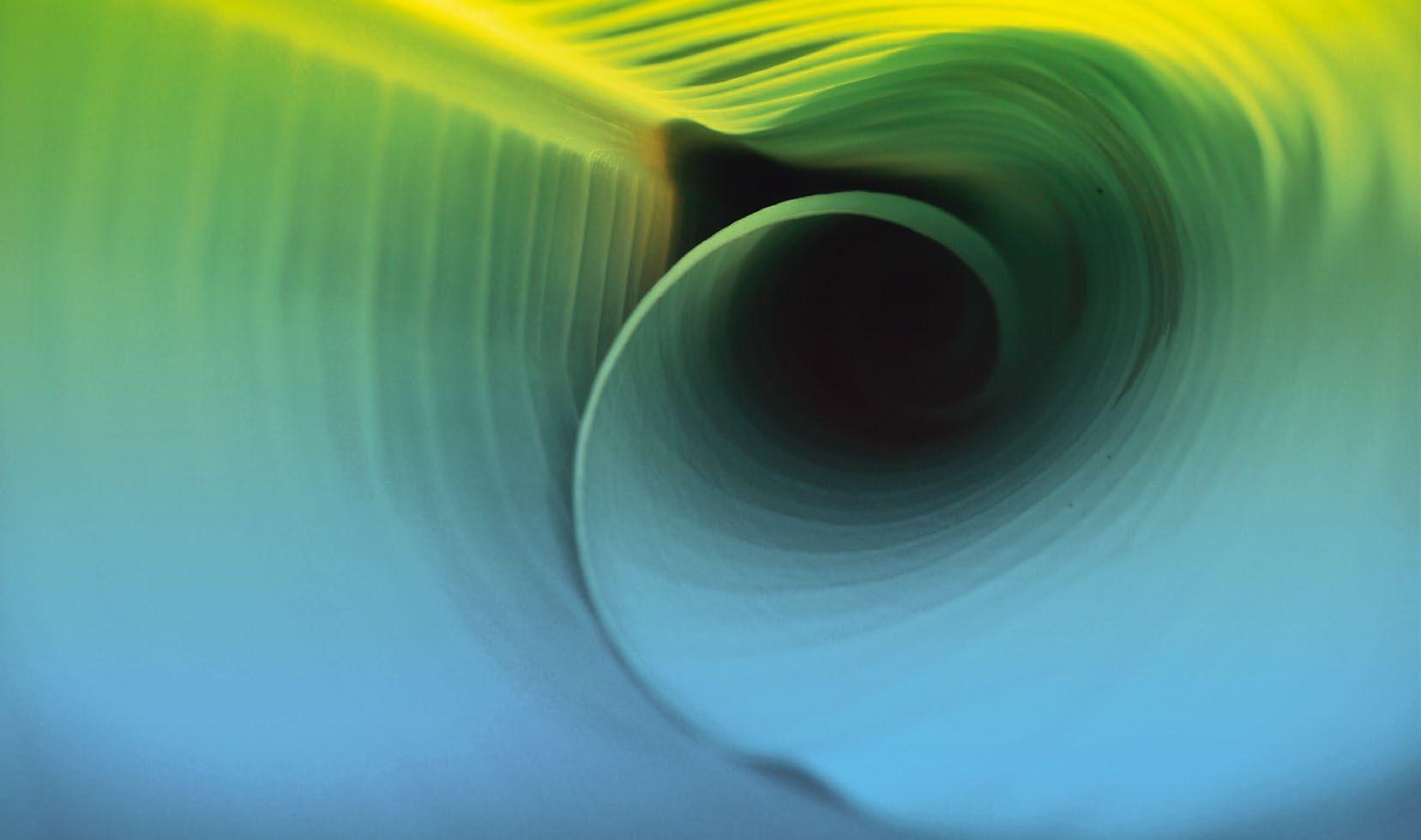
9 minute read
Light4Function
The creative power of chemistry lies in the ability of scientists to shape matter in unprecedented ways and generate new substances and materials with unique and advantageous properties. Now, researchers in the Light4Function project are looking to the next step, aiming to use light to control the timing and location of chemical and physical processes, as Professor Stefan Hecht explains
Researchers shine new light on chemical processes
There is a long history of chemists designing sophisticated molecules to create new pharmaceuticals and novel materials. This work has underpinned a great deal of technical and industrial development, but the level of sophistication is still way behind mother Nature’s machinery. Now researchers in the Light4Function project are looking to the next step, aiming to develop new methods to remotely yet precisely control chemical processes. “We are investigating the use of light as a trigger to control where and when chemical reactions take place,” explains Professor Stefan Hecht, the project’s Principal Investigator. The project aims to design and implement new functional photoswitchable systems, drawing inspiration from the natural world. “Nature uses stimuli – such as the presence of a messenger molecule, for example a hormone, or light – to trigger an event. The latter offers great advantages since light can be applied with exquisite spatio-temporal as well as energetic resolution – it’s the ideal remote-control,” says Professor Hecht.
