the spiral within contains the wound electrode layers. The images clearly show how the buckling at the center of the jelly roll acts as an initiation point for the collapse of the rest of the electrodes. This work highlights how the architectural damage that occurs from cell aging can affect safety behavior.
Summary
In conclusion, high-speed X-ray imaging has emerged as a useful investigative tool for researching the complex phenomenon of battery thermal runaway. Advances in synchrotron imaging have acted as a driver to enable researchers to better understand how batteries fail over different size and time scales. Evolution from early qualitative methods to an increasingly quantitative technique provides insight into the failure dynamics of cells.24 While catastrophic battery cell failure is rare, the consequences are severe. The techniques presented here are pushing the edge of our understanding to enable more energy dense module and pack design, opening new opportunities and applications. The progress made in investigating thermal runaway has already been vital in implementing several mitigation devices to produce safer and better performing batteries. With increased research effort, it will continue to play a key role in the transition to a greener future.
©The Electrochemical Society. DOI: 10.1149/2.F11243IF
(continued on next page)
Fig. 2. Selected orthoslices from high-speed X-ray CT imaging of a cycle aged LG M50 cell during thermal runaway.
(continued from previous page)
About the Authors
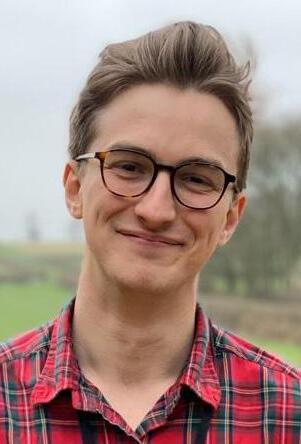
Hamish T. Reid, Post-doctoral Research
Associate, University College London (UCL)
Education: MChem (University of Sheffield)
Research Interests: Li-ion batteries, Aerospace electrification, Battery safety, X-ray characterization
Work Experience: Past positions include Polymer Scientist at Dow, Battery Scientist at Faradion Ltd., and PhD researcher and Research Fellow at UCL
Awards: STFC Experimental Design Award, RSC Outreach prize Pubs + Patents: 9 publications
Website: https://profiles.ucl.ac.uk/74779-hamish-reid
https://orcid.org/0000-0003-1970-8650
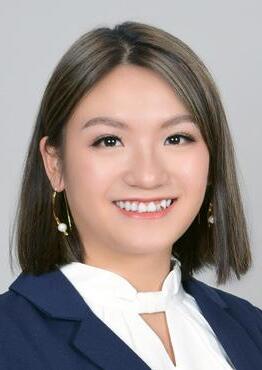
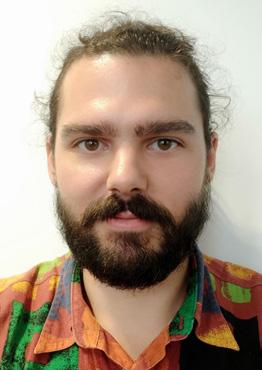
Inez Kesuma, PhD candidate, ZERO Institute, University of Oxford
Education: MSc in Chemistry (Leibniz University Hanover)
Research Interests: Li-ion batteries, Battery safety, Ultrasonic inspection, Radiography
Pubs + Patents: 2 publications https://orcid.org/0009-0005-4468-6168
Mark Buckwell, Advanced Propulsion Laboratory Manager, UCL
Education: PhD in Electrical and Electronic Engineering (UCL)
Research Interests: Batteries, fuel cells, motors, ventilation systems
Work Experience: Previous positions include Research Fellow roles on projects with the Faraday Institution, working on battery safety, and EPSRC, working on neuromorphic devices.
Awards: STFC Experimental Design Award, Worshipful Company of Scientific Instrument Makers Scholar Award
Pubs + Patents: >30 publications
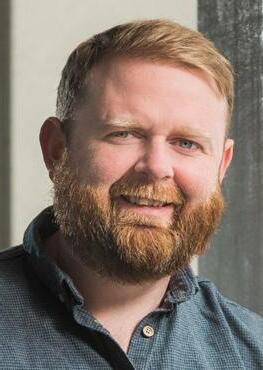
James B. Robinson, Lecturer in Advanced Propulsion, UCL
Education: PhD in Electrochemical Engineering (UCL)
Research Interests: Batteries, High-energy storage, Battery diagnostics and safety
Work Experience: He is the Project Lead on the Faraday Institution’s lithium sulfur focused LiSTAR project and has previously held EPSRC Doctoral Prize and RAEng UKIC research fellowships.
Work with ECS: ECS Member
Pubs + Patents: >60 papers
Website: https://profiles.ucl.ac.uk/36059-james-robinson
ORCID ID: https://orcid.org/0000-0002-6509-7769
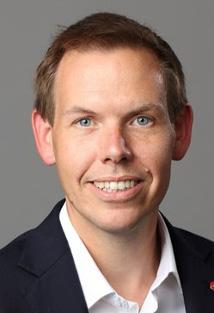
Paul R. Shearing, Professor of Sustainable Energy Engineering at the Department of Engineering Science and Director of the ZERO Institute at Oxford University
Education: Masters in Chemical Engineering (Birmingham University), PhD (Imperial College London, UCL)
Research Interests: Cover a broad range of electrochemical engineering themes with a particular interest in the characterization and understanding of materials for batteries, fuel cells, and other energy applications.
Work Experience: Chair in Sustainable Energy Engineering, University of Oxford (2023–), Professor in Chemical Engineering, UCL (2012–23), Royal Academy of Engineering Chair in Emerging Battery Technologies (2018–)
Pubs + Patents: >450 peer reviewed scientific papers, 1 patent Awards: Royal Academy of Engineering Chair in Emerging Battery Technologies. Founding investigator of The Faraday Institution, the UK’s independent institute for electrochemical energy storage research where he leads the LiSTAR and Safebatt research programs. 2022 Royal Academy of Engineering Silver Medal.
Work with ECS: Member since 2009
Websites: www.zero.ox.ac.uk


https://eng.ox.ac.uk/people/paul-shearing/ https://orcid.org/0000-0002-1387-9531
References
1. QBE, (2024).
2. A. Takou, Zev Alliance, 56 (2024).
3. S. Sripad, A. Bills, and V. Viswanathan, MRS Bull, 46, 435 (2021).
4. P. Christensen, W. Mrozik, and J. Weaving, Improving the Safety of Lithium-ion Battery Cells, (2023).
5. T. M. M. Heenan, C. Tan, J. Hack, D. J. L. Brett, and P. R. Shearing, Materials Today, 31, 69 (2019).
6. M. Buckwell et al., J Energy Storage, 65, 107069 (2023).
7. R. Carter, E. J. Klein, R. W. Atkinson, and C. T. Love, J Power Sources, 437, 226820 (2019).
8. X. Feng et al., Int J Electrochem Sci, 14, 44 (2019)
9. M. J. Cooper, Acta Crystallogr, 18, 813 (1965).
10. P. J. Withers et al., Nature Reviews Methods Primers, 1 (2021)
11. J. Hsieh, Computed Tomography: Principles, Design, Artifacts, and Recent Advances, SPIE PRESS, (2015).
12. S. Masuch, P. Gümbel, N. Kaden, and K. Dröder, Processes, 11, 1 (2023).
13. D. P. Finegan et al., Adv Sci, 5 (2018)
14. D. P. Finegan et al., Nat Commun, 6, 1 (2015).
15. E. Dimpault-Darcy et al., J Power Sources, 255 (2021).
16. Q. Sun et al., J Power Sources, 527 (2022)
17. M. T. M. Pham et al., Cell Rep Phys Sci, 2, 100360 (2021).
18. M. Sharp et al., J Electrochem Soc, 169, 020526 (2022).
19. D. P. Finegan et al., J Power Sources, 597 (2024)
20. W. Q. Walker et al., J Power Sources, 415, 207 (2019).
21. M. N. Richard and J. R. Dahn, J Electrochem Soc, 146, 2078 (1999).
22. J. Pfaff et al., J Synchrotron Radiat, 30, 192 (2023).
23. M. Fransson et al., J Energy Storage, 71, 108088 (2023).
24. A. N. P. Radhakrishnan et al., Energy Environ Sci, 15, 3503 (2022).
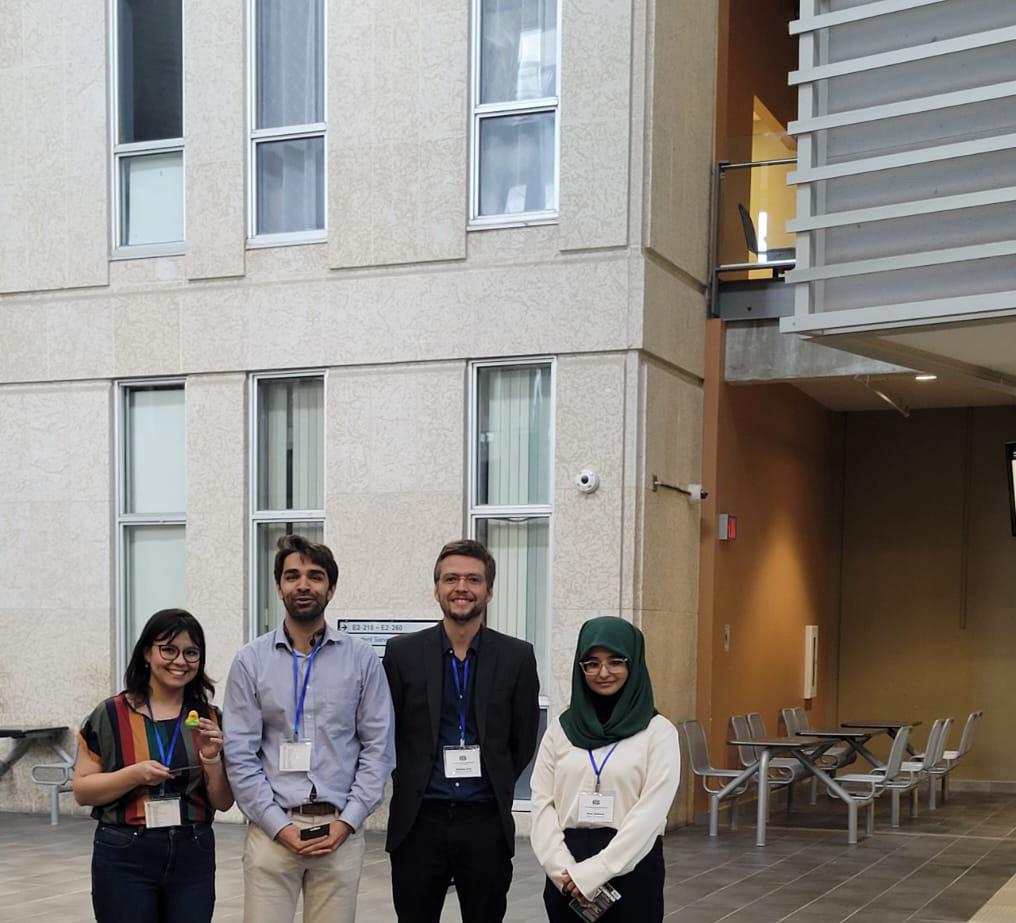
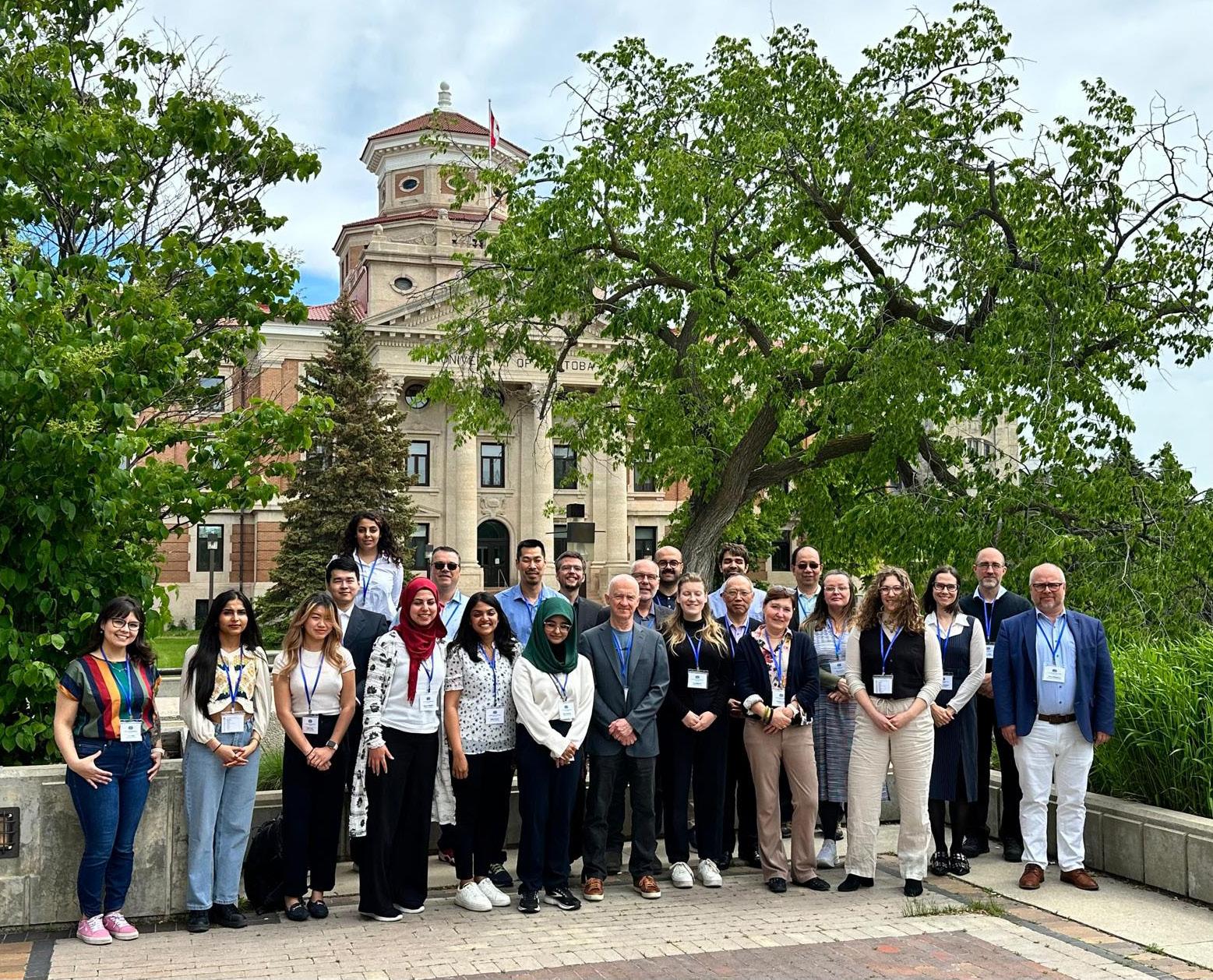
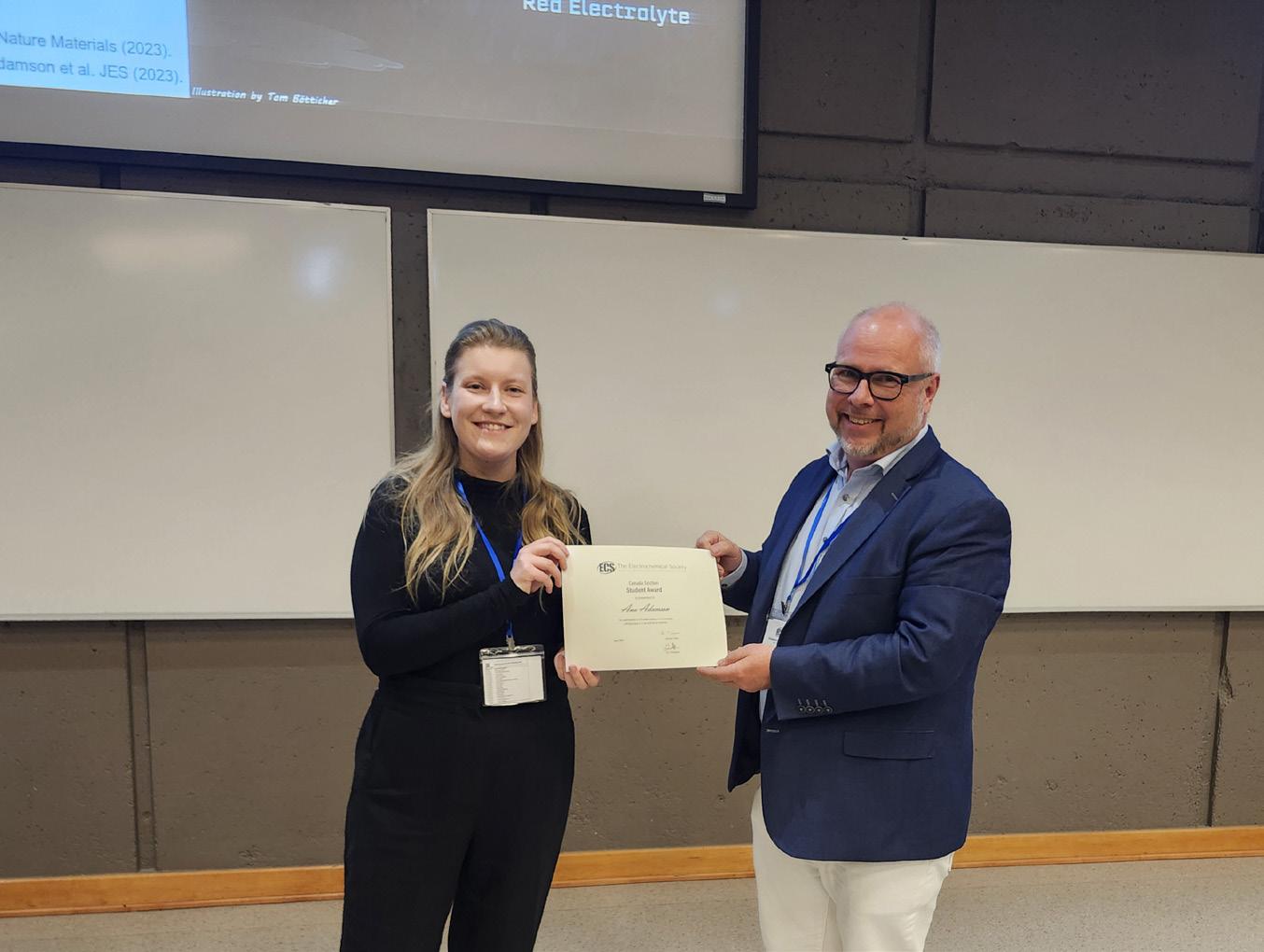
ECS Canada Section meeting participants at the University of Manitoba on June 7.
Photo: Sabine Kuss
Meeting organizer and host Christian Kuss (third from left) with poster presentation award winners (from left to right) Luma Lopes, Ameer Nizami, and Muna Abdulaziz
Photo: Mariam Odetallah
ECS Canada Section Student award winner, Anu Adamson, with ECS Canada Section Chair Steen Schougaard
Photo by Christian Kuss
ECS Israel Section
The national meeting of the Israel Electrochemical Society (ISEL) was held on July 8 at the Hebrew University in Jerusalem. The ECS Israel Section sponsored the meeting, and many section members participated. At the meeting, members suggested renewing the Israeli Student Chapter, which will be initiated soon.
The meeting was organized by Prof. Daniel Mandler and Dr. Daniel Sharon (both from the Institute of Chemistry at Hebrew University). An unprecedented number of 240 participants from all Israeli universities, colleges, industry, and research centers took part and enjoyed lectures given by the best electrochemists in Israel. Hebrew University President Prof. Asher Cohen opened the meeting, followed by greetings from Prof. Lital Alfonta who recently replaced Prof. Diana Golodnitzky as ISEL president. Prof. Doron Aurbach updated participants on the newly opened Israel National Institute for Energy Storage supported by the Ministry of Energy.
“Plus 1, 2, 3 & 4 Oxidation States in Anode Fuels…and More.” Two parallel sessions followed. Half of the lectures were invited
talks given primarily by the younger generation of recently recruited faculty. Students and postdocs presented the other half. Vendors who supported the meeting also gave 10-minute scientific talks. Students delivered an incredibly high number of posters—exactly 100— during the lunch break.
The meeting ended with a special and exciting award ceremony, where ten prizes for the best posters and four awards for the best student lectures were distributed. The awards were contributed by the families of three Israeli electrochemists who had passed away: Dr. Baruch Zinger (Soreq Nuclear Center) who was killed by terrorists in 2001, Prof. Judith Rishpon (Tel Aviv University), and Prof. Israel Rubinstein (Weizmann Institute of Science). The “crowd favorite” lecture prize was awarded to Mr. Pavel Savchenko for his talk on “The Effect of the Capping Agents of Nanoparticles on Their Redox Potential.” Last, but certainly not least, the meeting was dedicated to who mentored numerous Israeli professors and
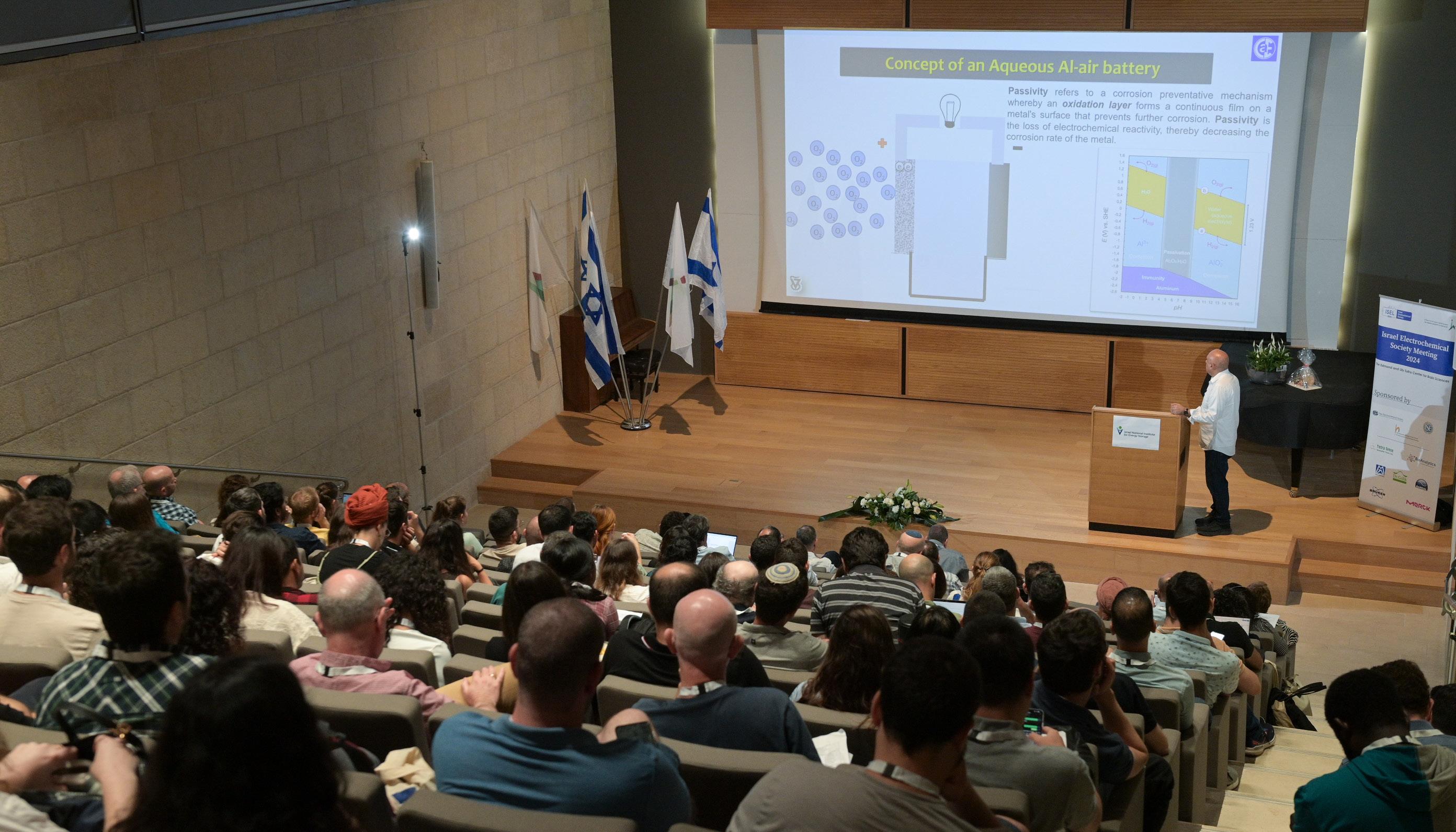
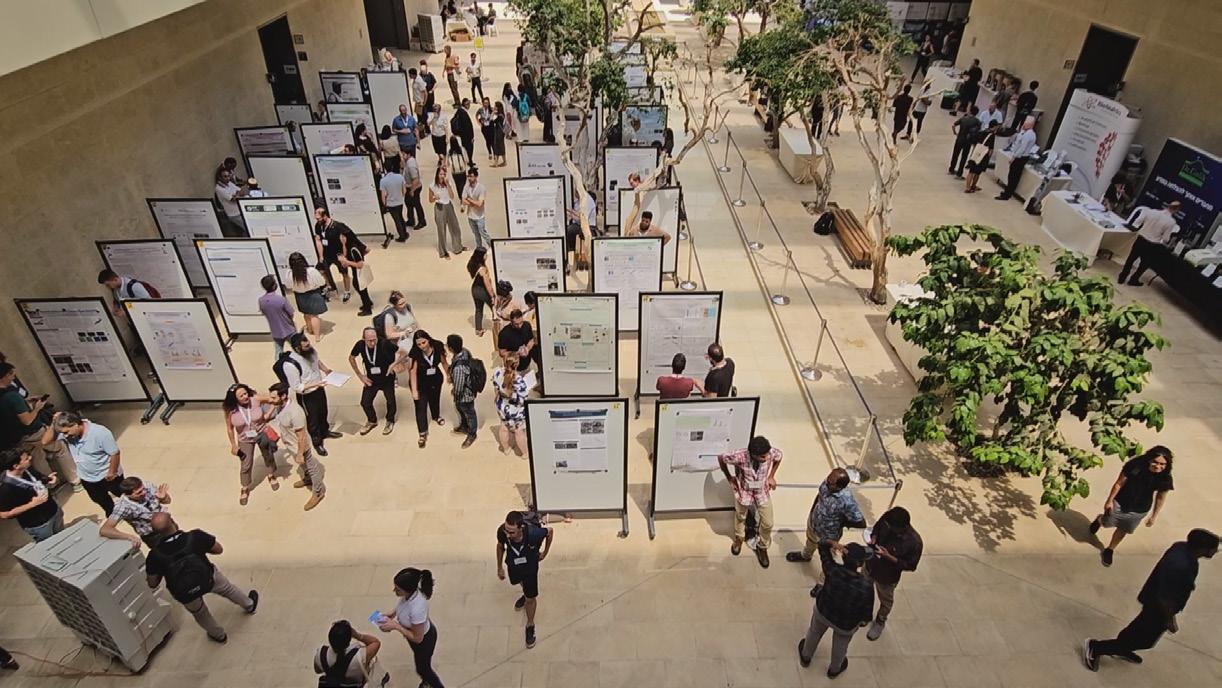
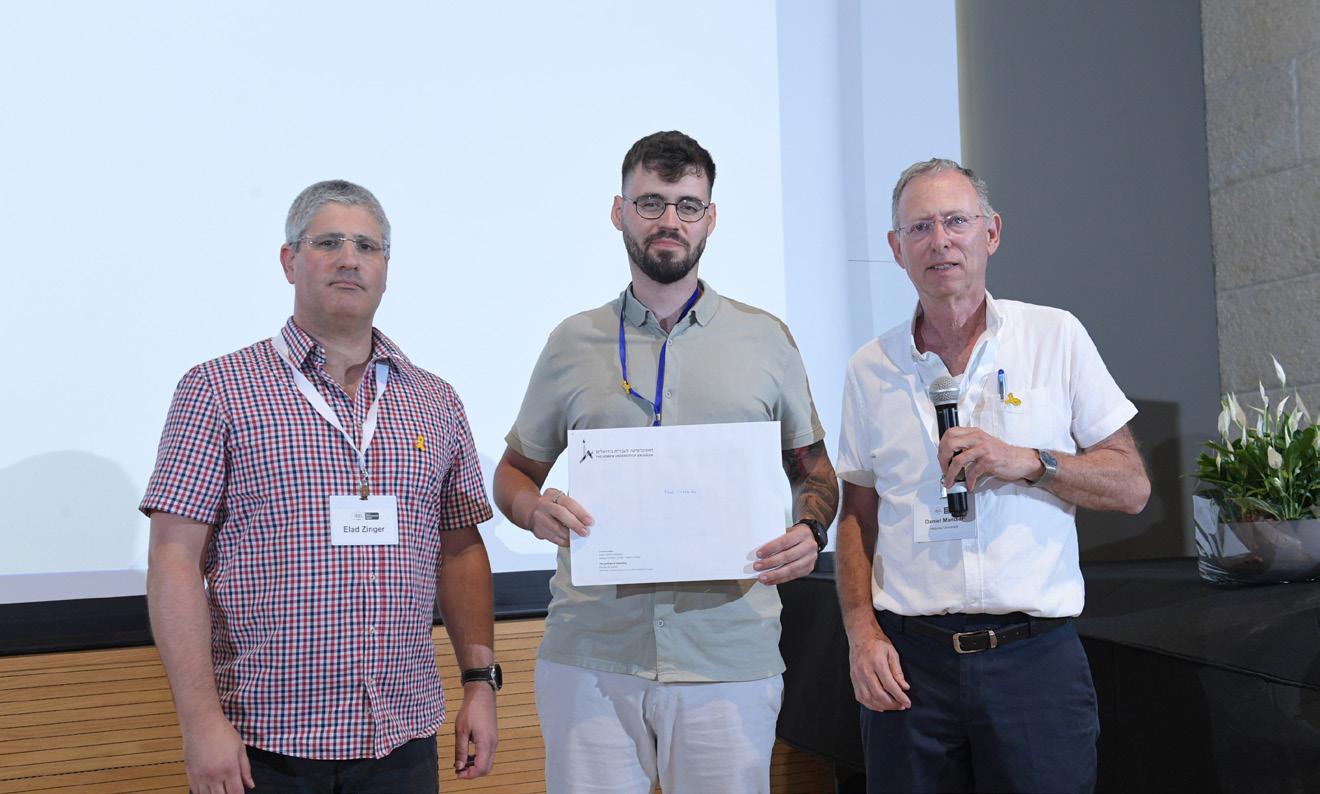
Prof. Yair Ein-Eli (Technion) presents the Israel Electrochemical Society national meeting plenary lecture, “Plus 1, 2, 3 & 4 Oxidation States in Anode Fuels… and More.”
One hundred students presented posters at the ECS Israel Section meeting. Elad Zinger and Daniel Mandler (left and right) present the “crowd favorite” lecture prize to Mr. Pavel Savchenko (center) for his talk on “The Effect of the Capping Agents of Nanoparticles on Their Redox Potential.”
ECS Pacific Northwest Section
The ECS Pacific Northwest (PNW) Section hosted its first Industry and Careers Day event on May 14, 2021. This inaugural event was created during the pandemic to connect prospective employees with potential companies, provide undergraduate and graduate students and postdoctoral researchers with mentorship, and promote careers in electrochemistry. Section Chair Prof. Corie L. Cobb and Secretary Prof. Shannon Boettcher co-organized the event. Virtual attendees participated in panels and breakout sessions with Microsoft, Boeing, Gamry, Thermo Fisher Scientific, Moses Lake Industries, Nel Hydrogen, Membrion, Oak Ridge National Laboratory, Pacific Northwest National Laboratory, and Lawrence Berkeley National Laboratory.
Building on the successful 2021 event, the ECS Pacific Northwest Section hosted their second Industry and Careers Day on June 13, 2024. To enable wide access, attendance was free and open to both ECS and non-ECS members. In addition to returning participants Microsoft and Oak Ridge National Laboratory, the section included new participants: Forge Nano Inc., Presidio Medical, Abbvie Inc., H10 Capital, and John Deere. Section Vice Chair Dr. April Li and Treasurer Dr. Jerome Babauta co-organized the event. Sessions
focused on providing career advice and perspectives for undergraduate and graduate students and postdoctoral researchers interested in careers in the electrochemical and solid state science fields. Panel members provided insights on their personal career experiences and skills valued in potential job candidates. The event helped connect students and postdoctoral researchers with industry and national laboratory representatives and provided information on upcoming opportunities in the rapidly expanding electrochemical technology area. The section thanks the speakers for their time engaging Pacific Northwest students in career discussions!
For those interested in learning more about electrochemistry and engaging with the section, the ECS Pacific Northwest Section is partnering with the Oregon Center for Electrochemistry (OCE) for a second year to host a joint electrochemistry conference at the University of Oregon (UO) on September 19 and 20.
It is free to join The Electrochemical Society Pacific Northwest Section. However, Society membership in good standing is required. To become a member or to renew your membership, contact the ECS Community Engagement Department
Reinventing
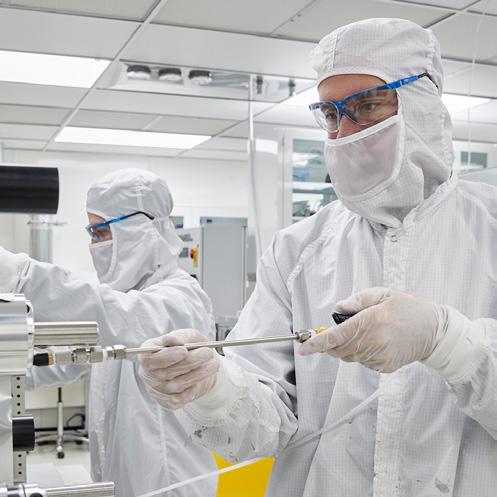
• Global leader in advanced battery materials
• Extensive patent portfolio available for licensing
• Unparalleled expertise in organic and inorganic synthesis applied to batteries
• In-house ultra-thin-lithium-foil (UTLF) anode production
• Cutting-edge pilot-lines in 5 dry rooms to provide mature proofs of concept
• State-of-the-art characterization labs in North America
• Capacity to carry out abuse and safety testing in accordance with international standards hydroquebec.com/center-excellence CEETSE-Partenariats2@hydroquebec.com
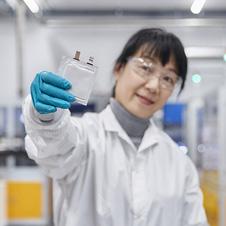
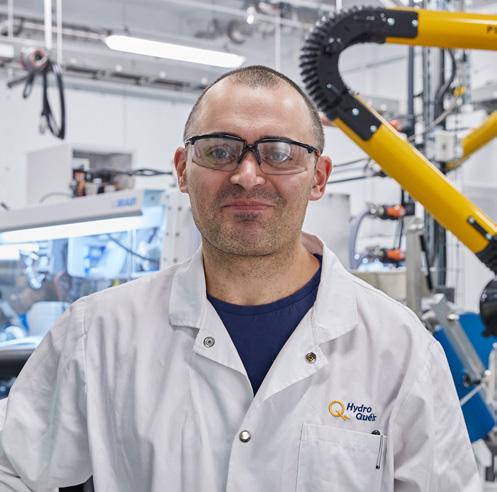
Section Name
Section Chair
Arizona Section Candace K. Chan
Brazil Section Raphael Nagao
Canada Section Steen B. Schougaard
Chile Section José H. Zagal
China Section Open
Detroit Section Erik Anderson
Europe Section Jan Macak
Georgia Section Faisal Alamgir
India Section Sinthai Ilangovan
Israel Section Daniel Mandler
Japan Section Yasushi Idemoto
Korea Section Won-Sub Yoon
Mexico Section Norberto Casillas Santana
Mid-America Section Nosang Myung
National Capital Section Chungsheng Wang
New England Section Sanjeev Mukerjee
Pacific Northwest Section Corie Cobb
Pittsburgh Section Open
San Francisco Section Xiong Peng
Singapore Section Zhichuan J. Xu
Taiwan Section Chi-Chang Hu
Texas Section Jeremy P. Meyers
Thailand Section Soorathep Kheawhom
Twin Cities Section Victoria Gelling
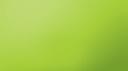


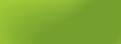
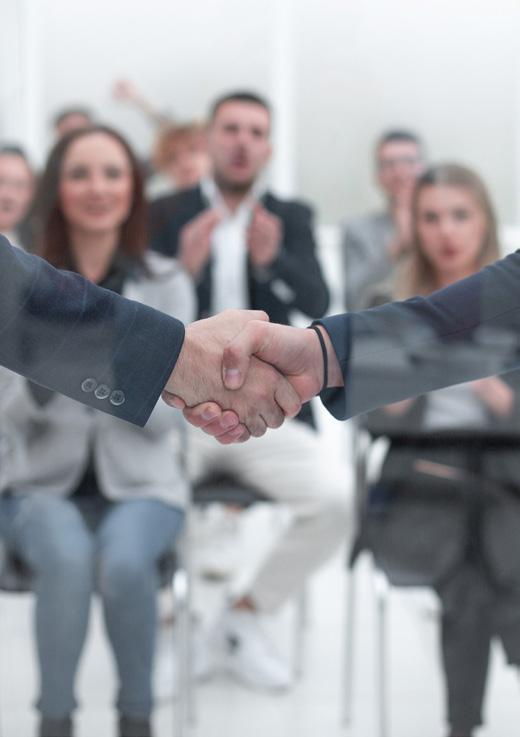
ECS Awards, Fellowships, and Grants
ECS recognizes outstanding technical achievements in electrochemistry, solid state science and related technologies, as well as exceptional service to the Society. Recognition is offered in Society, Division, Section, and Student Award categories. As today’s emerging scientists are our next generation of leaders, ECS also offers competitive fellowships and grants that enable students and young professionals in our field to make discoveries and shape science long into the future.
