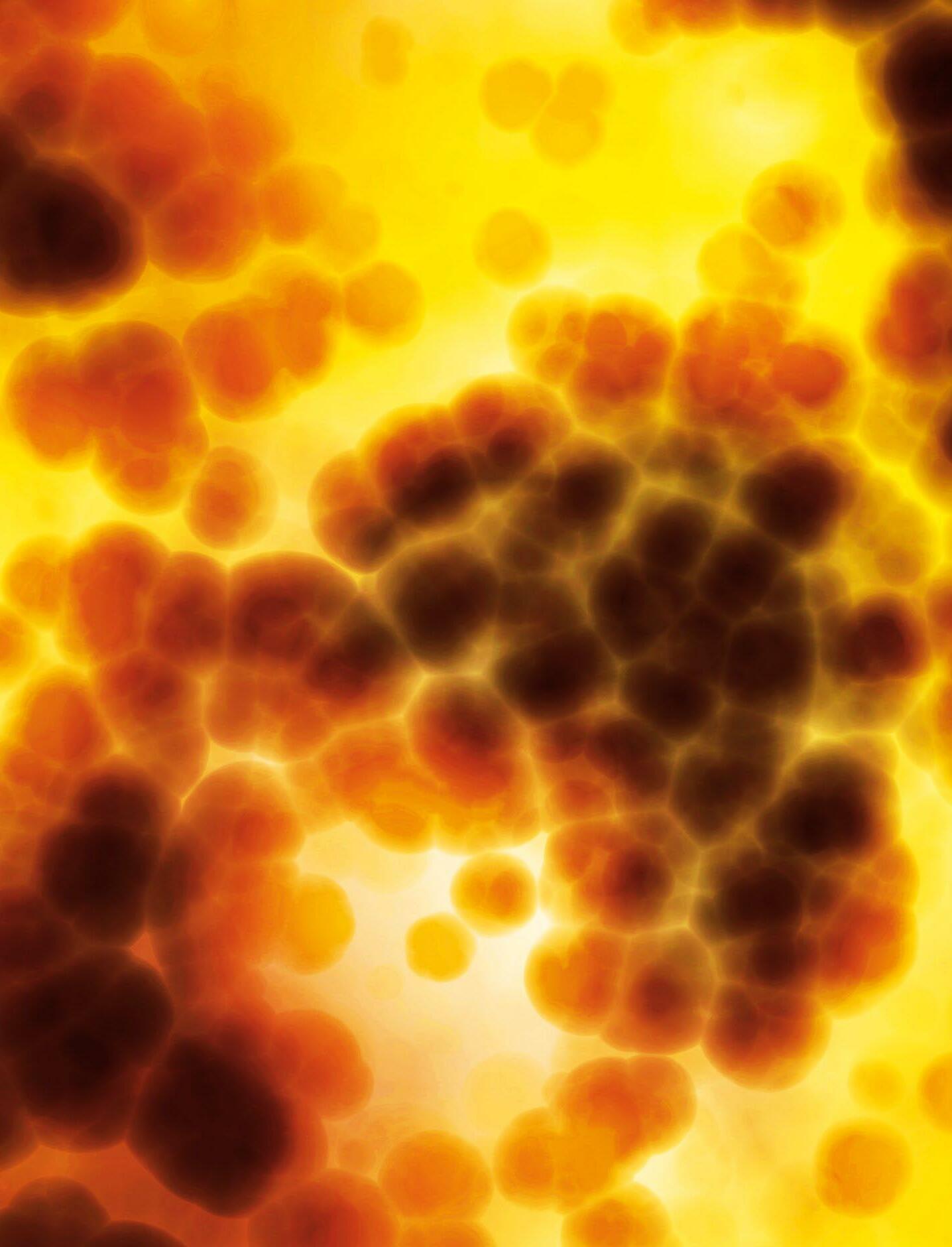
9 minute read
Nanoelectroablation
Nanoelectroablation is a completely new approach to treating tumors that triggers apoptosis, the tumor cell’s own programmed cell death pathway. Once triggered, the tumor slowly shrinks and is scavenged by the immune system, initiating an innate immune response that attacks other tumors in mice, writes Dr Richard Nuccitelli of BioElectroMed Corp
Electrical pulse technology has been
applied to living cells since the 1970’s and was first used to generate transient increases in the permeability of a cell’s plasma membrane, a technique called electroporation. Researchers continue to investigate the capacity of electric fields to modify cells, with a particular focus at BioElectroMed on how the electrical properties of tissues can be utilized to detect and treat disease.