This work is inspired by observations of the natural world, where light triggers and drives important processes. Professor Hecht points to the example of photosynthesis. “In photosynthesis, light generates a proton gradient across a membrane, and that drives synthesis of adenosine triphosphate,” he explains. With modern spectroscopy techniques, it is now possible to pulse light at very high frequencies, which allows researchers to control the timing of a chemical reaction using light. “Nowadays you can pulse light
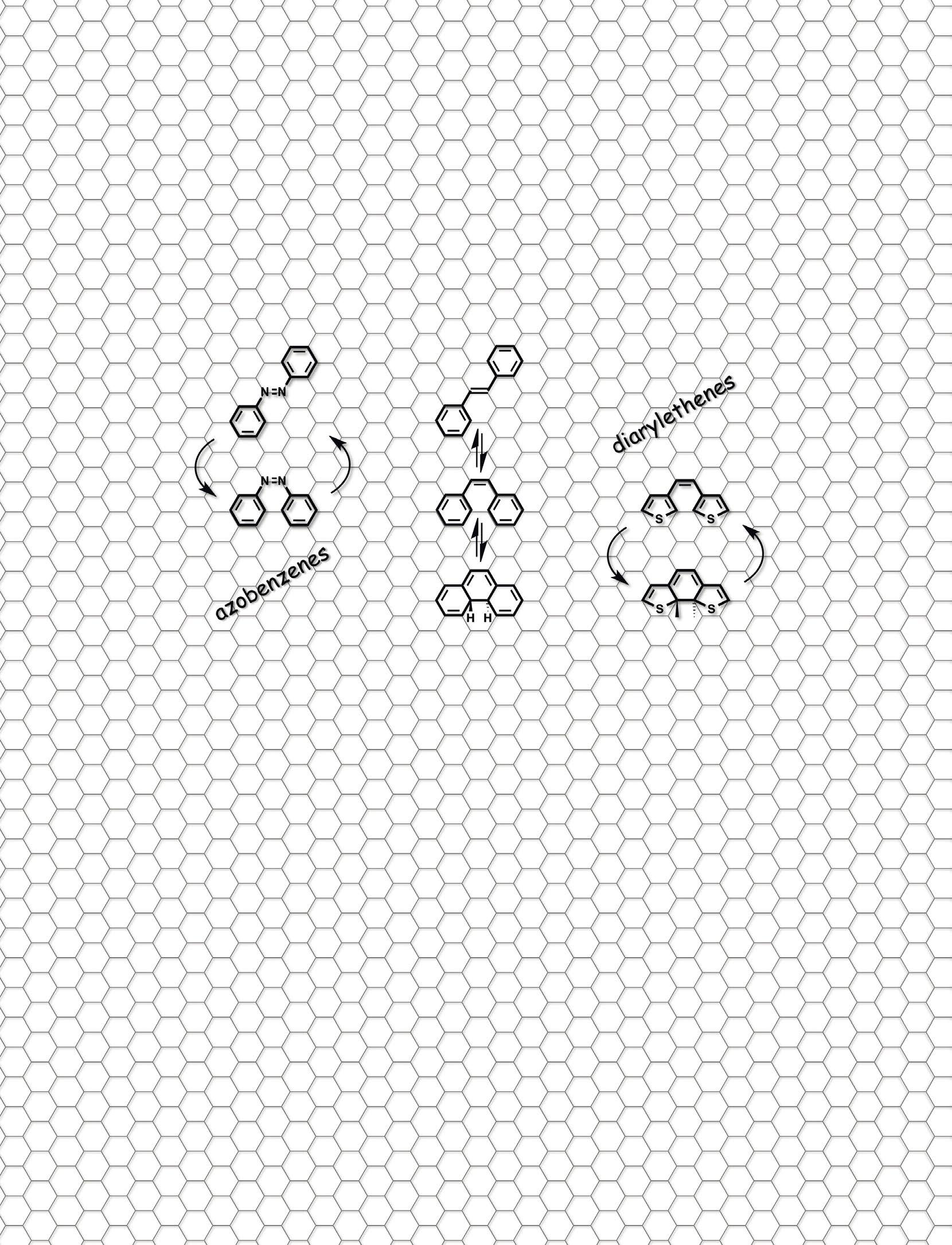
at the atto-second frequency (10-18 seconds). Even fast chemical processes occur on a timescale longer than 10-15 seconds and typically chemistry is much slower as it is limited by diffusion, which occurs at 10-10 seconds. So light gives you absolutely exquisite control over time and we can initiate a reaction very precisely,” outlines Professor Hecht. “For spatial resolution, light is inferior to techniques such as Atomic Force Microscopy and Scanning Tunnelling Microscopy, but with state-of-the-art equipment you can go well below the so-called diffraction limit.”
Photoswitches
Light can also be applied externally, in a non-invasive manner, offering a way to precisely control the outcome of a chemical reaction without adding a chemical. However, one component has to interact with the light; Professor Hecht and his colleagues hold deep expertise in this area, particularly in the design of a specific type of molecules, so-called photoswitches.
