Sensors that rise above the norm
The Internet of Things (IoT) is becoming a reality, and effective, reliable sensors are central to its ongoing development. Researchers in the IQLev project are investigating different levitation platforms, looking to develop a new type of high-performance inertial sensor, as Dr Joanna Zielinska explains.
cryostat, at a temperature of 15 millikelvin above absolute zero,” continues Dr Zielinska.
“The nanoparticle is levitated using a magnetic field generated by coils. The experiment is isolated from mechanical vibrations and shielded from outside magnetic fields.”
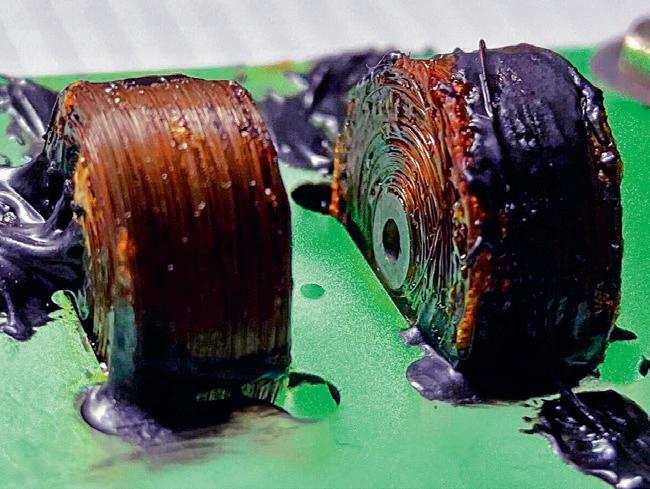
A device called a SQUID (Superconducting QUantum Interference Device) is used to detect the magnetic field of the levitated sphere, thanks to which the researchers can monitor its motion.
The researchers at the ETH Nanophotonics Systems Laboratory (also involved in the project) are investigating optical levitation in combination with electrostatic levitation on a chip. “They have a very compact setup, which is largely contained in a nanofabricated chip. The light for trapping the particle is delivered to the chip with optical fibres, which also collect the scattered light. Additionally the chip provides an electrostatic safety net, which catches the particle if it escapes from the optical trap,” outlines Dr Zielinska.
IQLev
Ground-breaking inertial sensing navigation for IoT devices
Project Objectives
The goal of the IQLev is to study different levitation platforms and explore their application as inertial sensors. Within the scope of this project, IQLev researchers are experimentally and theoretically advancing magnetic, optical and electrostatic levitation techniques and testing their performance as accelerometers and gyroscopes..
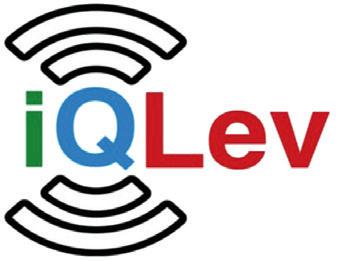
Project Funding
Potential applications
This project has received funding from the European Union’s Horizon 2020 research and innovation programme under grant agreement No 863132.
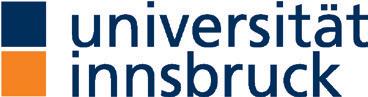
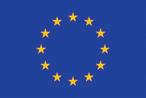
Project Partners
IQLev is represented by a consortium of world expert groups from academia and industry with different key competences. Each consortium member contributes significantly to the joint IQLev aim of establishing high-performance levitation-based inertial sensors. https://iqlev.ethz.ch/consortium.html
Contact Details
The
Internet
of Things (IoT)
is becoming a reality, with more and more everyday devices and systems now capable of sending and receiving information via the internet. High-quality, precision sensors are central to many IoT applications, while they also play an important role in certain highend applications such as autonomous vehicles moving in tunnels or under water, which rely on sensors to determine their position. “If you don’t have access to external signals to confirm your position then you need very good inertial sensors, or else any errors will accumulate and send the vehicle off course,” outlines Dr Joanna Zielinska, a researcher in the Photonics Laboratory at ETH Zurich.
This topic is at the core of Dr Zielinska’s work in the EU-funded IQLev project, in which researchers are developing inertial sensing systems, specifically accelerometers to sense acceleration and gyroscopes to sense external rotation. “The project consortium includes four research teams, exploring different platforms for levitation and looking at how they can be used for inertial sensing,” she says.