Electric fields
When electric fields on the order of 1 volt are applied across a biological membrane they cause a breakdown in the lipid bilayer of the cell’s plasma membrane. This results in a water-filled pathway across the lipid membrane that allows ions and other small molecules to cross the normally impermeable lipid bilayer.
Early studies in this area used pulsed electric fields in the microsecond and millisecond time domains, with field strengths on the order of 1 kV/cm. It was found that the pores that formed in cell membranes were transient and reversible unless the field strength was increased to 2-3 kV/cm, in which case irreversible pores were formed that killed cells by necrosis, where cell death is caused by the influx of external factors such as calcium ions.
It is now possible to apply much shorter electrical pulses than those used in electroporation, which opens up new possibilities in treatment. These shorter pulses, in the nanosecond time domain with electric field strengths of between 10-100 kV/cm, also introduce transient, water-filled defects across the plasma membrane. However, only molecules with a diameter less than 1 nanometre (nm) can cross the membrane through these transient nanopore pathways.
These nanosecond pulsed electric fields (nsPEFs) have profound effects on treated cells, with their most important characteristic being their ability to penetrate into the cell cytoplasm to permeabilize organelle membranes as well as the plasma membrane [1]. BioElectroMed is developing novel bioelectric approaches based on low energy, non-thermal, nsPEFs which penetrate into every cell and trigger apoptosis, the cell’s own programmed cell death pathway.
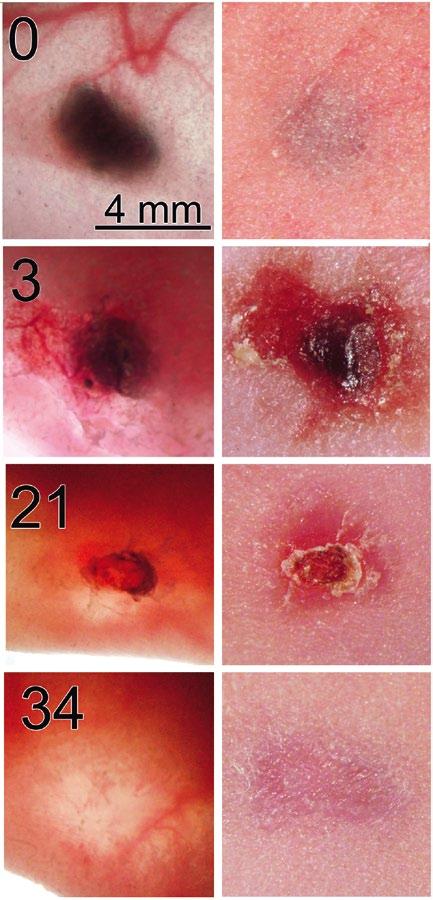
Figure 1. Melanoma treated on day 0 with 2000 pulses 30 kV/cm, 100 ns long. Left column shows transillumination image of tumor beneath nude mouse skin taken on the day after nsPEF treatment indicated in upper left of photo. Right column shows the reflected light image of the skin above the melanoma taken on the same day. The scale bar on the upper left applies to all images.
Unique Mechanism
All cells are bound by an outer plasma membrane, normally composed of a lipid bilayer with associated glycoproteins, which exhibits very low conductivity in comparison to the cytoplasm and extracellular fluid. This means the cell can be modelled as a conductor surrounded by an insulating layer, as is also generally true for organelles within the cytoplasm of cells.
When an electric field is imposed across a cell, ions in the cytoplasm respond by rapidly moving in the field direction to charge the capacitance of the membrane until they experience no further force. By definition, this will only occur when their redistribution establishes an equal and opposite field so that the net field in the cell interior is zero.
The duration of this redistribution is characterized by the charging time constant of the membrane, which is typically in the 0.1-1 microsecond (µs) range. Nanosecond pulsed electric fields (nsPEFs) are much faster than that so they penetrate into the cell before the charged molecules can redistribute to counteract the field. Once inside the cell, this field can generate nanopores in all organelle membranes if the field is large enough.
