Mineral transformation in action
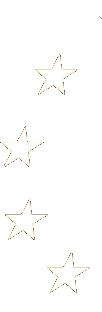
Oxygen is used up very quickly in wet or flooded soils, after which other types of respiration set in. Iron is a very important electron acceptor in soil environments for organisms that respire organic carbon to gain energy, processes which have an important influence on the behaviour of many nutrients and contaminants, as Professor Ruben Kretzschmar explains.
Many soils are periodically wet or even flooded, leading to poor aeration. When this happens, soil organisms use up the remaining oxygen in the soil very quickly, leading to a lack of oxygen for respiration.
“Under these conditions, other elements can be used by certain microorganisms for respiration, such as manganese, iron and sulphur. Iron is a very important electron acceptor in soils for organisms that respire organic carbon to gain energy, in the absence of oxygen,” explains Ruben Kretzschmar, Professor of Soil Chemistry at ETH Zurich. These processes hold wider importance, as they can trigger recrystallization and transformation processes of iron minerals, which in turn control the behaviour of many nutrients and contaminants in soils. “Elements like phosphorus, arsenic, chromium, nickel, cadmium and zinc strongly bind to certain iron minerals, so mineral dissolution or transformation can drastically change the mobility and bioavailability of these elements,” continues Professor Kretzschmar. “A lot of toxic contaminant elements can undergo oxidation and reduction (redox reactions).”
IRMIDYN project
As Principal Investigator of the ERC-funded IRMIDYN project, Professor Kretzschmar and his team are now looking at these iron mineral transformation processes at several different sites in Europe and Asia, work which holds relevance to understanding how nutrients and contaminants may behave in soils.
“We
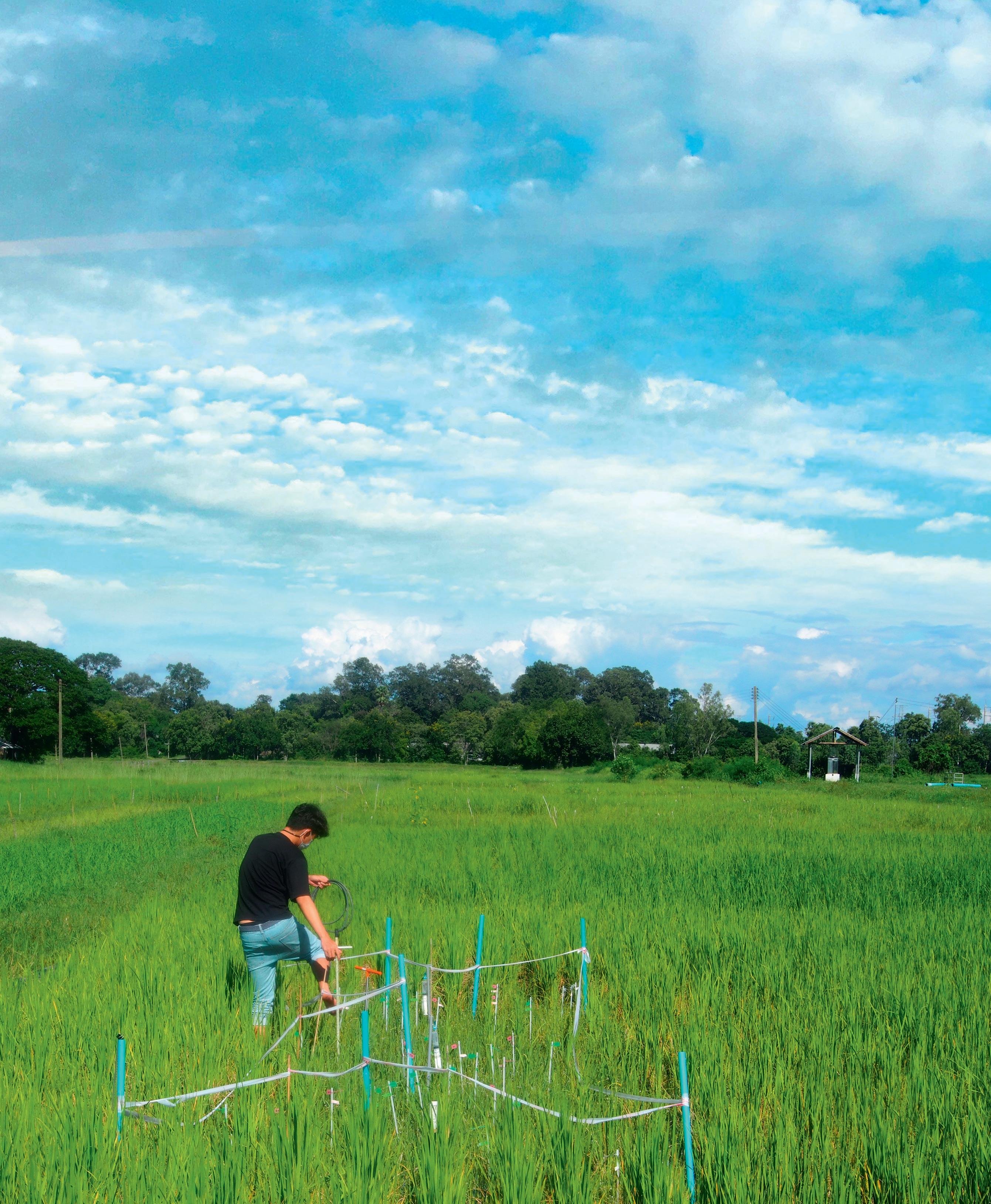