Levitating sensors
High-performance inertial sensing requires excellent isolation from the environment, as well as high mass and precise readout. By levitating the sensor in vacuum, the researchers are able to effectively isolate it from the environment, reducing noise exposure. Normally noise limits sensor performance; levitating a sensor represents a highly effective, clean way of isolating it from the environment and avoiding this issue. “The sensor is levitated in vacuum, so it does not actually interact with anything,” explains Dr Zielinska. However,
the main challenge is increasing the mass of the levitated object while retaining excellent detection and precise control of its motion.
The project partners are investigating different approaches to doing this, with Dr Zielinska and her colleagues at ETH focused primarily on optical levitation for gyroscope applications.
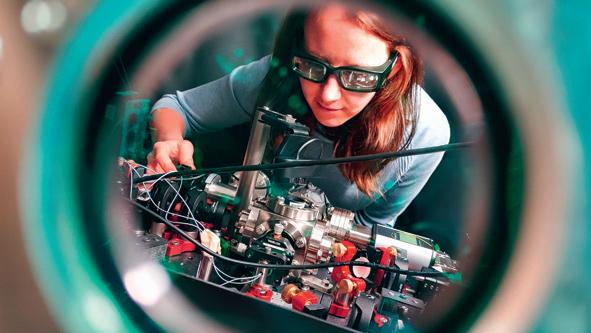
“We use a focused laser beam to levitate and control a silica nanoparticle, which is about a micrometer in size and rotates a billion times per second,” she says. “This silica nanoparticle is attracted to the maximum optical field intensity, so it oscillates around the focus of this laser beam. We study the motion of these nanoparticles which we read out by analysing the properties of the light they scatter.”
The project’s research involves performing optical levitation in vacuum. Inside the experimental chamber, a high numerical aperture lens focuses the infrared laser beam used for levitation. The experimental apparatus also includes other optical elements, which collect the light scattered by the levitated particle and
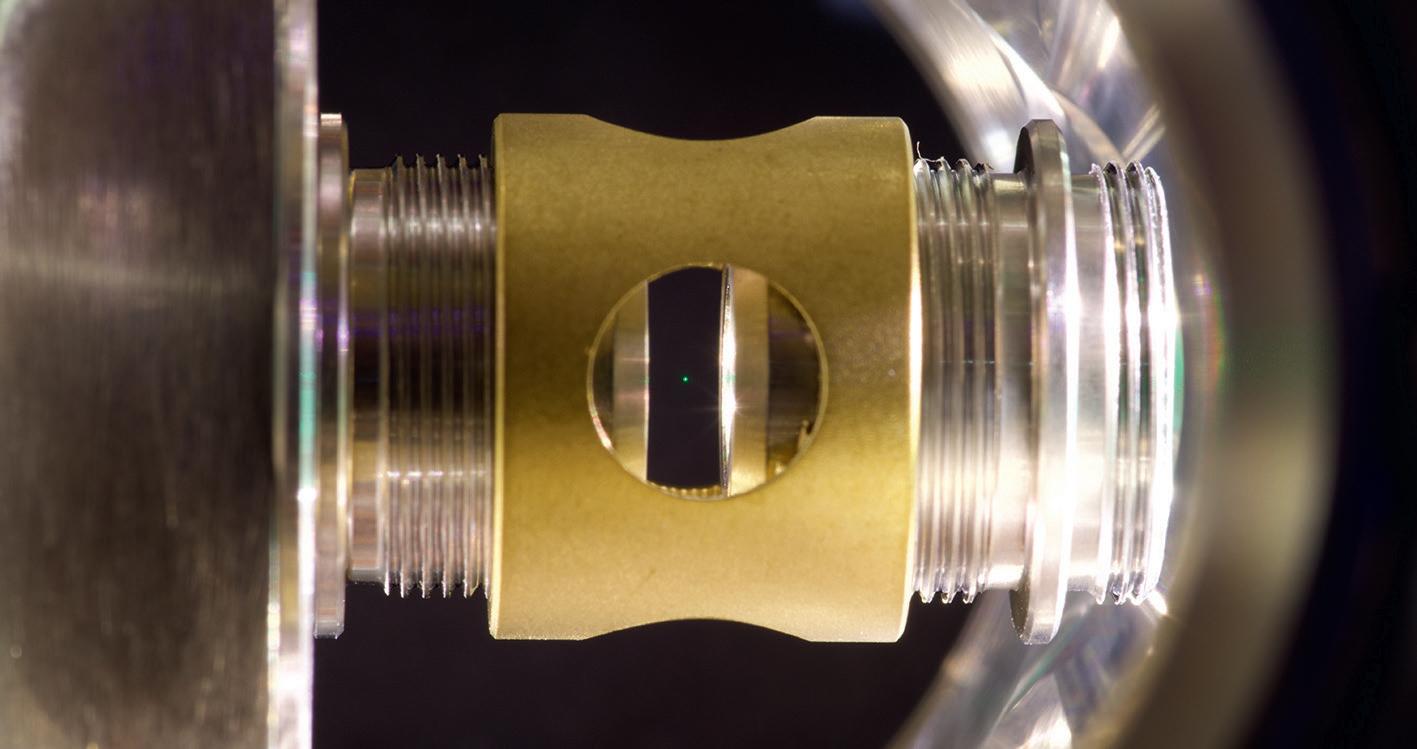
measure it in an optimum way. “The collected information about the particle is electronically processed and fed back to the system, in order to control the nanoparticle’s motion,” says Dr Zielinska. This method has proved extremely effective, and has allowed the IQLev researchers to cool an optically levitated particle to its motional ground state (Ref 1), where its behaviour is governed by quantum mechanics.