Society Awards
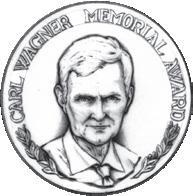
ECS Carl Wagner Memorial Award: Established in 1980, the award recognizes mid-career achievement, excellence in research areas of interest to the Society, and significant contributions in teaching/guiding students and colleagues in education, industry, or government. Awardees receive a sterling medal; framed certificate; ECS Life Membership; meeting registration; and travel assistance.
Nomination deadline: October 1, 2024
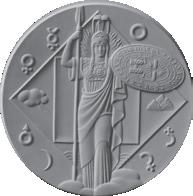
ECS Olin Palladium Award: Since 1950, this award has recognized distinguished contributions to electrochemical or corrosion science with a palladium medal; wall plaque; $7,500*; ECS Life Membership; and meeting registration.
Nomination deadline: October 1, 2024
ECS Toyota Young Investigator Fellowship:
Established in partnership with the Toyota Research Institute of North America in 2015, the award encourages young professionals and scholars to pursue research on batteries, fuel cells, hydrogen, and future sustainable technologies. Candidates receive restricted grants of no less than $50,000 to conduct their proposed research and ECS membership.
Materials deadline: January 31 annually
Fellow of The Electrochemical Society: Established in 1989, the fellow designation honors advanced individual technological contributions in electrochemical and solid state science and technology, and active membership and involvement with ECS. Fellows receive a framed certificate and a lapel pin.
Nomination period: September 1, 2024 – February 1, 2025
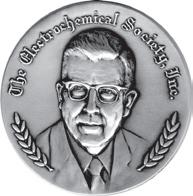
ECS Henry B. Linford Award for Distinguished Teaching: The award, which was established in 1981 to recognize excellence in teaching in subjects of interest to ECS, consists of a silver medal; plaque; $2,500; ECS Life Membership; and meeting registration.
Nomination period: September 1, 2024 – April 15, 2025
Leadership Circle Awards: Established in 2002 to honor and thank our electrochemistry and solid state science partners, these awards are granted in the year that an institutional member reaches a milestone level. Awardees receive a commemorative plaque and recognition in The Electrochemical Society Interface and ECS website.
Nominations are not accepted.
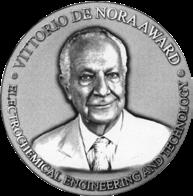
ECS Vittorio de Nora Award: The award was established in 1971 to recognize distinguished contributions to the field of electrochemical engineering and technology. It consists of a gold medal; plaque; $7,500; ECS Life Membership; and meeting registration.
Nomination period: September 1, 2024 – April 15, 2025
Division Awards
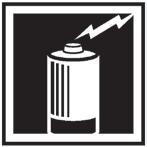
Battery Division Early Career Award Sponsored by Neware Corporation: Established in 2020 to encourage excellence among postdoctoral researchers in battery and fuel cell research, the award’s primary purpose is to recognize and support development of talented future leaders in the field. Winners receive a framed scroll; $2,000; and complimentary meeting registration.
Nomination period: October 15, 2024 – January 15, 2025
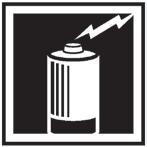
Battery Division Postdoctoral Associate Research Award Sponsored by MTI Corporation and the Jiang Family Foundation: Established in 2016 to encourage excellence among postdoctoral researchers in battery and fuel cell research, the award consists of a framed scroll; $2,000; and complimentary meeting registration. Two awards are granted annually.
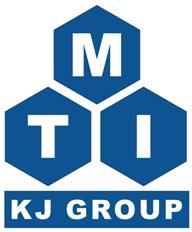
Nomination period: October 15, 2024 – January 15, 2025
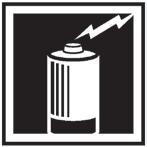
Battery Division Research Award: Established in 1958 to recognize excellence in battery and fuel cell research and to encourage publication in ECS outlets, the award recognizes outstanding contributions to the science of primary and secondary cells, batteries, and fuel cells. Winners receive a framed certificate and $2,000.
Nomination period: October 15, 2024 – January 15, 2025
(continued on next page)
AWARDS PROGRAM AWARDS PROGRAM
(continued from previous page)
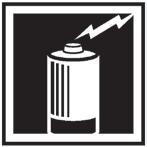
Battery Division Technology Award: To encourage the development of battery and fuel cell technology and to recognize significant achievements in this area, the award was established in 1993. It consists of a scroll; $2,000; and ECS Battery Division membership while recipients maintain ECS membership.
Nomination period: October 15, 2024 – January 15, 2025
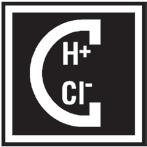
Corrosion Division H. H. Uhlig Award: This award of a scroll and $1,500 was established in 1973 to recognize excellence in corrosion research and outstanding technical contributions to the field of corrosion science and technology.
Nomination period: October 15, 2024 – January 15, 2025
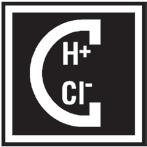
Corrosion Division Rusty Award for Mid-Career Excellence: Established in 2021 to recognize mid-career achievement and contributions to the field of corrosion science and technology, the award consists of a framed certificate; $1,000; meeting registration and possibly travel expenses.
Nomination period: October 15, 2024 – January 15, 2025
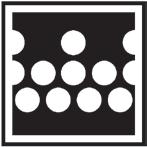
Electrodeposition Division Early Career Investigator Award: This award of a framed certificate and $1,000 was established in 2015 to recognize an outstanding young researcher in the field of electrochemical deposition science and technology.
Nomination period: October 15, 2024 – January 15, 2025
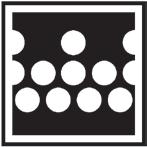
Electrodeposition Division Research Award: This award recognizes outstanding recent achievements in or research contributions to electrodeposition, demonstrated by quality papers published in the Journal of The Electrochemical Society or other ECS publications. Awardees receive a framed certificate and $2,000.
Nomination period: October 15, 2024 – January 15, 2025
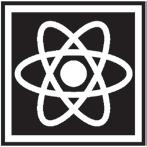
Energy Technology Division Walter van Schalkwijk Award for Sustainable Technology: Established in 2021 to recognize research scientists, academicians, and entrepreneurs making innovative and transformative contributions to sustainable energy technologies, the award consists of a framed certificate and $1,000.
Nomination period: October 15, 2024 – January 15, 2025
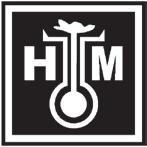
High-Temperature Energy, Materials, & Processes
Division J. Bruce Wagner, Jr. Young Investigator Award: The award (established in 1998) recognizes a young ECS member demonstrating exceptional promise for a successful career in science and/or technology in the field of high temperature materials. Awardees receive scrolls; $1,000; and possibly meeting registration and travel expenses.
Nomination period: October 15, even years – January 15, odd years
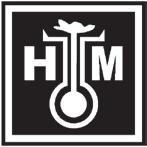
High-Temperature Energy, Materials, & Processes
Division Subhash Singhal Award: Excellence and exceptional SOFC and SOEC research contributions by distinguished researchers are recognized by this award established in 2017. Awardees receive a scroll and $1,000.
Nomination deadline: October 15, 2024 – January 15, 2025
Presented in July 2025 at the 19th International Symposium on Solid Oxide Fuel Cells (SOFC-XIX)
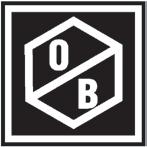
Organic and Biological Electrochemistry Division
Manuel M. Baizer Award: This award of a framed certificate and $1,000 was established in 1992 to recognize outstanding scientific achievements in the electrochemistry of organics and organometallic compounds and carbon-based polymers and biomass.
Nomination period: October 15, even years – January 15, odd years
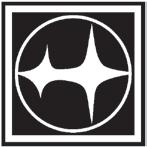
Physical and Analytical Electrochemistry Division
Max Bredig Award in Molten Salt and Ionic Liquid Chemistry: The award of a scroll and $1,500 was established in 1984 to recognize excellence in the field and to stimulate publication of high quality research papers in this area in the Journal of The Electrochemical Society
Nomination period: October 15, even years – January 15, odd years
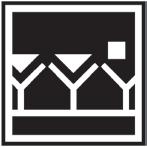
Sensor Division Early Career Award: Established in 2021 to recognize promising early career engineers’ and scientists’ research contributions to the field of sensors, the award encourages recipients to continue careers in the field and to remain active in the ECS Sensor Division. Awardees receive a framed certificate; $1,000; meeting registration; and division business meeting luncheon ticket.
Nomination period: October 15, 2024 – January 15, 2025
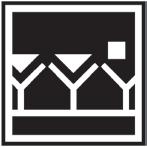
Sensor Division Outstanding Achievement Award: The award was created in 1989 to recognize outstanding achievement in service to the sensor community and research and/or technical contributions to the field of sensors, and to encourage excellence in the field. Awardees receive a framed certificate; $1,500; meeting registration; and division business meeting luncheon ticket.
Nomination period: October 15, 2024 – January 15, 2025
Student Awards
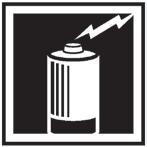
Battery Division Student Research Award Sponsored by Mercedes-Benz Research & Development: Founded to recognize promising young engineers and scientists enrolled in a college or university in the field of electrochemical power sources, the award of a complimentary meeting registration and up to $1,000 in travel reimbursement . encourages recipients to initiate or to continue careers in the field.
Nomination period: October 15, 2024 – January 15, 2025
Biannual Meeting Travel Grants: ECS divisions and sections offer travel grants to undergraduates, graduate students, postdoctoral researchers, young professionals, and faculty presenting papers at ECS biannual meetings. Grants range from complimentary meeting registration to luncheon/reception tickets, travel expenses, and more. The divisions and sections maintain their own application requirements.
Application period for 247th ECS Meeting Travel Grants: December 6, 2024 – February 24, 2025
Canada Section Student Award: Established in 1987 to recognize promising young engineers and scientists in the field of electrochemical power sources, this award of $1,500 is intended to encourage recipients to initiate or to continue careers in the field.
Nomination period: September 30, 2024 – February 28, 2025
AWARDS AWARDSAWARDSPROGRAM AWARDS PROGRAM
Colin Garfield Fink Fellowship: Since 1962, the fellowship has supported research by a postdoctoral scientist/researcher from June through September in a field of interest to the Society. The award consists of $5,000 and publication of a summary report in ECS Interface
Materials deadline: January 15 annually
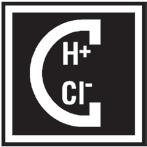
Corrosion Division Morris Cohen Graduate Student Award: Established in 1991 to recognize and reward outstanding graduate research in the field of corrosion science and/or engineering, the award consists of a framed certificate; $1,000; and possibly travel expenses.
Nomination period: October 15, 2024 – January 15, 2025
ECS General Student Poster Session Awards: Established in 1993 to stimulate active student interest and participation in the Society, the session enables undergraduate and graduate students to present research results of general interest to ECS. Accepted posters are eligible for General Student Poster Awards: 1st place, $1,500; 2nd place, $1,000; and 3rd place, $500. For award consideration, authors must submit abstracts and be accepted into the Z01 General Student Poster Session; upload a digital poster; and be present during inperson judging.
Materials deadline: 247th ECS Meeting abstract deadline, December 6, 2024
ECS Korea Section Student Award: This award of $500 was established in 2005 to recognize academic accomplishments by a student pursuing a PhD at a Korean University in any area of science or engineering in which electrochemical and/or solid state science and technology is the central consideration.
Nomination period: September 15 – December 31, 2024
ECS Outstanding Student Chapter Award: Student chapters are recognized for demonstrating active participation in the Society’s technical activities; establishing community and outreach activities in electrochemical and solid state science and engineering education; and creating and maintaining robust membership bases. The Outstanding Student Chapter receives a plaque; certificates; $1,000; and award recognition with chapter photo in ECS Interface or other electronic communications. Up to two Chapters of Excellence are also awarded.
Materials deadline: April 15 annually
ECS Summer Fellowships: The Edward G. Weston, Joseph W. Richards, F. M. Becket, and H. H. Uhlig Fellowships were established in 1928 to support student research from June through August in fields of interest to ECS. Fellows receive $5,000 and publication of a summary report in ECS Interface.
Materials deadline: January 15 annually
Pacific Northwest Section Electrochemistry Student Award Sponsored by Thermo Fisher Scientific: This $1,000 award was established in 2021 to recognize promising young engineers and scientists in Washington, Oregon, and Idaho pursuing PhDs in electrochemical engineering and applied electrochemistry.
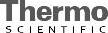
Nomination period: September 15, 2024 – February 28, 2025
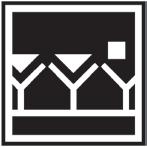
Sensor Division Student Research Award: Established in 2021 to recognize promising graduate students conducting outstanding research in the field of sensors, the award consists of a framed certificate; $500; meeting registration; and division luncheon ticket.
Nomination period: October 15, 2024 – January 15, 2025
* All awards are in US dollars.
Class of 2024 Fellows of The Electrochemical Society
ECS has named 13 members to the 2024 Class of ECS Fellows. The designation “Fellow of The Electrochemical Society” was established in 1989 for advanced individual technological contributions to the fields of electrochemistry and solid state science and technology, and for service to the Society. These members are recognized for scientific achievement, leadership, and active participation in ECS affairs. Each year, their peers choose up to 15 renowned scientists and engineers for this honor.
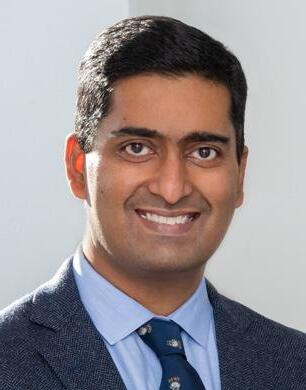
Rohan Akolkar is the Milton and Tamar Maltz Professor of Energy Innovation at Case Western Reserve University (CWRU). He is an Ohio Eminent Scholar in Advanced Energy Research, serves as Faculty Director of CWRU’s Great Lakes Energy Institute, and holds a joint appointment as Chief Scientist at Pacific Northwest National Laboratory. His research spans many areas of electrochemical engineering, embracing electrodeposition, electrometallurgy, and electrochemical materials development for applications in nanoelectronics, batteries, sensors, and in extracting and refining critical materials. He has made important contributions to fundamental and applied electrochemistry, including patented electrochemical processes and materials which enable high-performance interconnects in advanced semiconductor devices; novel electrowinning and electrorefining processes for the extraction and recycling of metals; fundamental studies unraveling mechanisms of dendrite formation in batteries; and a novel sensor for detecting heavy-metal contaminants in water.
Prof. Akolkar received a PhD in Chemical Engineering from CWRU in 2004. He worked in industrial R&D at Intel Corporation for eight years before joining the CWRU faculty in 2012. His research
has been recognized by CWRU’s School of Engineering Innovation and Research Awards, 2023 ECS Electrodeposition Division Research Award, 2004 ECS Norman Hackerman Young Author Award, and numerous industry awards during his tenure at Intel. In 2021, he was elected Senior Member of the National Academy of Inventors. Prof. Alkokar joined ECS in 2009, has served as Associate Editor of the Journal of The Electrochemical Society, and currently is the inaugural Editor-in-Chief of ECS Advances, as well as Secretary of the ECS Electrodeposition Division.
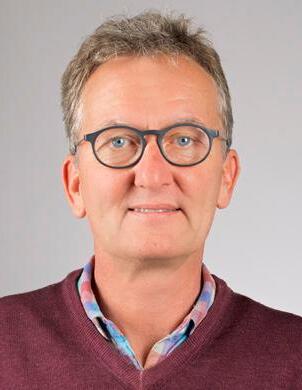
Felix N. Büchi is Group Head of the Electrochemistry Laboratory at the Paul Scherrer Institut (PSI) Center for Energy and Environmental Sciences. He graduated as a chemist from the Universität Bern, received a PhD in Fundamental Electrochemistry in 1989, and then joined Alan Bond’s group at Deakin University as a Postdoctoral Fellow. In 1991, Dr. Büchi became a Staff Scientist in PSI’s Electrochemistry Laboratory, working on PEM fuel cell technology. Dr. Büchi spent a year as Guest Scientist in S. Srinivasan’s group at Texas A&M (continued on next page)
AWARDS PROGRAM AWARDS PROGRAM
University (1994–1995). He became leader of the PSI Fuel Cell Systems and Diagnostics group in 2002, experimenting with the engineering of mobile fuel cell systems and material developments. On the diagnostics level, he and his team focused on the development of X-ray imaging under operando conditions to characterize mass transport and two-phase flow in the porous materials of PEM fuel cells and electrolyzers. Advancing this important characterization/ imaging technology of electrochemical converters has resulted in about 50 dedicated papers since 2009. From 2018 to 2024, he also served as the head of PSI’s Electrochemistry Laboratory. Dr. Büchi became an ECS member in 1994.
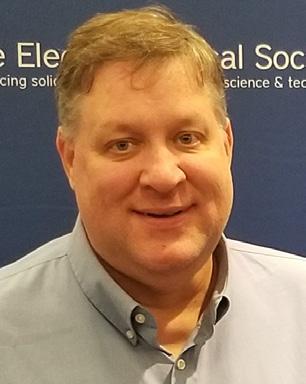
David Cliffel is the Cornelius Vanderbilt Professor and Vice Chair and Director of Graduate Studies in the Department of Chemistry at Vanderbilt University. His innovative research group focuses on electroanalytical measurements and sensor development in nanoscale and biological systems and created multianalyte microphysiometry by combining microfluidic technologies with electrochemical detection to study the dynamic behaviors of metabolic pathways with potential applications in cancer, diabetes, and toxicology. In addition, he developed Photosystem I-based biohybrid solar cells using photosynthetic protein molecules isolated from spinach leaves. His aim is to increase direct charge transfer between Photosystem I protein molecules and the electrode surface, using small molecule mediators, nano-carbon materials, conductive polymers, and nanoparticles.
Prof. Cliffel received his PhD in Chemistry from the University of Texas at Austin in 1998 under the direction of Allen J. Bard, was a Postdoctoral Assistant with Royce W. Murray at the University of North Carolina at Chapel Hill, and joined Vanderbilt University in September 2000. He has published more than 130 peer-reviewed journal articles and three book chapters. Prof. Cliffel joined ECS in 1996 and has served as JES’s Technical Editor for Physical and Analytical Electrochemistry, Electrocatalysis, and Photoelectrochemistry since 2016.
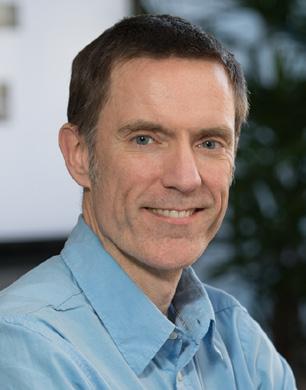
Jeffrey Elam is a Distinguished Fellow, Senior Chemist, and Group Leader in the Applied Materials Division at Argonne National Laboratory. His research focuses on building a fundamental understanding of the chemical mechanisms for thin film growth with an emphasis on atomic layer deposition. Dr. Elam has advanced the science and technology of thin film coatings to solve critical materials challenges. He helped develop the world’s largest microchannel plate (MCP), a solid state, 2D electron amplifier critical to a variety of imaging and sensing applications. Dr. Elam used nanocomposite coatings to create a manufacturing strategy for MCPs that drove down cost, improved performance, and widened applications. He coinvented the Oleo Sponge, an engineered foam that absorbs oil from water. This reusable sorbent is functionalized to be oleophilic and hydrophobic using sequential infiltration synthesis, a method patented by Elam.
After completing his BA at Cornell University, Dr. Elam received a PhD in Physical Chemistry from the University of Chicago, followed by a postdoc in Steve George’s group at the University of Colorado, Boulder. He has published more than 300 manuscripts and holds more than 90 inventions, including licensed patents that have led to commercial products. An AVS Fellow, his work has been recognized by five R&D100 Awards and the 2019 University of Chicago Board of Governors Distinguished Performance Award, 2018 Argonne Lifetime Achievement Award for Commercialization Excellence, 2017 Editor’s Choice Top Invention Award, and 2017 AVS ALD Innovation Award. He joined ECS in 2007.
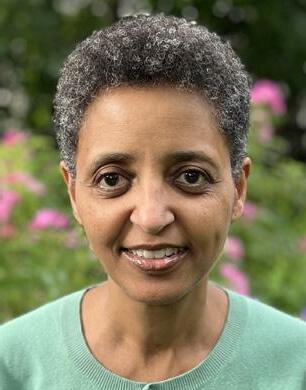
Sossina M. Haile is the Walter P. Murphy Professor of Materials Science and Engineering at Northwestern University (NU). Her research broadly encompasses materials, especially oxides, for sustainable electrochemical energy technologies. Her work in fuel cell science and technology has pushed the field to new insights and record performance metrics. In parallel, she has created new avenues for harnessing sunlight to meet rising energy demands and demonstrated viable solutions to the challenge of hydrogen delivery.
Prof. Haile earned her PhD in Materials Science and Engineering from the Massachusetts Institute of Technology in 1992. She was a California Institute of Technology faculty member for 18 years before joining NU in 2015. Among her many awards, she has received the 2020 Turnbull Lectureship; 2012 International Ceramics Prize; 2010 Chemical Pioneer Award; and in 2008, an American Competitiveness and Innovation (ACI) Fellowship from the US National Science Foundation in recognition of “her timely and transformative research in the energy field and her dedication to inclusive mentoring, education, and outreach across many levels.” She is a Fellow of the Materials Research Society, American Ceramics Society, Royal Society of Chemistry, African Academy of Sciences, and Ethiopian Academy of Sciences, and serves on the editorial boards of Joule and MRS Energy and Sustainability. Currently, she is on the DOE Basic Energy Sciences Advisory Board and the nonprofit Ethiopia Education Initiatives board. She joined ECS in 2006.
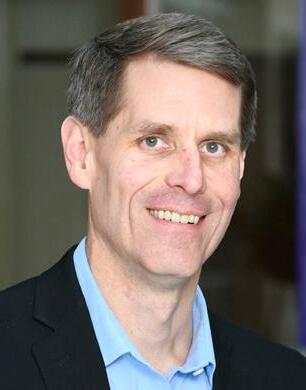
Mark C. Hersam is the Walter P. Murphy Professor of Materials Science and Engineering, Director of the Materials Research Center, and Chair of the Materials Science and Engineering Department at Northwestern University. He also holds faculty appointments in Chemistry, Applied Physics, Medicine, and Electrical Engineering. His research interests include nanomaterials, additive manufacturing, nanoelectronics, scanning probe microscopy, renewable energy, and quantum information science.
Prof. Hersam earned a BS in Electrical Engineering from the University of Illinois at Urbana-Champaign (UIUC) in 1996, MPhil in Physics from the University of Cambridge in 1997, and PhD in Electrical Engineering from UIUC in 2000. Dr. Hersam has received honors that include the Presidential Early Career Award for Scientists and Engineers, TMS Robert Lansing Hardy Award, MRS Mid-Career Researcher Award, AVS Medard Welch Award, US Science Envoy, MacArthur Fellowship, and eight Teacher of the Year Awards. Dr. Hersam has been repeatedly named a Clarivate Highly Cited Researcher with more than 700 peer-reviewed publications and over 70,000 citations. An elected member of the American Academy of Arts and Sciences, National Academy of Engineering, and National Academy of Inventors with more than 170 issued and pending patents, Dr. Hersam has founded two companies, NanoIntegris and Volexion, which are suppliers of nanoelectronic and battery materials, respectively. Dr. Hersam is a Fellow of MRS, ACS, AVS, APS, AAAS, SPIE, and IEEE, and serves as an Executive Editor of ACS Nano. An ECS member since 2007, he received the 2010 Nanocarbons SES Research Young Investigator Award.

Masayuki Itagaki is a Professor in the Department of Pure and Applied Chemistry in the Faculty of Science and Technology at the Tokyo University of Science. He is known for his contributions to theories of electrochemical impedance spectroscopy, including 3D impedance measurement, transmission line models for fractal structure, Faraday impedance for metal dissolution and passivation, and combination with channel flow electrodes. With his co-workers, he has
Photo: Adam Matthews
AWARDS AWARDSAWARDSPROGRAM AWARDS PROGRAM
successfully explored the electrochemistry of localized corrosion, corrosion sensors, electro and electroless plating, fuel cells, biosensors, and Li-ion batteries.
Prof. Itagaki received a PhD in Metallurgical Engineering from the Tokyo Institute of Technology in 1993, followed by a postdoctorate in the Corrosion Laboratory at the Université de Bourgogne. He was named Assistant Professor at the Tokyo University of Science in 1994, Lecturer in 1998, Associate Professor in 2001, and full Professor in 2005. Prof. Itaki joined ECS in 1997 and has served as Chair of the ECS Corrosion Division (2018–2020) and member of the Board of Directors (2018–2020). Since 2020, he has convened the ISO technical group working on electrochemical test methods for corrosion of metals and alloys (ISO/TC156/WG11) and taken part in establishing many international standards for test methods for corrosion engineering. The author of more than 300 original research papers in the field of electrochemistry, he has chaired the 9th International Symposium on Electrochemical Impedance Spectroscopy (EIS 2013) and 2nd Asian Symposium on Electrochemical Impedance Spectroscopy
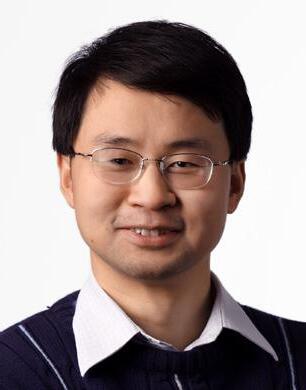
Song Jin is the Francis J. DiSalvo Professor of Physical Science at the University of Wisconsin–Madison. His research interests focus on the chemistry, physics, and technological applications of nanoscale and solid state materials. He has developed innovative syntheses of a variety of nanomaterials, including metal chalcogenides, silicides, and halide perovskites, and discovered and developed the screw dislocation-driven growth of nanomaterials. Building on the fundamental understanding of novel physical properties, Prof. Jin has advanced the exploitation of (nano)materials for electrocatalysis, photoelectrochemical solar energy conversion, electrochemical energy storage, optoelectronics, nanospintronics, and biotechnology.
Prof. Jin received his BS in Chemistry from Peking University in 1997, PhD in 2002 from Cornell University under the direction of Prof. Francis J. DiSalvo, and completed postdoctoral research under Prof. Charles M. Lieber at Harvard University. He has authored or co-authored more than 270 publications and 13 patents. Prof. Jin serves as a Senior Editor for ACS Energy Letters. Recognition of his achievements includes in 2022, the WARF Named Professorship at UW–Madison; 2014 ACS Inorganic Nanoscience Award; 2013 H. I. Romnes Faculty Fellowship; 2012 Vilas Associate Award; 2012 Research Corporation Scialog Collaborative Innovation Award for Solar Energy Conversion; 2011 Research Corporation Scialog Award for Solar Energy Conversion; 2009 Alfred P. Sloan Research Fellowship; 2008 ACS ExxonMobil Solid State Chemistry Fellowship; 2007 Research Corporation Cottrell Scholar Award; 2006 MIT Technology Review TR35 Award (Top 35 Young Innovators under the age of 35); and 2006 NSF CAREER Award. Clarivate has identified Prof. Jin as a Highly Cited Researcher continuously since 2018 He became an ECS member in 2008.
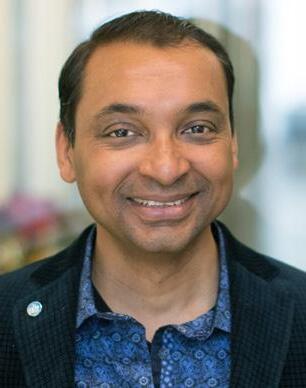
Sushanta Mitra is Full Professor in the Department of Mechanical and Mechatronics Engineering at the University of Waterloo with cross-appointments in Chemical Engineering, Electrical and Computer Engineering, Physics and Astronomy, and Chemistry. As the Executive Director of the Waterloo Institute for Nanotechnology (WIN), Prof. Mitra spearheads Canada’s largest nanotechnology institute, a hub for cutting-edge research and innovation. He is the Founder and CEO of successful Canadian and Dutch start-ups, bridging scientific innovation with practical applications.
Prof. Mitra completed a BS at Jadavpur University (1995), MS at University of Victoria (1997), and PhD in Mechanical Engineering at the University of Waterloo (2001). Prior to joining Waterloo’s faculty, Prof. Mitra served as Department Chair at the Lassonde School of Engineering, Associate Vice-President Research at York University, and Assistant Vice-President of Research at the University of Alberta.
He is a Fellow of the Canadian Academy of Engineering, American Society of Mechanical Engineers, Canadian Society for Mechanical Engineering, Engineering Institute of Canada, Royal Society of Chemistry, American Physical Society, American Association for the Advancement of Science, Indian National Academy of Engineering, and National Academy of Sciences India. His work has garnered significant recognition, including UWaterloo’s 2018 and 2020 President’s Excellence in Teaching and Research awards; American Society of Mechanical Engineers 2019 Prominent Researcher Award and 2011 Outstanding Researcher Award; 2018 NRI Welfare Society of India Hind Rattan Award (Jewel of India); 2015, 2016, and 2017 York University Research Leaders Awards; and 2015 Ontario Society of Professional Engineers Engineering Excellence Medal. He joined ECS in 2012.
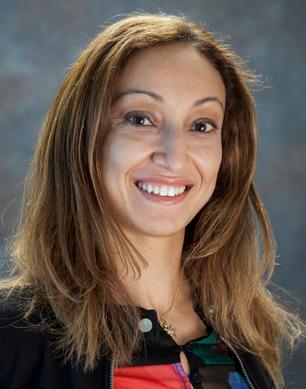
Rana Mohtadi is a Senior Principal Scientist at Toyota Research Institute of North America (TRINA) and holds a Principal Investigator affiliate position at the Advanced Institute for Materials Research (AIMR) at Tohoku University. She has established and spearheaded research programs aimed at the design and demonstration of high value materials for chemical and electrochemical energy storage technologies. Her research encompasses the development of liquid and solid state electrolyte materials for mono and multivalent beyond–Li-ion batteries, the creation of environmentally friendly chemistries, enabling sustainable batteries, and developing hydrogen storage materials. Her groundbreaking work has led to transformative material advancements resulting in technology handovers for application considerations and influential peer-reviewed articles, including her pioneering work that closed key gaps and uncovered unexpected fundamental interfacial phenomena that set a new trend of electrolytes research in multivalent batteries.
Dr. Mohtadi received her PhD in Chemical Engineering from the University of South Carolina where she researched proton exchange membrane PEM fuel cells. She has published in the areas of batteries, hydrogen storage materials, and fuel cells, and holds more than 35 patents. The recipient of a 2011 R&D 100 award, she was named in “40 under 40” in 2014 by Automotive News and Crain’s Detroit Business News, and recognized and showcased as a face of cuttingedge innovation in Michigan State in the governor’s 2015 “We Run On Brain Power” initiative. She became an ECS member in 2012.
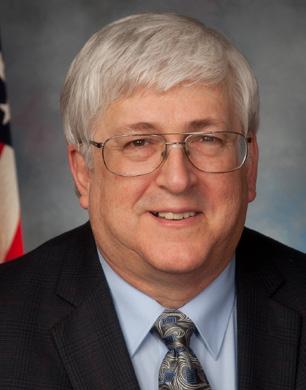
David A. Shifler is a Science & Technology Program Officer at the Office of Naval Research (ONR) supporting fundamental research of high temperature propulsion materials for aircraft and shipboard gas turbine engines. He also manages and supports fundamental and applied research in ONR’s program on corrosion science and corrosion control technologies. Dr. Shifler is responsible for recognizing promising new scientific and technological concepts and findings in their infancy and evaluating their feasibility for, and applicability to Department of Navy (DoN) missions. He supports research in the creation, development, and characterization of materials by involving the use of integrated computational materials science and engineering with experimental validation to develop models describing material degradation and corrosion mechanisms and phenomena as well as material processes that guide understanding of materials performance and drive further materials innovations. In the Corrosion Program, Dr. Shifler seeks to create a science-based understanding of corrosion and protective options through damage evolution mechanisms, corrosion-informed materials concepts, processing, electrochemistry, and surface protection and modification sciences.
After completing a BA in Chemistry at Western Maryland College (1973), Dr. Shifler earned his MSE and PhD at Johns Hopkins University under Drs. Patrick Moran and Jerome Kruger, exploring the phenomena of nonaqueous corrosion. He spent over 20 years
(continued on next page)
Photo: Department of Chemistry, University of Wisconsin–Madison
Photo: ONR
(continued from previous page)
AWARDS PROGRAM AWARDS PROGRAM
in industry before joining ONR. A Fellow of NACE International, ASM International, Institute of Corrosion, American Association for the Advance of Science, and Washington Academy of Sciences, Dr. Shifler received NACE International’s 2016 Frank Newman Speller Award for outstanding national and international contributions to corrosion engineering. He has written and/or edited three books (including LaQue’s Handbook of Marine Corrosion, 2nd Edition, [ECS Series of Texts and Monographs, Wiley, 2022]), and numerous book chapters and technical papers. An ECS member for more than 35 years, he has served as Chair or Co-chair of ECS aqueous and high temperature symposia and on numerous administrative committees.
