Natural catalysts for a sustainable future
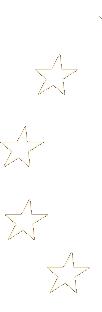
As natural and biodegradable agents, enzymes are key to advancing sustainable, green manufacturing practices across diverse sectors. These natural proteins/catalyst facilitate oxidation reactions by transferring oxygen or electrons and speed up oxidation reactions so that a wide variety of products can be made without using high temperatures or harsh chemicals, as Professor John Woodley explains.
The majority of oxidation reactions carried out in industrial chemistry involve the use of environmentally harmful oxidants, while the reactions themselves are not particularly selective. This means many of these reactions are not sustainable. Nevertheless, oxidations are amongst the most important chemical reactions in industry. Fortunately, enzymes found in nature could provide a more sustainable way of catalysing some of these reactions (for example in producing pharmaceuticals), and researchers are exploring their wider potential. “There is a lot of interest in using enzymes to carry out and catalyse reactions in industrial reactors and process plants,” explains John Woodley, Professor of Chemical and Biochemical engineering at the Technical University of Denmark (DTU), and Principal Investigator of the FLUIZYME project. Enzymes catalyse a wide variety of reactions in the natural world, but they may lose their structure and lose stability in an industrial reactor, which will then affect how they function. “Enzymes are large molecules, made up of lots of amino acids, which form into a particular structure (or shape). Enzymes need to maintain their structure in order to maintain stability, “ says Professor Woodley. “And when an enzyme loses stability, it can no longer perform at the same level as it did initially.”