In the future this will lead to high-performance quantum-enhanced inertial sensors. In order to guide these future experimental efforts, researchers from University of Innsbruck have developed a theoretical formalism to understand how the electromagnetic field interacts with levitated particles of arbitrary size in the quantum regime (Ref 2).
The project’s agenda also includes research into magnetic levitation; the setup here is highly complex, as very low temperatures are required.
“Our partners at the University of Vienna are using magnetic fields to levitate a nanoparticle (a superconducting lead-tin sphere) in a

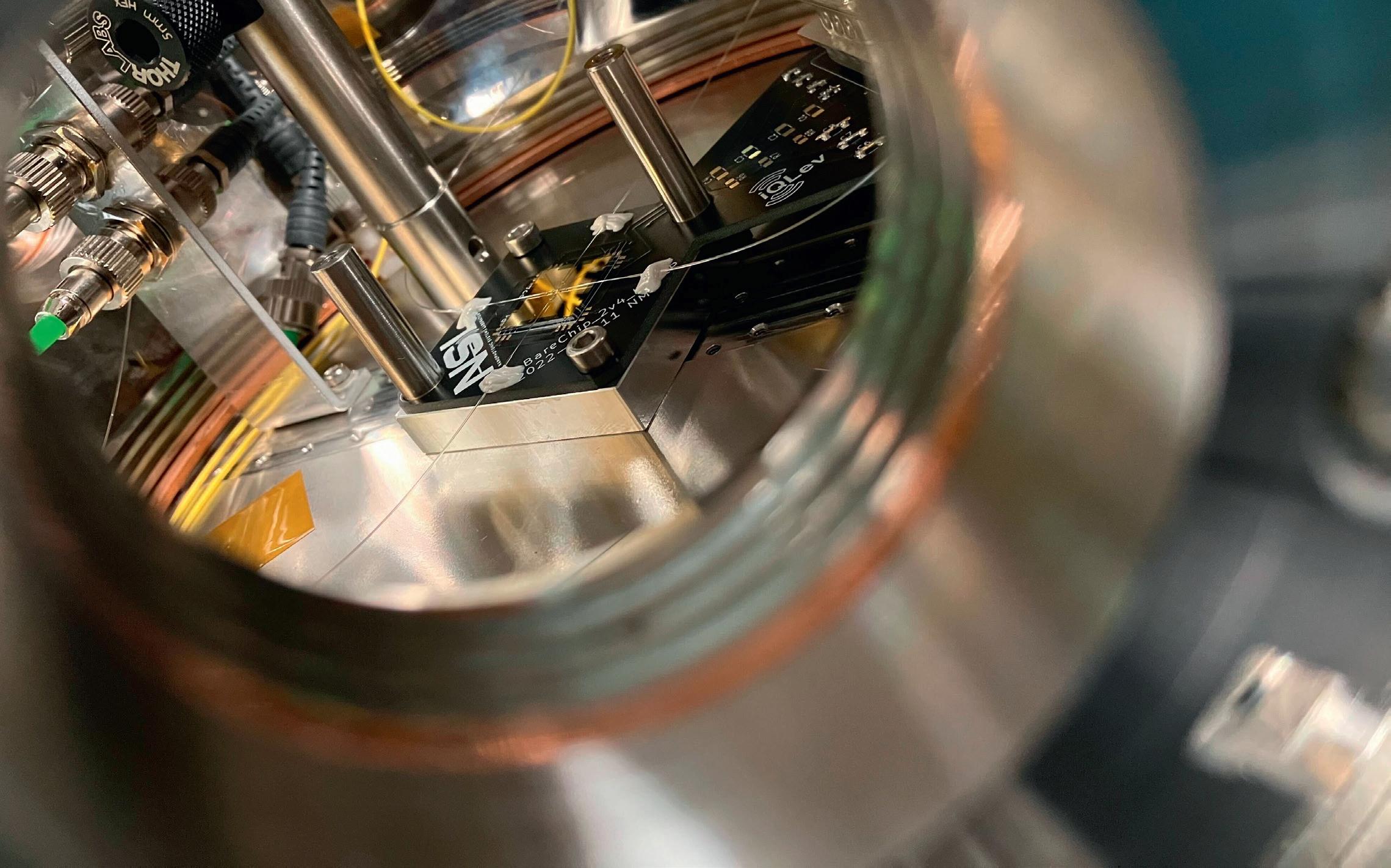
Researchers in the project have been working to improve each of these levitation methods, although Dr Zielinska says each has their own drawbacks and advantages. “Optically levitation has a high detection efficiency, but only works for low masses. We cannot optically levitate particles which are larger than 20 micrometers, which limits the performance of an accelerometer,” she explains.