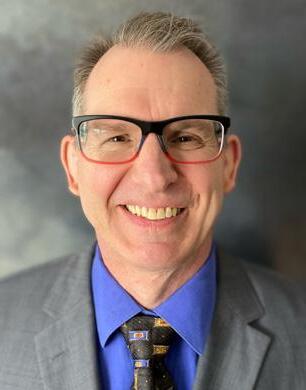
Jean St-Pierre is Technical Advisor at Cummins, Inc. and Affiliate Graduate Faculty/Adjunct in the Department of Electrical and Computer Engineering at the University of Hawaiʻi at Mānoa. His research focuses mostly on degradation aspects of proton exchange membrane fuel cells (PEMFCs) for heavy duty applications and proton exchange membrane water electrolyzers (PEMWEs), including contamination and the behavior of PEMFCs below water’s freezing point.
After completing a PhD in Metallurgical Engineering at Polytechnique Montréal, he held the positions of Principal Research Scientist, Research Professor, and Researcher at, respectively, Ballard Power Systems (1995–2005), an acknowledged fuel cell manufacturing leader; University of South Carolina (2006–2010), formerly the site of the sole National Science Foundation industry/ university collaborative research center for fuel cells; and the Hawaiʻi Natural Energy Institute (2010–2021). Dr. St-Pierre has published more than 85 journal papers and book chapters with more than 6,000 citations and contributed to 30+ patents. He joined ECS in 1984 and has been active with the Finance Committee (2014–2018), ECS Energy Technology Division (Treasurer, Secretary, Vice Chair, and Chair, 2005–2013), and currently as a member of the Honors & Awards Committee (2012–2024) and PRiME 2024 Organizing Committee (2022–2024). Dr. St-Pierre received the first ECS Canada
Section Student Award (1988) and currently is co-editor of the JES focus issues on PEMFC and PEMWE durability (2018, 2021, 2024). An editorial board member of Molecules, Dr. St-Pierre co-edited its special issue on PEMFCs.
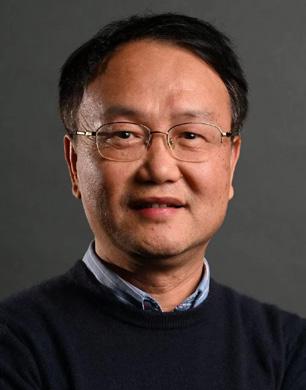
Yong Yang is Distinguished Professor in Chemistry in the State Key Lab for Physical Chemistry of Solid Surfaces at Xiamen University. His main research interests are electrode/electrolyte materials for highenergy density batteries, solid state batteries, interfacial electrochemical reaction mechanisms and in situ spectroscopic techniques such as NMR and XAS, and Liion battery aging and degradation analysis for power and storage applications.
Prof. Yang received his BSc (1984) and PhD (1992) in Physical Chemistry from Xiamen University. After joining the university’s faculty in 1993, he was promoted to his current position in 2005. He was a Visiting Scholar in the Physical and Theoretical Chemistry Lab at Oxford University from 1997 to 1998 and Director of the Electrochemistry and Electrochemical Engineering Research Institute at Xiamen University from 2008 to 2018. The author of more than 500 papers in refereed national and international journals with a Google h-index of 93, he is the lead editor of Solid State Electrochemistry (Chemical Industry Press, 2017 [Chinese language edition]) and NMR/MRI of Electrochemical Energy Storage Materials and Devices (Royal Society of Chemistry, 2021 [English language edition]). Prof. Yang has received notable awards that include the 2020 ECS Battery Division Technology Award, 2017 Chinese Electrochemical Society Distinguished Contribution Award, 2014 International Battery Materials Association Technology Award, and 1999 National Natural Science Foundation of China Outstanding Young Scholar Fund award. He has been an Editor for the Journal of Power Sources since 2016. The President of the International Battery Materials Association for the 2024–2025 term, Prof. Yang is a board member of the International Meeting on Lithium Batteries (2012–present) and International Battery Materials Association (2015–present). Over the past 30 years, he has mentored more than 80 postdocs, PhD, and MSc students. He became an ECS member in 1997.
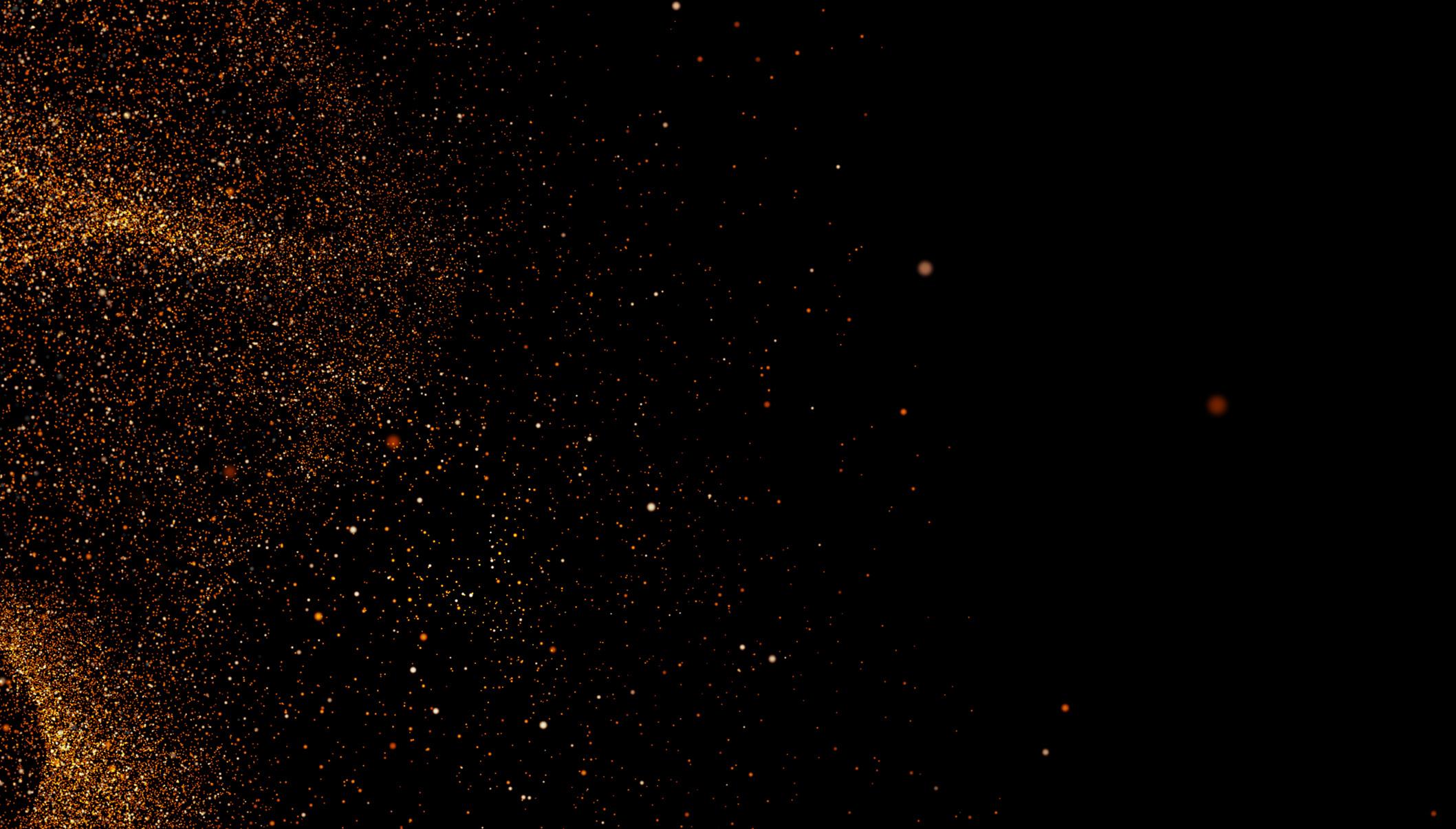
ECS FELLOWS 2025
Call for Nominations
Deadline: February 1, 2025
ECS is proud to announce the new members for April, May, and June 2024 (Members are listed alphabetically by family/last name.)
Members
A
Ryan Abranovic, Lincoln, RI, USA
Yaset Acevedo, Charlottesville, VA, USA
Faiz Ahmed, Berkeley, CA, USA
Juhyeon Ahn, Berkeley, CA, USA
Zakiya Al Amri, Salalah, Oman, Oman
Khalid Alhooshani, Dhahran, El Hasa Province, Saudi Arabia
Tawaddod Alkindi, Dubai, Abu Dhabi, UAE
Beyene Aregawi, New Taipei, New Taipei City, Taiwan
Dipak Aryal, Pflugerville, TX, USA
Fikret Aydin, Dublin, CA, USA
B
Deepak Badgurjar, Chicago, IL, USA
Todd Ballinger, Downingtown, PA, USA
Mira Baraket, Taastrup, Sjaelland, Denmark
Pooja Basera, Menlo Park, CA, USA
Mikhael Bechelany, Montpellier, Occitanie, France
Dagmar Becker, Palo Alto, CA, USA
Lucas Becker, Burghausen, BY, Germany
Joris Bleekman, Velp, Gelderland, Netherlands
Luca Boarino, Torino, PM, Italy
Peter Bouwman, Herzogenaurach, BY, Germany
Buket Boz, Vienna, Bundesland, Austria
Jennifer Brantley, New Braunfels, TX, USA
William Brezinski, Beaverton, OR, USA
Michaela Burke Stevens, Menlo Park, CA, USA
C
Carlos Casadevall, Hayward, CA, USA
Cagdas Cetin, Maple Ridge, BC, Canada
Merika Chanthanumataporn, Patumwan, Bangkok, Thailand
Rongrong Cheacharoen, Bangkok, Bangkok, Thailand
Tao Cheng, Arcadia, CA, USA
Tonya Cherukuri, Houston, TX, USA
Jun-Chau Chien, Berkeley, CA, USA
Suji Choi, San Jose, CA, USA
Yin-Chi Chu, Burnaby, BC, Canada
Jason Cooper, Berkeley, CA, USA
Stephen Cox, Cambridge, Cambridgeshire, UK
Tanja Cuk, Boulder, CO, USA
DJahan Dawlaty, Los Angeles, CA, USA
Maria De Leo, Torino, PM, Italy
Lynn Dennany, Glasgow, Scotland, UK
Allison Dennis, Boston, MA, USA
Maria Vittoria Diamanti, Milan, LM, Italy
Eric Dickey, Hillsboro, OR, USA
Angus Dickinson, Swindon, Wiltshire, UK
Johanna Dombrovskis, Gothenburg, Vastra Gotaland, Sweden
EKen Elen, Diepenbeek, Limburg, Belgium
FFelix Fischer, Oakland, CA, USA
Salome Forel, Villeurbanne, AuvergneRhône-Alpes, France
David Frank, Berkeley, CA, USA
Kai Fu, Salt Lake City, UT, USA
Jay Fuhr, Del Mar, CA, USA
Mayumi Fukumine, Yokohama-shi, Kanagawa, Japan
GNicolas Gauthier, Grenoble, AuvergneRhône-Alpes, France
Nemanja Gavrilov, Belgrade, Serbia and Montenegro, Serbia
Alice Gillen, Alameda, CA, USA
Omar Gomez Rojas, Ueda, Nagano, Japan
Zachary Gossage, Shinjuku, Tokyo, Japan
Alexis Grimaud, Cambridge, MA, USA
Nathan Guisinger, Woodridge, IL, USA
Jinghua Guo, Berkeley, CA, USA
HMai-Anh Ha, Lakewood, CO, USA
Amy Haghdoost, Pleasanton, CA, USA
Gwon Deok Han, Seoul, Gyeonggi-do, ROK
Jeong Hwan Han, Seoul, Gyeonggi-do, ROK
Masanori Hashimoto, Hagagun, Tochigi, Japan
Ryu Hasunuma, Namiki, Tsukuba, Ibaraki, Japan
Simelys Hernandez, Turin, PM, Italy
Phillip Hicks, Houston, TX, USA
Satoru Hiroto, Kyoto, Kyoto, Japan
Watcharaporn Hoisang, Ueda, Nagano, Japan
Guosong Hong, Stanford, CA, USA
Jefford Humes, Highland Park, IL, USA
Zelia Hunts, Santa Clara, CA, USA
Dorottya Hursan, Szeged, Southern Great Plain, Hungary
Gyuweon Hwang, Seoul, Gyeonggi-do, ROK
IPawin Iamprasertkun, Khlong Luang, Pathum Thani, Thailand
Shoji Iguchi, Kyoto, Kyoto, Japan
Muhammad Ihsan-Ul-Haq, Berkeley, CA, USA
Sung Gap Im, Daejeon, South Chungcheong, ROK
Mizuki Ito, Hiratsuka, Kanagawa, Japan
Jyotsna Iyer, San Jose, CA, USA
J
Badrinath Jagannath, Santa Clara, CA, USA
Bruno Janegitz, Araras, São Paulo, Brazil
Dawoon Jang, Columbus, OH, USA
Nuwanthaka Jayaweera, Romeoville, IL, USA
Sohee Jeong, Seoul, Gyeonggi-do, ROK
Qiu Jin, Corvallis, OR, USA
Xiuyu Jin, Pinole, CA, USA
Siriporn Jungsuttiwong, Bangkok, Bangkok, Thailand
K
Matthew Kanan, Stanford, CA, USA
Byoungwoo Kang, Pohang, North Gyeongsang, ROK
Mijeong Kang, Busan, South Gyeongsang, ROK
Surendra Karki, Richland, WA, USA
Ayon Karmakar, Merced, CA, USA
Maarit Karppinen, Espoo, Uusimaa, Finland
Jitti Kasemchainan, Bangkok, Bangkok, Thailand
Tomoya Kawaguchi, Sendai, Miyagi, Japan
Egon Kecsenovity, Szeged, Southern Great Plain, Hungary
Sunilkumar Khandavalli, Golden, CO, USA
Vaishali Khokhar, Cleveland, OH, USA
Pinit Kidhunthod, Bangkok, Bangkok, Thailand
Chang-Hyun Kim, Ottawa, ON, Canada
Juye Kim, Providence, RI, USA
Kihoon Kim, Lisle, IL, USA
Takao Kitagawa, Minato-ku, Tokyo, Japan
Hiroaki Komoda, Minami-Ku, Saitama, Japan
Shreya Kundu, Leuven, Flemish Brabant, Belgium
Agnieszka Kurek, Bristol, Somerset, UK
Akiyoshi Kuzume, Kofu, Yamanashi, Japan
LJonathan Larson, Waco, TX, USA
Thi Thu Le, Geesthacht, SH, Germany
Chaewon Lee, Ulsan, South Gyeongsang, ROK
Doohwan Lee, Seoul, Gyeonggi-do, ROK
Jun Yeob Lee, Suwon, Gyeonggi-do, ROK
Sang-Joon Lee, San Jose, CA, USA
Sunyoup Lee, Daejeon, South Chungcheong, ROK
Woongkyu Lee, Seoul, Gyeonggi-do, ROK
Marina Leite, Davis, CA, USA
Bin Li, Knoxville, TN, USA
Chastity Li, Menlo Park, CA, USA
(continued on next page)
(continued from previous page)
Defu Li, Los Angeles, CA, USA
Jiaqi Li, El Cerrito, CA, USA
Wanwisa Limphirat, Muang, Nakhon Ratchasima, Thailand
Jeng-Yu Lin, Taichung City, Taichung County, Taiwan
Chong Liu, Chicago, IL, USA
Jennifer Lu, Merced, CA, USA
Mei-Chien Lu, Saratoga, CA, USA
Alicia Lund, San Leandro, CA, USA
M
Shengcun Ma, Fremont, CA, USA
Carine Maaliki, Tours, Centre-Val de Loire, France
Santi Maensiri, Patumwan, Bangkok, Thailand
Sabine Maier, Erlangen, BY, Germany
Anwesha Maitra, Berkeley, CA, USA
Filip Maletic, Zagreb, Croatia, Croatia
Miika Mattinen, Helsinki, Uusimaa, Finland
Alex McGillivary, Santa Fe Springs, CA, USA
Osama Metawea, Ueda, Nagano, Japan
Matthew Metz, Beaverton, OR, USA
Yuzuru Miyazaki, Sendai, Miyagi, Japan
Debananda Mohapatra, Ulsan, Gyeongsangbuk-do, ROK
Jeffrey Monroe, Marlborough, MA, USA
Elisa Moretti, Venice, VN, Italy
NHayate Nakatsuji, Haga, Tochigi, Japan
SungWoo Nam, Irvine, CA, USA
Supawadee Namuangruk, Klong Luang, Pathum Thani, Thailand
Jorg Neugebauer, Düsseldorf, NRW, Germany
Fuma Nezaki, Inuyamashi Maehara, Aichi, Japan
Aleksandr Noy, Livermore, CA, USA
Khanin Nueangnoraj, Khlong Luang, Central Thailand, Thailand
OMyoung Hwan Oh, Naju, South Jeolla Province, ROK
Kazuki Okaya, Hiratsuka, Kanagawa, Japan
Manunya Okhawilai, Bangkok, Bangkok, Thailand
Gary Ong, Chicago, IL, USA
PMaarja Paalo, Tartu, Tartumaa, Estonia
Gregory Pach, Thornton, CO, USA
Heemin Park, Troy, NY, USA
Sangho Park, Jeonju-si, Jeollabuk-do, ROK
MariaPia Pedeferri, Milan, LM, Italy
Chatwarin Poochai, Patumwan, Bangkok, Thailand
Ilja Popovs, Knoxville, TN, USA
Chanon Pornrungroj, Bangkok, Bangkok, Thailand
NEW MEMBERS NEW MEMBERS
Thomas Porter, Alameda, CA, USA
Deborah Prezzi, Modena, EM, Italy
QJiaqian Qin, Bangkok, Bangkok, Thailand
Deyang Qu, Milwaukee, WI, USA
RSinu Rajappan, Kgs. Lyngby, Capital Region, Denmark
Farshid Ramezanipour, Louisville, KY, USA
Clarita Regalado, Lincoln, RI, USA
Bernardine Rinkel, Berkeley, CA, USA
Sebastian Risse, Berlin, BE, Germany
SRyota Sakamoto, Miyagi, Miyagi, Japan
Martial Santorelli, Grenoble, AuvergneRhone-Alpes, France
Nathan Schattke, Columbia, MO, USA
Makoto Sekine, Nagoya, Aichi, Japan
Hyung Kee Seo, Jeonju, Jeollabuk-do, ROK
Takumi Shibusawa, Mountain View, CA, USA
Alexander Shluger, Middlesex, London, UK
Andrej Singer, Ithaca, NY, USA
Neal Sleszynski, Lebanon, OR, USA
Biljana Sljukic, Lisboa, Lisbon, Portugal
Anongnat Somwangthanaroj, Patumwan, Bangkok, Thailand
Hyun-Kon Song, Ulsan, Gyeongsangnam-do, ROK
Yuefeng Song, Dalian, Liaoning, China
Chakrit Sriprachuabwong, Patumwan, Bangkok, Thailand
Ash Stott, Ash Vale, Surrey, UK
Shizhao Su, Marina, CA, USA
Manaswee Suttipong, Pathumwan, Bangkok, Thailand
T
Amir Taghavi Nasrabadi, Austin, TX, USA
Giulia Tagliabue, Lausanne, Vaud, Switzerland
Kazunao Takahashi, Yokohama, Kanagawa, Japan
Zhihong Tang, Indianapolis, IN, USA
Nisit Tantavichet, Pathumwan, Bangkok, Thailand
Amir Taqieddin, Fremont, CA, USA
Seiji Terada, Kyotanabe, Kyoto, Japan
Patchanita Thamyongkit, Bangkok, Bangkok, Thailand
Seamus Thomson, Mountain View, CA, USA
Brian Tieskoetter, Sunnyvale, CA, USA
Mira Todorova, Düsseldorf, NRW, Germany
Robert Triebl, Graz, Styria, Austria
Manisha Tripathy, San Jose, CA, USA
John Trouba, Elkton, MD, USA
Adisorn Tuantranont, Pathumthani, Pathum Thani, Thailand
UMasanobu Uchimura, Santa Clara, CA, USA
VVeenasri Vallem, San Leandro, CA, USA
Subramanian Venkatachalam, Kings Mountain, NC, USA
Vineetha Vinayakumar, Duisburg, NRW, Germany
Alberto Vomiero, Lulea, Norrbotten, Sweden
Lars Voss, Livermore, CA, USA
W
Jenna Walrath, North Plains, OR, USA
Marc Walter, Mannheim, BW, Germany
Chih-Chieh Wang, Kaohsiung City, Kaohsiung, Taiwan
Suttipong Wannapaiboon, Patumwan, Bangkok, Thailand
Stephan Warnat, Bozeman, MT, USA
Matthew Weimer, Denver, CO, USA
Michelle Wiebenga, Warren, MI, USA
Julia Wind, Kjeller, Akershus, Norway
Judy Wu, Lawrence, KS, USA
Tzu-Ho Wu, Douliou, Yunlin County, Taiwan
X
Guangrui Xia, Vancouver, BC, Canada
Tao Xu, Changsha, Hunan, China
Y
Changcheng Yang, Kaohsiung City, Kaohsiung, Taiwan
Hui Yang, Sunnyvale, CA, USA
Angel Yanguas-Gil, Lemont, IL, USA
Jianchao Ye, Tracy, CA, USA
Yuki Yoshida, Koshi City, Kumamoto, Japan
Z
Riccardo Zaffaroni, Delft, Zuid Holland, Netherlands
Yuepeng Zhang, Naperville, IL, USA
Ziting Zhu, Richmond, CA, USA
Students
A
Karen Acurio Cerda, Lincoln, NE, USA
Randall Adcock, Tempe, AZ, USA
Mohsen Afshari, Houston, TX, USA
Yogeshwaran Agilan, Boston, MA, USA
Ibrar Alam, Bang Mot, Thungkhru, Bangkok, Thailand
Simon Albers, Münster, NRW, Germany
Haidar Alolaywi, Lexington, KY, USA
Anuththara Alujjage, West Lafayette, IN, USA
Joseah Amai, Huntsville, AL, USA
Seth Anderson, Cookeville, TN, USA
Victor Anyanwu, Saint Louis, MO, USA
Simon Appelhaus, Goslar, NI, Germany
John Archer, Austin, TX, USA
Sierra Astle, Lakewood, CO, USA
BYu Bean Bae, Yong-in, Gyeonggi-do, ROK
Mahla Bakhshi, Grimstad, Vest Agder, Norway
Rosa Barrera Quiroz, Puebla, Puebla, Mexico
Caitlin Benway-Trejo, Austin, TX, USA
Rebecca Beswick, Boulder, CO, USA
Rajkumar Bhaduri, Welwyn Garden City, Hertfordshire, UK
Dev Bhakta, Levelland, TX, USA
Bernard Biney, Gainesville, FL, USA
Richa Bisht, Chennai, TN, India
Diprajit Biswas, Madison, WI, USA
William Black, Halifax, NS, Canada
Casey Bloomquist, Brooklyn, NY, USA
Daniel Bonneau, Montréal, QC, Canada
Seo Boseok, Jeju-si, Jeju Province, ROK
Shahd Boud, Jeonju si, Jeollabuk-do, ROK
Gracie Boyer, Cadet, MO, USA
Alessandro Brega, Ulm, BW, Germany
Matthew Brooks, Neath, Wales, UK
Rowan Brower, Davis, CA, USA
C
Riccardo Candeago, Urbana, IL, USA
Camryn Capek, College Station, TX, USA
Alberta Carella, Modena, EM, Italy
Timothy Carlson, Hayward, CA, USA
Angelica Chacon, El Paso, TX, USA
Sheng-Ming Chang, New Taipei City, New Taipei, Taiwan
Suzatra Chatterjee, El Paso, TX, USA
Akshaya Chemmangat, Notre Dame, IN, USA
Bo Yan Chen, Hsinchu, Hsinchu County, Taiwan
Jiazhe “Loki” Chen, Irvine, CA, USA
Kung-Hsing Chen, Hukou, Hsinchu County, Taiwan
Lin Chen, Taipei, New Taipei, Taiwan
Nathan Chen, Storrs, CT, USA
Chen-Lung Chiu, Kaohsiung, Kaohsiung City, Taiwan
Yen-Shuo Chiu, Zhonghe Dist, New Taipei, Taiwan
Yi Hao Chiu, New Taipei City, New Taipei, Taiwan
Yoon Jae Cho, Incheon, Gyeonggi do, ROK
Hojun Choi, Seoul, Gyeonggi do, ROK
Suin Choi, Chicago, IL, USA
Pin-Yu Chou, Hsinchu City, Hsinchu County, Taiwan
Rohit Choudhury, Kandi, TG, India
Ching-Hsiu Chung, Urbana, IL, USA
Courtney Clark, West Lafayette, IN, USA
Brian Cole, Raleigh, NC, USA
Daniel Collins, Frisco, TX, USA
Jack Corbin, Falmouth, England, UK
Luis Corona-Elizarraras, Reno, NV, USA
Allison Crow, Golden, CO, USA
Joshua Cruddos, London, Greater London, UK
Megan Cunningham, Tewksbury, MA, USA
Cole Custodio, Mount Vernon, OH, USA
Ruei-Hong Cyu, Hsinchu, Hsinchu County, Taiwan
D
Summer Dalgamouni, Houston, TX, USA
Marilia Dalla Benetta, Celbridge, Leinster, Ireland
Sourav Das, Ames, IA, USA
Israel David, Gainesville, FL, USA
Tanisha Davidson, Fishers, IN, USA
Alizée Debiais, Montréal, QC, Canada
Jonathan Delaney, Ulm, BW, Germany
Aknachew Demeku, Taipei City, Taipei, Taiwan
Suman Devkota, Toledo, OH, USA
Mukhilan Dhasarathaboopathy, Cleveland, OH, USA
Sokhna Dieng, Philadelphia, PA, USA
Tim Doerenkamp, Villigen PSI, AG, Switzerland
Ana Dogan, Gainesville, FL, USA
Ghazaleh Donyapeyma, Oshawa, ON, Canada
Sydnee Dronsfield, Calgary, AB, Canada
Eric Dzramado, Kofu, Yamanashi, Japan
E
Nadia Ebrahimpour Tolouei, Irvine, CA, USA
Youness El Issmaeli, Trois-Rivieres, QC, Canada
Christian Emeodi, Eugene, OR, USA
Dong Yun Eom, Yongin-si, Gyeonggi-do, ROK
Brooke Erickson, Gainesville, FL, USA
FMohammad Fakhimi Bonab, Merced, CA, USA
Jonathan Fakkema, Madison, WI, USA
Roxana Family, Philadelphia, PA, USA
Xiaozong Fan, Nashville, TN, USA
Rosa Favela Robledo, El Paso, TX, USA
Emily Fenner, Boulder, CO, USA
Daniela Ferreira Garcia, Lubbock, TX, USA
Yuval Fishler, Boulder, CO, USA
Richie Fong, Montréal, QC, Canada
Daniel Frank, Ulm, BW, Germany
Matteo Fratarcangeli, Durham, NC, USA
Algene Fryer, Tuscaloosa, AL, USA
Norihiro Fukaya, Nagakute, Aichi, Japan
Ryuichi Fukuda, Los Angeles, CA, USA
GPhilip Gamboa, Montréal, QC, Canada
Akash Ganesan, State College, PA, USA
Oskar Garcia, Pittsburg, CA, USA
Eduardo Garcia Cuateco, Puebla, Puebla, Mexico
Jesús Garcia Zavaleta, Santiago de Queretaro, Queretaro de Arteaga, Mexico
Avi Gargye, Chicago, IL, USA
Ian Garvie, Elk Grove, CA, USA
Jaylin George, Grand Prairie, TX, USA
Tony George, Newcastle, ON, Canada
Tullio Geraci, Phoenix, AZ, USA
Advaita Ghosh, Taichung, Taichung, Taiwan
Bikram Ghosh, South Bend, IN, USA
Leon Glaeser, Ulm, BW, Germany
Maike Gnutzmann, Münster, NRW, Germany
Jie Min Goh, Gainesville, FL, USA
Andrew Goldman, Livingston, NJ, USA
Dafne González, Puebla, Puebla, Mexico
José Dariel González Gallardo, Pedro Escobedo, Queretaro de Arteaga, Mexico
Carina Goetz, Erlangen, BY, Germany
Christophe Guerin, Laval, QC, Canada
Wendi Guo, Aalborg, North Jutland, Denmark
Alejandro Gutierrez-Carballo, Lubbock, TX, USA
HGeu-Rim Ha, Ansan, Gyeonggi-do, ROK
Shae Hagler, Ann Arbor, MI, USA
Emmanuelle Hagopian, London, England, UK
Adane Hailemariam, Taipei, New Taipei, Taiwan
Jiho Han, Cambridge, Cambridgeshire, UK
Seokjun Han, Gainesville, FL, USA
Zongyi Han, Zurich, ZH, Switzerland
Naila Haroon, Saint Louis, MO, USA
Ryan Hayes, Berkeley, CA, USA
Junyi He, The Woodlands, TX, USA
Luis Henriquez, Irvine, CA, USA
Grant Hill, Chicago, IL, USA
Keshani Hiniduma, Storrs, CT, USA
Evan Hodkinson, Dalton, OH, USA
Tucker Holstun, Berkeley, CA, USA
Seung Hee Hong, Seoul, Gyeonggi-do, ROK
Sungwook Hong, Suwon-si, Gyeonggi-do, ROK
Emma Hopkins, La Jolla, CA, USA
Oliver Horner, Vancouver, BC, Canada