Contaminants may also get into rice paddy fields when they are irrigated using contaminated surface water or groundwater, another topic of interest in the project. “Our group has in the past worked on rice paddies in East and South-East Asia, where arsenic and cadmium pollution is a widespread problem in rice production” says Professor Kretzschmar.
want to look at iron mineral transformations in actual soils and understand if
they happen in the same way as we observe in the laboratory.”
Environments in which redox-induced iron mineral transformation processes play an important role include, for example, river floodplains, rice paddies, minerotrophic peatlands and inter-tidal flats, such as the Wadden Sea or tropical mangrove ecosystems. “Soils close to aquatic systems effectively act as sinks for contaminants,” outlines Professor Kretzschmar. “For example, there are many rivers in Europe and other countries where floodplains are contaminated, from mining and other industries located along a river.”
A paddy field used for crop cultivation is typically drained and then flooded for an extended period, and it can remain flooded for several months. By contrast soils in the Wadden Sea, which is affected by the tides of the North Sea, are flooded and drained on a more regular basis, says Professor Kretzschmar. “The soils of the inter-tidal flats in the Wadden sea are exposed to oxygen and then flooded again at a much higher frequency than soils in rice paddies,” he explains. The team of researchers in the IRMIDYN project are looking at the different iron
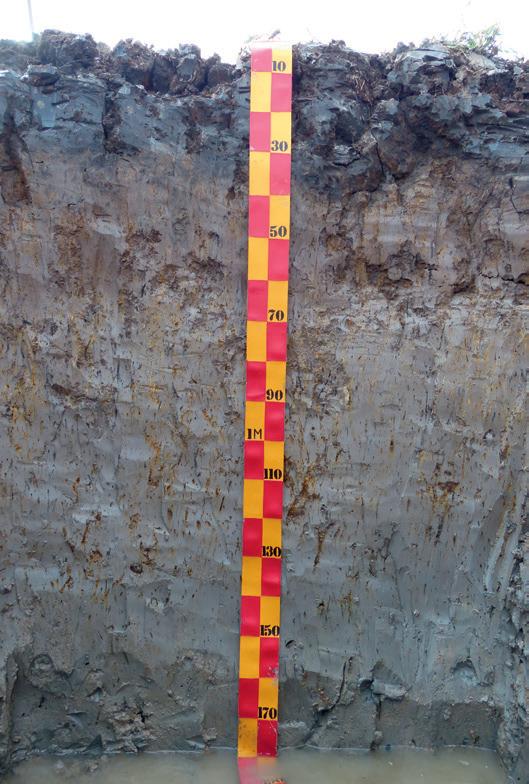
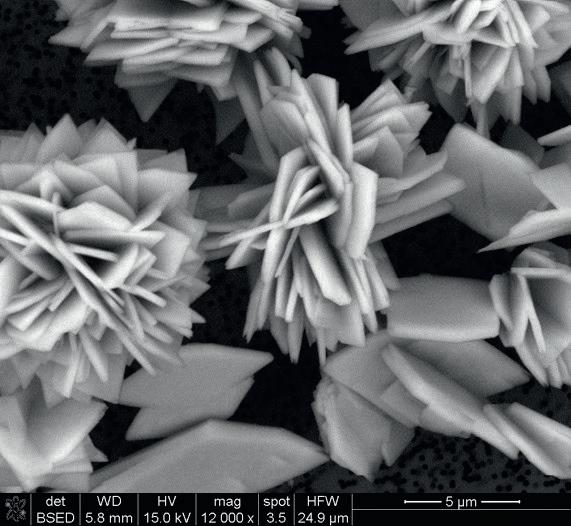
mineral transformation processes which occur in these contrasting environments. One important process is the transformation of ferrihydrite, a weakly crystalline iron oxyhydroxide, to more crystalline iron oxyhydroxide minerals. “It’s essentially the first product that forms when iron oxidises and precipitates into a weakly-crystalline iron oxyhydroxide mineral,” outlines Professor Kretzschmar. “There are also some other more crystalline minerals in soils, like goethite or lepidocrocite, a bright orange mineral often found in soils with variable redox conditions.”