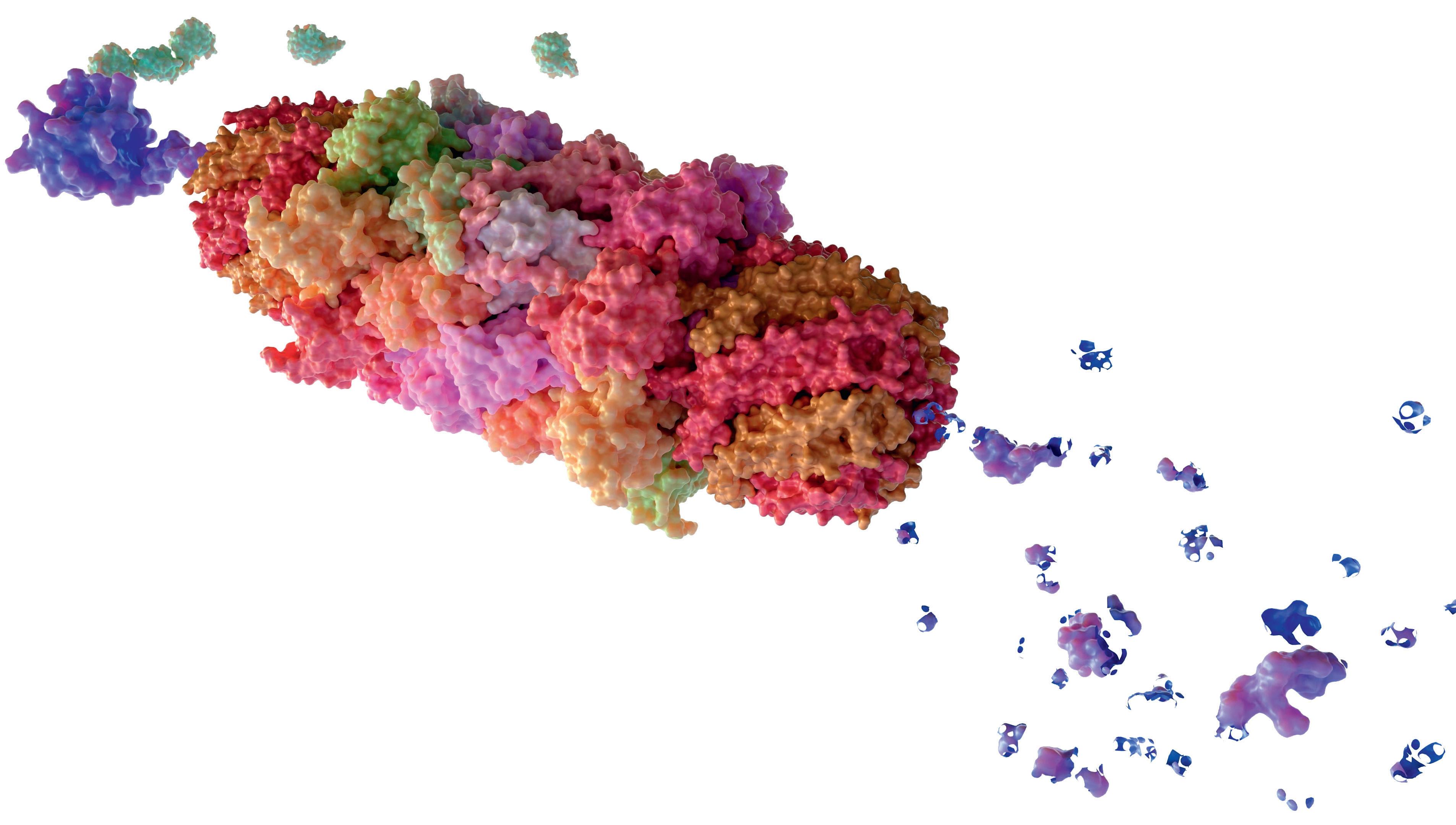
Enzyme stability
This may occur naturally, as a result of higher temperatures or changes in the pH of the solution for example, while other factors may also be involved. The FLUIZYME project team is investigating whether the rate at which this transition takes place is accelerated in industrial environments, using scale down apparatus designed to mimic the conditions in the larger reactors. “For example, we put bubbles containing oxygen in at the bottom of a vertical tube, which then rise to the top. We do this in a very defined way, so we know there’s a bubble coming at a specific point, and then a second will come later on,” outlines Professor Woodley. The bubbles rise to the top and the enzyme inside the tube is then exposed to the bubble, with Professor Woodley and his colleagues investigating changes in enzyme
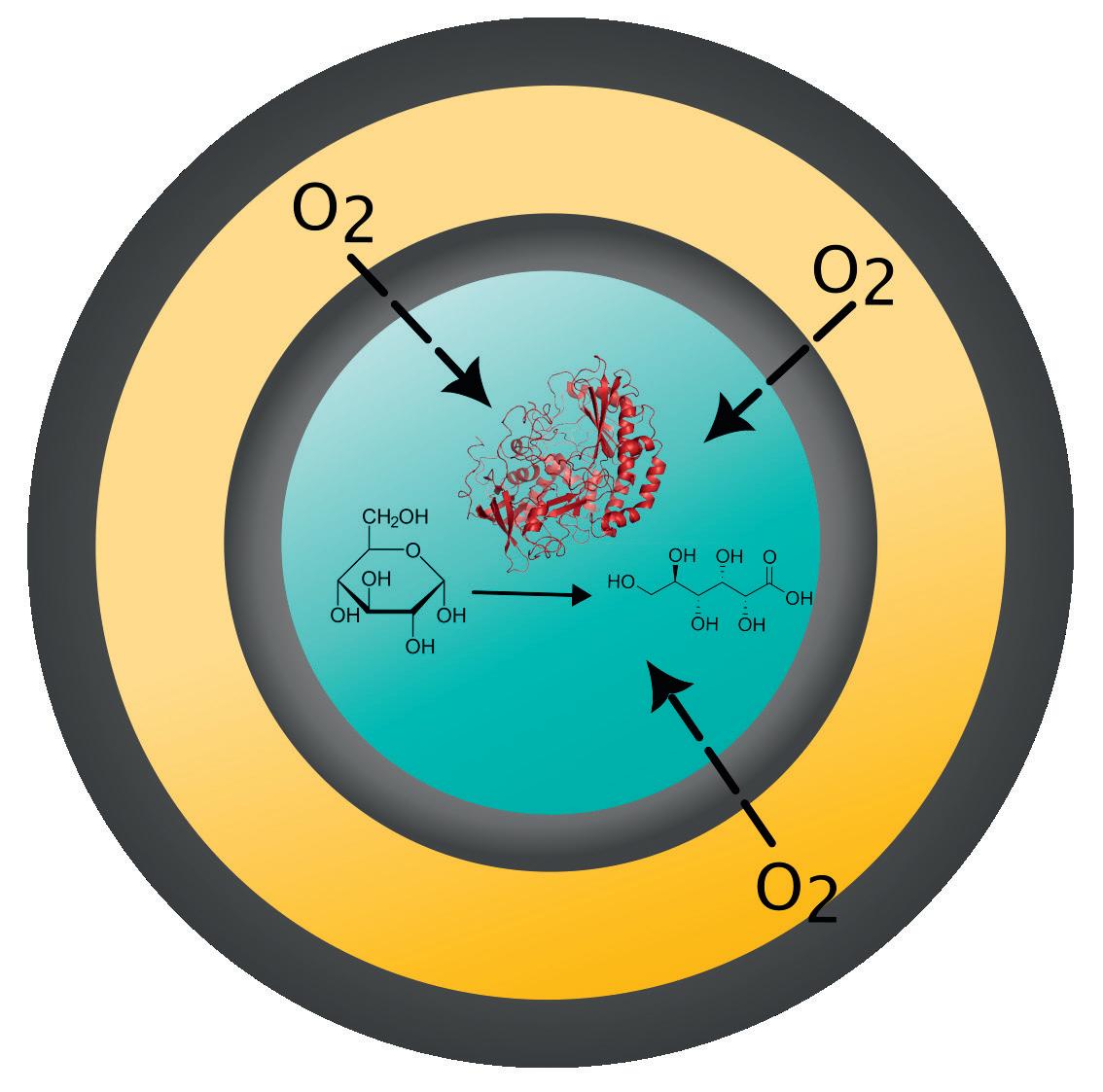
stability in this environment. “We know the size of the bubbles and how much protein is in there as well. We can measure the activity of the enzyme before and afterwards, and we can also look at the structure of the enzyme before and afterwards, and so on,” he says.
“We expose enzymes to these different conditions for periods of around 70 hours, and over that time we see that they lose structure and therefore stability.”
“Enzymes are large
their stability in this bubble apparatus,” explains Professor Woodley. This approach has already yielded some interesting insights. “We’ve seen that an enzyme in solution gets pulled towards the bubble, and likes to stick on the bubble.