“These molecules can exist in two different forms. Light can turn the molecules from one form into another. We want these two forms to behave very differently – like Dr. Jekyll and Mr. Hyde – because we want to exploit their differences to regulate various chemical and physical processes,” he says. The project’s agenda includes fundamental research into these photoswitches, of which there are several different types. “We are looking to both design new photoswitches and improve existing photoswitches. We want them to switch reliably and very efficiently, ideally upon exposure to
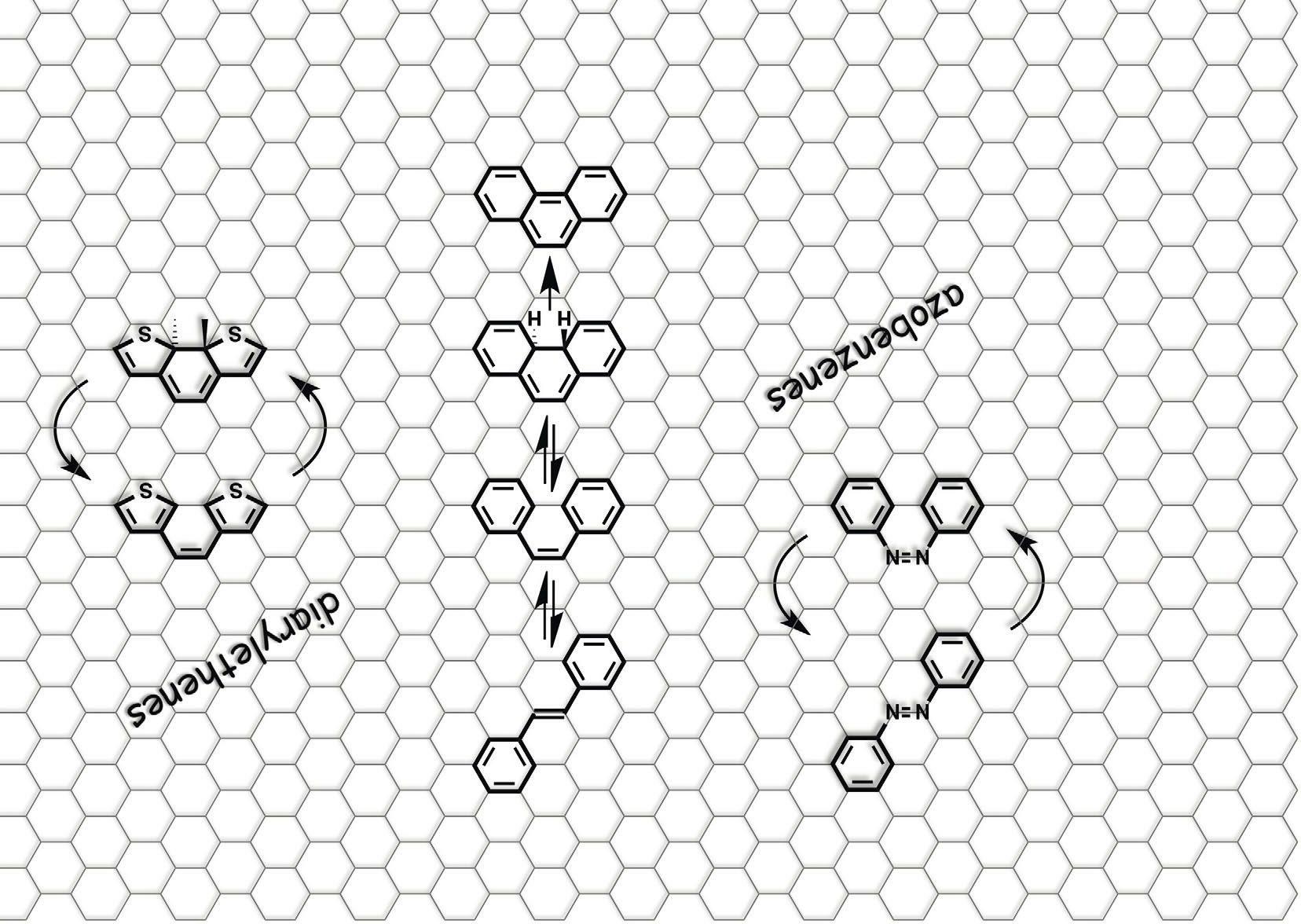
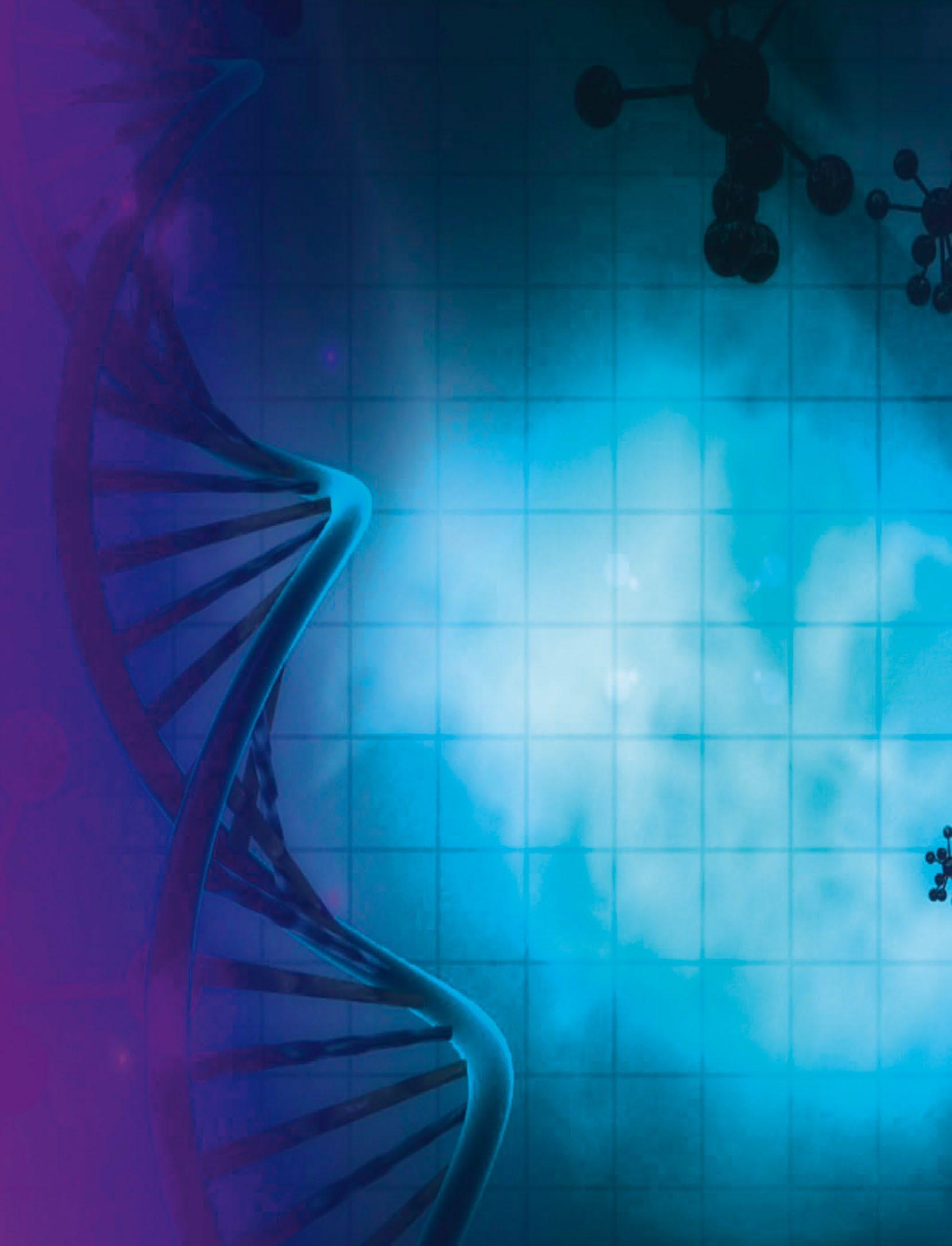
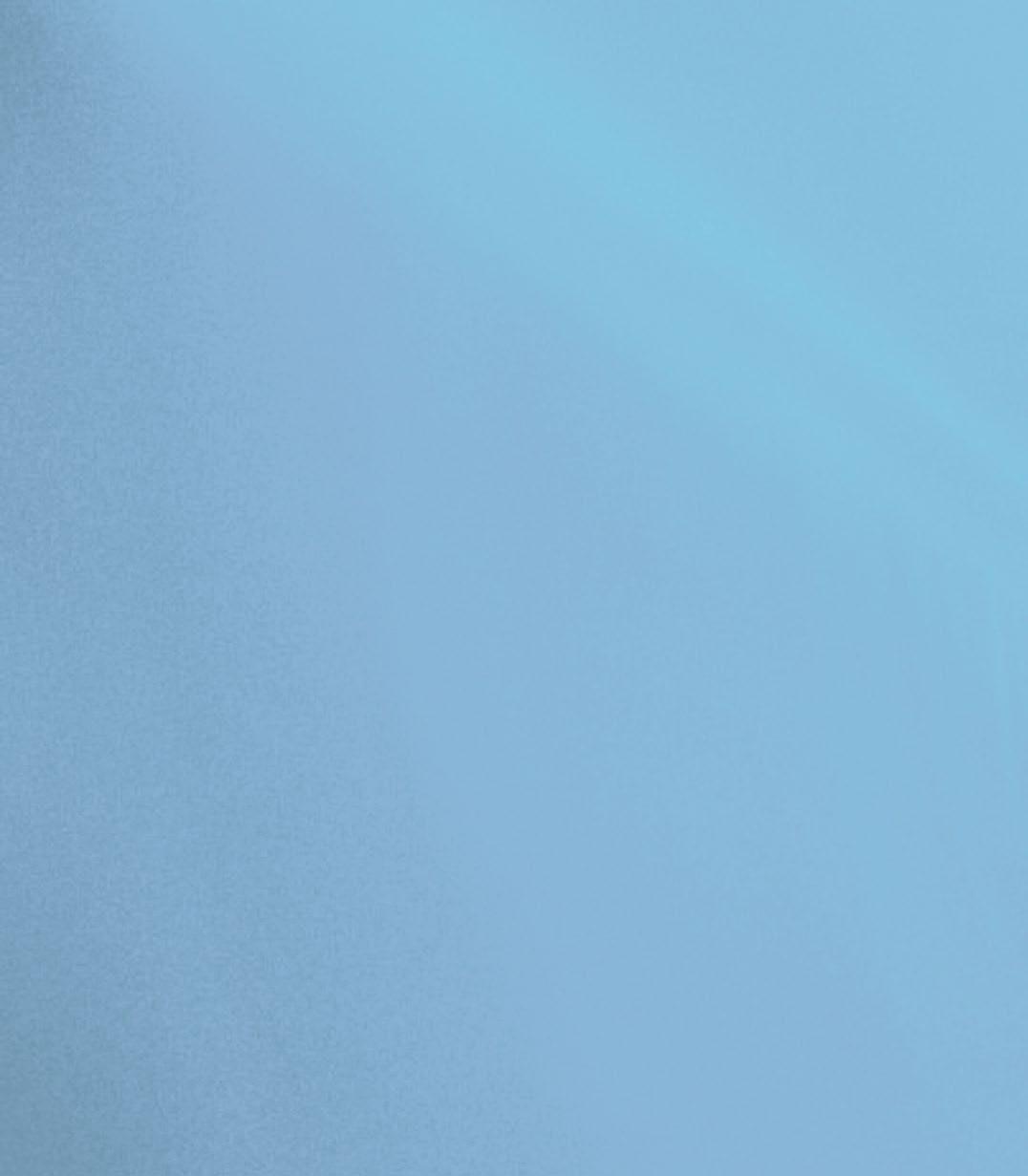
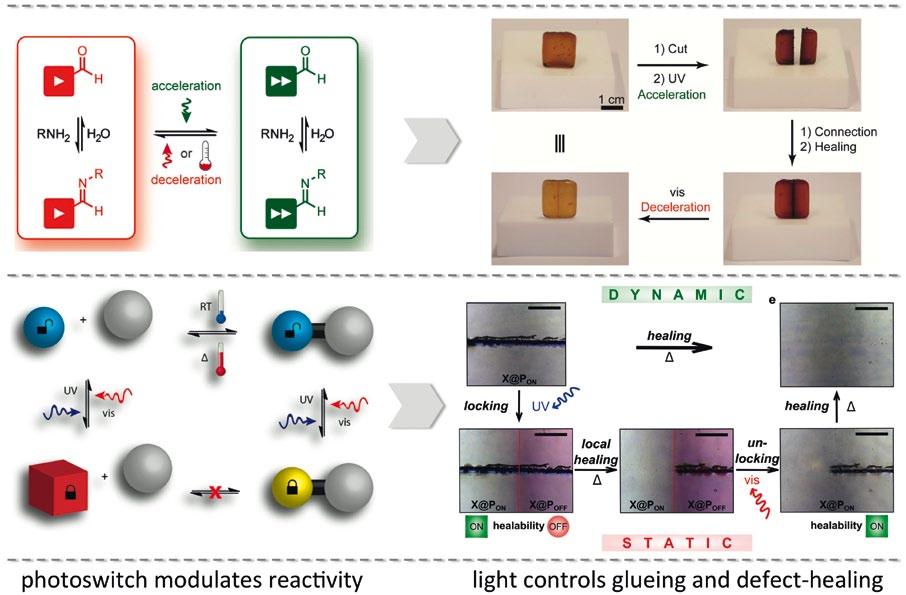
sunlight,” continues Professor Hecht. “This is important for people working in photopharmacology in particular. We want to push the wavelength very far into the red part of the spectrum, so that it can penetrate the skin.”
Using the different properties of the two switch forms, it is possible to precisely modulate local chemical reactivity. This should impact a number of fields, including medicine. “In treatment, you want to make sure that a drug only kills unhealthy cells and not the healthy ones – this selectivity is a big problem in chemotherapy for example,” explains Professor Hecht. With precise spatial control over the location of a chemical reaction, the drug in its inactive form could be located close to a tumor, at which point the drug could be optically switched into its active form. “However,” notes Professor Hecht “There is no such universal molecule that can be used across all different chemical processes. We always need to optimize the photoswitch for its intended purpose.”