The transformation of ferrihydrite into lepidocrocite and goethite has been intensively studied in the laboratory, yet researchers haven’t really been able to study how it happens in natural soil settings, a topic that Professor Kretzschmar and his team are addressing in the project. “We want to look at these transformations in actual soils to understand whether they occur in the same way as we observe in the laboratory,” he says.
A variety of other minerals can also be formed when iron is exposed to different environments. For example in sulphurrich environments it forms iron sulphide minerals like mackinawite, which can then be transformed further. “Mackinawite can be transformed to greigite, which may be the first step towards the formation of pyrite. This happens in certain types of sediments and other sulphur-rich reducing environments,” outlines Professor Kretzschmar. In-situ pyrite formation in inter-tidal sediments is another topic of investigation in the project, but preliminary results show that it will require longer field experiments over months to years, rather than weeks. “When pyrite oxidises, iron sulphate minerals like jarosite may form under acidic conditions. Jarosite can also host certain contaminant elements. We are therefore studying the structure and dynamics of jarosites in acid sulphate soils in Thailand,” says Professor Kretzschmar. Studying iron mineral transformations in-situ in the field is not an easy task, however, as iron minerals form only a relatively small part of
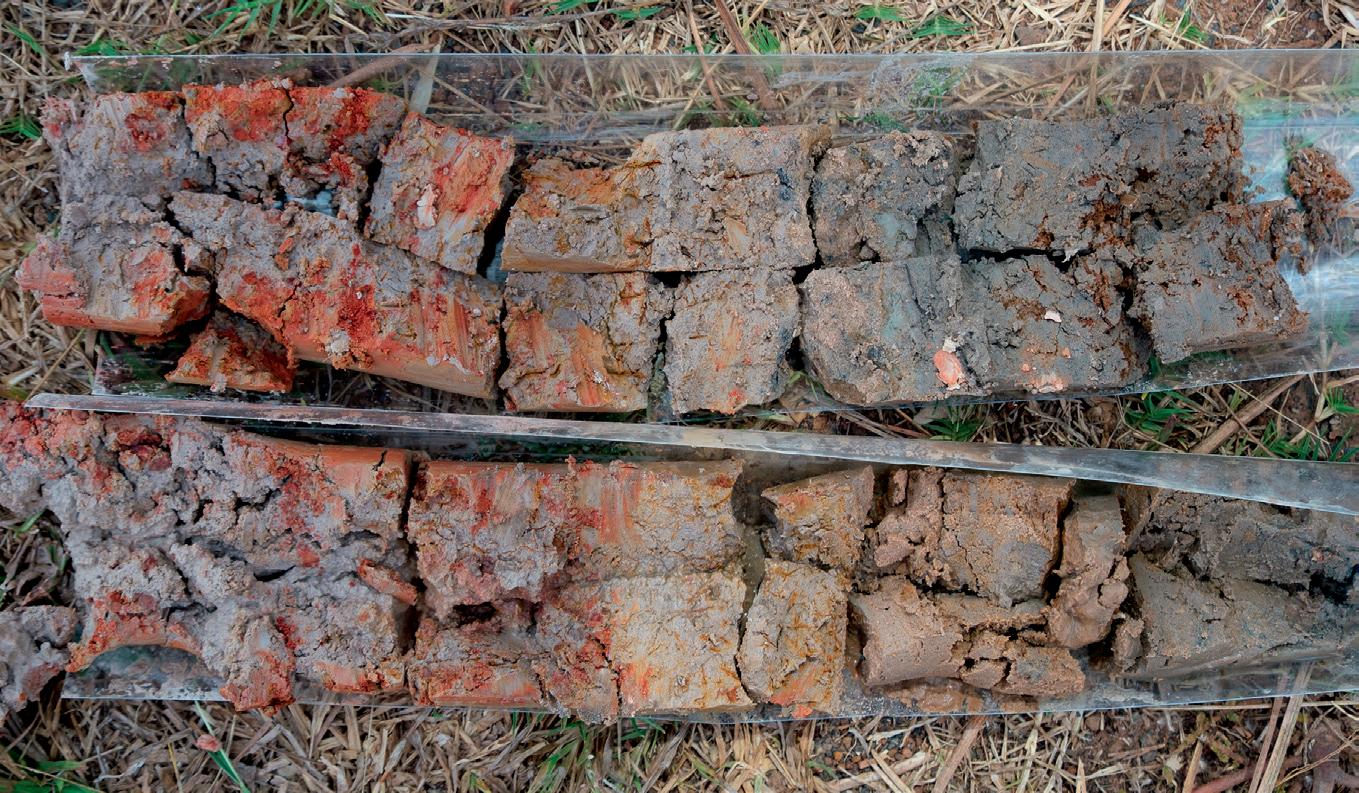