This ability to penetrate into the cytoplasm allows nsPEFs to permeabilize the organelle membranes as well as the plasma membrane, which is one of the

major differences between nsPEFs and earlier protocols (Fig. 2). However, the varying sizes of the organelles also need to be taken into account in this work.
Organelle sizes range from the large nucleus, between 2 and 6 micrometers (µm), to the much smaller endoplasmic reticulum tubular network and mitochondria in the 0.3-1 µm range. Once the field has penetrated the cytoplasm, fields of 1 volt over 0.3-1 µm (10-33 kV/cm) are required to achieve the permeabilizing field of 1 volt across these smaller organelles. These strengths are an order of magnitude higher than those used for the original electroporation work using microsecond and millisecond pulse lengths.
Small amount of energy delivered
The energy delivered to the cell is an important consideration when applying such large electric fields. As these nanosecond pulses are 1,000 times shorter than the classical electroporation pulses, the duration of energy delivery is also lower by a factor of 1,000. So even when a 10-fold higher amplitude is applied, the energy delivered is only 2.17 Joules, which will increase tissue temperature by only 0.5º C.
Therefore the nsPEF is able to both penetrate into the cytoplasm and also apply a field strength large enough to influence organelle permeability, two critical features which make it a very powerful tool. The focus now is on using these features to improve treatment; BioElectroMed has been developing a non-thermal therapy to ablate tumors implanted in mice with a single treatment, and the results to date are impressive.
Nanoelectroablation Evidence
Work started with the B16 murine melanoma allograft model system, in which murine melanoma cells injected beneath the skin grow into a melanoma that can be treated with nsPEF. It was found that a single treatment of 2000 pulses, each 100 ns in duration and 30 kV/ cm in amplitude, was sufficient to ablate these tumors [2]. (Fig. 1).
The goal in this work was to study the effectiveness of nsPEFs in treating more naturally occurring melanomas, which led to a collaboration with Ed DeFabo of
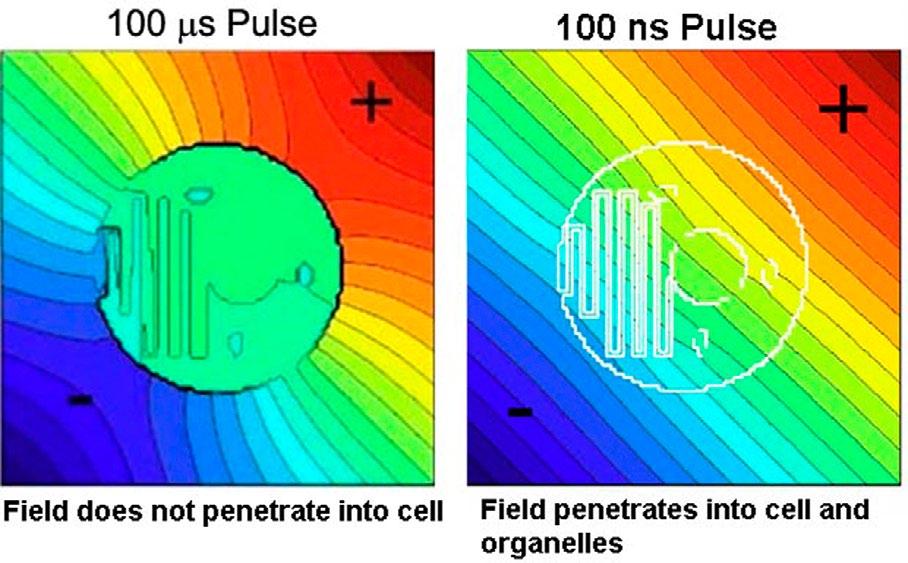
Figure 2. Electric field distribution in and around a cell during a 100 µs pulse (left) and a 100 ns pulse (right). For a 100 ns pulse the field penetrates into the cell and every organelle to generate small water-filled pores in the organelle membranes.