Researchers in the project aim to target several different processes using these photoswitches, alongside their fundamental research into improving their properties. The first aspect that researchers investigated was control over chemical connections, so bond-making.
Figure 2 (Right). Photoswitches, which allow to modulate current flow through organic semiconductors and transistor devices made thereof (left), enable the design of fast and flexible optical memories (right). Related publications: Nature Chemistry 2012, 4, 675-679 and Nature Nanotechnology 2016, 11, 769-775. Figure 1 (Left). Photoswitches, which allow for modulation of their reactivity in exchange reactions (top left) as well as for locking adducts (bottom left), can be employed as crosslinkers to enable light-controlled glueing (top right) and local scratch healing (bottom right) of polymers. Related publications: Angewandte Chemie International Edition 2016, 55, 13882-13886 and Nature Communications DOI: 10.1038/ncomms13623.
“Here we follow two conceptually different approaches: in the first, we employ the switch itself as a starting material in the chemical reaction. But then for every connection that you want to make, you have to use one photon. So if you want to connect one thousand molecules, you will need at least one thousand photons,” explains Professor Hecht. In the second area of the project, the researchers are investigating lightcontrolled catalysis. “In this case, the switch would be integrated in the catalyst, and because the catalyst can turn over more than one substrate molecule, we get a regime where we can make a thousand molecules while only using a few photons,” says Professor Hecht. “This is the main difference between these two project areas.” The researchers have already exploited this fundamental work on light-controlled bond-making and bond-breaking to prepare polymeric materials which can be glued and healed - they even self-heal upon exposure to sunlight (Figure 1).
Physical processes
Beyond chemistry, the project is also trying to control various physical processes with light, most importantly charge transport. Professor Hecht collaborates closely with Professor Paolo Samori of the University of Strasbourg. “He is an expert on electronic devices using transistors made from organic molecules,” says Professor Hecht. In this case, photoswitches have been integrated into so-called organic thin film transistors (OTFTs), which are used in several different electronic devices, including computers, mobile phones, and television screens (Figure 2). “Some companies are working on optical memories that use this kind of technology,” continues Professor Hecht. “Here we can exploit the very different electronic properties, not chemical properties. In one form, they would trap a charge, while in the other the switch doesn’t interfere with charge transport. Thus, we can turn the transistor on and off at the speed of light.”