soil and they are not easy to detect with X-ray diffraction or other conventional mineralogical analysis techniques. Researchers in the project have developed a method where an isotope of iron – 57Fe – is used as a tracer, allowing Professor Kretzschmar and his team members to monitor any transformations. “57Fe makes up approximately 2 percent of naturally occurring iron. If we produce a mineral that consists of 96 percent 57Fe, and mix it into some soil, then most of the 57Fe will come from this mineral. This allows us to follow its transformation with Mössbauer spectroscopy, as we can selectively detect just 57Fe with this method,” he explains. The project team is also exploring new ways to apply confocal Raman microscopy to study
What happens when microorganisms breathe iron?
You can learn about the work of the IRMIDYN project in this video: https://www.youtube.com/ watch?v=XFWnumTda5s
iron minerals and gain spatial information on iron mineral distribution. “We have used Raman spectroscopy to generate high-resolution maps of iron mineral transformation products in soil microcosms,” continues Professor Kretzschmar.
Mineral transformation processes
The IRMIDYN researchers are using these approaches to gain deeper insights into mineral transformation processes at different sites, mainly in Thailand, Germany and Iceland. It is known that some of these processes can occur relatively quickly in a lab setting – for example, ferrihydrite can completely recrystallise to goethite within days, or even hours – yet the expectation is that they would take longer in natural settings. “We have been monitoring the samples over weeks rather than days,” says Professor Kretzschmar. For example, there are diffusion limitations in the field, as dissolved ferrous iron has to diffuse to the places where it reacts with iron mineral surfaces, so Professor Kretzschmar says transformation processes are generally slower than those seen in the lab.