Hao-Chen Hsiao, München, BY, Germany
Hsin-Lu Hsiao, Taipei City, New Taipei, Taiwan
Chia-Chi Hsu, Taipei City, New Taipei, Taiwan
Po Ching (Eric) Hsu, Los Angeles, CA, USA
Simeng Hu, Changsha, Hunan, China
Hua-Jing Huang, New Taipei City, New Taipei, Taiwan
Kara Hughes, Montréal, QC, Canada
I
Alejandra Ibarra Espinoza, Hamilton, ON, Canada
(continued on next page)
(continued from previous page)
J
Salma Jadali, Oshawa, ON, Canada
Jo Jae Ho, Seoul, Gyeonggi-do, ROK
Ben Jagger, Oxford, Oxfordshire, UK
Shankar Jaisi, Reno, NV, USA
Jieun Jang, Seoul, Gyeonggi-do, ROK
Jihong Jeong, Ulsan, South Gyeongsang, ROK
Peilu Jiang, Tokyo, Tokyo, Japan
Junning Jiao, Boston, MA, USA
Ladan Jiracek, Gainesville, FL, USA
Park Jisoo, Seoul, Gyeonggi-do, ROK
Meera Jolly, Peterborough, ON, Canada
KYuichi Kajiura, Davis, CA, USA
Derin Kalay, Princeton Junction, NJ, USA
Mihir Kalvakaalva, Pearland, TX, USA
Zahra Kamali Khanghah, Lincoln, NE, USA
Rajesh Kandel, Davis, CA, USA
Sakshi Kansal, Kharagpur, WB, India
Jake Kattelman, Reno, NV, USA
Christian Kellamis, Canton, OH, USA
Maria Kelly, Lakewood, CO, USA
Arshad Khan, Hsinchu, Hsinchu County, Taiwan
Usman Ali Khan, Baltimore, MD, USA
Fadi Khoury, Beirut, Beirut, Lebanon
Changgyun Kim, Daegu, North Gyeongsang, ROK
Chuljung Kim, Ulm, BY, Germany
Daehyun Kim, Hwasung, Gyeonggi-do, ROK
Dongho Kim, Daejeon, Chungcheongnamdo, ROK
Dong-Young Kim, Naju-si, Jeollanam-do, ROK
Hyong June Kim, Pohang, Gyeongsangbukdo, ROK
Junseong Kim, Incheon, Gyeonggi-do, ROK
Kanghyeon Kim, Seoul, Gyeonggi-do, ROK
Woojin Kim, Naju-si, Jeollanam-do, ROK
Yeongin Kim, Gwangju, Gyeonggi Province, ROK
Jackson King, Goshen, IN, USA
Julian Kink, Erlangen, BY, Germany
Leopold Kloyer, Cambridge, Cambridgeshire, UK
Dasol Ko, Incheon, Gyeonggi-do, ROK
Lukas Koenig, Ulm, BW, Germany
Dongjae Kong, Stanford, CA, USA
Fanqi Kong, Oshawa, ON, Canada
Declan Kopper, Palo Alto, CA, USA
Mena-Alexander Kraenbring, Essen, NRW, Germany
Jo Kubota, Philadelphia, PA, USA
Nitish Kumar, Taichung, Taichung, Taiwan
Ankita Kumari, Davis, CA, USA
Tomohiro Kuriyama, Yokohama-shi, Kanagawa, Japan
LAmina Lahrichi, Trois-Rivieres, QC, Canada
Jack Lang, Irvine, CA, USA
Qingguo Le, Kowloon Tong, Kowloon, Hong Kong
Maximilian Lechner, Garching, BY, Germany
Dong-Yeol Lee, Gwangju, Gyeonggi Province, ROK
Geunchang Lee, Incheon, Gyeonggi-do, ROK
HyoJae Lee, Suwon-si, Gyeonggi-do, ROK
Kevin Lee, Davis, CA, USA
Serin Lee, Cambridge, MA, USA
Rebecca Lentz, Ann Arbor, MI, USA
Niklaus Leuenberger, Stanford, CA, USA
Mauricio Leyva Aranzabal, El Paso, TX, USA
Qingdong Li, Guangzhou, Guangdong, China
Chaewon Lim, Irvine, CA, USA
Chih-Yen Lin, Taipei City, Hsinchu County, Taiwan
Ziqing Lin, Durham, NC, USA
Kang Liu, Changshsa, Hunan, China
Ziqi Liu, Evanston, IL, USA
Chia-Hung Lo, Hsinchu, Hsinchu County, Taiwan
Danielle Loftis, Gainesville, FL, USA
Jacob Lombardo, Baltimore, MD, USA
Rodrigo Lopez Baez, Heverlee, Flemish Brabant, Belgium
Nicolas Lorenzo Serrat, Sugar Land, TX, USA
Binita Lubanjar, Reno, NV, USA
Joseph Neil Lucero, Stanford, CA, USA
Sunil Luhar, Carlton, Victoria, Australia
Axel Lundkvist, Stockholm, Uppland and Soedermanland, Sweden
Cheng Lyu, Penryn, England, UK
MRuiyu Ma, Guangzhao City, Guangdong, China
Wenting Ma, Redwood City, CA, USA
Zhefan Ma, Tuscaloosa, AL, USA
Ananya Maddegalla, Raman Gan, Ramat Gan, Israel
Nergis Mahajar, Anaheim, CA, USA
Emily Mahoney, Chicago, IL, USA
Alejandra Maldonado Pérez, Pedro Escobedo, Queretaro de Arteaga, Mexico
Najia Malik, Toronto, ON, Canada
Akshay Manohar V, Tirupati, AP, India
Seyed Mohammad Ali Manzourolajdad, North York, ON, Canada
Nathalie Mapue, Oshawa, ON, Canada
Thomas Marchese, Orland Park, IL, USA
Konstantina Mason, Davis, CA, USA
Aditya Matam, Folsom, CA, USA
Yasser Matos-Peralta, Montréal, QC, Canada
Kenta Matsumoto, Ube, Yamaguchi, Japan
Måns Mattsson, Corvallis, OR, USA
Oshnik Maurya, Mumbai, MH, India
Glen McClea, Christchurch, Canterbury, New Zealand
Elizabeth McDonnell, Bolingbrook, IL, USA
Jory McIntosh, Davis, CA, USA
Nicholas McKalip, Lakewood, CO, USA
Qiusu Miao, Berkeley, CA, USA
Guillermo Mier y Teran, San Francisco, CA, USA
Antony Elvin Fernando Milton, Austin, TX, USA
Kyeongseok Min, Incheon, Gyeonggi-do, ROK
Jeong Minseok, Gwangmyeong-si, Gyeonggi-do, ROK
Shunosuke Momose, Higasikurumesi, Tokyo, Japan
Ana Montiel, Puebla, Puebla, Mexico
Jaron Moon, Tooele, UT, USA
Seongbak Moon, Raleigh, NC, USA
Edward Mu, Stanford, CA, USA
Manish Mukherjee, South Bend, IN, USA
Konrad Münch, Giessen, HE, Germany
Andrew Murphy, Manhattan, IL, USA
Vincenzo Musico, Cary, NC, USA
NSeunggyu Na, Seoul, Gyeonggi-do, ROK
Ashkan Nahvibayani, Ulm, BW, Germany
Zabihollah Najafianashrafi, Merced, CA, USA
Mahadeen Nashiru, Chicago, IL, USA
Soichiro Natori, Nirasaki, Yamanashi, Japan
Ida Nawrocka, Penryn, England, UK
Carter Ness, Eugene, OR, USA
Ashley Tu Anh Nguyen, Los Angeles, CA, USA
Yan Nie, Kensington, New South Wales, Australia
Hasan Nikkhah, Mansfield Center, CT, USA
Danika Nimlos, Pasadena, CA, USA
Pranay Ninawe, Davis, CA, USA
Hwiyoon Noh, West Lafayette, IN, USA
O
Neidy Ocuane, El Paso, TX, USA
Charles Otieno Ogolla, Siegen, NRW, Germany
Kyung Ho Oh, Incheon, Gyeonggi-do, ROK
Tomohisa Ohmoto, Omihachiman, Shiga, Japan
Victor Okoro, Kingston, ON, Canada
Abayomi Omoogun, El Paso, TX, USA
Philip Onffroy, Stanford, CA, USA
Niklas Oppel, Karlsruhe, BW, Germany
Safeer Rahman Ottakath Cholakkal, Coventry, England, UK
Dilara Ozdemir, Pullman, WA, USA
P
Manisha Pandey, Kanpur, UP, India
Soutam Panja, Ulm, BW, Germany
ChangHyun Park, Daejeon, South Chungcheong, ROK
Jaehee Park, Chicago, IL, USA
Ki Hyun Park, Daejeon, South Chungcheong, ROK
Chirag Patel, Greater London, Greater London, UK
Sairaj Patil, Los Angeles, CA, USA
Ruma Paul, El Paso, TX, USA
Kanchan Pawar, Mumbai, MH, India
Ethan Perkins, Vernon, CT, USA
Tristan Perodeau, Montréal, QC, Canada
Sam Peterson, Elkhorn, NE, USA
Saurabh Pethe, Oak Ridge, TN, USA
Jason Pfeilsticker, Boulder, CO, USA
Timothy Pickarski, Berkeley, CA, USA
Zixin Ping, Stanford, CA, USA
Caroline Pliego, Puebla, Puebla, Mexico
Aamani Ponnekanti, New York, NY, USA
Pitambar Poudel, Christchurch, Canterbury, New Zealand
Scott Prins, Montréal, QC, Canada
RSebastian Raab, Karlsruhe, BW, Germany
Vikalp Raj, Austin, TX, USA
José Ramos Villaseñor, Tlalnepantla, Estado de Mexico, Mexico
Nikhil Rao, Bengaluru, KA, India
Arjun Rego, Etobicoke, ON, Canada
Andrew Reinhard, Columbia, MO, USA
Qiu Ren, Santa Cruz, CA, USA
Arnaldo Rendon, State College, PA, USA
Raul Reyes, Madrid, MAD, Spain
Hannah Richards, White House, TN, USA
Rashid Ahmed Rifat, Ixelles, Brussels, Belgium
Kingston Robinson, Davis, CA, USA
Jose Rochin, State College, PA, USA
Sameer Rodrigues, Leuven, Leuven, Belgium
Lukas Roessler Escudero, Graz, Styria, Austria
Mirjam Rogler, Erlangen, BY, Germany
Noah Rondou, Leuven, Flemish Brabant, Belgium
Alexandra Rousseau, Vancouver, BC, Canada
Alexia Rozman, Tolland, CT, USA
Cindy Rusly, Taipei City, New Taipei, Taiwan
S
Jyothilakshmi S, Tirupati, AP, India
Marjan Saeidi, Oshawa, ON, Canada
Kundan Saha, Medford, MA, USA
Kavish Saini, El Paso, TX, USA
Ferdows Sajedi, Durham, NH, USA
Ali Amir Saleh, Heverlee, Vlaams Brabant, Belgium
Shadi Salehian, Merced, CA, USA
Yash Samantaray, Cambridge, MA, USA
Lars Sanderbrandes, Clausthal-Zellerfeld, NI, Germany
Kenzie Sanroman Gutierrez, Burlingame, CA, USA
Thomas Selmi, Reno, NV, USA
Angela Selva Ochoa, Vancouver, BC, Canada
Haemin Seong, Incheon, Gyeonggi-do, ROK
Yadul Sethi, Mumbai, MH, India
Wenhao Shao, West Lafayette, IN, USA
Atul Sharma, Medford, MA, USA
Raj Shekhar, Eugene, OR, USA
Advay Shirwalkar, Pittsburgh, PA, USA
Varun Shreyas, Louisville, KY, USA
Pui Hang Shum, Glasgow, Scotland, UK
Shweta Shweta, San Juan, PR, USA
Rachel Siegel, Davis, CA, USA
Julia Simon, New Haven, CT, USA
Elena Sjoblom, Santee, CA, USA
Gulam Smdani, Rapid City, SD, USA
Collin Smith, Eugene, OR, USA
Kayla Smith, Macungie, PA, USA
Conner Soderstedt, Durham, NC, USA
Yujin Son, Incheon, Gyeonggi-do, ROK
Jae Hyeok Song, Yongin-si, Gyeonggi-do, ROK
Charles Soulen, La Jolla, CA, USA
Jan Speulmanns, Dresden, SN, Germany
Riley Stephenson, Mebane, NC, USA
Ian Street, Columbia, SC, USA
Errol John Suarez, Legazpi City, Albay, Philippines
Isha Sura, Evanston, IL, USA
Hasika Suresh, Medford, MA, USA
Sourav Sutradhar, Lincoln, NE, USA
Alana Sweeney, Lakewood, CO, USA
TGbenga Taiwo, Newark, DE, USA
Toshiyuki Takimoto, Sendai, Miyagi, Japan
Niladri Talukder, Harrison, NJ, USA
Xiting Tang, Changsha, Hunan, China
Wuttichai Tanmathusorachai, Taipei City, New Taipei, Taiwan
Shikha Thapa, Chennai, TN, India
David Thompson, Fayetteville, AR, USA
Michael Thuis, Evanston, IL, USA
Campbell Tiffin, Christchurch, Canterbury, New Zealand
Surya P Timilsina, Montréal, QC, Canada
Carlo Tomuschat, München, BY, Germany
Isabella Trevino, Tuscaloosa, AL, USA
Cheng-Feng Tsai, Taichung, Taichung City, Taiwan
Ming-Hao Tsai, Hsinchu City, Hsinchu County, Taiwan
Matthew Tudball, London, Greater London, UK
UKovuri Umadevi, Nizamabad, TG, India
Gozde Ustuner, Patchogue, NY, USA
VOscar Valle-Reyes, México City, Distrito Federal, Mexico
Geetha Valurouthu, Philadelphia, PA, USA
Matthew Vasil, Cleveland, OH, USA
Abril Veliz Camayo, München, BY, Germany
Anu Verma, Kharagpur, WB, India
Yogapriya Vetriselvam, Muthupet, TN, India
Guilherme Vieira da Motta Missaka, Chicago, IL, USA
Simon Vigil, Durham, NC, USA
Shiljashree Vijay, Coralville, IA, USA
Tony Vuu, Montréal, QC, Canada
WSuguru Wada, Kofu, Yamanashi, Japan
Zahid Wajdan, Potsdam, NY, USA
Bingning Wang, Darien, IL, USA
Bo Wang, Changsha Shi, Hunan, China
Meijing Wang, Montréal, QC, Canada
Mengli Wang, Wuhan, Hubei Province, China
Yuanshen Wang, Glasgow, Scotland, UK
Zhikui Wang, Gainesville, FL, USA
Yu-Ting Wei, Miaoli City, Miaoli County, Taiwan
Timo Werner, Ulm, BW, Germany
Greg Wettlaufer, Tigard, OR, USA
Megan Whisonant, Columbia, SC, USA
Wen Yu Wu, Vancouver, BC, Canada
Yifan Wu, Berkeley, CA, USA
X
Xinzhe Xue, Santa Cruz, CA, USA
Y
Gangbin Yan, Chicago, IL, USA
Ziyan Yang, Eugene, OR, USA
Tatsuki Yasuda, Kofu, Yamanashi, Japan
Wenshuai Ye, Beijing, Beijing, China
Jenna Ynzunza, Livermore, CA, USA
Kentaro Yoneda, Yokohama Hiyoshi, Kanagawa, Japan
Donghyun Yoon, Gwangju, Gyeonggi-do, ROK
Subhin Yoon, Ulsan, South Gyeongsang, ROK
Youngbin Yoon, Ulsan, South Gyeongsang, ROK
Xintong Yuan, Los Angeles, CA, USA
Z
Yi-Syuan Zeng, Kaohsiung City, Kaohsiung, Taiwan
Haozhe Zhang, Naperville, IL, USA
Penghao Zhang, St. Louis, MO, USA
Sipei Zhang, Urbana, IL, USA
Yixuan Zhang, Montréal, QC, Canada
Yang Zhao, Eugene, OR, USA
Runtian Zheng, Namur, Namur, Belgium
Linan Zhu, Moscow, ID, USA
Michelle Zorigt, Urbana, IL, USA
Siqi Zou, Chicago, IL, USA
Huiyan Zuo, Wuhan, Hubei, China
(continued on next page)
(continued from previous page)
New Members by Country
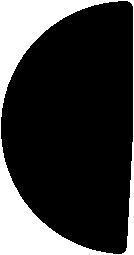
Look who joined ECS in the Second Quarter of 2024
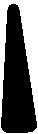
AUSTRALIA . 2
3
8
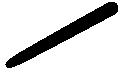
1
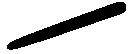
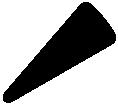
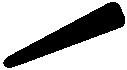
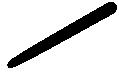
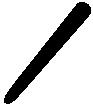
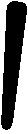
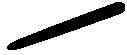
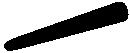
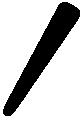
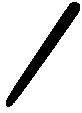
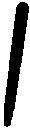
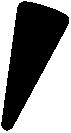
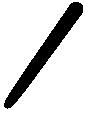
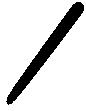
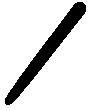
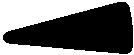
1 CANADA 37 CHINA. 11
8 JAPAN . 36

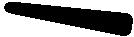
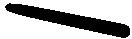
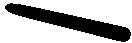
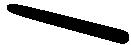
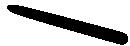
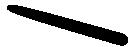
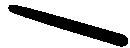
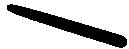
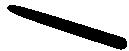
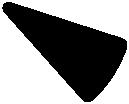
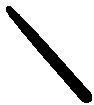
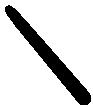
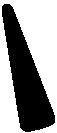
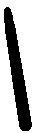
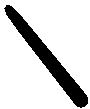

. 3
1
1 MEXICO. 10
2 NEW ZEALAND 3
2
. 1 PHILIPPINES. 1
1 FINLAND 2 FRANCE 5 GERMANY . 35 HONG KONG. 1 HUNGARY . 2 INDIA. 14 IRELAND 1
1 SAUDI ARABIA . 1 SERBIA 1 SOUTH KOREA 55 SPAIN 1 SWEDEN. 3 SWITZERLAND. 3 TAIWAN. 30 THAILAND 22 U A E 1 UK 21
. 320
AUSTRALIA AUSTRIA BELGIUM BRAZIL CANADA CHINA
CROATIA DENMARK ESTONIA FINLAND FRANCE GERMANY
HONG KONG HUNGARY INDIA IRELAND ISRAEL ITALY
JAPAN LEBANON MEXICO NETHERLANDS NEW ZEALAND NORWAY
OMAN PHILIPPINES PORTUGAL SAUDI ARABIA SERBIA SOUTH KOREA
SPAIN SWEDEN SWITZERLAND TAIWAN THAILAND U.A.E. UK USA
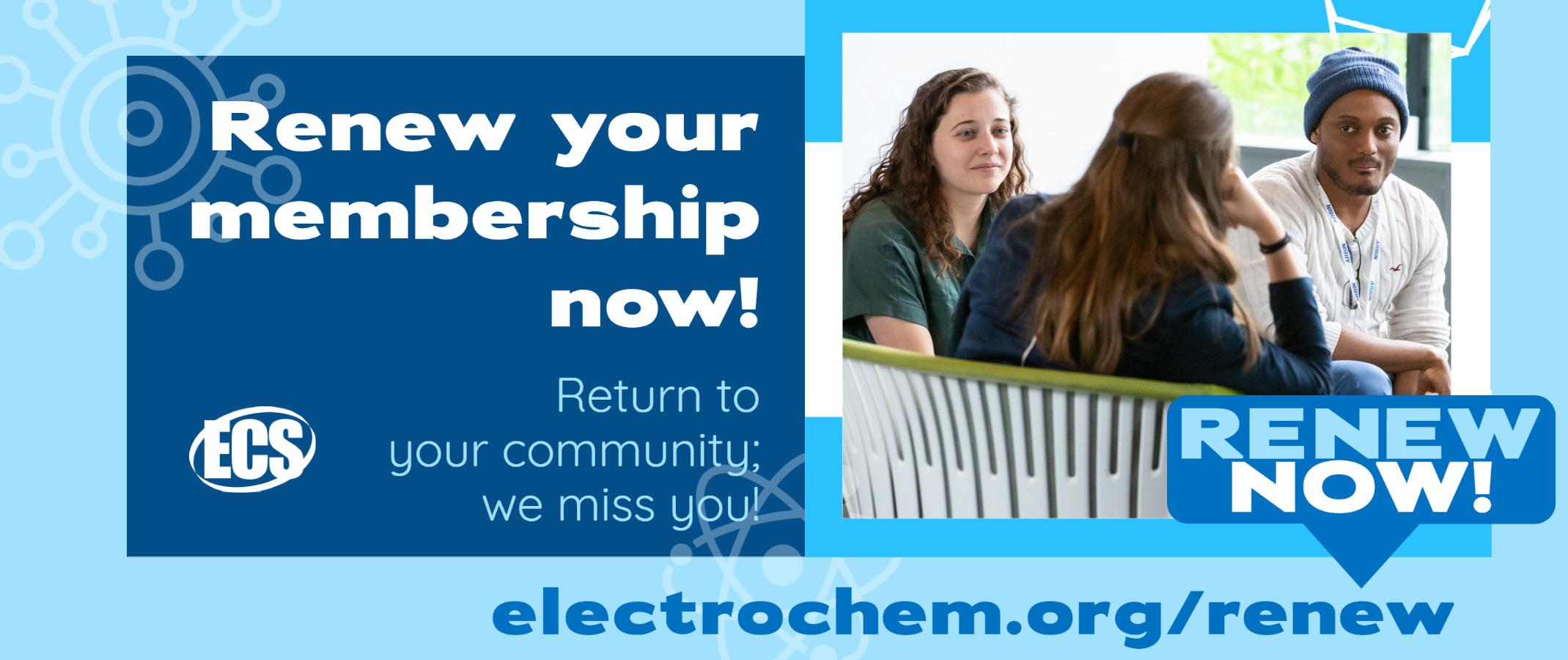
STUDENT
ECS Chartered 11 New Student Chapters in May 2024
Eleven new student chapters in Canada, China, Mexico, Poland, Turkey, the UK, and the US were chartered at the ECS Board of Directors’ meeting on May 30, bringing the total number of chapters around the world to 147!
Please join us in welcoming the new chapters into our supportive, global community.
New Student Chapters
• Benemérita Universidad Autónoma de Puebla, Mexico
NEW
• Ontario Chapter, Canada
• Universidad Autónoma de Querétaro, Mexico
• University of California, Davis, USA
• University of Miami, USA
• Yeditepe Üniversitesi, Turkey
• Central South University of Forestry and Technology, China
• Politechnika Gdańska, Poland
• University College London, UK
• University of Connecticut, USA
• University of Texas at El Paso, USA
Chapter Benefits
ECS Student Chapter membership provides many benefits, including:
• Engaging with students and peers;
• Organizing technical meeting programs and scholarly activities;
• Collaborating with members to present posters at ECS biannual meetings;
• Networking with 8,000+ international ECS members;
• Accessing career resources;
• Adding impressive extracurricular activities to resumes;
• Funding to support chapter activities;
• Partnering with local ECS sections on activities and technical programs;
• Receiving recognition on the ECS website and in Interface
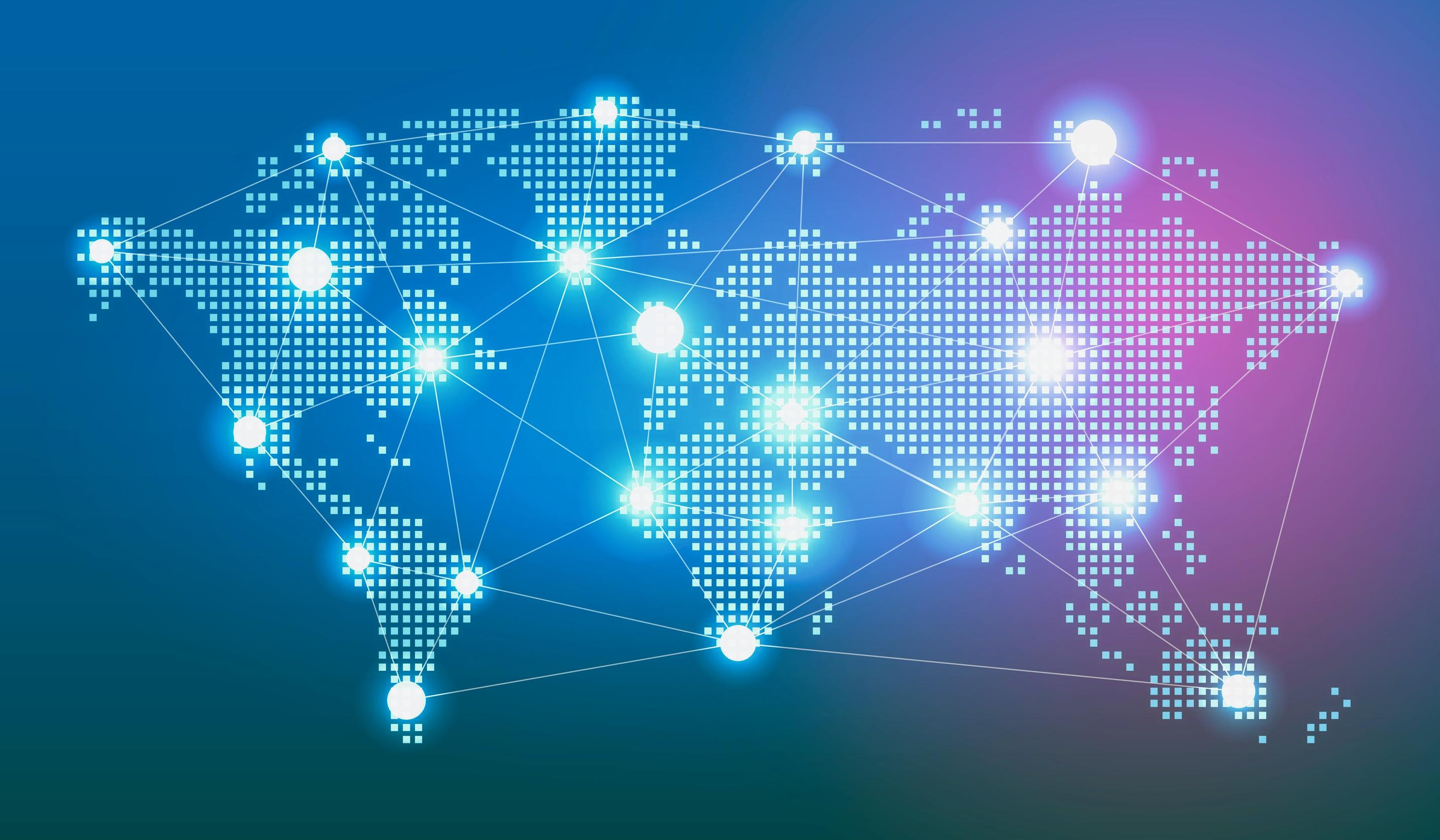
VISIT THE ECS STUDENT CENTER
for more information about student chapters. For the global scope of the Society’s student chapter network, view the Student Chapter Directory
Interested in establishing an ECS Student Chapter at your academic institution?
REVIEW THE GUIDELINES FOR STARTING A CHAPTER AND SUBMIT A NEW STUDENT CHAPTER APPLICATION TODAY!
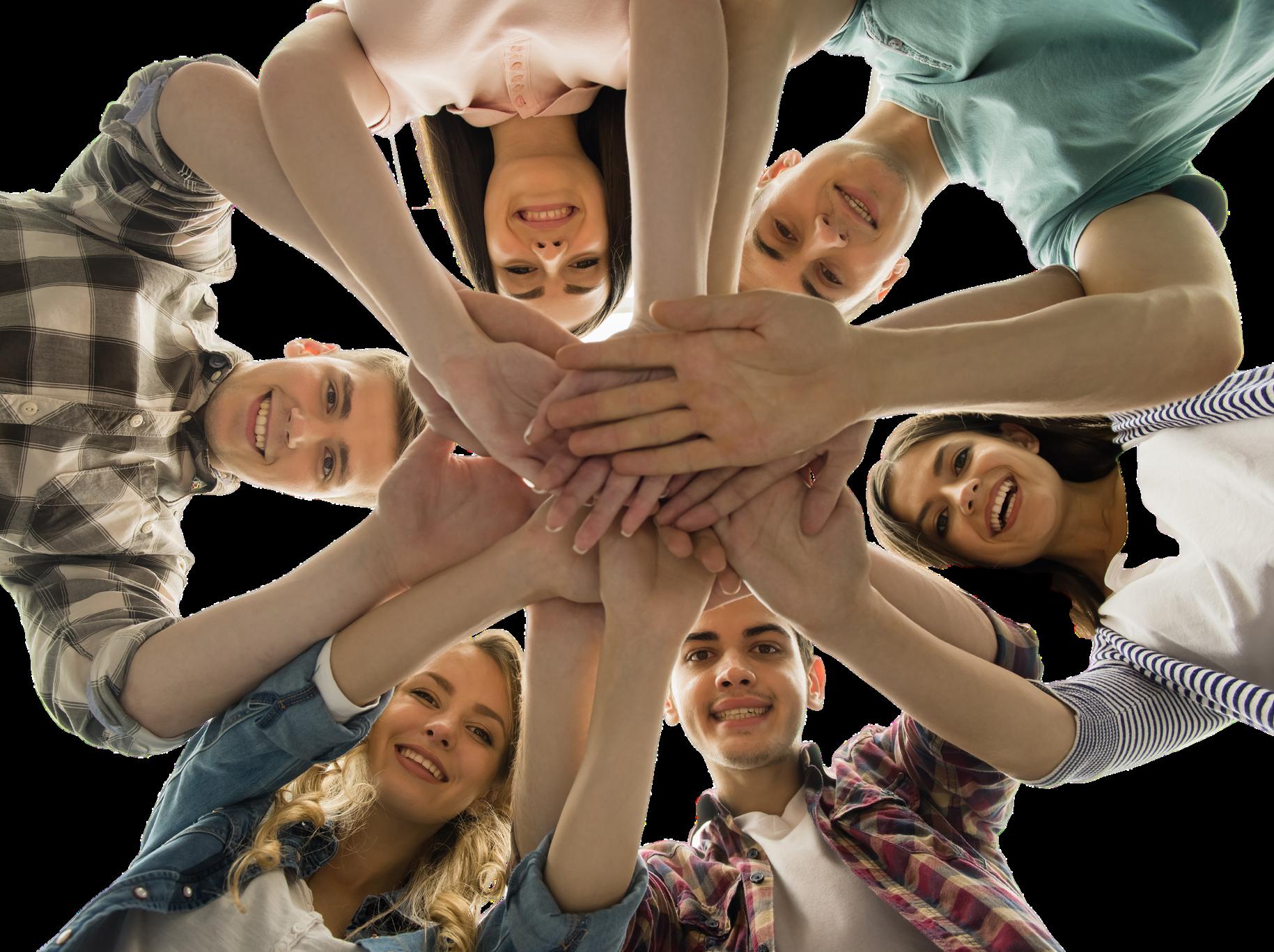
SUBMIT NOW
ECS Case Western Reserve University Student Chapter
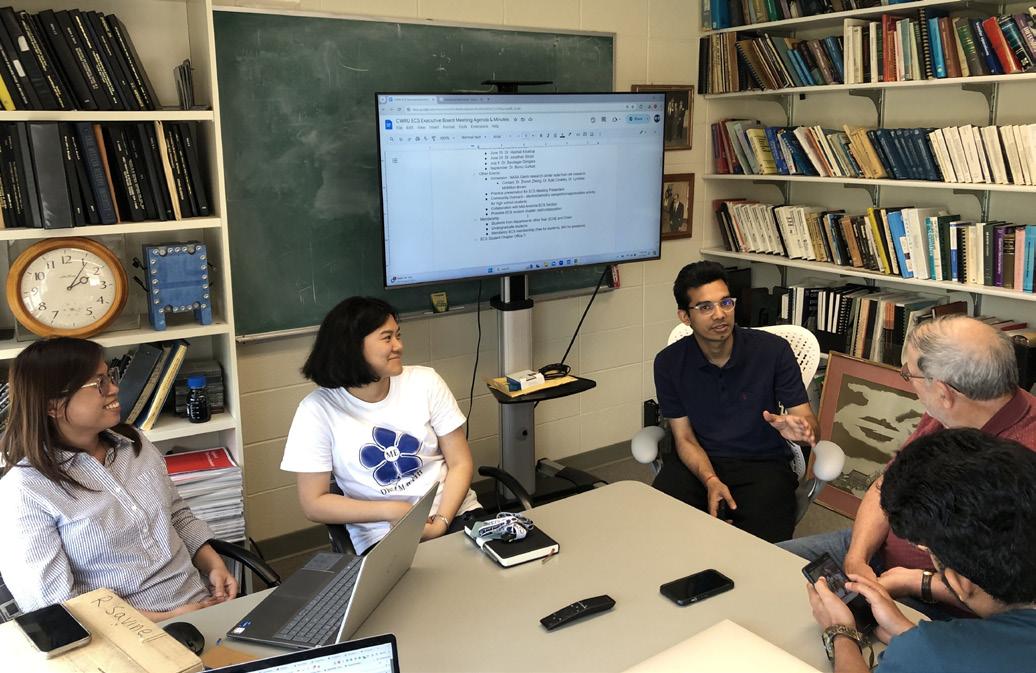
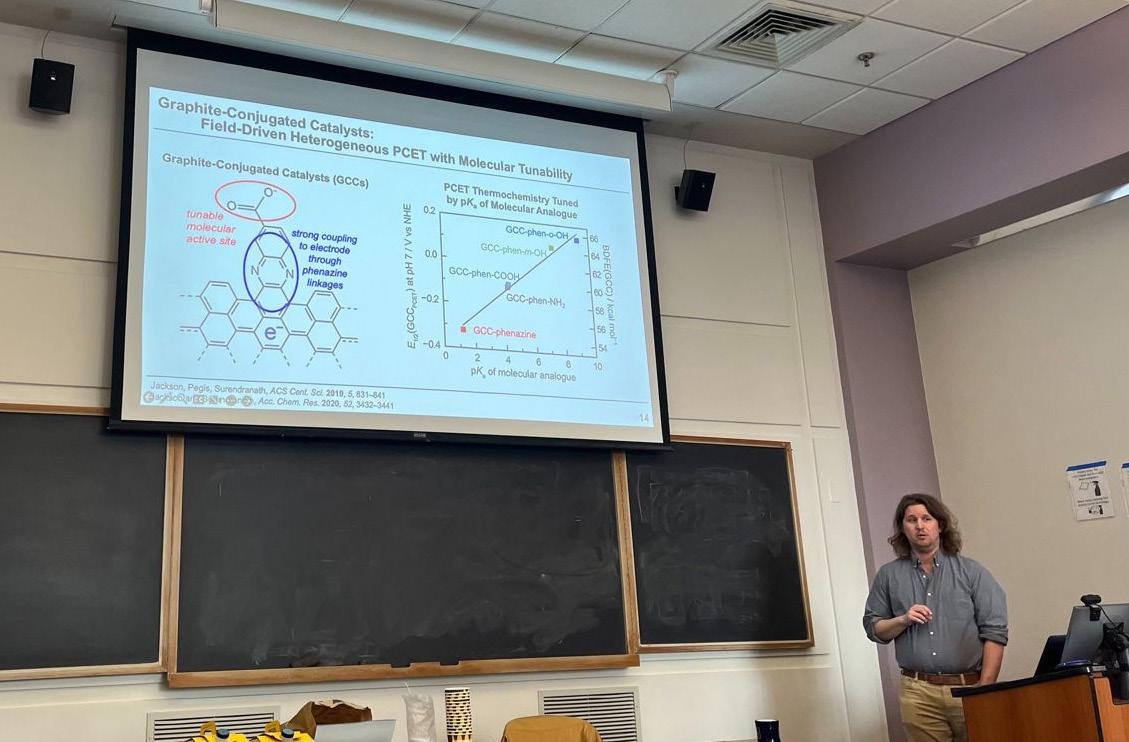
The ECS Case Western Reserve University (CWRU) Student Chapter welcomed its new executive board officers: President Desiree Mae Prado, Vice President Yuanman Ma, Secretary Saurabh Pathak, Treasurer Mukhilan Dhasarathaboopathy, and Social Media Liaison Miguel Muñoz. To begin their term, the officers gathered with their supportive faculty advisor, Prof. Robert Savinell, to discuss and strategize initiatives and events for the next academic year. The agenda included plans for hosting the Electrochemistry Lecture Series, organizing community outreach activities, and fostering collaborations with other ECS sections and student chapters. The chapter aims to engage a diverse membership and to ensure active participation in ECS events and publications.
In April, Assistant Professor Dr. Robert Warburton, Department of Chemical and Biomolecular Engineering, CWRU, discussed his work on electrochemical proton-coupled electron transfer (PCET). He explored how the reactivity of molecules is influenced by an interfacial electric field. Key topics included the fundamentals of PCET in protic electrolytes, which are crucial for energy conversion and storage materials. Theoretical modeling using periodic density functional theory (DFT) was reviewed to understand PCET reactions at solid-liquid and solid-gas interfaces, examining factors
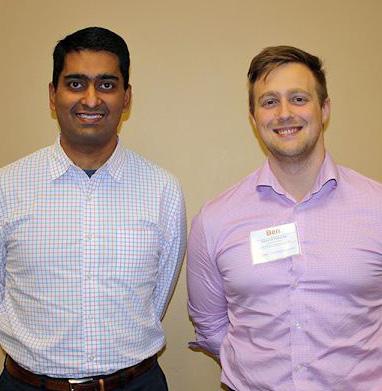
like electrochemical potential, electronic conjugation, and polaron reorganization. He also presented a rate constant model for PCET on transition metal oxides, highlighting factors that determine PCET rate constants and kinetic isotope effects.
Faculty and student chapter members showcased their research at the 245th ECS Meeting in San Francisco. Ben Holcombe presented his work on sustainable rare-earth electrowinning in chloride-based molten salts. Vincent Tam discussed current distribution control in slurry electrolytes for redox flow batteries. Arvind Singh Heer explored electrostatic stimulation of carbon monoxide oxidation on platinum in acidic electrolytes. Theodore Phung presented potentialdependent BDAC adsorption on zinc for selective suppression of zinc corrosion in energy storage. Zeynep Bagbudar modeled interfacial proton-coupled electron transfer reactions with imidazolium proton donors. Chapter members enjoyed networking with other ECS members, making valuable connections.
Invited CWRU faculty members delivered insightful lectures. Prof. Changyong Cao discussed “Triboelectric Nanogenerators for Self-Powered Sensing and Human-Machine Interfaces.” Dr. Robert Warburton presented “Computational Models of ProtonCoupled Electron Transfer in Energy Conversion and Storage,” and
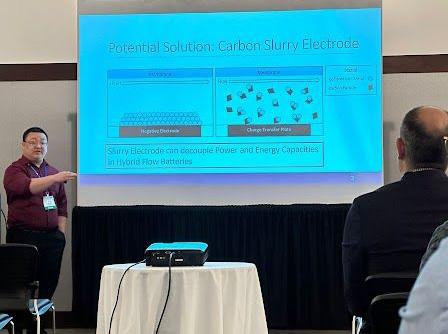
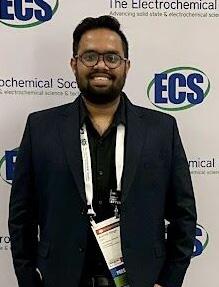
ECS Case Western Reserve University (CWRU) Student Chapter Faculty Advisor Prof. Robert Savinell (second from right) meets with (from left to right): chapter President Desiree Mae Prado, Vice President Yuanman Ma, Secretary Saurabh Pathak, and Treasurer Mukhilan Dhasarathaboopathy
Photo: Miguel Muñoz
From left to right: CWRU faculty and student chapter members Prof. Rohan Akolkar, Ben Holcombe, Vincent Tam, and Arvind Singh Heer presented their research at the 245th ECS Meeting.
Photos: Miguel Muñoz
CRWU Professor Robert Warburton discusses his work on electrochemical proton-coupled electron transfer (PCET).