A fourth area of investigation in the project centers around light-driven molecular motion, where the researchers try to convert light directly into motion. The key challenge here is translating light-induced molecular changes into macroscopic movements. “We developed molecules that were very good at changing their shape under sunlight. Then, we collaborated with Professor Albert Schenning and his colleagues at TU Eindhoven and they integrated these molecules into a special
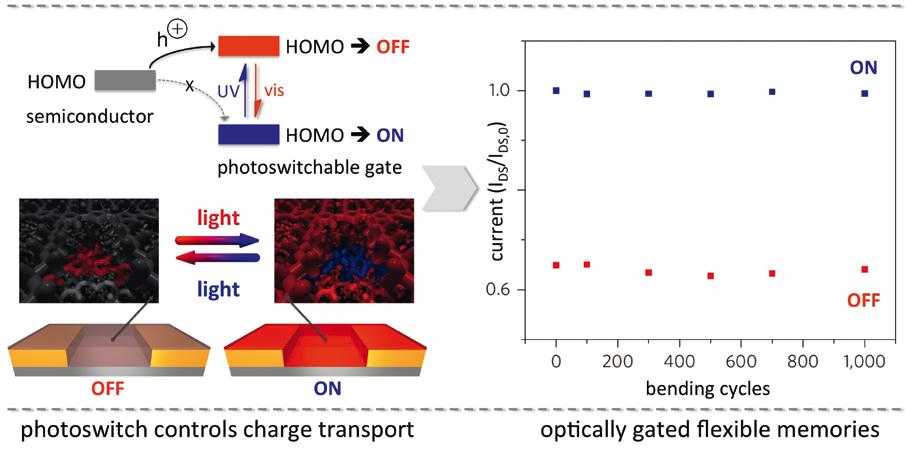
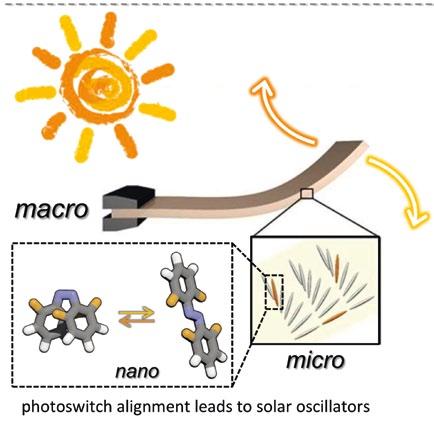
plastic film, in which the switch molecules are now aligned and hence organized, such that they all change their shape and work in concert. If you put these plastic films in sunlight, they chaotically bend up and down
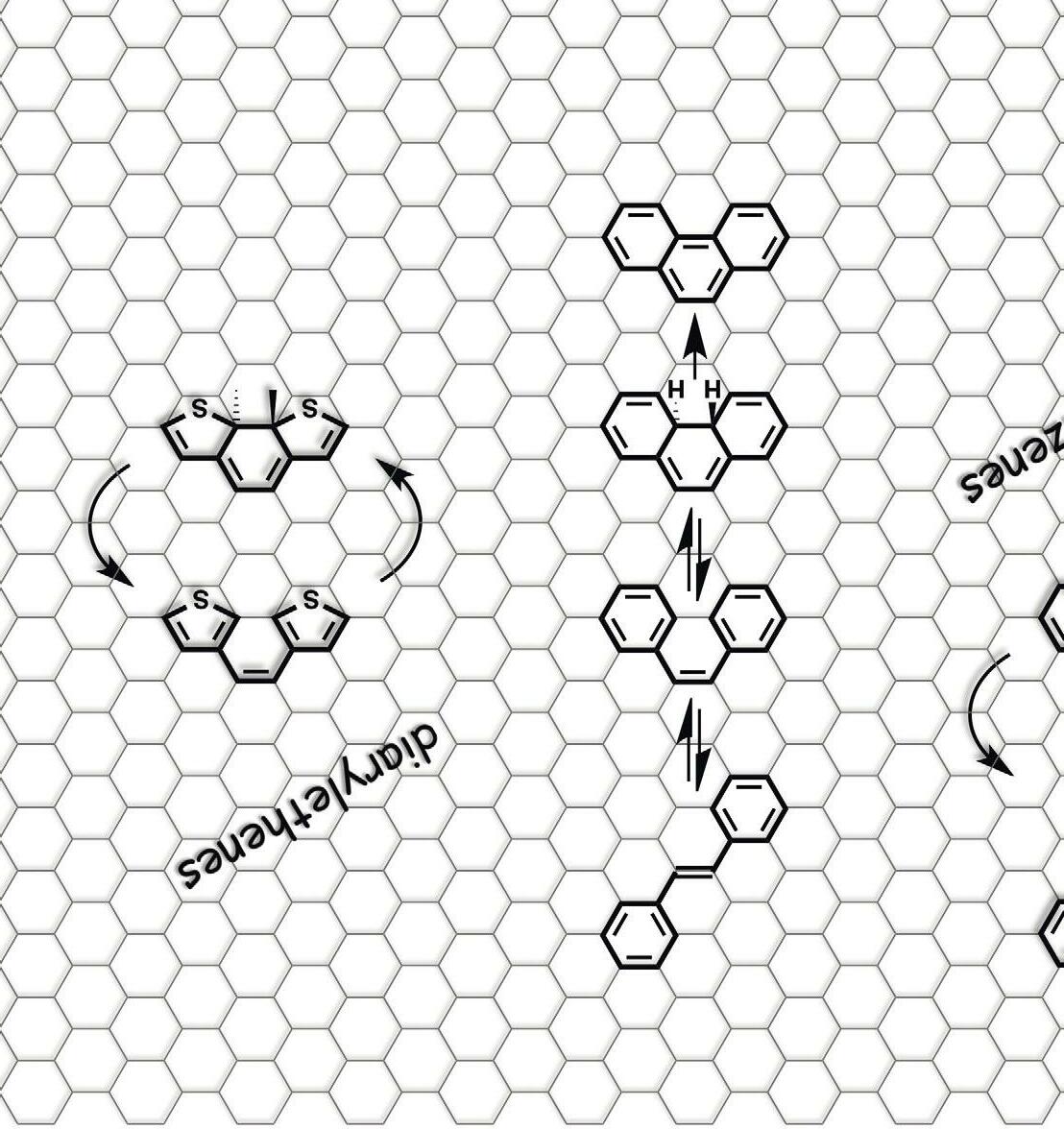
- they oscillate,” outlines Professor Hecht. These properties could prove useful in selfcleaning surface coatings, for example for solar cells, notes Professor Hecht (Figure 3). “The efficiency of solar cells declines over time simply because they get dirty,” he explains. “Cleaning them costs money and can destroy them, so it would be very exciting if we could use part of the light hitting the solar cell to brush away dust because of a smoothly vibrating surface.” Figure 3 (Left). Photoswitches, which undergo large geometrical changes upon exposure to visible light (bottom left), can be aligned in polymer films (bottom right) that oscillate in sun-light (top right). Related publication: Nature Communications 2016, 7, 11975.