This may not seem surprising in itself, but this happens to quite a marked degree,” continues Professor Woodley. “We think that hydrophobic amino acids buried inside the enzyme are dragged towards the gas-liquid interface (bubble
molecules, made up of lots of amino acids, which form into a particular structure or shape. Enzymes need to maintain their shape in order to catalyse reactions.”
The project team works on many enzymes, but mostly oxidases such as NADPH oxidase and also HMFO oxidase, alongside a number of variants.
One of the standard ways of measuring the stability of these enzymes has been to heat them up and observe what happens to the structure until it reaches its melting temperature, at which it essentially falls to pieces. “To date, this socalled melting temperature has been used as an indicator of the stability of an enzyme. Now we are trying with these variants to measure their melting temperatures, and to correlate that with
surface). This then has the effect of pulling the enzyme out of solution, so that it is no longer available for reactions to take place.”
This then raises the question of whether introducing a substance like a surfactant to the solution would have a positive effect, so that an enzyme would no longer stick to the bubble, which could be an interesting avenue of investigation.
In future, Professor Woodley and his colleagues plan to test many more enzymes, and build a mathematical model of their behaviour. “We are in the process of building a model which can be
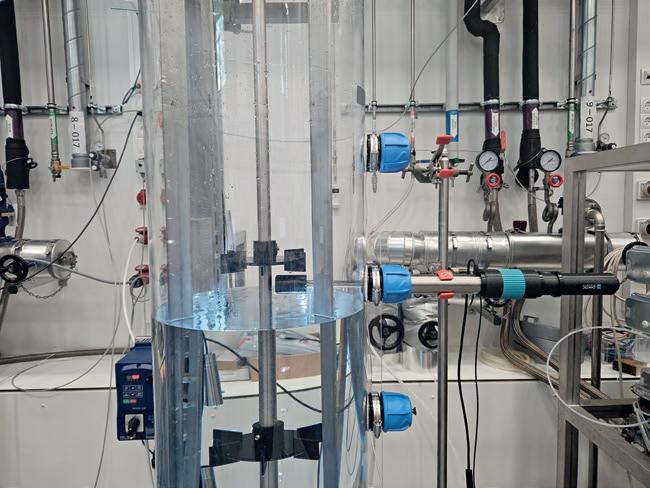
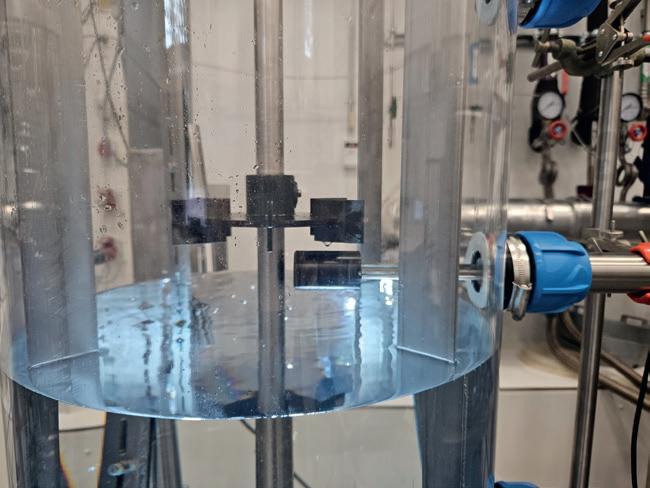
Pilot scale (200 L) apparatus for enzyme testing.