Photo: Miguel Muñoz
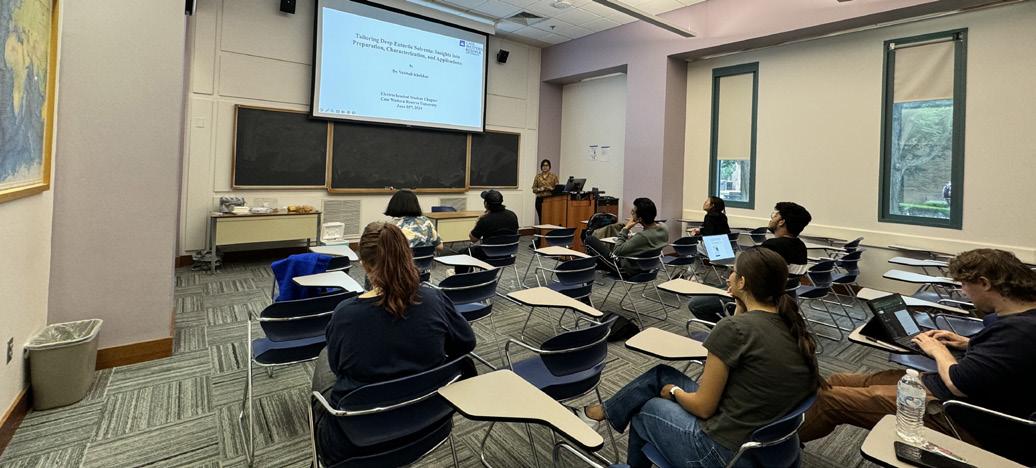
Postdoctoral Researcher Dr. Vaishali Khokhar
her
on deep eutectic solvents for electrochemical energy storage applications at the ECS CWRU Student Chapter summer electrochemistry lecture series.
Prof. Daniel Scherson focused on “Mechanistic Aspects of Selenate Reduction on Cu(UPD) on Au Electrodes.” Prof. Uziel Landau, recipient of the ECS Henry B. Linford Award for Distinguished Teaching, shared his expertise on “Overcoming Challenges in Teaching Electrochemical Engineering.”
The chapter kicked off its summer electrochemistry lecture series last June with a presentation by Dr. Vaishali Khokhar, a postdoctoral researcher under the supervision of Prof. Burcu Gurkan, Department of Chemical and Biomolecular Engineering. Dr. Khokar explained the composition of deep eutectic solvents and emphasized their distinction from conventional solvents, ionic liquids, and binary mixtures. She provided a comprehensive overview of their essential physicochemical properties and the methods used for characterization. Dr. Khokar underscored their wide-ranging applications, such as in electrochemical separations, electrodeposition processes, and the development of electrolytes for energy storage applications.
Chapter members Miguel Muñoz, Desiree Mae Prado, and Dr. Zechariah Pfaffenberger successfully participated in and completed the Electrochemistry Boot Camp organized by Dr. Joaquín Rodríguez-López and his research group at the University of Illinois Urbana-Champaign (UIUC) from June 12 to 14. This intensive course focused on experimental electrochemistry, featuring 18 experiments, 6 lectures, simulations, and final projects. Participants enhanced their expertise through a variety of activities, including constructing reference electrodes to verify the Nernst equation, addressing challenges in voltammetry, understanding pH effects, characterizing electrocatalysts, assembling ultramicroelectrodes, conducting bulk electrolysis, setting up in-situ IR measurements, and mastering feedback and generation/collection SECM techniques. UIUC Professor Dr. Theresa Schoetz delivered a lecture on electrochemical impedance spectroscopy and presented an experiment focusing on this technique.
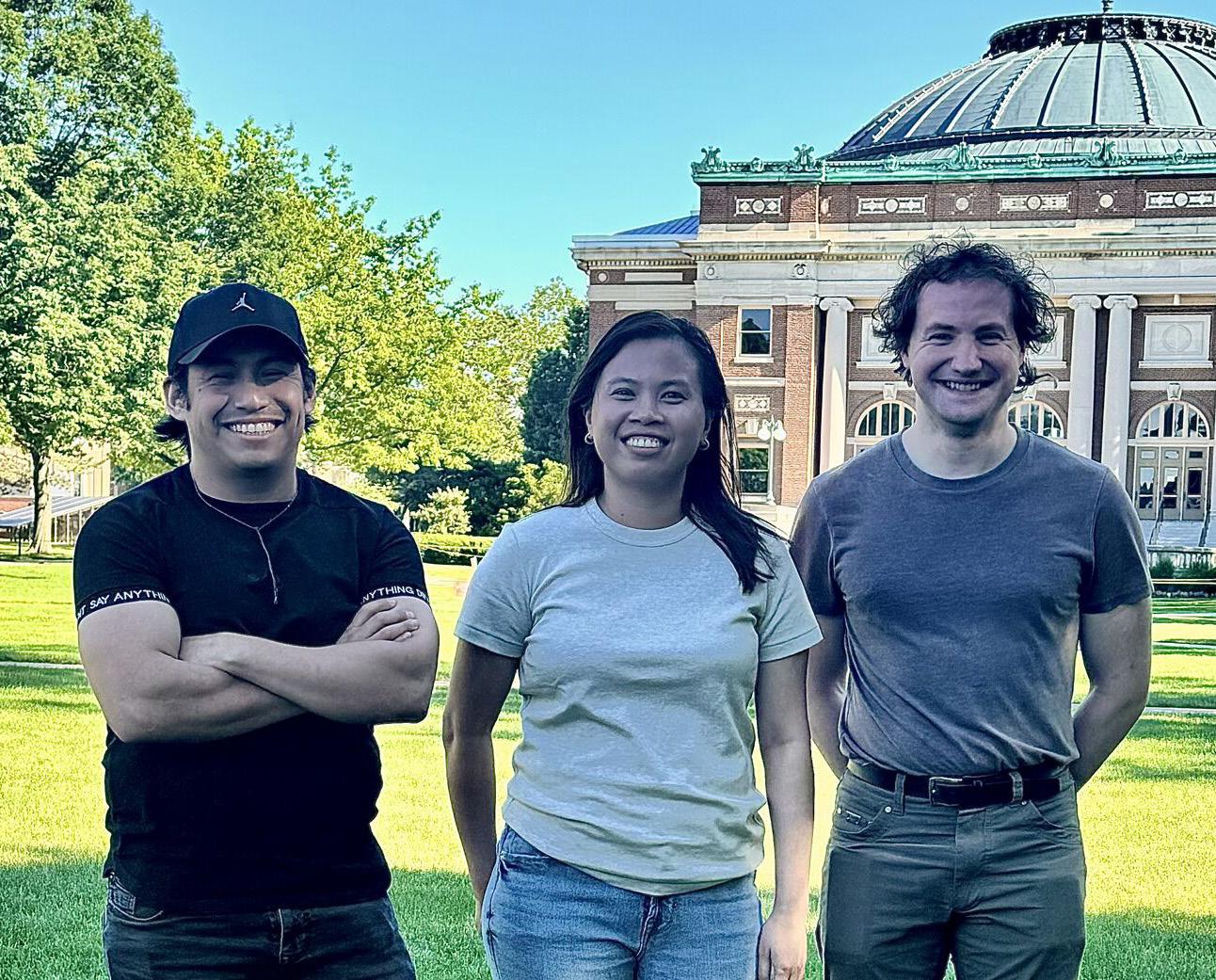
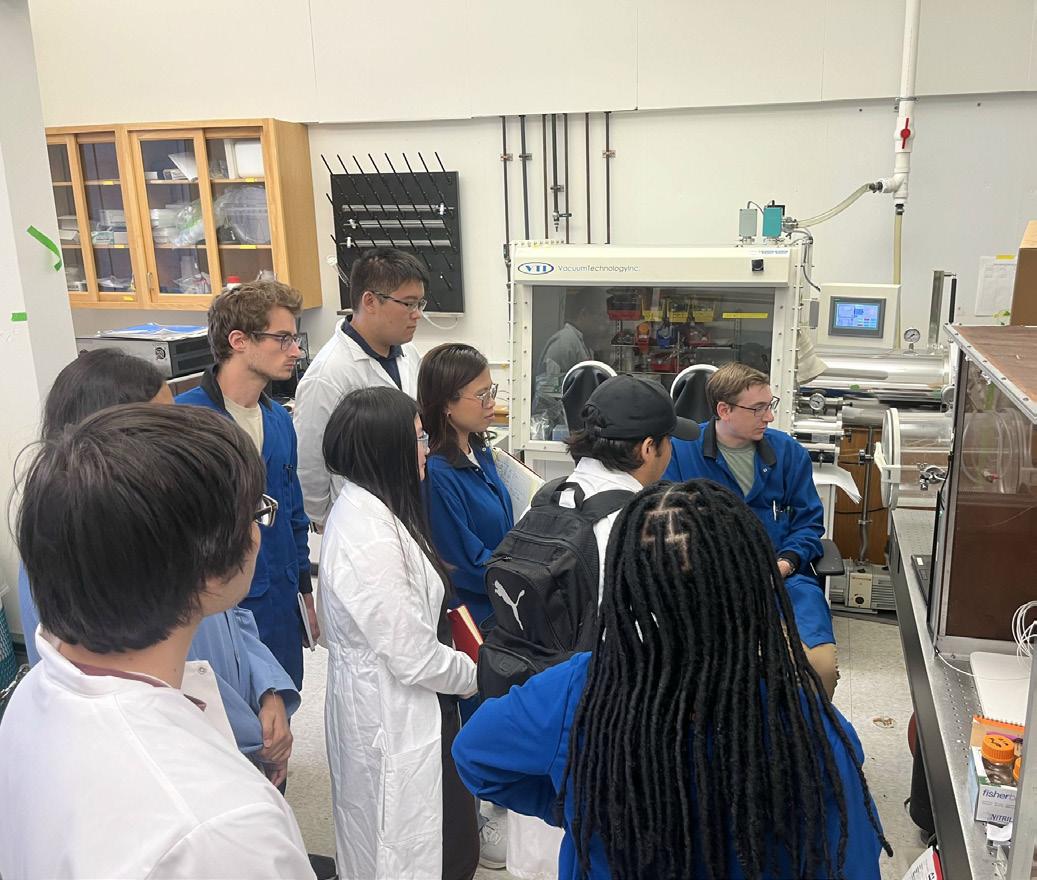
Left to right: CWRU chapter members Miguel Muñoz, Desiree Mae Prado, and Dr. Zechariah Pfaffenberger attend the Electrochemistry Boot Camp at the University of Illinois Urbana-Champaign (UIUC).
presents
research
Photo: Miguel Muñoz
CWRU chapter members observe a hands-on experiment involving a rotating ring disk electrode at the UIUC Electrochemistry Boot Camp Photos: Miguel Muñoz
STUDENT
ECS Gdan , s k University of Technology Student Chapter
The Gdańsk University of Technology student chapter is happy to announce that in June 2024 it became the first ECS student chapter in Poland! The chapter’s officers are Chair Iwona Kaczmarzyk, Vice Chair Klauida Prusik, Secretary Hubert Grabowski, Treasurer Zuzanna Zarach, and Social Media Liaison Daria Roda. The board and members appreciate the support of their Faculty Advisor, Prof. Jacek Ryl. The chapter is an initiative for undergraduate and PhD students interested in electrochemistry, photochemistry, catalysis, and related issues. It aims to expand their knowledge and skills and to conduct fundamental and implementation research on electric charge transfer processes and materials of interest in electrochemistry. In addition, the goal is to develop the students’ academic lives, broaden their interests, and support their creative work.
Chapter members undertook several activities related to their scientific goals. Starting in October 2023, they organized lectures delivered by experts in electrocatalysis, photochemistry, and 3D
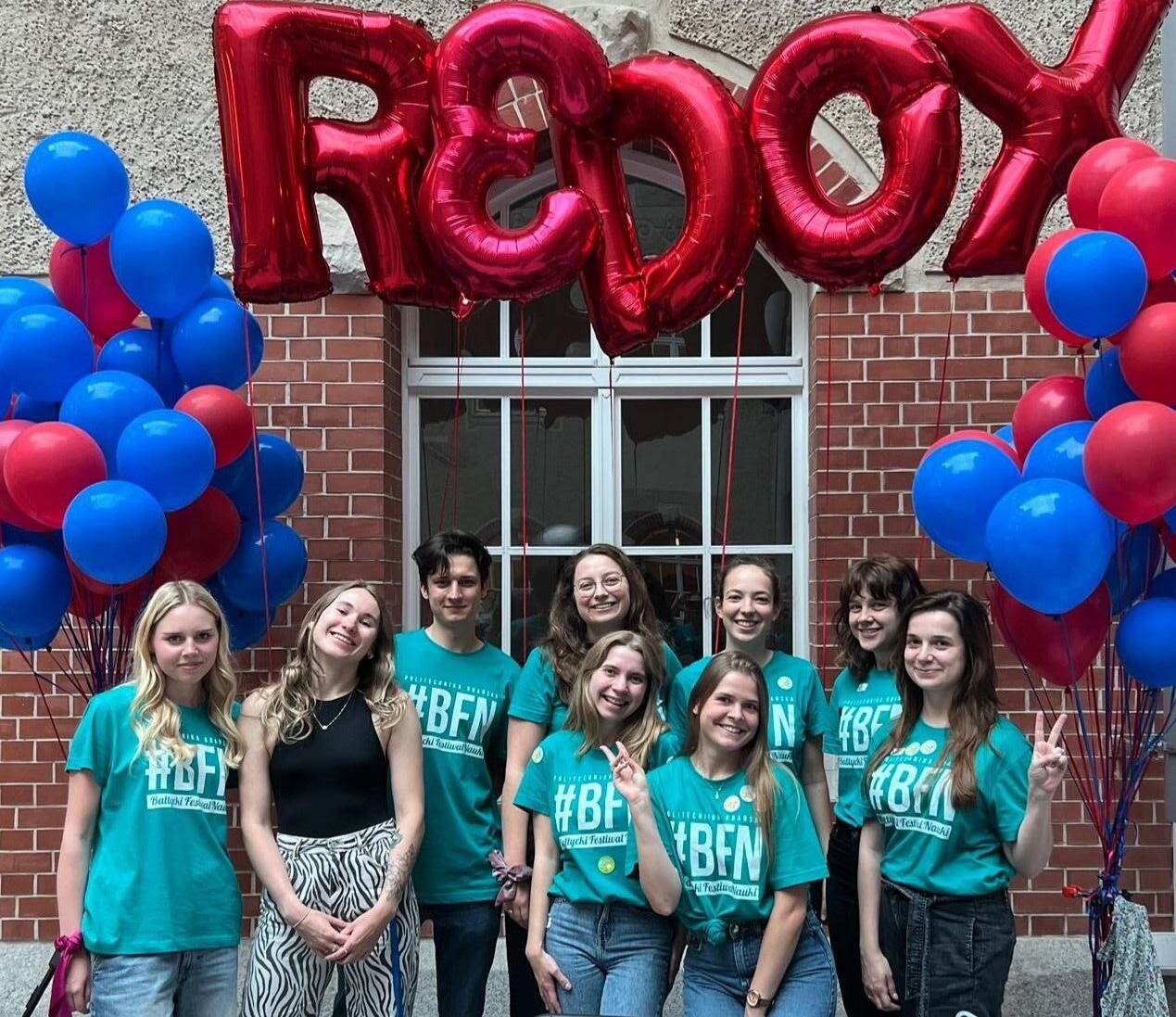
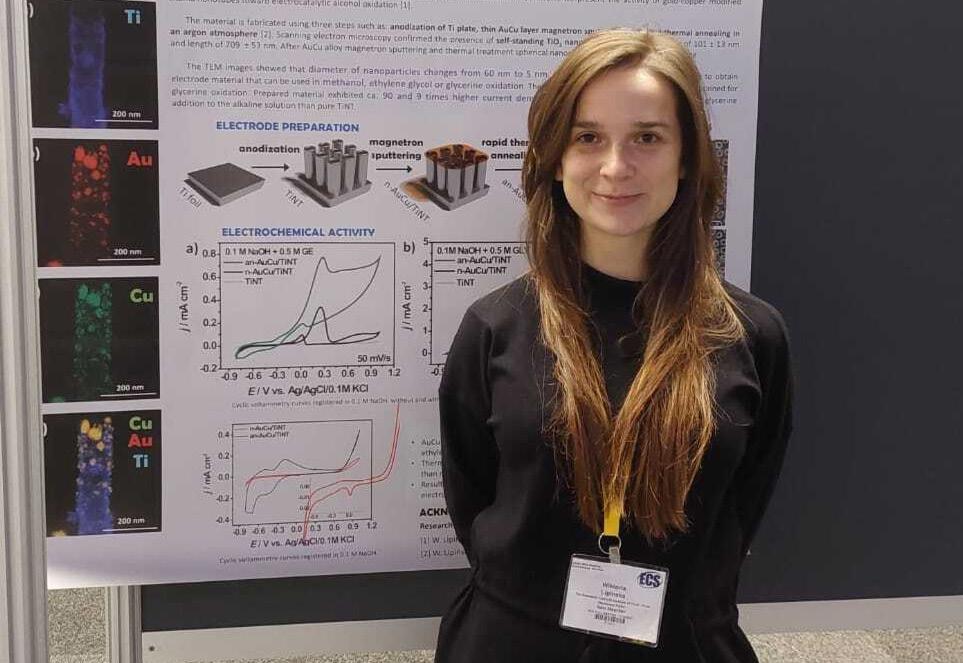
printing, as part of the “Knowledge Transfer” series. View videos of the lectures on our YouTube channel
Members are working on scientific projects using organic waste in electrochemistry and 3D printing to obtain electrode materials. Wiktoria Lipińska and Adrian Olejnik took part in the 244th ECS Meeting in Gothenburg, Sweden. They presented their research on using modified TiO2 nanotubes in alcohol oxidation and the electrochemistry of doped diamond surfaces. Chapter members actively participated in the Baltic Science Festival, Materials Engineering Day, and Forum of Academic Organizations and Chapters. This activity brought them great joy, as they shared their knowledge and passion for science. During Gdańsk Tech’s summer semester, a science project on 3D-printed materials was dedicated specifically to chapter members. The students are currently preparing to present research at scientific conferences. The executive committee plans to continue the “Knowledge Transfer” lecture series and work on scientific projects.
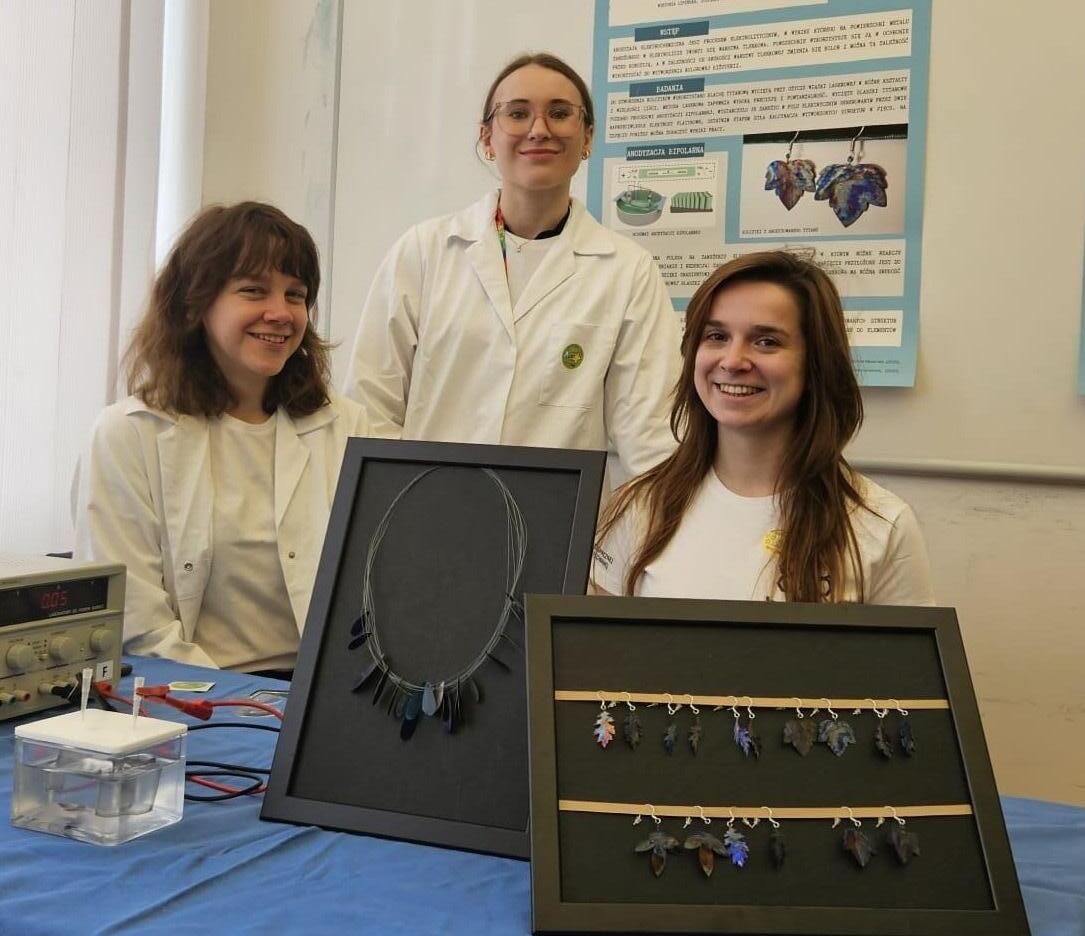
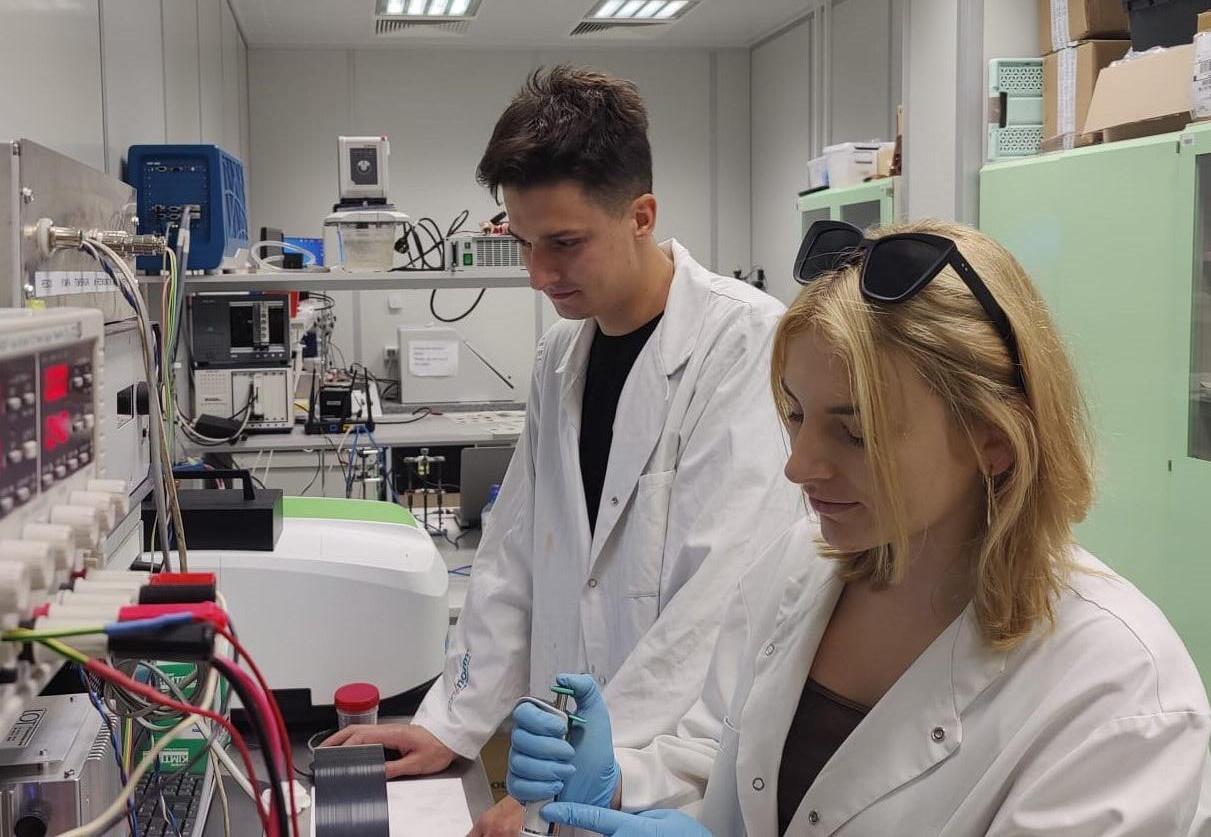
Members of the ECS Gdańsk Tech Student Chapter (also known as the RedOx Scientific Club) gather at the Baltic Science Festival.
Photo: Jacek Ryl
ECS Gdańsk Tech Student Chapter Secretary Hubert Grabowski (left) and member Zofia Jeleniewska (right) perform electrochemical measurements in the chapter ҆s“3D Printing in Electrochemistry” science project.
Photo: Daria Roda
From left to right: ECS Gdańsk Tech Student Chapter officers Stefania Wolff and Iwona Kaczmarzyk and member Wiktoria Lipińska at Materials Engineering Day
Photo: Daria Roda
ECD Gdańsk Tech Student Chapter member Wiktoria Lipińska presents a poster at the 244th ECS Meeting.
Photo: Wiktoria Lipińska
ECS Montréal Student Chapter
The ECS Montréal Student Chapter held a Meet & Greet, its first meeting, on May 22 in the Chemistry Department at McGill University. The goal was to welcome new members, reintroduce the student chapter, and introduce its new executives. Thomas Boulanger and Louis Hamlet promoted the chapter to potential members,
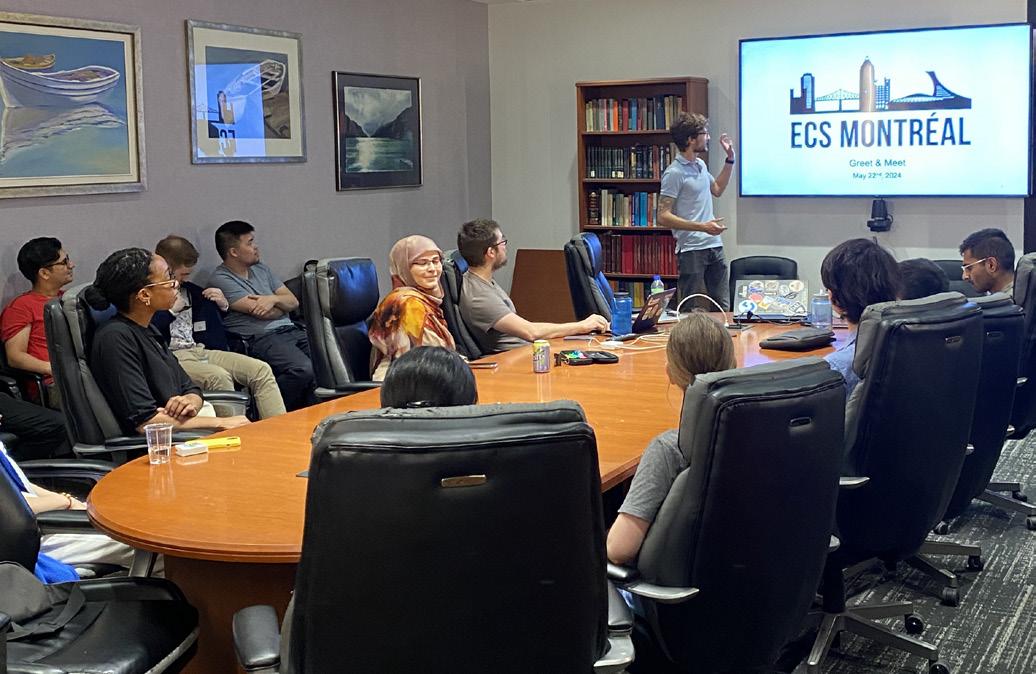
Lila Laundry-Mottiar
explained how to register, and advertised the fall symposium. At the end of the presentation, members and participants were encouraged to introduce themselves, network, and have friendly conversations with their neighbors about their electrochemistry research and create new connections.
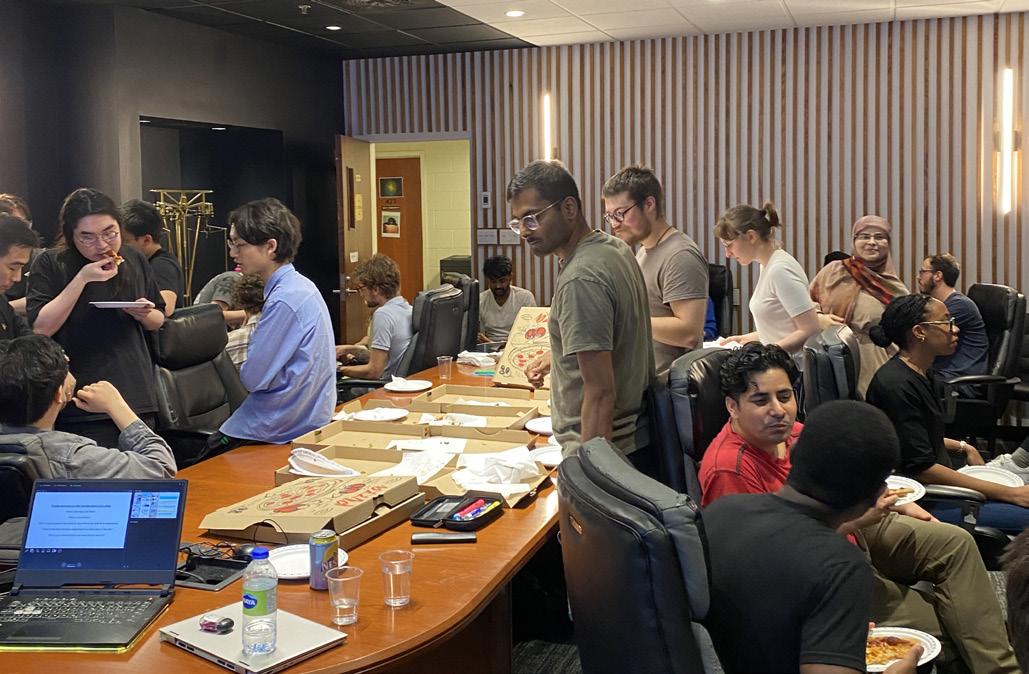
ECS Munich Student Chapter
In June, 25 students and members of the ECS Munich Student Chapter had the opportunity to visit the BMW Cell Manufacturing Competence Center (CMCC) in Parsdorf, Germany. The visit started with Stefan Zangerle, Vice President of Planning, Industrialization, and R&D Battery Cells, BMW, providing an exciting introduction and overview of the company’s strategy, R&D activities, and BEV portfolio. After chapter Secretary Markus Schilling gave a short presentation on the chapter, the group toured the BMW pilot plant for battery production of cylindrical cells. The full cell fabrication process, starting from slurry preparation, coating, and calendaring, to electrode cutting, jelly rolling, and electrolyte filling, was covered. The visit concluded with presentations by Dr. Sophie Solchenbach
and Korbinian Huber detailing the BMW group’s battery research activities. Overall, the visit gave an introduction into what it means to fabricate battery cells on an industrial scale. It was a perfect networking opportunity for chapter members.
The chapter organized an internal university conference titled “Energizing the Future – Battery and Hydrogen Research at TUM (Technische Universität München),” which took place on September 24 at TUM’s Garching campus. The aim of the conference was to generate a platform for enthusiastic electrochemistry and electrocatalysis undergraduate and PhD students to connect and to get an overview of the ongoing battery and hydrogen research and infrastructure at TUM.
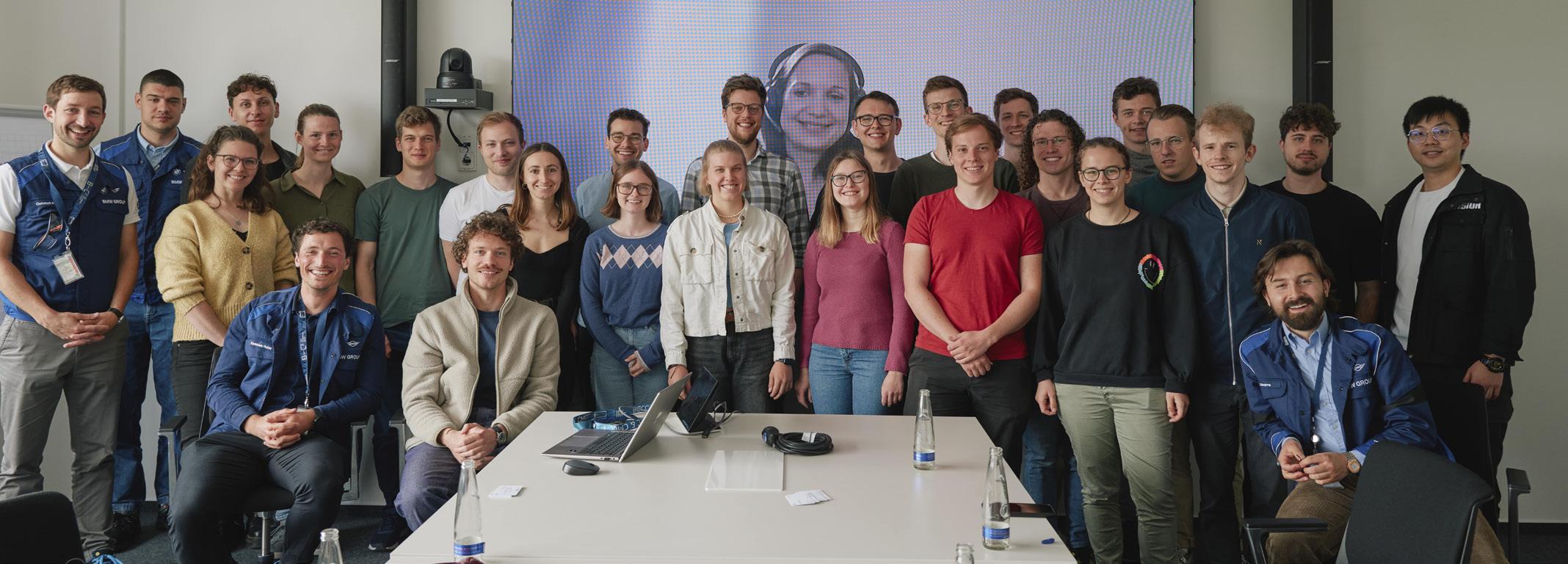
Thomas Boulanger gives an introductory presentation about the ECS Montréal Student Chapter at the Meet & Greet event held at McGill University.
Photo:
ECS Munich Student Chapter members visit the BMW Cell Manufacturing Competence Center (CMCC) in Parsdorf, Germany
Photo: Philip Rapp
New ECS Montréal Student Chapter Members network while enjoying pizza and drinks at the Meet & Greet event held at McGill University.
Photo: Lila Laundry-Mottiar
STUDENT NEWS STUDENT
ECS Ohio University Student Chapter
The ECS Ohio University Student Chapter President Abigail Paul relaunched the chapter in February 2024. With 13 new members, the chapter is off to a great start. New officers were elected on March 5, 2024: President Abigail Paul, Vice President Zhuldyz Zhigulina, Secretary Isabel Baca, and Treasurer Nathan Wichert
The chapter held events during the semester that included a game night and a guest lecture by Dr. Kody Wolfe titled “A Comparison of Methods for Electrochemically Active Surface Area Measurements.” Guest lectures and a fuel cell car outreach event at a local high school take place in the fall term. As the chapter grows, the officers are looking forward to hosting more events and having a significant presence in the community with STEM outreach.