bring significant benefits is in biomedical applications. “We have made some molecules that are highly relevant for people who want to go in the biological direction. We have successfully made these molecules that switch using visible light and red light, and beyond this we have even achieved two-photon induced switching,” outlines Professor Hecht.
This latter area represents a way of improving the resolution in space, using non-linear optical effects. “The idea is that a process only happens when a certain light
Light can turn the molecules from one form into
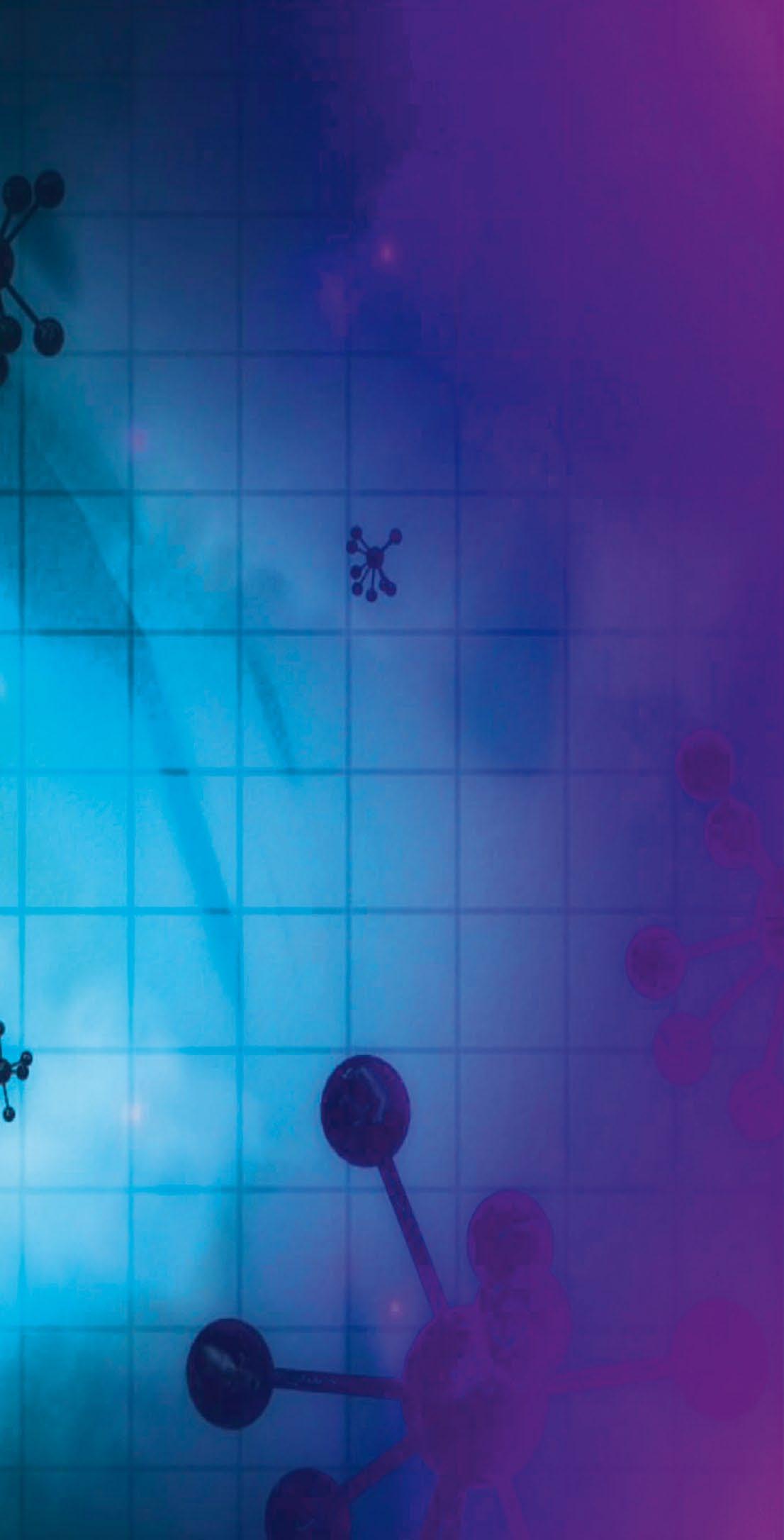
Potential applications
The primary focus of the project has been on the development of new photoswitchable systems, but Professor Hecht is well aware of the wider potential of this research, and is keen to explore applications outside the academic sphere. One area in which these new photoswitchable molecules could intensity threshold has been reached,” explains Professor Hecht. “You could then think about two laser beams intersecting each other in a very small, very precise spot, and only in this tiny spot would the intensity be high enough that the molecules could absorb two photons.” Recently Professor Hecht and his colleagues have developed photoswitches which could be switched with two photons. “In the biological context, our basic research is about laying the molecular foundation for the areas in which photoswitches could eventually be used. This work is highly relevant for the bio community,” he outlines. “We don’t use photoswitches in the project to regulate drug action for example, it’s not our primary focus, but that’s one promising area in which they could eventually be applied.”