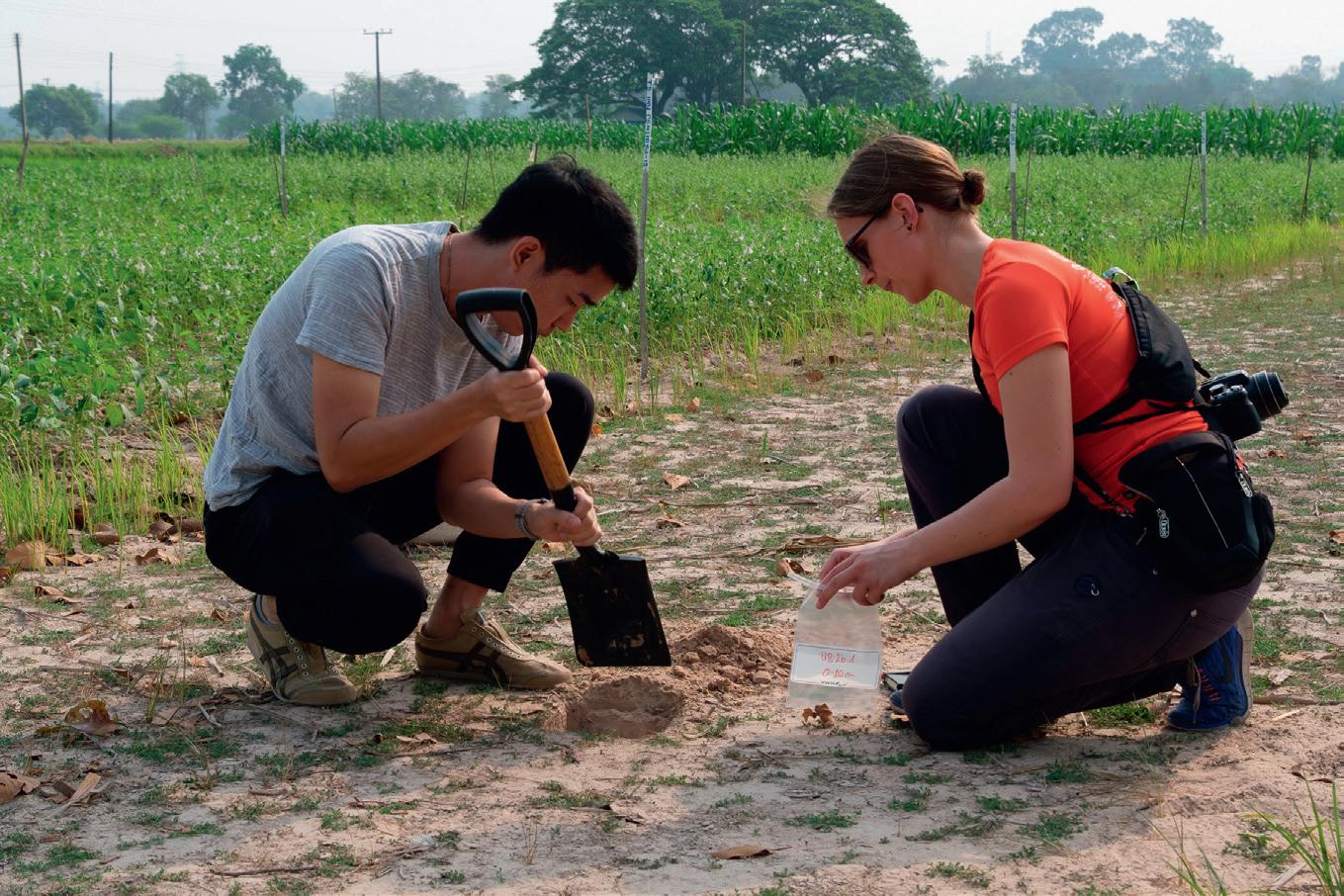
IRMIDYN
Iron mineral dynamics in soils and sediments: Moving the frontier toward in‐situ studies
Project Objectives
The thermodynamic stability and occurrence of iron minerals in sufficiently stable Earth surface environments is fairly well understood and supported by field observations. However, the kinetics of iron mineral recrystallisation and transformation processes under rapidly changing redox conditions is far less understood, and has to date mostly been studied in mixed reactors with pure minerals or sediment slurries, but rarely in-situ in complex soils and sediments. This project will take a large step toward a better understanding of iron mineral dynamics in redox-affected Earth surface environments, with wide implications in biogeochemistry and other fields including environmental engineering, corrosion sciences, archaeology and cultural heritage sciences, and planetary sciences.