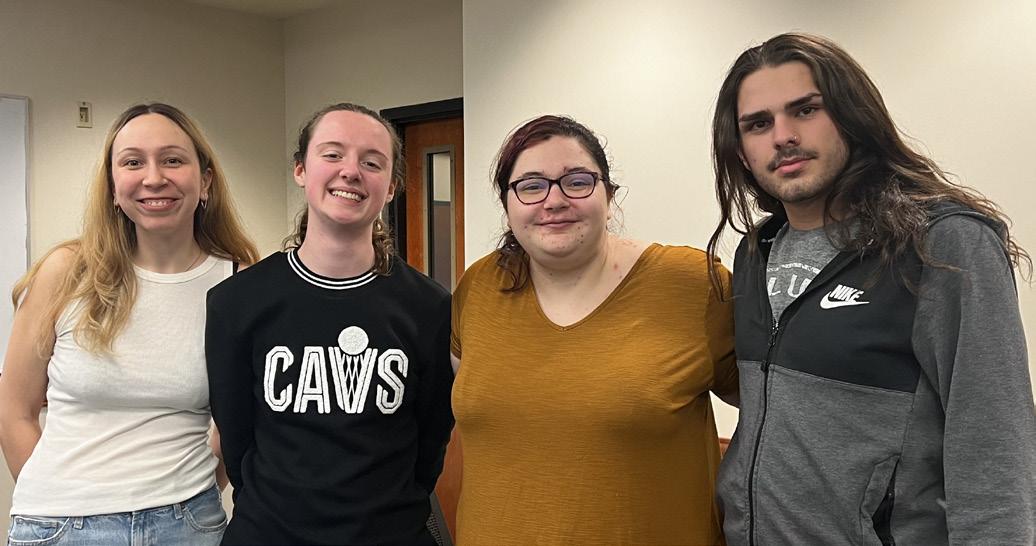
ECS Purdue University Student Chapter
The ECS Purdue Student Chapter continued its MoChA (Modeling, Characterization, and Analytics) series in Fall 2023 with several in-person seminars. The first invited speaker, Dr. Eva Allen, Postdoctoral Researcher, Argonne National Laboratory, delivered an insightful presentation, “Advanced Techniques of 3D Spatial Characterization for the Diagnosis, Synthesis, and Recycling of Lithium-Ion Batteries.” Prof. Justin L. Andrews, Assistant Professor, Department of Chemistry, Purdue, followed with a talk titled “Atomic and Mesoscale Approaches to Improving Capacity and Rate in V2O5 Cathode Materials.”
On November 14, 2023, the chapter hosted the inaugural “E-Chem Day” at Purdue. The event began with a talk by Dr. Peng Bai, Associate Professor, Department of Energy, Environmental & Chemical Engineering, Washington University in St. Louis, titled “Analytical Electrochemical Engineering of Safe and Sustainable Batteries.” It was followed by a thought-provoking student panel discussion that explored different facets of the battery industry. An engaging Kahoot! trivia session blended entertainment and education with electrochemistry-themed questions. The day ended with “E-Chem Art & Science,” an art and photography exhibition symbolizing the fusion of art and science within electrochemistry. Prof. Partha P. Mukherjee, the chapter’s lead faculty advisor, launched the exhibition that celebrated students’ artistic talents along with their scientific pursuits.
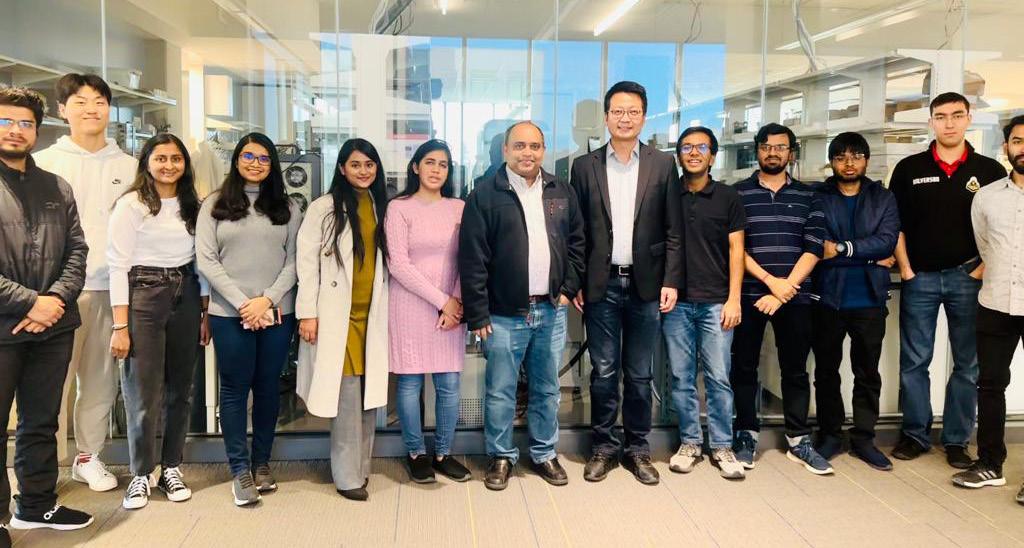
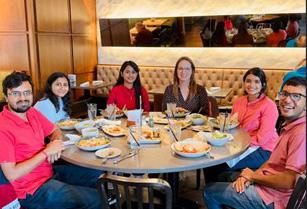
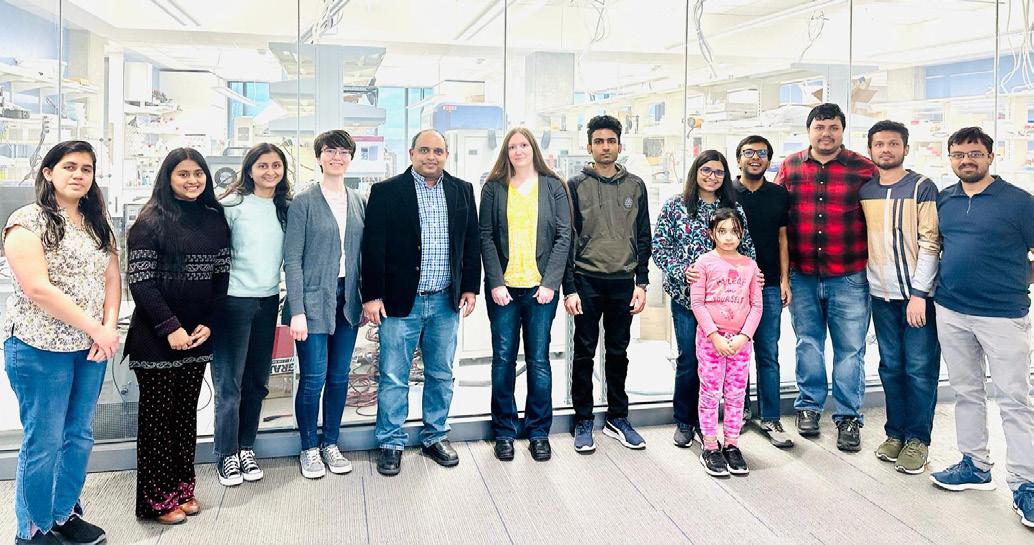
Newly elected ECS Ohio University Student Chapter officers are (from left to right): Vice President Zhuldyz Zhigulina, Secretary Isabel Baca, President Abigail Paul, and Treasurer Nathan Wichert
ECS Purdue University Student Chapter members lunch with invited speaker Dr. Eva Allen (from left to right): Secretary Sourim Banerjee, Events Coordinator Anuththara Alujjage, Communications Director Pretty Mitra, speaker Dr. Eva Allen, President Debanjali Chatterjee, and Treasurer Aditya Singla.
Photo by Aditya Singla
Prof. Partha P. Mukherjee (fifth from the left); invited speaker, Prof. Jennifer L. Schaefer (sixth from the left) with the Energy and Transport Sciences Laboratory group.
Photo: Aditya Singla
As part of the inaugural “E-Chem Day” at Purdue, Prof. Peng Bai (eighth from the left) delivered a talk; seen here with Prof. Partha P. Mukherjee (seventh from the left) and the Energy and Transport Sciences Laboratory group.
Photo: Aditya Singla
STUDENT NEWS STUDENT NEWS
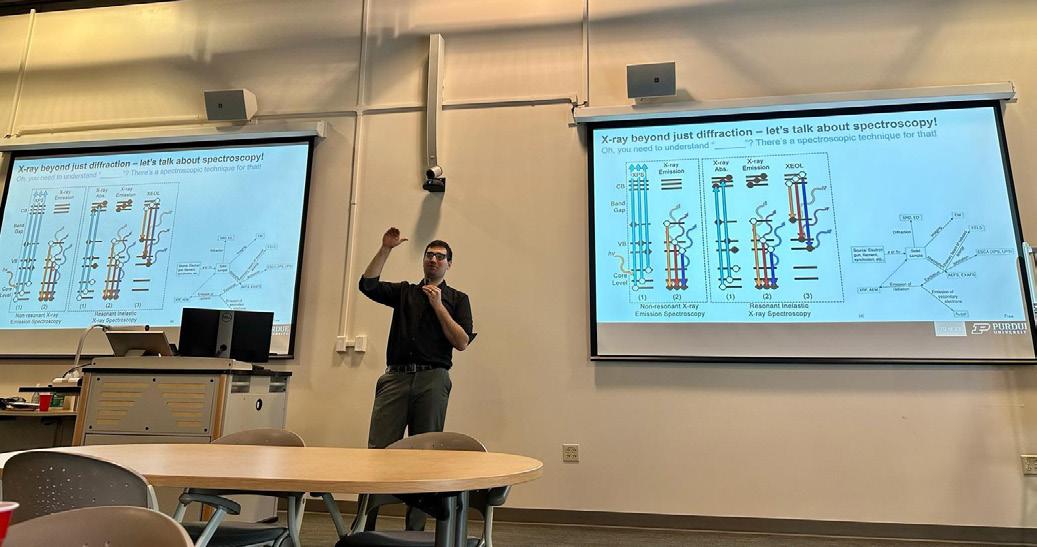
Prof. Justin L. Andrews delivered the ECS Purdue Student Chapter’s inaugural MoChA (Modeling, Characterization, and Analytics) series workshop, “How to Design a Cathode Material: Insights from X-ray Spectroscopy and Scattering.”
Photo: Aditya Singla
In Spring 2024, Prof. Jennifer L. Schaefer, Associate Professor, Department of Chemical and Biomolecular Engineering, University of Notre Dame, visited Purdue and delivered the talk, “Polymers for Next-Generation Electrochemical Energy Storage Devices.” Prof. Babak Anasori, Associate Professor, School of Materials Engineering and Mechanical Engineering, Purdue, presented the next seminar in the series, “Tunable Chemistry of 2D Carbide MXenes for the Hydrogen Evolution Reaction.” As part of their visits, the invited speakers joined chapter officers for lunch, interacting with faculty members and graduate students involved in electrochemistry research.
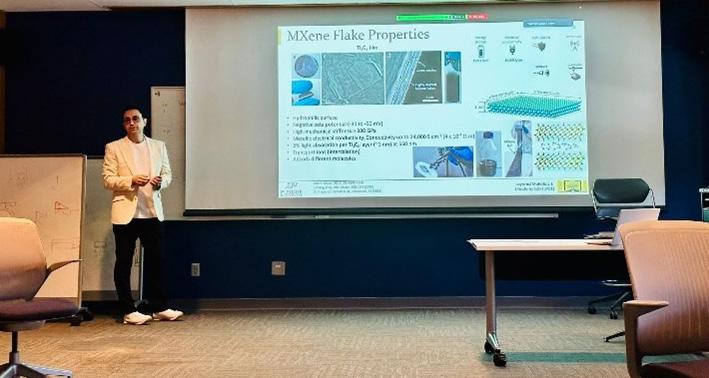
Prof. Babak Anasori presented his talk, “Tunable Chemistry of 2D Carbide MXenes for the Hydrogen Evolution Reaction,” at the concluding ECS Purdue Student Chapter spring semester seminar.
Photo: Aditya Singla
The chapter organized its inaugural MoChA workshop titled “How to Design a Cathode Material: Insights from X-ray Spectroscopy and Scattering,” with Prof. Justin L. Andrews, Assistant Professor, Department of Chemistry, Purdue. The workshop provided valuable insights into material considerations for cathode design by understanding local structural changes. The chapter plans to continue this series in the current academic year and extend its focus to different areas in electrochemical sciences and engineering.
The chapter extends its sincere appreciation to The Electrochemical Society for offering a valuable platform for scientific outreach, and lead faculty advisor Prof. Partha P. Mukherjee for his invaluable time and unwavering guidance. Interface readers are invited to stay up to date with the chapter’s latest activities by following the chapter’s Twitter handle, @EcsPurdue.
ECS Texas Tech University Student Chapter
The ECS Texas Tech University Student Chapter conducted officer elections for the next academic year during the spring semester’s last general meeting on April 24. Chapter members voted the volunteer candidates into office. The new executives—President Jedidian Adjei, Undergraduate Chair Archer Montgomery, Vice President Alejandro Gutierrez, General Secretary Johanna Gonzalez, and Treasurer Bengu Mete—started work in the fall semester, continuing the good work of strengthening the chapter.
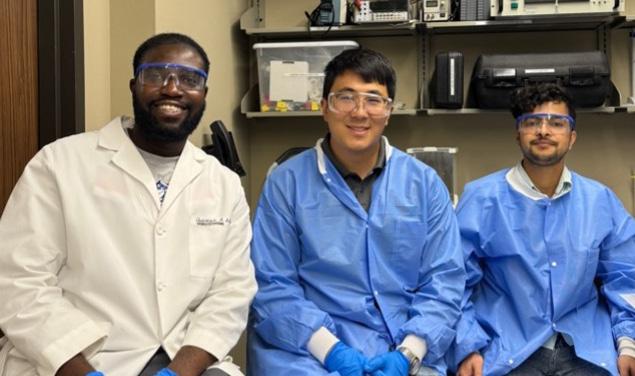
For the final webinar of the spring semester, on May 1, the chapter hosted NASA Sr. Research Scientist/Engineer Dr. James J. Wu, who presented an “Overview and Perspective of Batteries as Electrochemical Energy Storage for Future NASA Missions.” His presentation exposed students to the continuously expanding applications of electrochemistry.
The Center for Advancing Sustainable and Distributed Fertilizer Production (CASFER) collaborated with Pine Research
(continued on next page)
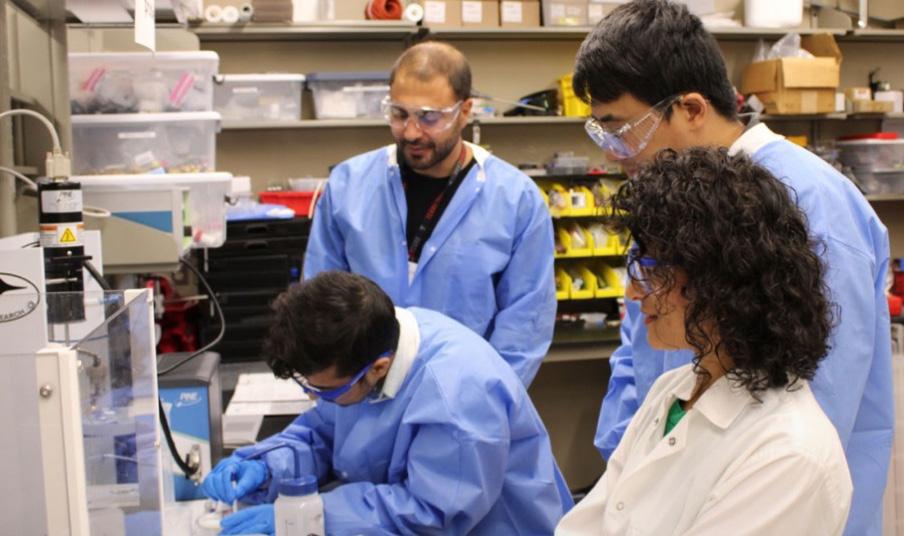
Participants at the two-day electrochemistry workshop hosted by the Center for Advancing Sustainable and Distributed Fertilizer Production (CASFER) in collaboration with Pine Research Instrumentation and the ECS Texas Tech Student Chapter.
Photos: Nathan Caballero
(continued from previous page)
STUDENT NEWS STUDENT NEWS
Instrumentation and the ECS Texas Tech Student Chapter to host a two-day electrochemical workshop on June 13 and 14 at the Maddox Engineering Research Center at Texas Tech. The workshop aimed to provide students and scholars with academic and professional development through a deep understanding of electrochemical processes via hands-on laboratory-designed experiments. The event featured presentations by Prof. Gerardine G. Botte, Texas Tech; Prof. Paul Kohl, Georgia Tech; Dr. Christian Alvarez-Pugliese, Texas Tech; and Dr. Alex Peroff, Pine Research Instrumentation. The event was insightful as participants learned about the complexities of electrochemical research and how to troubleshoot them.
In the future, the chapter aims to increase student engagement and embark on outreach programs. Our efforts to bridge the gap between industry and academia continue as we partner with leading experts, especially industry personnel in the field of electrochemistry in Lubbock and its environs.
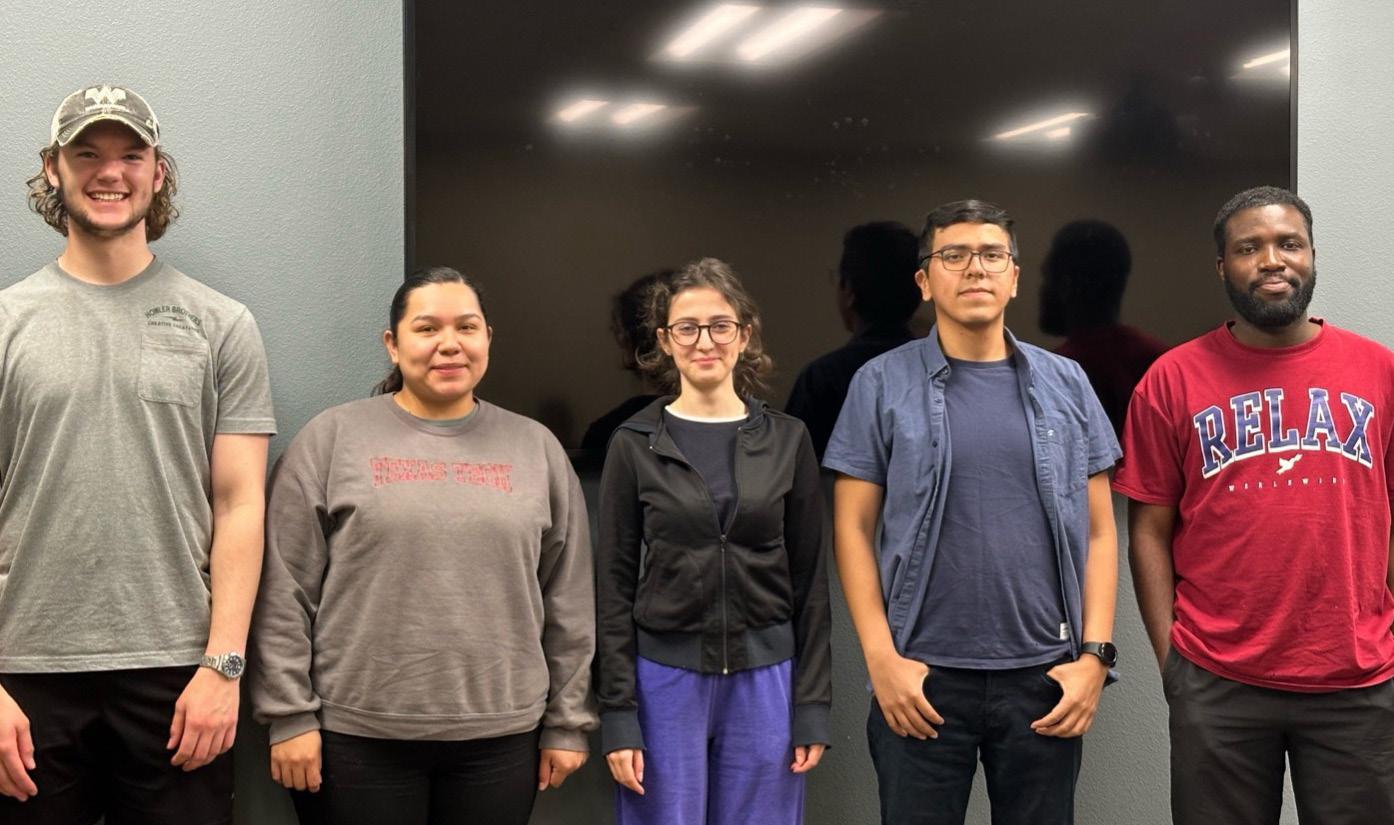
ECS Universidad Autónoma de Querétaro Student Chapter
The executive committee officers for the recently approved ECS Universidad Autónoma de Querétaro (UAQ) Student Chapter are Chair Sebastián Mori Huerta; Vice Chair Dania Leyva Ruiz; Secretary Zyanya Lily Mota Dávila; and Treasurer Erick Manuel Soto Hernández. The chapter acknowledges the invaluable support of their faculty advisor, Dr. Luis A. Godinez, in establishing the student chapter. We are a group of Mexican students enthusiastic about making this multidisciplinary community bigger and contributing to the promotion of electrochemistry all over the world, especially in our country, where there are important challenges that this new generation of scientists will have to face. Furthermore, we aim to establish a platform for students and to provide professional development opportunities through workshops, seminars, and conferences.
On June 20, some student chapter members participated in the IV Symposium on Sustainability and Renewable Energy hosted by the UAQ Faculty of Chemistry Master’s Degree in Environmental Science and Technology and Postgraduate Degree in Energy Sciences. A brief presentation was made at the conclusion of the symposium highlighting the benefits of chapter membership and inviting students to join.
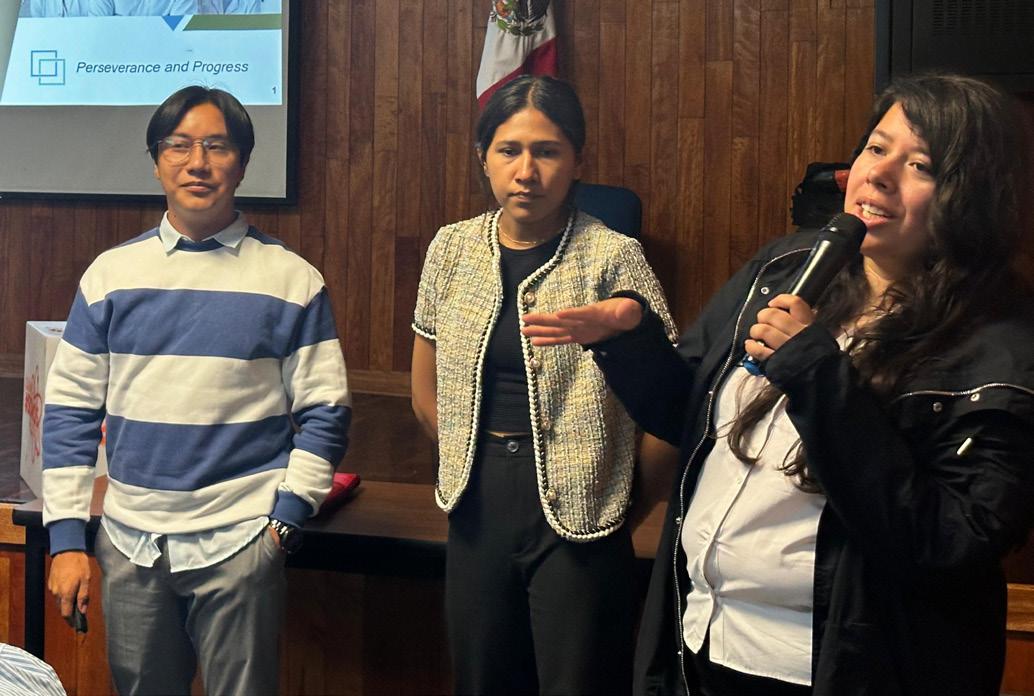
ECS University of California, Irvine Student Chapter
After a hiatus during the COVID-19 pandemic, the ECS University of California, Irvine (UCI) Student Chapter resumed operations in the fall of 2022 with a new board: (now Dr.) Adam Grosvirt-Dramen, Cliffton Wang, Giovanni Ferro, Celine Chen, and Christopher Pantayatiwong Liu. The chapter’s chief aim was, and is, to intellectually enrich young researchers in electrochemical and solid state science by building community and providing opportunities for learning.
For their first event, the chapter hosted Prof. Karthish Manthiram from the California Institute of Technology at UCI’s National Fuel Cell Research Center on November 11, 2022. He shared his group’s recent advances on nitrogen fixation through a lithium-mediated approach for the electrochemical synthesis of ammonia at ambient conditions, as well as the development of non-aqueous gas-diffusion electrodes for high rates of ammonia synthesis. The seminar followed a catered outdoor lunch with ample time for attendees to connect with each other and Prof. Manthiram.
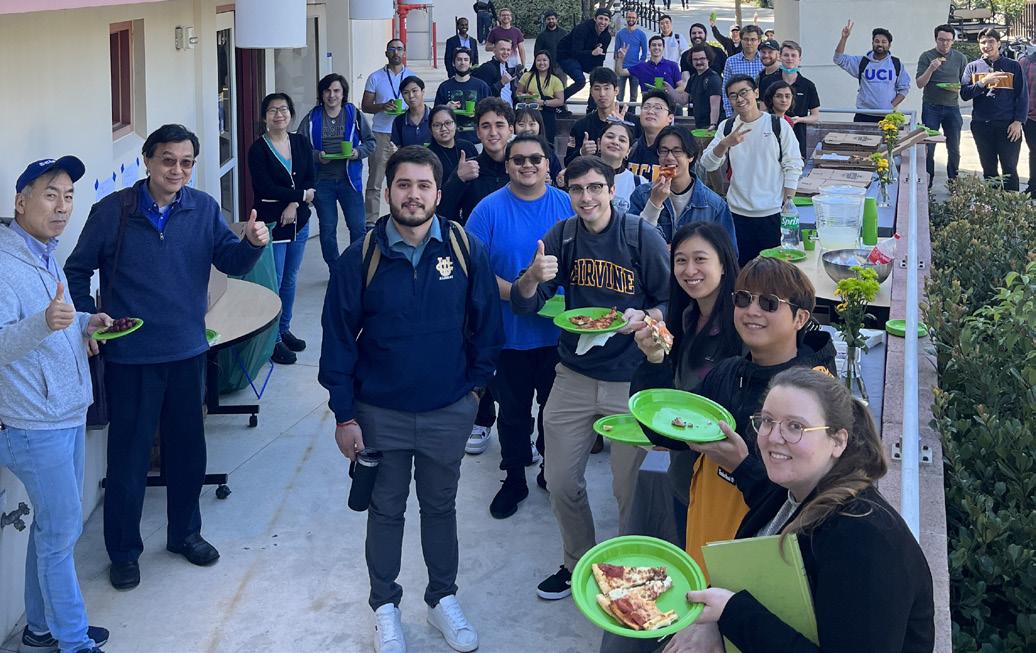
The ECS Texas Tech Student Chapter elected new officers (from left to right): Undergraduate Chair Archer Montgomery, General Secretary Johanna Gonzalez, Treasurer Bengu Mete, Vice President Alejandro Gutierrez, and President Jedidian Adjei
Photo: Nathan Caballero
ECS Universidad Autónoma de Querétaro Student Chapter Officers are (from left to right): Chair Sebastián Mori Huerta, Vice Chair Dania Leyva Ruiz, and Secretary Zyanya Lily Mota Dávila.
Prof. Karthish Manthiram joined the ECS University of California, Irvine (UCI) Student Chapter fall 2022 kickoff lunch.
Photo: Yu-Han Chen
STUDENT NEWS STUDENT NEWS
After the fall seminar, chapter leaders considered how to create an event connecting UCI’s student electrochemical and solid state community with the greater Southern California community. From this was born the SoCal Electrochemistry Conference for Students (SCECS), which took place on April 19, 2023, at UCI’s Interdisciplinary Science and Engineering Building. Students from UCI and neighboring California State University, Long Beach; California Institute of Technology; Pomona College; Universities of California, San Diego and Santa Barbara; and the University of Southern California (USC) presented 20 student talks and 20 student posters. Keynote speakers Prof. Kimberly See, Caltech, and Dr. Adam Weber, Lawrence Berkeley National Laboratory, shared advances in battery chemistries beyond Li-ion technologies and multiphysics modeling of transport processes in gas diffusion electrodes and ionomers in CO2 reduction electrolyzers, respectively. While the chapter is proud of many aspects of the conference—given that it was entirely student originated, planned, and executed—we are perhaps proudest that the conference provided a platform for many students to give their first poster and/or conference talk. The feedback from the 80-plus attendees was enthusiastically positive, with many
(continued on next page)
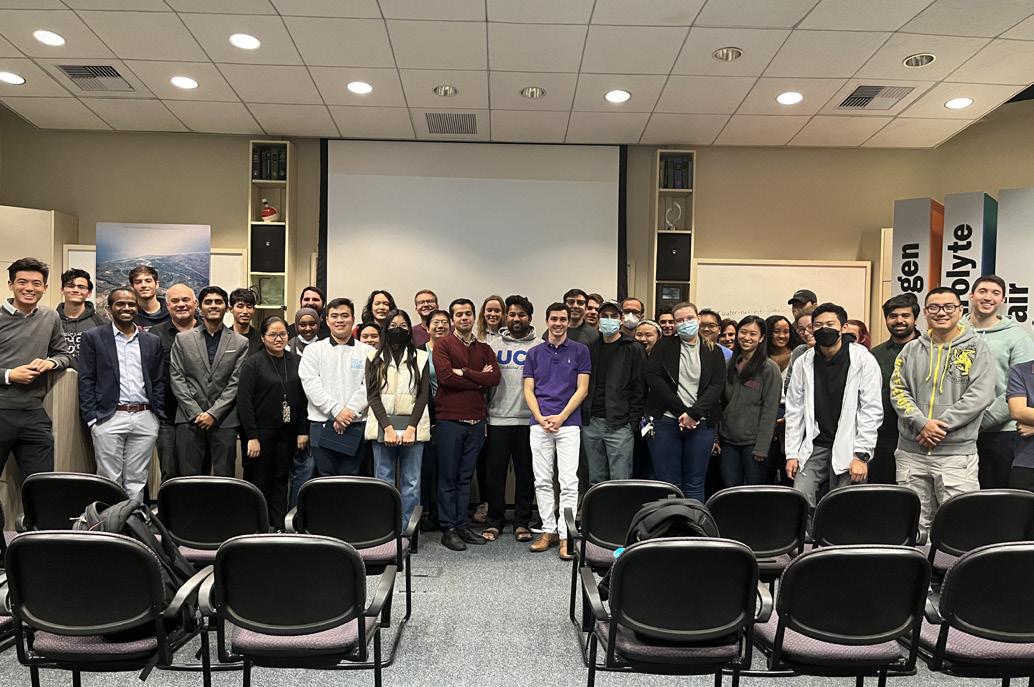
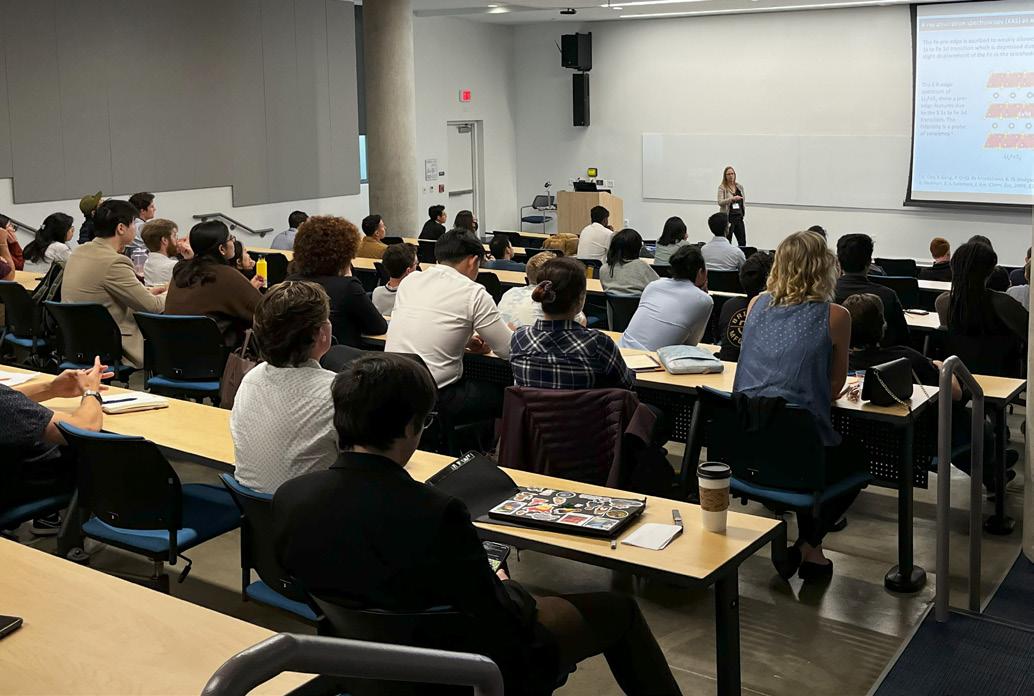
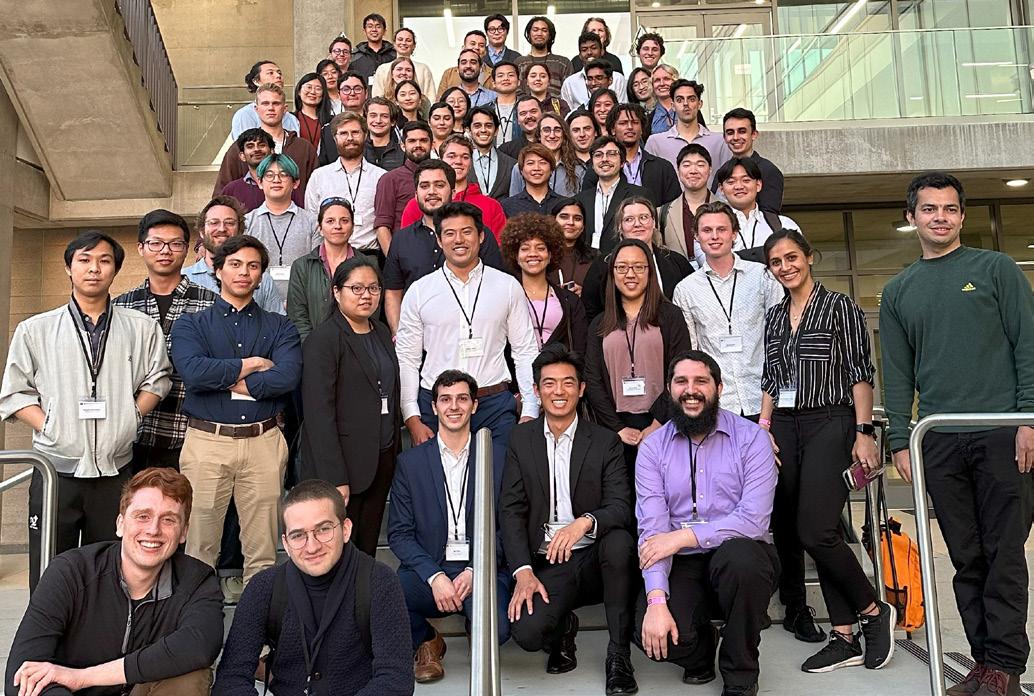
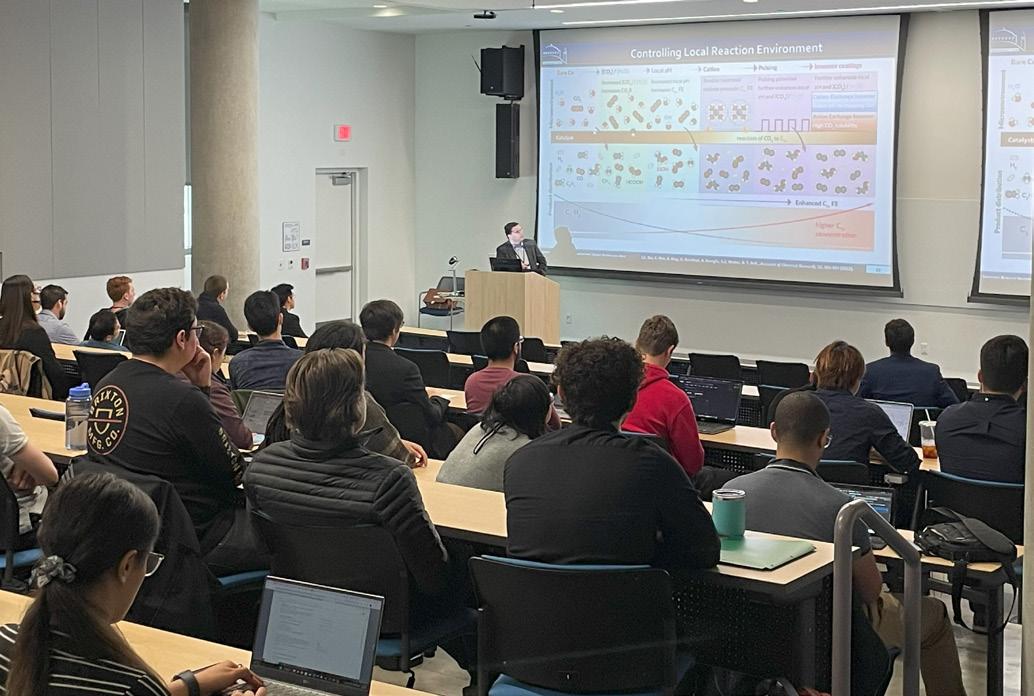
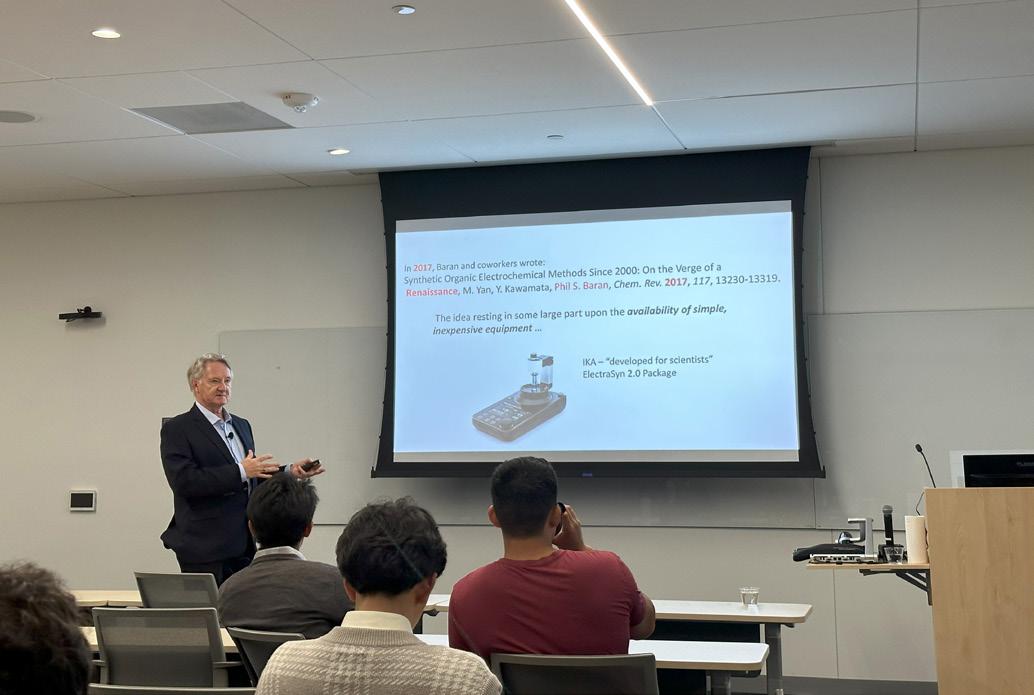
Dr. Adam Weber delivers a keynote lecture during the 2023 SCECS.