Full Project Title
Light-controlled and Light-driven Molecular Action (Light4Function)
Project Objectives
Light4Function aims to develop and optimize photoswitchable molecules as tools to utilize light to control and drive various chemical and physical processes. These range from remote-controlling bond making and breaking and catalysis, relevant for the creation of adaptive materials, to optically programming charge transport in electronic devices and light-driven actuation.
Contact Details
Professor Stefan Hecht Laboratory of Organic Chemistry and Functional Materials, Department of Chemistry, Humboldt-Universität zu Berlin Brook-Taylor-Str. 2 12489 Berlin Germany T: +49 (0)30 2093 7365 E: sh@chemie.hu-berlin.de W: www.hechtlab.de
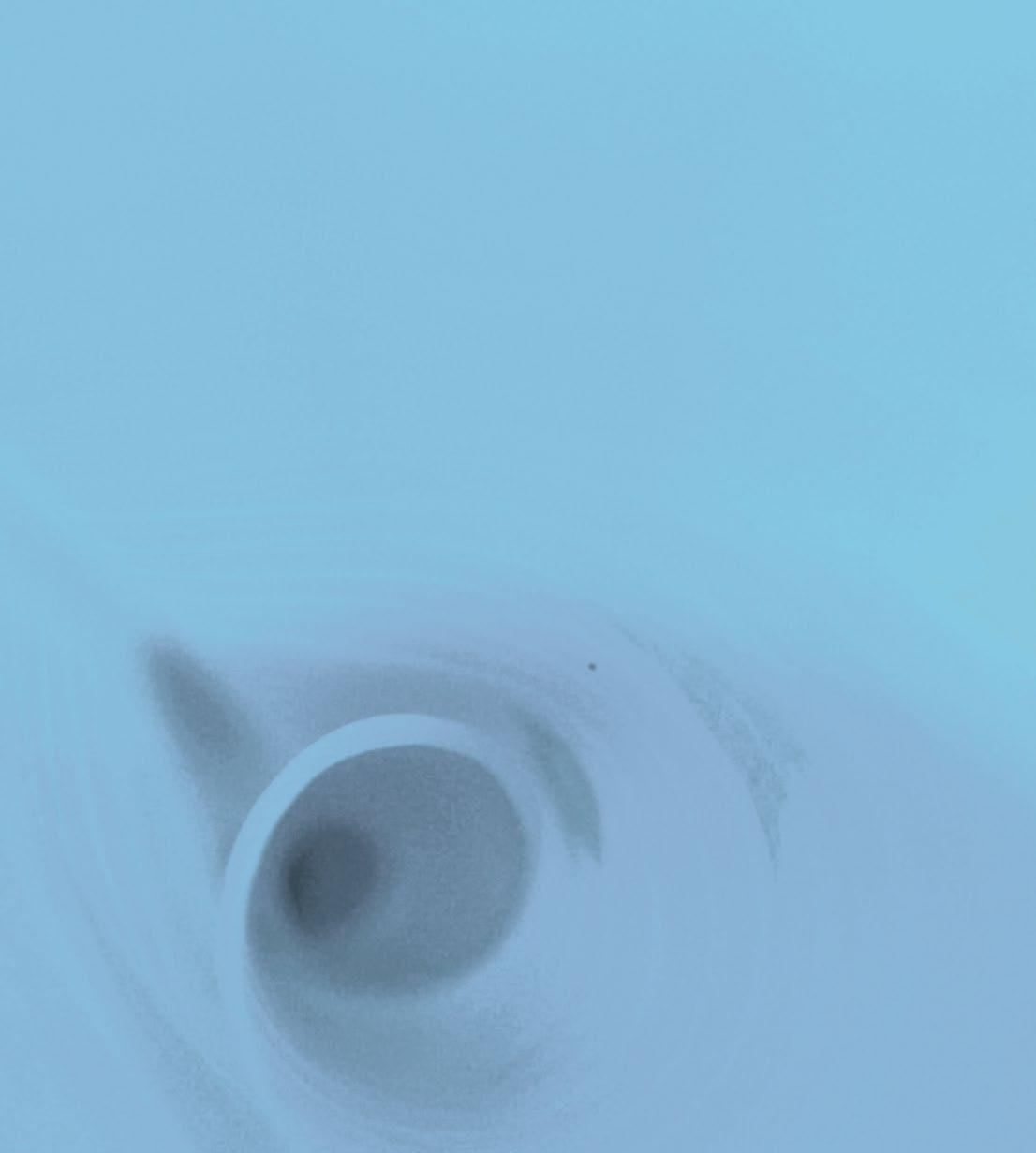
Göstl, Senf, & Hecht: “Remote-controlling chemical reactions by light: Towards chemistry with high spatio-temporal resolution” Chem. Soc. Rev. 2014, 43, 1982-1996. Bléger & Hecht: “Visible-Light-Activated Molecular Switches” Angew. Chem. Int. Ed. 2015, 54, 11338-11349.
Professor Stefan Hecht
Professor Stefan Hecht is heading the Laboratory of Organic Chemistry and Functional Materials at the Department of Chemistry of Humboldt-Universität zu Berlin. His research interests range from synthetic macromolecular and supramolecular chemistry to surface science, with particular focus on utilizing photoswitchable molecules for remote-controlling materials, devices, and processes.