The aim of the project is to establish a proofof-principle rather than develop a specific product, although this work could be relevant to certain areas of industry in the future. While Dr Zielinska and her colleagues are working on fundamental science, they are also very much aware of wider possibilities. “This area is still highly attractive for us as researchers, because we are exploring new physics and new working parameters. It’s also attractive for our industrial partners, as they will learn more about the capabilities of levitated sensors,” she outlines. “These types of systems could potentially be applied in seismology as well as in satellite missions. We are also interested in the possibility of applying this technology to tackle future challenges, such as highend navigation and positioning, as well as gravimetry and seismometry.”
With optical levitation we use a focused laser beam to move and control a silica nanoparticle, which is about a micrometer in size and can rotate a billion times per second.
The particle is attracted to the maximum optical field intensity, so it oscillates around the focus of this laser beam.
This is not the case with magnetic levitation, where it’s possible to work with particles that are orders of magnitude heavier, which means that they are better inertial sensors. The drawback here is that the technology is more complex, including the detection method. “Detecting particle motion using magnetic fields is much harder than using optical signals,” says Dr Zielinska. Over the course of the project researchers have been able to improve this detection capability, and Dr Zielinska says the performance of the magnetic levitation-based accelerometers is highly promising (Ref 3). “We’ve shown excellent performance of accelerometers, based on magnetic levitation,” she continues. “At ETH we are working on improving the performance of optically levitated gyroscopes by using extremely high rotational speeds to mitigate the sensitivity limitation due to low mass. However, we need to develop these systems further before we can look towards practical applications.”
This research is set to continue beyond the conclusion of the IQLev project, with Dr Zielinska planning to continue her work on optical levitation. “These levitated nanoparticles are among the fastest rotating objects on Earth, which translates into excellent rotation-sensing performance. In the future I hope to explore different applications of the gyroscopes based on levitated rotating particles, for example seismology” she says. This could be as part of a successor project to IQLev, although a future project wouldn’t have such a broad scope. “We have now learned what’s good for acceleration and what’s good for rotation sensing, so any subsequent project could be more narrowly focused,” continues Dr Zielinska. “More attention will be focused on developing products on the basis of this research, and our industrial partners will play a major part in that.”
Dr. Joanna A. Zielińska, Postdoctoral Researcher Photonics Laboratory, ETH Zürich HPP M 24, Hönggerbergring 64 CH-8093 Zürich, Switzerland T: +41 44 633 06 12 e: jzielinska@ethz.ch W: www.photonics.ethz.ch

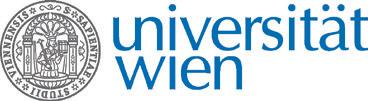
Ref 1: Magrini et al, Nature 595, 373–377 (2021); Tebbenjohanns et al Nature 595, 378–382 (2021)
Ref 2: Maurer et al, arXiv:2106.07975 [quant-ph] (2021) Ref 3: Hofer et al, Phys. Rev. Lett. 131, 043603 (2023) Joanna Zielinska
Joanna Zielinska received her PhD in 2018 at the Institute of Photonics Sciences (ICFO) in Barcelona for her research into quantum light generation. She subsequently undertook postdoctoral research focused on molecular physics at Imperial College London. She joined ETH Zurich in 2020 to study rotational degrees of freedom of optically levitated nanoparticles.
Magnetic levitation experiment in the cryogenic environment. The coils used to generate magnetic field are glued into a sapphire holder (green). Between the levitation experiments, the particle rests in the bowl visible between the coils.