Photo: Patrick Yang
Attendees gather after the 2023 SCECS happy hour and dinner. Pictured in the second row, kneeling, are board members (left to right): Giovanni Ferro, Christopher Pantayatiwong Liu, and Dr. Adam Grosvirt-Dramen In the third row, standing, are board members Celine Chen (third from left) and Cliffton Wang (fourth from left). In the fourth row is student chapter faculty advisor Prof. Iryna Zenyuk (second from left).
Photo: Patrick Yang
Prof. Daniel Little delivers a lecture on redox mediators during the 2023 fall kickoff seminar.
Photo: Patrick Yang
For their November 11, 2022 fall kickoff event, the chapter hosted Prof. Karthish Manthiram, California Institute of Technology, at UCI’s National Fuel Cell Research Center.
Photo: Patrick Yang
Prof Kimberly See delivered the 2023 SoCal Electrochemistry Conference for Students (SCECS) keynote lecture.
Photo: Patrick Yang
(continued from previous page)
STUDENT NEWS STUDENT NEWS
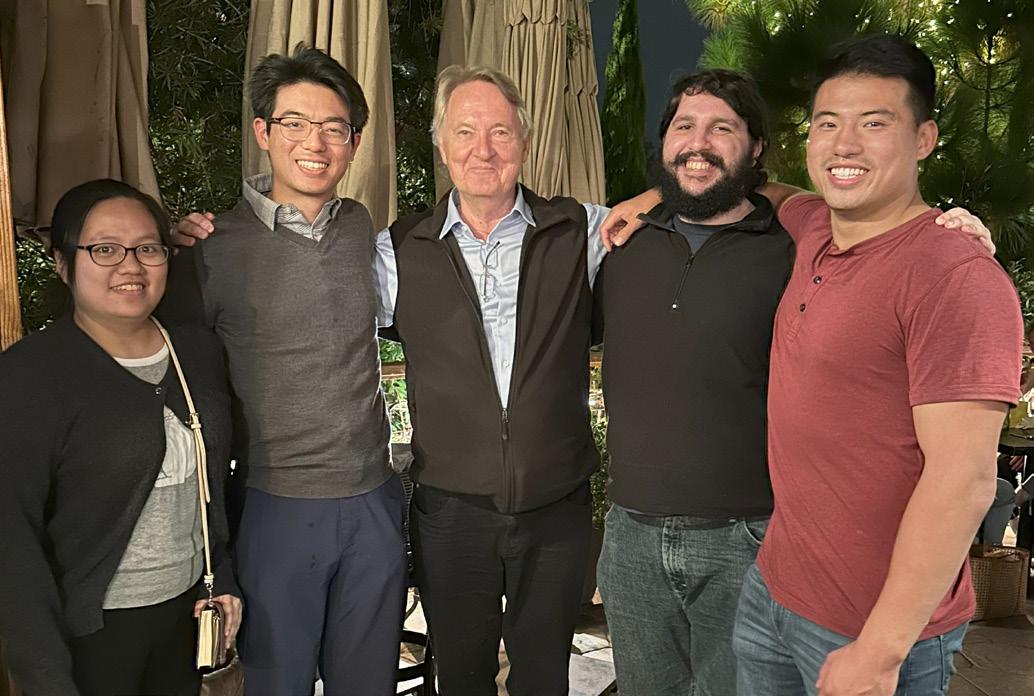
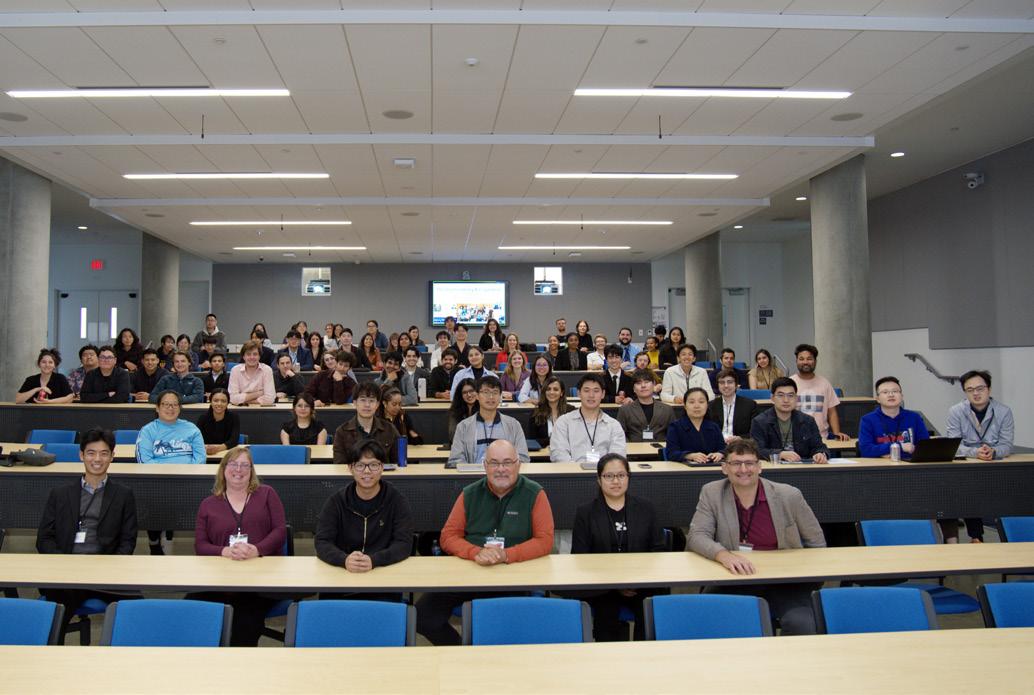
citing the student-centric nature and “just-right” size as reasons they would attend again. The 2023 SCECS was generously sponsored by the Clean Energy Institute, National Fuel Cell Research Center, and ECS.
The chapter maintained its original board and program for the 2023–2024 academic year. Prof. Daniel Little, UC Santa Barbara, presented the 2023 fall kickoff seminar with a lecture on the history, behavior, and use of redox mediators for electrosynthesis. Following the lecture, Prof. Little joined the chapter’s board for dinner, generously sharing research and career advice with the team.
On April 19, 2024, the chapter hosted the second SoCal Electrochemistry Conference for Students, with 18 student presentations and 21 student posters. Over 100 attendees were present, representing (in addition to UC Irvine) Cal State Long Beach, Cal State Los Angeles, Caltech, Pomona College, UC Los Angeles, UC San Diego, UC Santa Barbara, and USC. Keynote speakers Prof.
Shelley Minteer, Missouri Institute of Science and Technology, and Prof. Shannon Boettcher, University of California, Berkeley, lectured on enzymatic electrocatalysis and alkaline water electrolysis and bipolar membrane electrodialysis, respectively. The student organizers were happy to see repeat attendees and new faces, as well as many cross-laboratory and cross-campus exchanges. Attendees from several institutions mentioned their interest in starting student chapters at their home institutions.
For the 2024–2025 year, the chapter welcomed new board members Jared Stanley, Ivy Wang, Hannah Ruffo, Ciara Gillis, and Adam Sabatose. We are excited to implement additional programs to better support the UCI electrochemistry and solid state science student community, such as rotating journal clubs, research talks and discussions, tutorials, guest lectures, and even technique demonstrations, as well as social outings, since personal relationships are an integral part of a community.
ECS University of Michigan Student Chapter
The ECS University of Michigan (U-M) Student Chapter closed the spring semester with “From Fundamentals to Frontiers: Exploring PEMFCs (proton-exchange membrane fuel cells) Innovations at Toyota Research Institute of North America,” a seminar by Dr. Liang Wang, Senior Scientist, Toyota Research Institute of North America. Dr. Wang and other Toyota research scientists provided invaluable insight into Toyota’s research, culture, and facilities, and a unique networking opportunity for student participants. The chapter looks forward to future collaborations with Toyota and other local industries.
Partnering with U-M’s Battery Club continued with a co-hosted fireside chat with Dr. Halle Cheeseman, ARPA-E Program Director, and Dr. Apoorv Agarwal, ARPA-E Technology-to-Market Advisor, moderated by Prof. Venkat Viswanathan. Dr. Cheeseman and Dr. Agarwal shared details about ARPA-E’s initiatives, programs, and fellowship opportunities along with their own career paths and their outlook on future innovative battery technologies.
The chapter also hosted social and recruitment events to encourage engagement with student members and new electrochemists. A sand volleyball and boba social sparked the start of summer, bringing student members out to enjoy the weather and build community. Stay tuned in the coming months as chapter leadership looks forward to many more events and the first student elections!
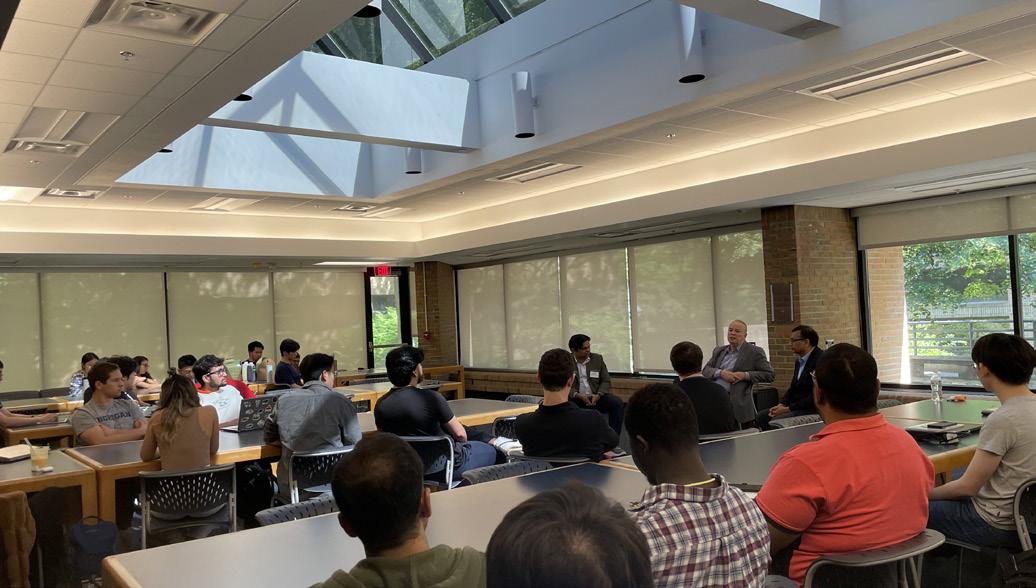
The ECS U-M Student Chapter collaborates with the U-M Battery Club to present a fireside chat moderated by Prof. Venkat Viswanathan (seated facing the audience, left) with Dr. Halle Cheeseman, ARPA-E Program Director (seated facing the audience, middle), and Dr. Apoorv Agarwal, ARPA-E Technology-to-Market Advisor (seated facing the audience, right).
Daniel Liao.
Participants in the 2024 SCECS included (front row, left to right): Chapter President Christopher Pantayatiwong Liu, keynote speaker Prof. Shelley Minteer, member Loki Chen, faculty advisor Prof. Plamen Atanassov, Vice President Celine Chen, and keynote speaker Prof Shannon Boettcher
Photo: Patrick Yang
Prof. Daniel Little dined with the chapter board at Roger’s Garden (from left to right): Celine Chen, Christopher Pantayatiwong Liu, Prof. Daniel Little, Adam Grosvirt-Dramen, and Cliffton Wang
Photo: Patrick Yang
Photo:
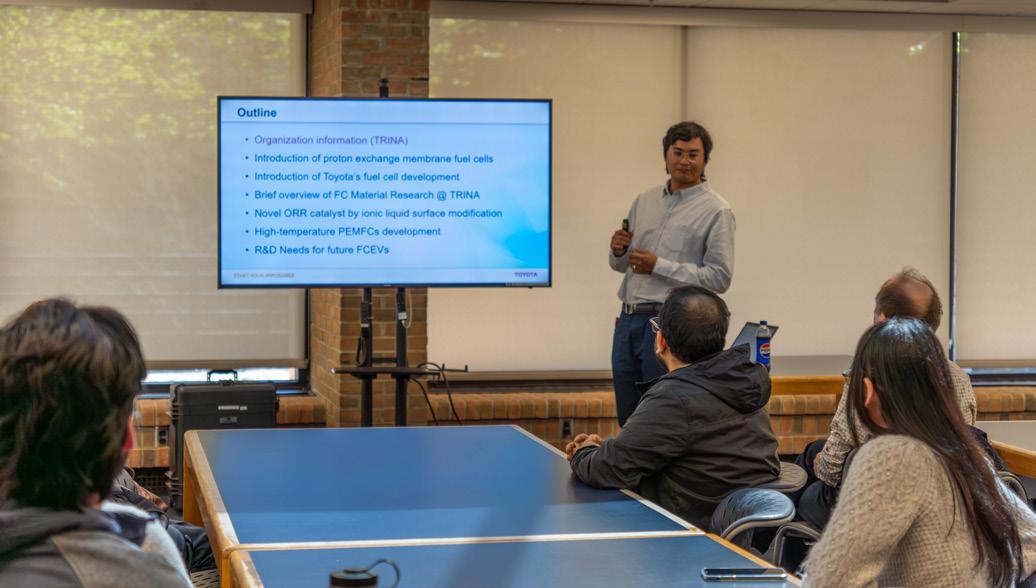
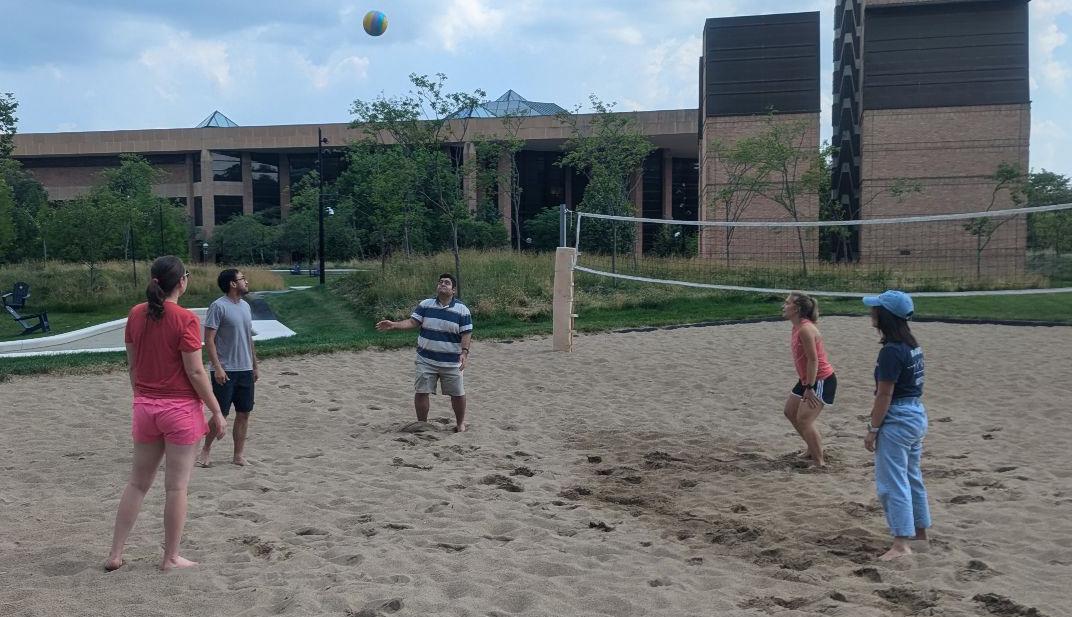
ECS University of Nebraska-Lincoln Student Chapter
The University of Nebraska-Lincoln (UNL) Student Chapter proudly participated in a week-long NSF-supported Molecular Engineering Summer Camp, engaging 30 high school students with hands-on experimental work geared to highlight the significance of molecular engineering in the field of electrochemistry. The event occurred at UNL Othmer Hall, where our chapter members and high school attendees showcased their passion and expertise.
All graduate and undergraduate chapter members in the Department of Chemical and Biomolecular Engineering actively contributed to the event. Chapter President Sahand Serajian introduced the high school students to ECS and presented the fundamentals of electrochemistry. One of the key activities during the camp was an electroplating demonstration, where students observed the electrodeposition of nickel on copper foils in the presence of organic additives. An undergraduate chapter member, Sam Peterson, planned the hands-on experiment, which was executed under the supervision of scientists, the camp director, and ECS chapter advisors. This hands-on experiment demonstrated the practical applications of electrochemical principles and provided valuable insights into the process of electroplating.
After the presentation, the high school students were given comprehensive lab tours of the electrochemistry facilities. This immersive experience allowed them to see firsthand the various techniques and equipment used in electrochemical research.
The presentation was well received by attendees, sparking great interest in joining the chapter when they get to college and fostering curiosity and enthusiasm for electrochemistry among the next generation of scientists. We look forward to continuing our outreach efforts and inspiring more students in future events.
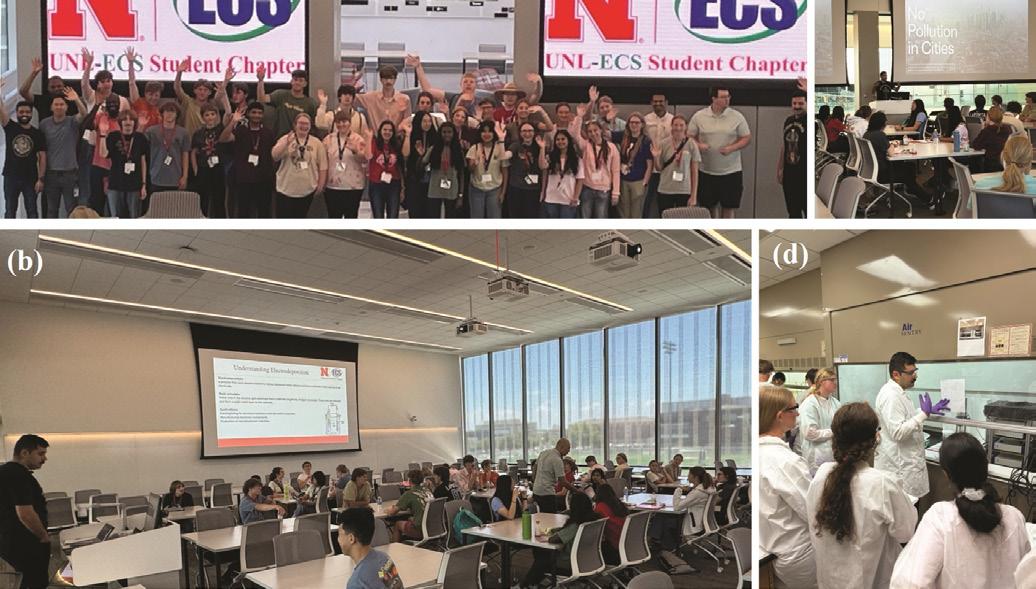
ECS University of Nebraska-Lincoln (UNL) Student Chapter officers and members participated in the NSF-supported High School Research Experience in Molecular Engineering (HiREME) Summer Camp. (a) Group photo. (b) Chapter President Sahand Serajian presented a talk on electrochemistry fundamentals. (c) Sahand Serajian discussed the significance of electrochemistry in sustainability and pollution reduction in cities. (d) Camp students tour the UNL electrochemistry lab.
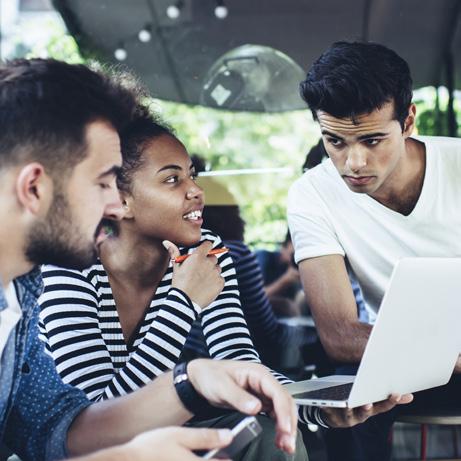
Invited speaker Dr. Liang Wang, Senior Scientist at Toyota Research Institute of North America, presents “From Fundamentals to Frontiers: Exploring PEMFCs Innovations at Toyota Research Institute of North America,” to the ECS U-M Student Chapter.
Photo: Daniel Liao
The ECS U-M Student Chapter wraps up the spring semester on a warm day with a sand volleyball and boba social/recruitment event!
Photo: Daniel Liao
ECS University of Texas at El Paso Student Chapter
The ECS University of Texas at El Paso (UTEP) Student Chapter hosted a successful two-day inaugural event in collaboration with the UTEP Chemistry and Biochemistry Department and the NSF Center for Synthetic Organic Electrochemistry. The event was a platform for renowned experts and enthusiastic students to converge and exchange knowledge. The first day featured a series of lectures by distinguished scholars, including Professors Dylan Boucher, Baylor University; Nathan Friede, California Institute of Technology; Kevin Moeller, Washington University in St. Louis; Shelly Minteer, Missouri University of Science and Technology; Long Luo, Wayne State University; Scott Anderson, University of Utah; and Matthew Neurock, University of Minnesota. UTEP students Satya Prakash Sumon and Sheng Yin presented their research, showcasing promising talent within the university.
The second day was dedicated to a poster presentation session with UTEP electrochemistry groups exhibiting their ongoing research projects. The session provided a collaborative atmosphere
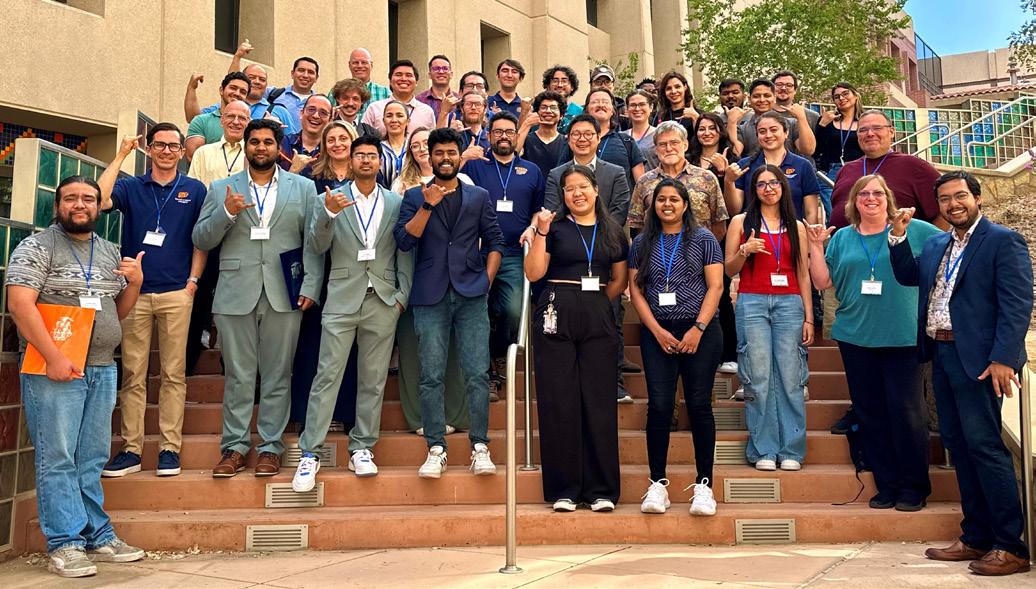
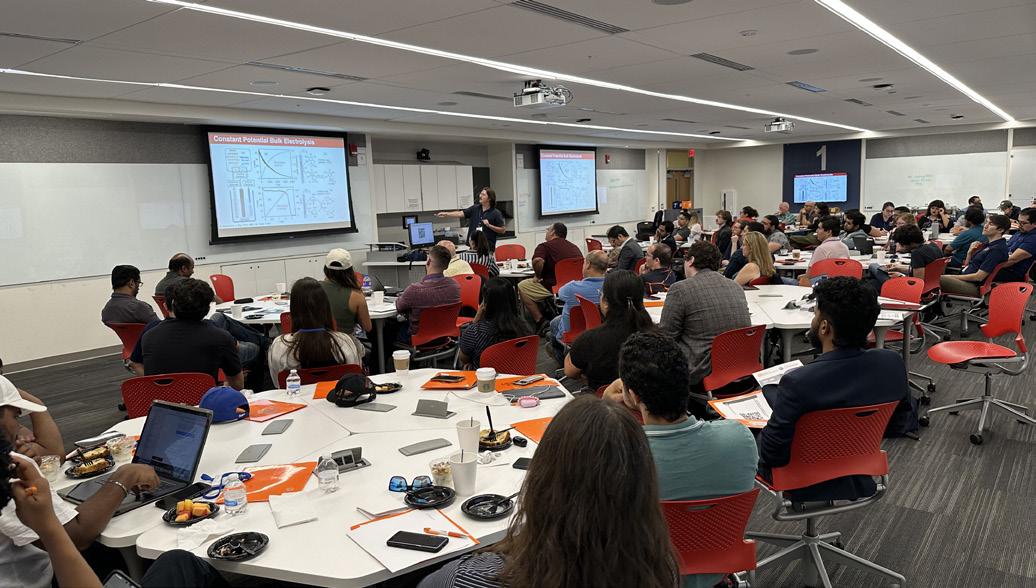
for students and faculty to engage in discussions, fostering a deeper understanding of various electrochemical processes and innovations. The posters covered a wide range of topics, reflecting UTEP’s vibrant scientific community, diverse research interests, and cutting-edge research, while promoting the interdisciplinary interactions that are essential for the advancement of electrochemical studies.
The chapter expresses its gratitude to all the professors who supported the event, especially Professors Skye Fortier and Elizabeth Day, whose guidance and cooperation were instrumental in its success. This event marked a significant step toward enhancing students’ electrochemical knowledge and skills, providing them with invaluable exposure to leading experts and current research trends in the field. The collaborative efforts and intellectual exchanges facilitated by this event are expected to inspire and propel the next generation of electrochemists at UTEP, fostering an environment of innovation and academic excellence.
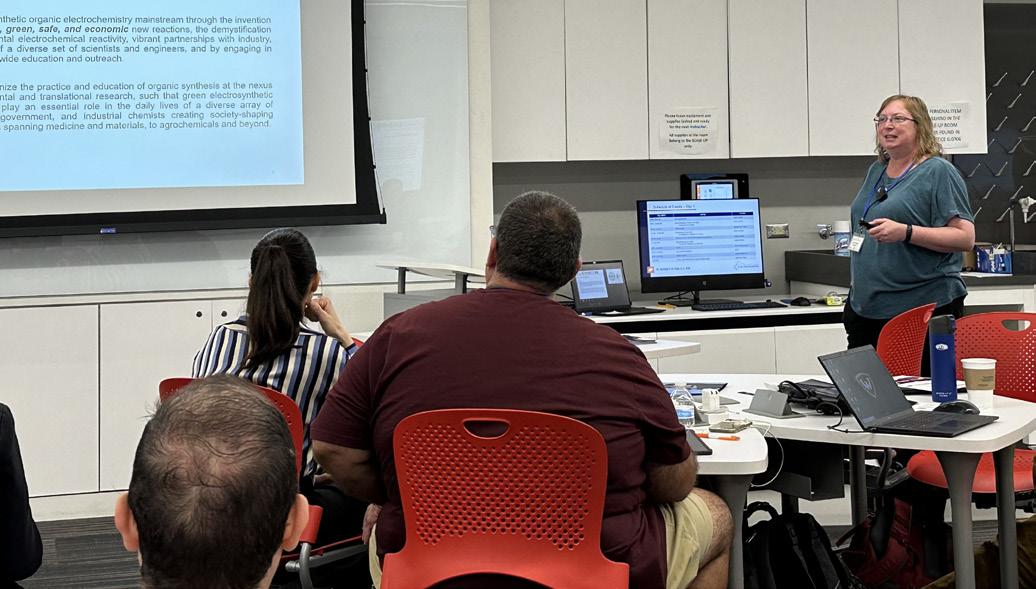
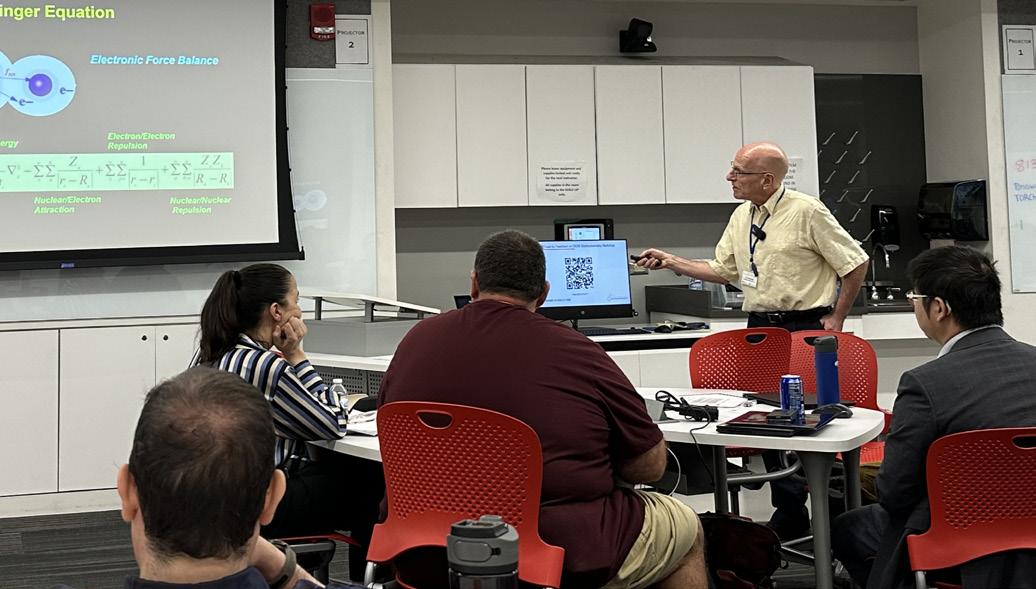
University of Texas at El Paso (UTEP) students and faculty gather following their inaugural workshop.
Dr. Shelly Minteer lectures at the inaugural ECS UTEP Student Chapter seminar.
Dr. Matthew Neurock introduces participants to computational organic electrochemistry at the inaugural ECS UTEP Student Chapter seminar.
Dr. Dylan Boucher presents his research at the inaugural ECS UTEP Student Chapter seminar.