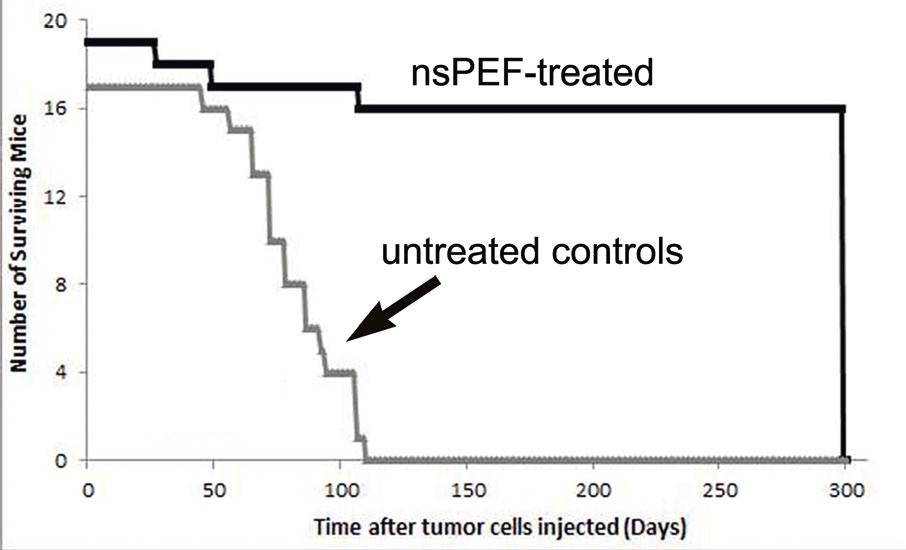
Figure 3. Survival curve for nude mice with a single human pancreatic carcinoma xenograft tumor. Tumors in 19 mice were treated with 2000 pulses, 30 kV/cm, 100 ns long. Tumors in 17 mice served as untreated controls. All of the nanoelectroablated tumors disappeared without recurrence for the 300 days tested. All of the control tumors grew so large that the mice had to be sacrificed.

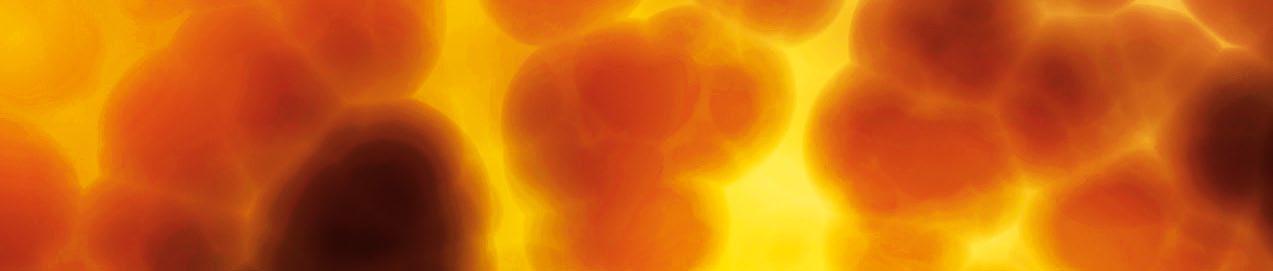
George Washington University, who had developed a UV-induced melanoma model using C57/BL6 HGF/SF mice. All 27 melanomas treated in 14 of these mice were successfully ablated using 2000 pulses, each of which were 100 ns long and 30 kV/cm in amplitude[3].
This treatment was called ‘non-thermal nanoelectroablation’ and it was found to have significant effects on melanomas. All nanoelectroablated melanomas gradually disappeared over a period of 1229 days. Pyknosis of the nuclei was evident within an hour and DNA fragmentation as detected by TUNEL staining was evident within six hours of nsPEF treatment, while other effects were also observed. day of treatment. After four weeks they were 99.8% ablated if the tumor was located within the ablation zone. Pyknosis was evident within two days of nsPEF treatment and DNA fragmentation as detected by TUNEL staining was also found.
The biologists at BioElectroMed have been ablating xenograft tumors created by injecting human pancreatic carcinoma cells beneath the skin of immunosupressed mice. After treating dozens of human pancreatic tumor cells with a range of pulse numbers, they determined that 500 pulses of 100 ns duration and 30 kV/cm amplitude were sufficient to ablate these tumors completely with a single treatment.
It is this ability to penetrate into the cytoplasm and to permeabilize the organelle membranes
as well as the plasma membrane which provides one of the major distinctions between nsPEFs and other electroporation treatments.