used to make predictions. We hope to be able to predict whether a particular enzyme with certain properties will have problems with the bubbling, or if we need to control the bubbling in some way,” he says. This is with a view to maintaining the stability of an enzyme and enabling more efficient industrial biocatalysis. “A big part of the cost of implementing industrial biocatalysis processes relates to enzyme stability. In many cases, the more stable an enzyme is, the lower the process cost,” continues Professor Woodley. “This research is very relevant to the industrial application of enzymes.”
Environmentally friendly methods
The wider backdrop to this research is the challenge of developing more environmentally friendly and sustainable methods of producing valuable chemicals (including pharmaceuticals). Biocatalysis represents a more sustainable approach than existing methods, yet Professor Woodley says it remains difficult to run a large-scale process. “Biocatalysis takes place in water, and the solubility of oxygen in water is
for enzymes,” he explains. The project’s work holds wider importance in terms of running any process with proteins on larger scales, and Professor Woodley is looking to share their findings more widely. “A lot of the processes use proteins as catalysts (enzymes) or produce proteins as products. Potential exposure to air is always a risk in stirred tanks, so it’s a very big issue,” he says. “We need to understand how to avoid the unnecessary effects of air drawn from the surface and also the supply oxygen to oxidation reactions. This is a very different kind of environment to those typically considered in enzymology.”
This research could ultimately help improve the design of bioprocess plants, reduce the cost of implementing industrial processes, and address concerns around the sustainability of the chemical and biotechnology industries. Alongside these more long-term considerations, Professor Woodley is also keen to utilise the apparatus developed in the project, and contribute to the goal of building a deeper picture of the factors that affect the stability of different