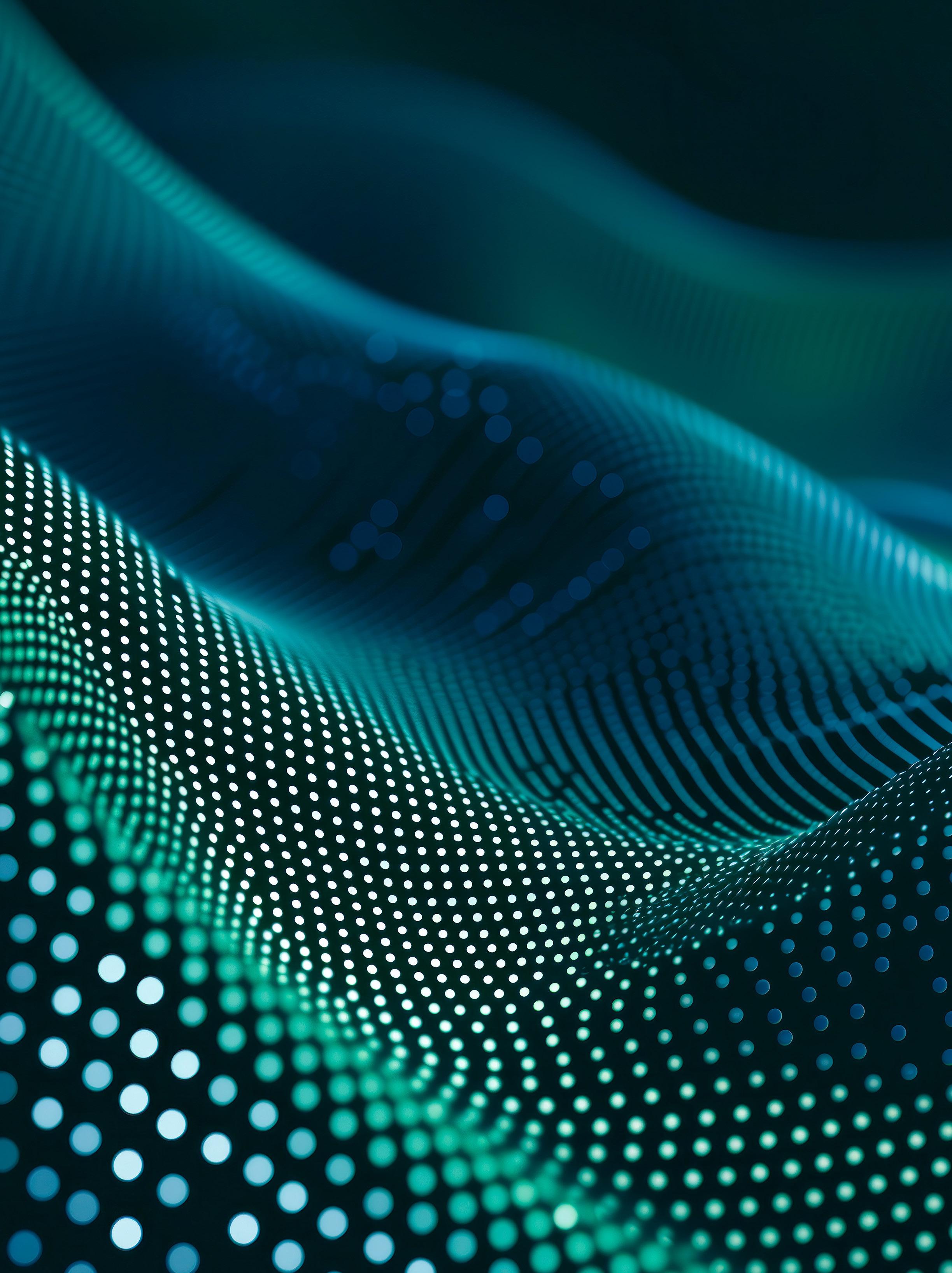
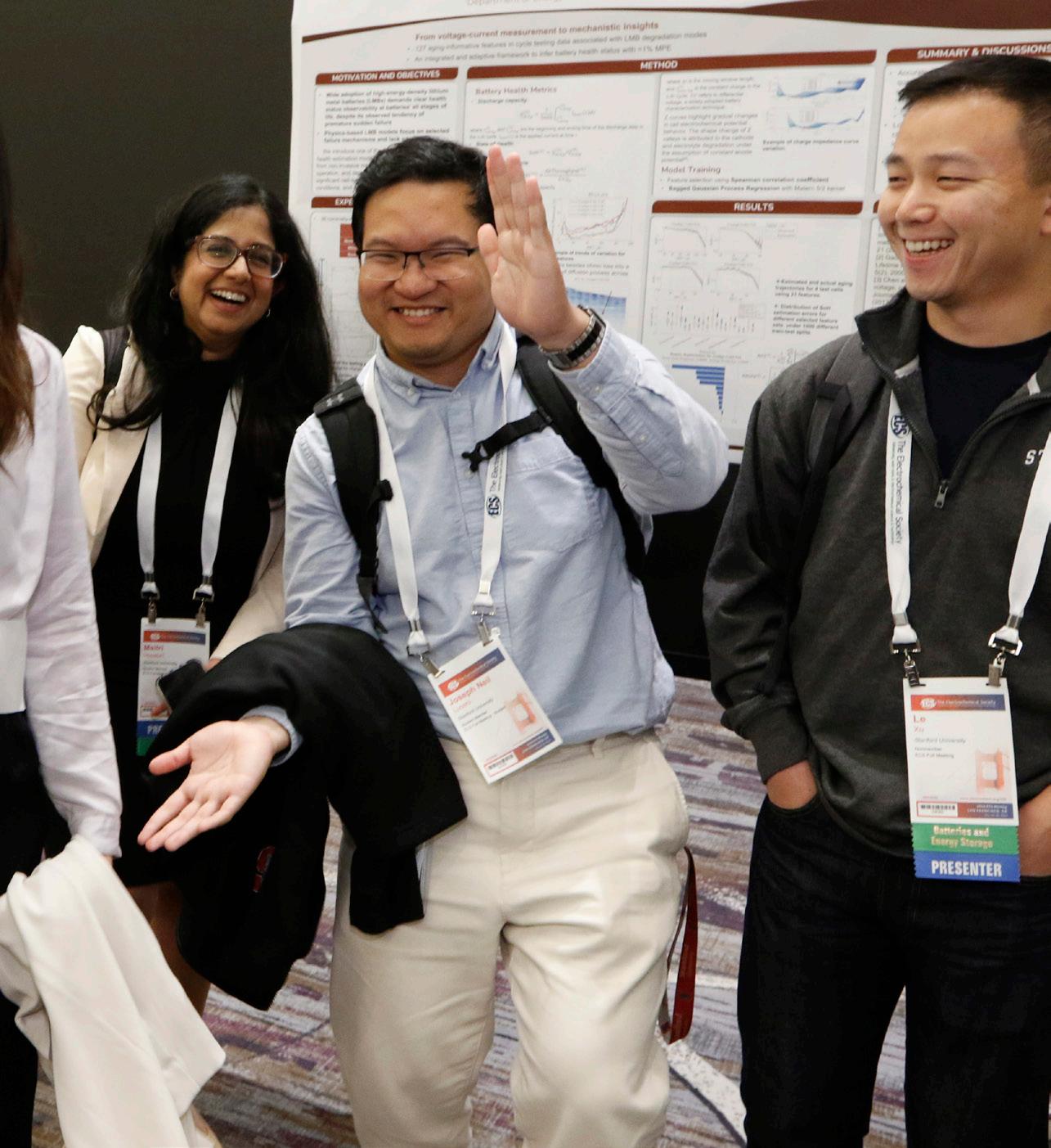
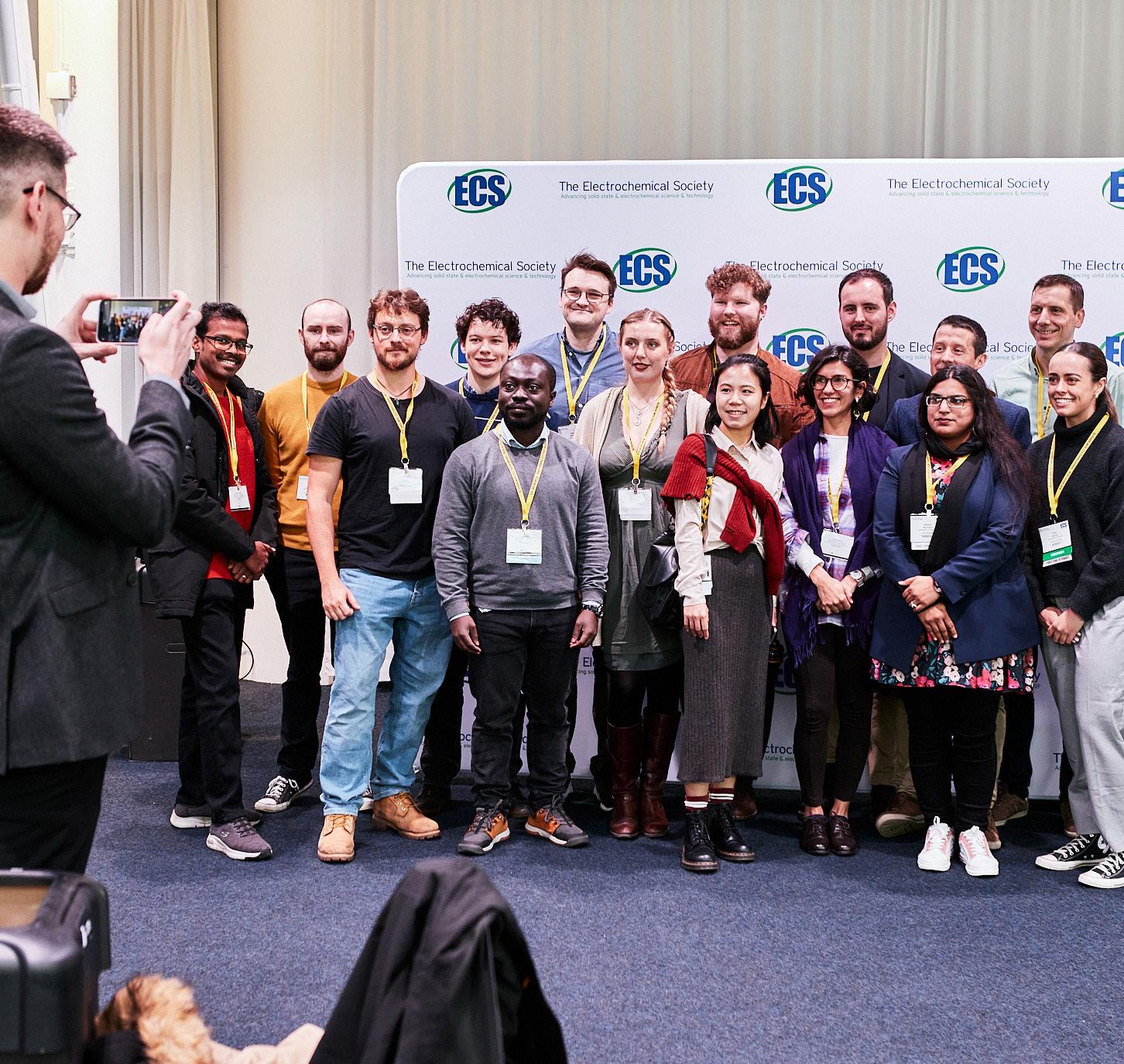
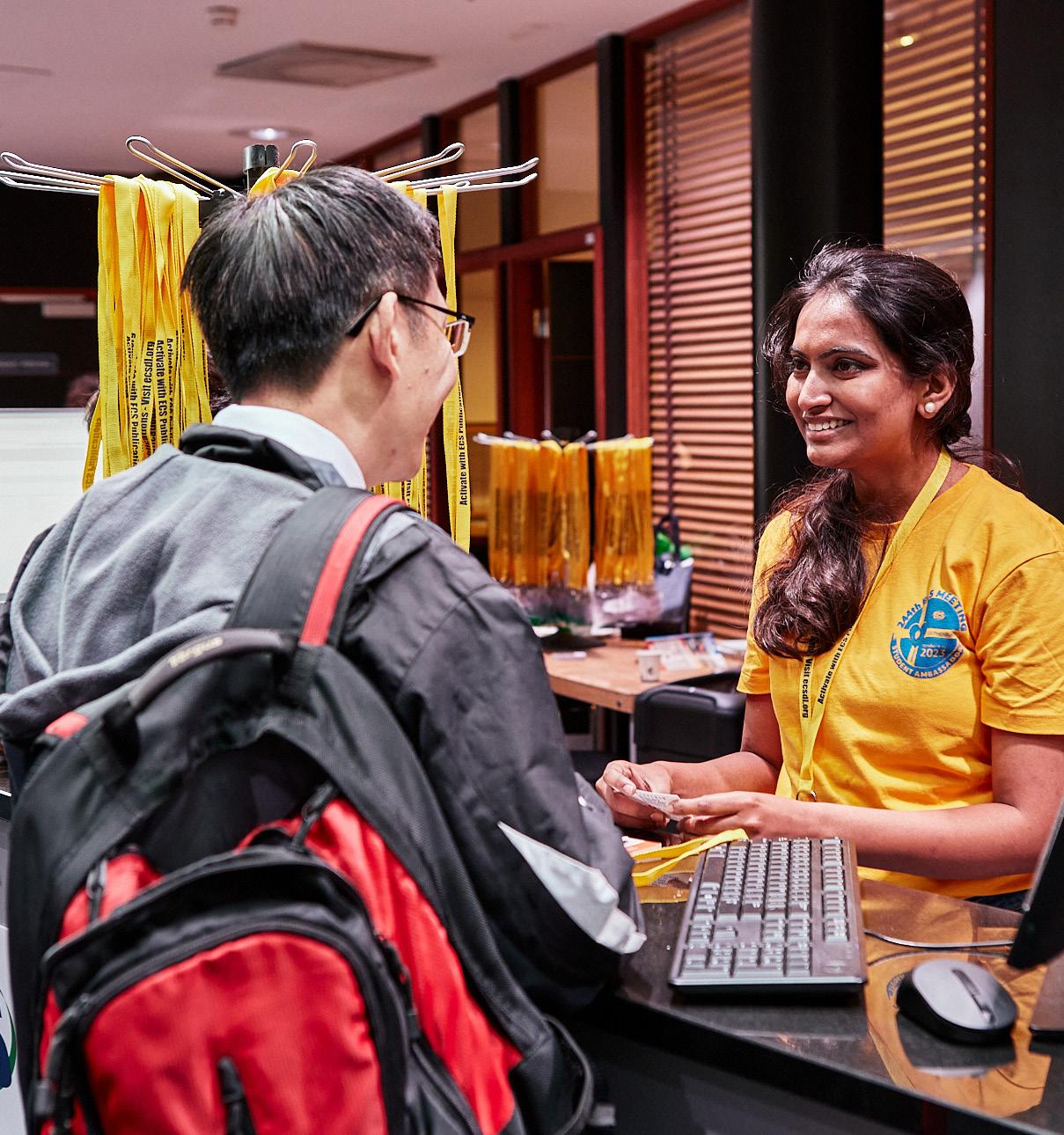
GENERAL INFORMATION
The 247th ECS Meeting takes place in Montréal, Canada, May 18-22, 2025, at the Palais des Congrès de Montréal. This international conference brings together scientists, engineers, and researchers from academia, industry, and government laboratories to share results and discuss issues on related topics through a variety of formats, including oral presentations, panel discussions, poster sessions, tutorial sessions, Short Courses, professional development workshops, and exhibits. The unique blend of electrochemical and solid state science and technology at an ECS meeting provides a forum to learn and exchange information on the latest scientific and technical developments in a variety of interdisciplinary areas.
ABSTRACT SUBMISSION
To give an oral or poster presentation at the 247th ECS Meeting, submit an original abstract for consideration via the ECS website https://ecs.confex.com/ecs/247/cfp.cgi no later than December 6, 2024. Faxed, emailed, and/or late abstracts are not accepted. Meeting abstracts should explicitly state the work’s objectives, new results, and conclusions or significance. After the submission deadline, symposium organizers evaluate all abstracts for content and relevance to the symposium topic, and schedule accepted submissions as either oral or poster presentations.
Letters of Acceptance/Invitation are emailed in February 2025, notifying corresponding authors of accepted abstracts, and the date, time, and location of their presentations.
How and when a poster or oral presentation is scheduled is at the symposium organizers’ discretion, regardless of presenters’ requests.
PAPER PRESENTATION
Oral presentations must be in English. LCD projectors and laptops are provided for all oral presentations. Presenting authors MUST bring their presentations on USB flash drives to use with dedicated laptops located in each technical session room. Speakers requiring additional equipment must make written request to meetings@electrochem.org at least one month prior to the meeting so appropriate arrangements can be made, subject to availability, and at the author’s expense.
Poster presentations must be in English. Print posters in A0 format (84.1cm x 118.9cm or 33.1in x 46.8in) and label them with the abstract number and day of presentation as printed in the final program.
Participants in the Z01—General Student Poster Competition are required to (1) Upload a digital poster file in advance of the meeting and (2) Be present during the in-person judging session on Tuesday evening. The deadline to upload a digital file for the competition is emailed to presenting authors. The prize categories are 1st Place ($1,500 award), 2nd Place ($1,000 award), and 3rd Place ($500 award).
MEETING PUBLICATIONS
ECS Meeting Abstracts—All meeting abstracts associated with a presentation given at the scheduled meeting are published in the ECS Digital Library approximately six months after the meeting closes. All abstracts are copyrighted by ECS and become ECS’s property upon presentation.
ECS Journals—Authors presenting papers at ECS meetings are encouraged to submit to the Society’s technical journals: Journal of The Electrochemical Society, ECS Journal of Solid State Science and Technology, ECS Advances, and ECS Sensors Plus. Although there is no hard deadline for submitting these papers, six months from the symposium date is considered sufficient time to revise a paper to meet stricter journal criteria. Author instructions are on the ECS website.
SHORT COURSES
ECS Short Courses provide students and seasoned professionals with in-depth education on a wide range of topics. Novices and experts advance their technical expertise and knowledge through personalized instruction by academic and industry experts. Short Courses require
advance registration and may be canceled if course enrollment is under 10 registrants. Learn more at https://www.electrochem.org/ short-courses
EXHIBIT HALL
The 247th ECS Meeting is the right place to exhibit. ECS provides a powerful platform for meeting major new customers and enhancing relationships with current customers from around the world. Traffic in the exhibit hall is generated by coffee and networking breaks along with evening poster sessions.
Your presence at ECS’s leading industry event positions your brand as serious and reliable—and it’s a great way to build buzz for new products! Exhibit opportunities can be combined with sponsorship to suit your marketing needs. Contact sponsorship@electrochem.org for further details.
MEETING REGISTRATION
All participants including authors and invited speakers are required to pay the appropriate registration fees. Meeting registration information is posted on the ECS website as it becomes available. The deadline for discounted early registration is April 21, 2025.
HOTEL RESERVATIONS
For the most up-to-date information on hotel availability and blocks of rooms with special rates for meeting participants, refer to the meeting website. The hotel block is open until April 21, 2025, or it sells out.
LETTERS OF INVITATION
Letters of Invitation are emailed in February 2025 to the presenting authors of all accepted abstracts, notifying them of the date, time, and location of their presentations. Email abstracts@electrochem.org if you require an official Letter of Invitation (these letters do not imply any financial responsibility on the part of ECS).
BIANNUAL MEETING TRAVEL GRANTS
Some ECS divisions and sections offer travel grants to assist students, postdoctoral researchers, and young professionals in attending ECS biannual meetings. Applications are available beginning December 6, 2024, at www.electrochem.org/travel-grants. The submission deadline is February 24, 2025.
SYMPOSIA FUNDING ASSISTANCE
Additional financial assistance is limited and generally governed by symposium organizers. To inquire if additional funding is available, contact the organizers of the symposium in which you are presenting.
SPONSORSHIP OPPORTUNITIES
ECS biannual meetings provide a wonderful opportunity to solidify and strengthen your brand through sponsorship. Give your brand more visibility and reinforce your position as an industry leader by sponsoring ECS meeting events and enhancing ECS meetings. Choose from a wide array of activities—from symposia to special events—which deliver worldwide recognition as a supporter of electrochemical and solid state research.
ECS also offers specific symposium sponsorships which help offset travel expenses or registration fees. Or sponsors can host receptions for invited speakers, researchers, and students. Contact sponsorship@electrochem.org for details.
CONTACT INFORMATION
If you have questions and/or need more information, contact ECS
The Electrochemical Society 65 South Main Street, Pennington, NJ, 08534-2839, USA Tel: 1.609.737.1902; fax: 1.609.737.2743 meetings@electrochem.org www.electrochem.org
247th ECS MEETING SYMPOSIUM TOPICS AND DEADLINES
A Batteries and Energy Storage
A01 New Approaches and Advances in Electrochemical Energy Systems
A02 Whittingham Young Investigator and Student Slam
A03 Lithium Batteries and Beyond
A04 Large-Scale Energy Storage
A05 Battery Characterization and Diagnosis
A06 Metal Anodes and Interfacial Design in Batteries
A07 New Developments and Applications of Electrode Binders for Rechargeable Battery and other Electrochemical Systems
A08 Interplay between Temperature and Battery Phenomenon 2
B
B01
B03 Carbon Nanotubes – From Fundamentals to Devices
B04 NANO in China
B05 Fullerenes – Endohedral Fullerenes and Molecular Carbon
B06 2D Layered Materials from Fundamental Science to Applications
B07 Light Energy Conversion with Metal Halide Perovskites, Inorganic/ Organic Hybrid Materials, and Dynamic Exciton
B08 Porphyrins, Phthalocyanines, and Supramolecular Assemblies
B09
of Fuels 9
I05 Crosscutting Materials Innovation for Transformational Chemical and Electrochemical Energy Conversion Technologies 6 I06 Energy Conversion Based on N, P, and Other Nutrients 4 I07 Advanced Manufacturing for High-Temperature Materials and Devices 2
I08 Flow Batteries: Beyond Vanadium
New
L03 Electrocatalysis 12: In Memory of Shimshon Gottesfeld
L04 Charge Transfer: Electrons, Protons, and Other Ions 6
L05 Invited Perspectives and Tutorials in Physical and Analytical Electrochemistry
L06 Development of Catalytic Systems and Mechanistic Understanding of Oxygen Electrochemistry
L07 New Horizons in Spectroelectrochemistry and Photoelectrochemistry
L08 Electrochemistry: Unusual Media, Novel Conditions
Sensors M01 Recent Advances in Sensors Systems 6
Biosensors, Lab-on-Chips, Point-of-Care Testing, In Vitro and In Vivo Bio-imaging 3
M03 AI, Machine Learning, and IoT for Sensors
Z02 Materials, Devices, and Systems for Neuromorphic Computing and Artificial Intelligence Hardware 2
Important Dates and Deadlines
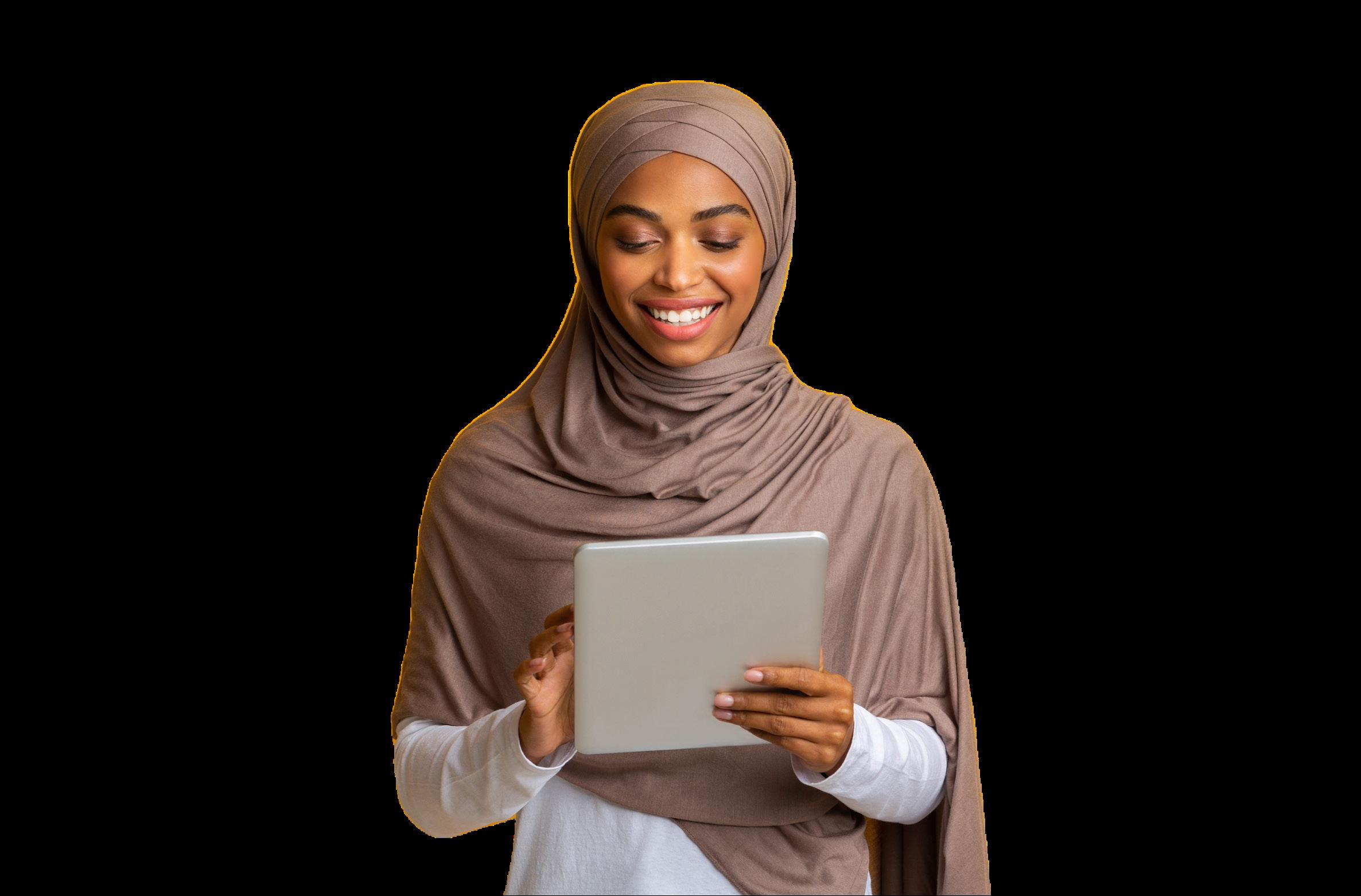
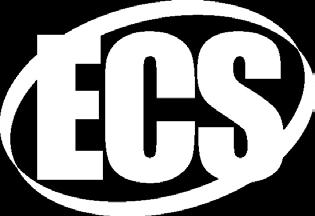
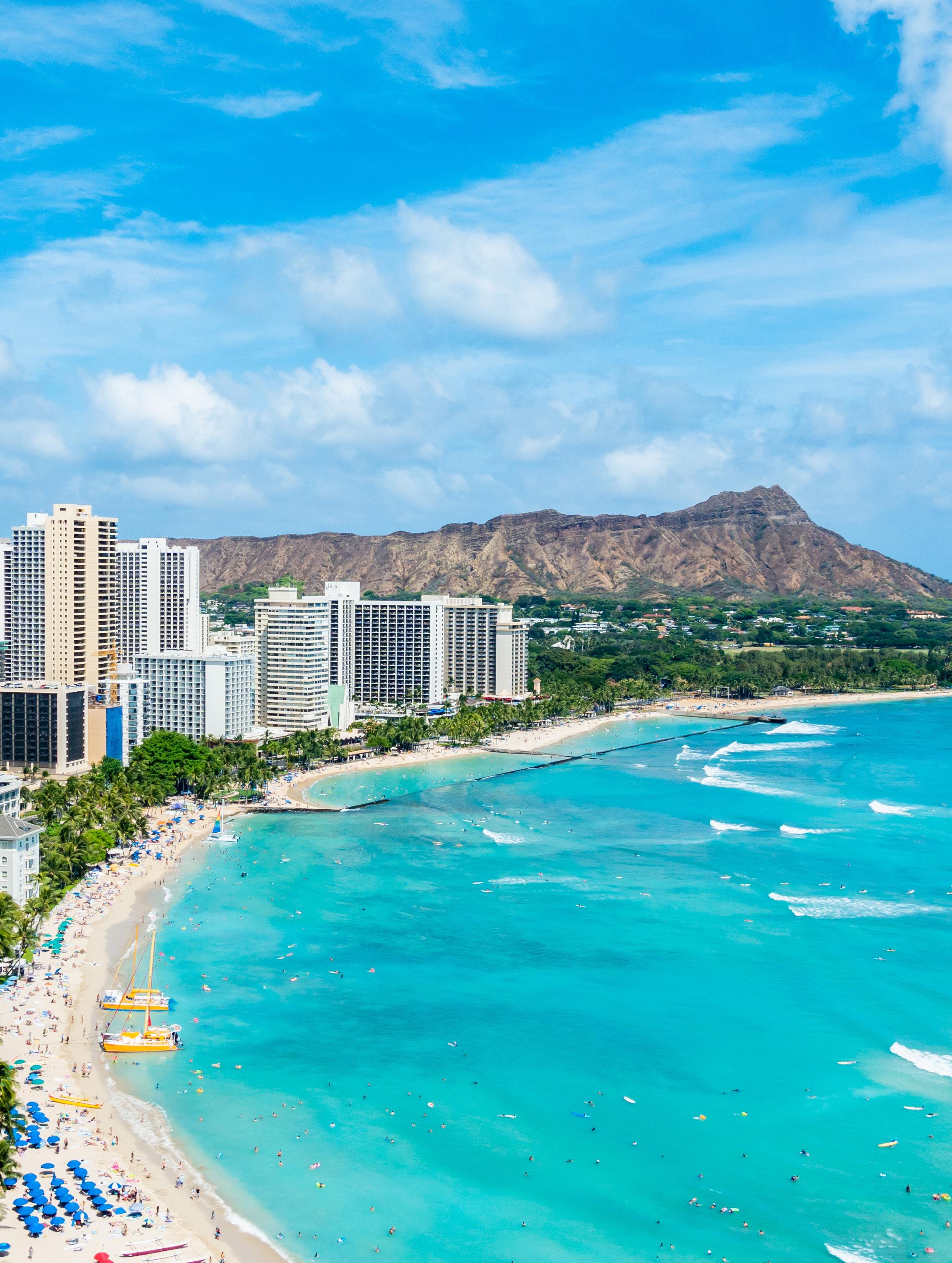
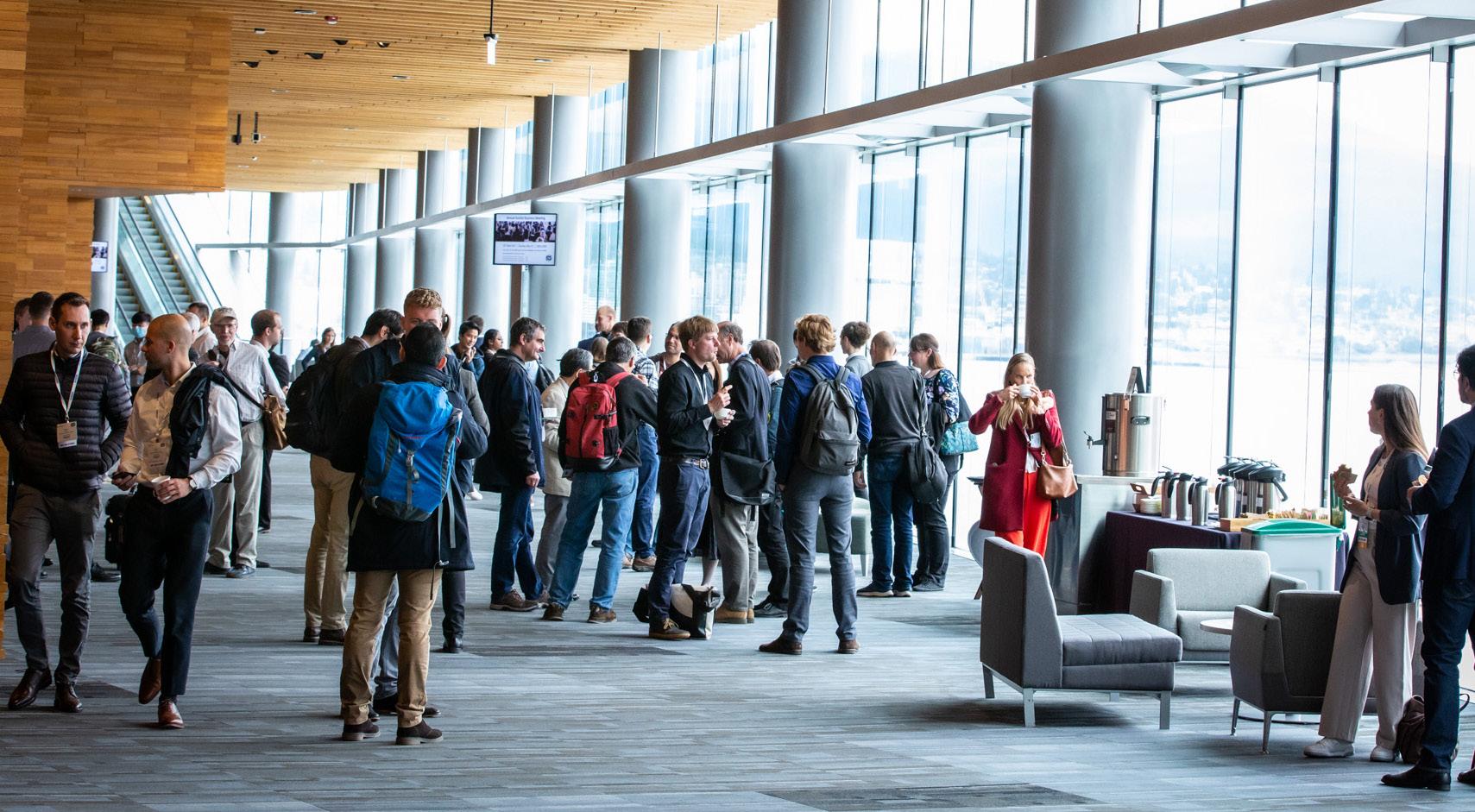
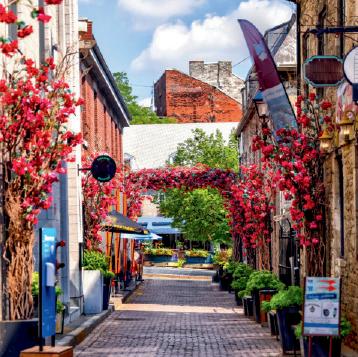
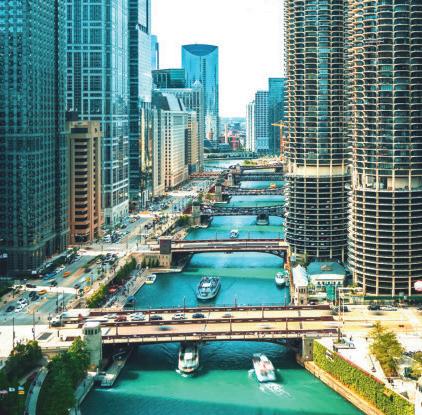
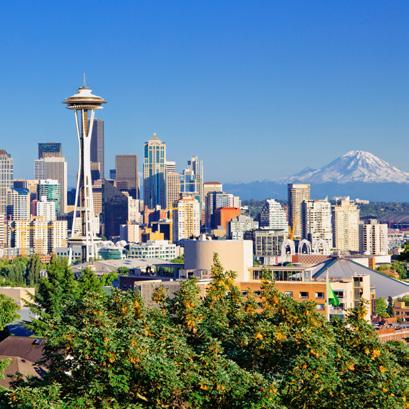
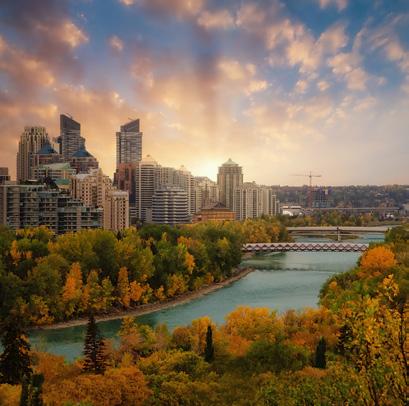
247th ECS Meeting
Montréal, Canada
May 18-22, 2025
Palais des Congrès de Montréal
248th ECS Meeting
Chicago, IL
October 12-16, 2025
Hilton Chicago
249th ECS Meeting
Seattle, WA
May 24-28, 2026
Washington State Convention Center
250th ECS Meeting
Calgary, Canada
October 25-29, 2026
BMO Centre
electrochem.org/meetings