BioElectroMed also demonstrated that nanoelectroablation stimulated an immune response that actually inhibited secondary tumor formation in an allograft model. Other groups have also reported that apoptotic cells can trigger an immune response. BioElectroMed is continuing research in this area and has found that secondary tumors injected into mice whose original tumors had been nanoelectroablated are invaded by natural killer T cells.
Another more naturally occurring skin cancer model is the basal cell carcinoma (BCC) Ptch1+/-K14-Cre-ER p53 fl/fl mouse model, which was developed by Dr Ervin Epstein of the Children’s Hospital Oakland Research Institute. These BCCs developed naturally on the skin of mice that were treated as pups with ionizing radiation. Twenty-seven of these BCCs were treated and all began to shrink within a
They also conducted a long-term study of 19 mice in which a single tumor was nanoelectroablated and observed over a period of at least 300 days (Fig. 3). No tumor recurrence was detected over this period, whereas untreated control tumors continued to grow and we were forced to sacrifice all control mice within 110 days.
Human carcinomas
The ultimate goal of this research is to use nanoelectroablation to treat human carcinomas, and indeed an initial clinical trial has already been held treating three patients with multiple BCCs.
Seventy percent of the BCCs were eliminated with a single treatment and no scarring occurred. These very interesting data confirm that nanoelectroablation is also an effective therapy for treating human tumors.
Full Project Title
Nanosecond pulsed electric fields trigger apoptosis in treated tumors (Nanoelectroablation)
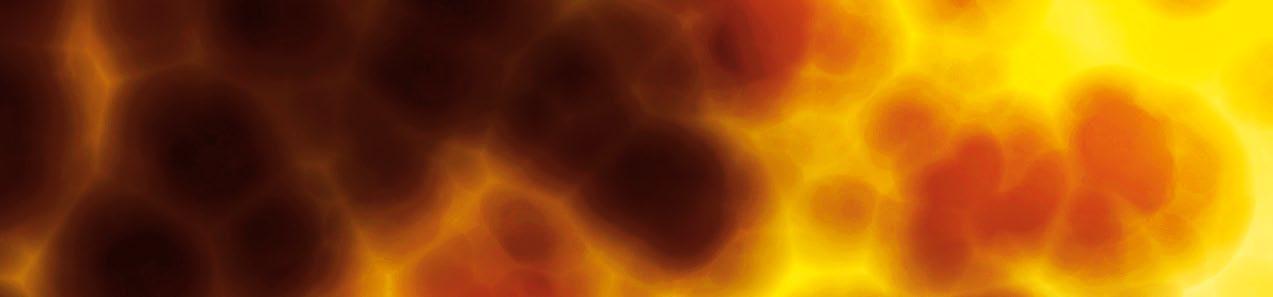
Project Objectives
BioElectroMed is a biotech company developing medical devices for the detection and treatment of cancer. The company is developing low energy, non-thermal, nanosecond pulsed electric fields, which penetrate into every cell and organelle located between the delivery electrodes and trigger apoptosis, the tumour cell’s own programmed cell death pathway.
Contact Details
Project Coordinator, Richard Nuccitelli, Ph.D. Vice President BioElectroMed Corp. 849 Mitten Rd., Suite 104 Burlingame, CA 94010 E: rich@bioelectromed.com W: www.bioelectromed.com
[1] Schoenbach KH, Beebe SJ, Buescher ES. Bioelectromagnetics 2001; 22(6):440-448. [2] Nuccitelli R, Tran K, Sheikh S, Athos B, Kreis M, Nuccitelli P. Int J Cancer 2010; 127(7):1727-1736. [3] Nuccitelli R, Tran K, Lui K, Huynh J, Athos B, Kreis M et al. Pigment Cell Melanoma Res 2012; 25:618-629.
Dr Richard Nuccitelli
Project Coordinator
Richard Nuccitelli is a biophysicist who received his M.S. degree in Physics and his Ph.D. in Biological Sciences from Purdue University. He has been a professor at both the University of California at Davis and at the Frank Reidy Research Center for Bioelectrics at Old Dominion University. He cofounded BioElectroMed with Pamela Nuccitelli in 2001.
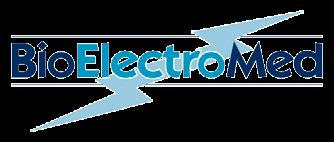