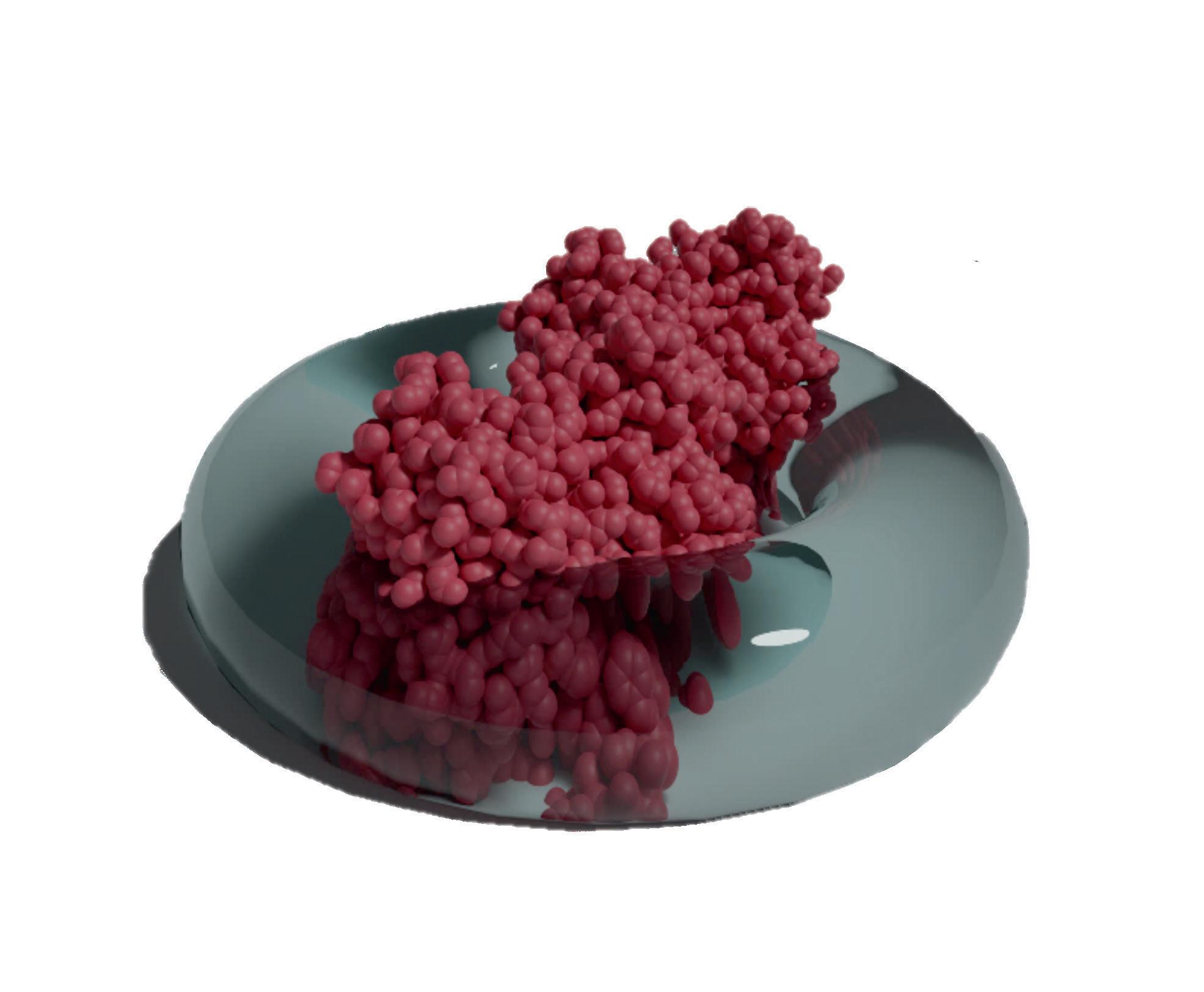
FLUIZYME
Understanding the Effect of Non-natural Fluid Environments on Enzyme Stability
Project Objectives
Fluizyme is an Advanced ERC project with the overall objective of understanding the effect of new-to nature conditions on enzyme stability. Such conditions occur when biocatalytic reactions are scaled-up in industry, in large tanks with fluctuating concentrations and often with interfaces (gas-liquid and liquid-liquid). In particular the focus in this project is on understanding the effect of the gas-liquid interface on the stability of oxygen-dependent enzymes, such as oxidases.
Project Funding
This project has received funding from the European Union’s Horizon 2020 research and innovation programme under Grant agreement No 101021024.
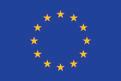
Project Participants
DTU Chemical Engineering
Contact Details
Project Coordinator, Professor John Woodley
DTU Chemical Engineering
Department of Chemical and Biochemical Engineering
Søltofts Plads 228A 2800 Kgs.
Lyngby
Denmark
T: +45 5350 3747
E: jw@kt.dtu.dk
W: https://www.kt.dtu.dk/research/prosys/ projects#fluizyme
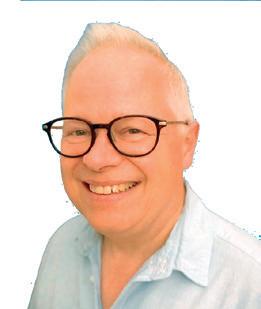
John Woodley is Professor of Chemical Engineering at the Technical University of Denmark. He has a PhD from University College London (UK) and after gaining industrial and academic experience, in 2007 he moved to Denmark. He leads research at the interface of process and protein engineering, and has long standing interest in biocatalysis, where enzymes are used for the synthesis of valuable chemicals. A dedicated teacher, he has educated more than 60 PhD students. He was co-chair of the GRC Biocatalysis conference in 2016 (USA) and will be co-chair of the EIC Enzyme Engineering conference in 2025 (Denmark). John is a Fellow of the Royal Academy of Engineering (UK).
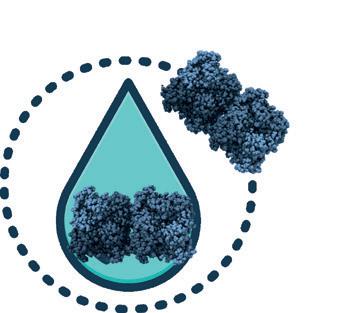