Project Funding
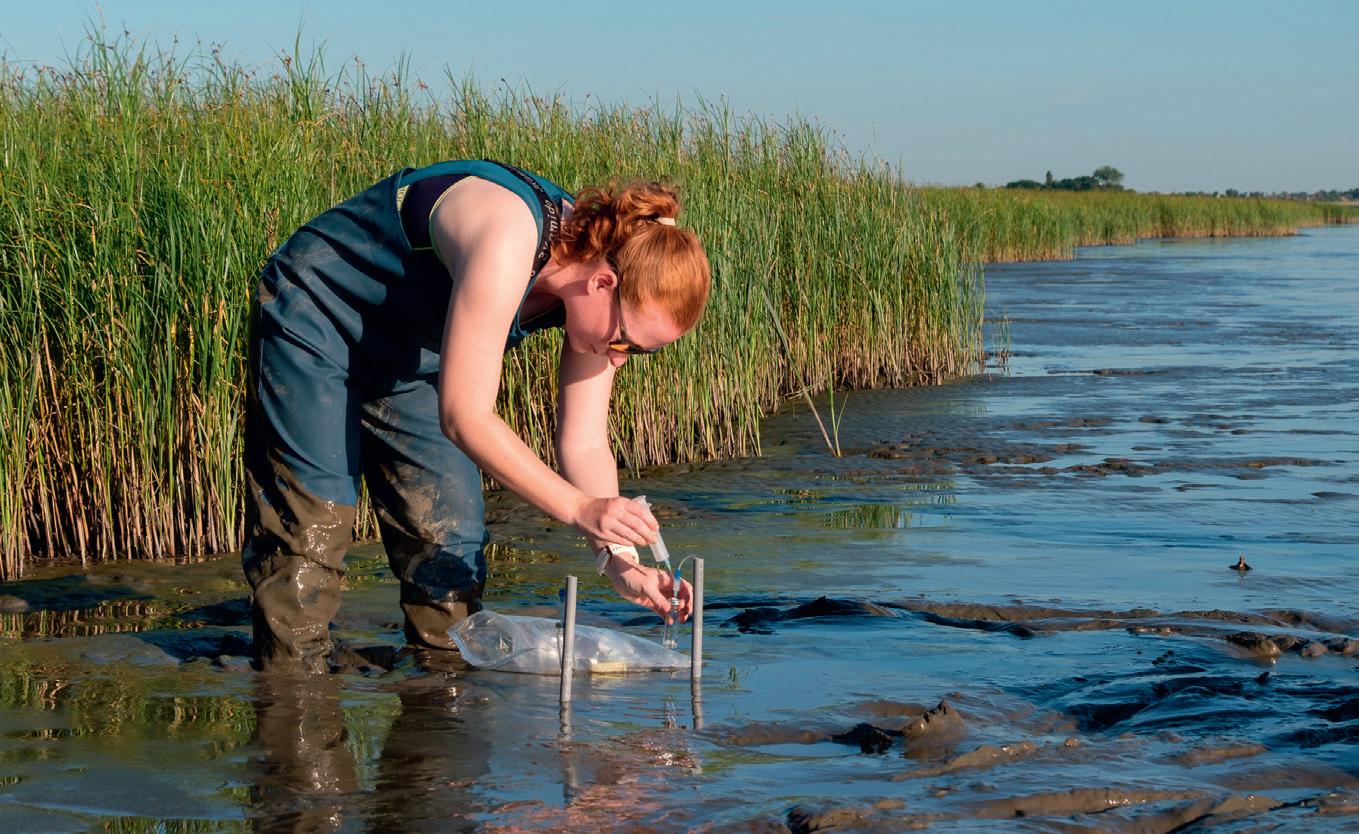
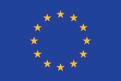
This research is part of a project that has received funding from the European Research Council (ERC) under the European Union’s Horizon 2020 research and innovation programme (788009IRMIDYN-ERC-2017-ADG).
Project Team
Current members: Dr. Andrew Grigg - Dr. Joëlle Kubeneck - Dr. Pierre Lefebvre - Dr. Sara Martinengo
Former members: Dr. Laurel ThomasArrigo - Dr. Luiza Notini - Dr. Katherine Rothwell - Dr. Katrin Schulz - Giulia Fantappiè
https://soilchem.ethz.ch/IRMIDYN/meet-the-team.html
Contact Details
Project Coordinator,
Prof. Dr. Ruben Kretzschmar
Soil Chemistry Group
Institute of Biogeochemistry and Pollutant Dynamics
Department of Environmental Systems Science
ETH Zurich
CHN F23.1, 8092 Zurich
Switzerland
T: +41 44 6336003
E: kretzschmar@env.ethz.ch
W: https://soilchem.ethz.ch/IRMIDYN.html
W: http://soilchem.ethz.ch
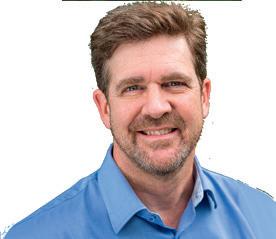
Ruben Kretzschmar earned his PhD in Soil Science in 1994 at North Carolina State University, and has been Professor of Soil Chemistry at ETH Zurich since 1999. His recent research focuses on the speciation and bioavailability of trace elements in redox-affected soils and their coupling to major elements such as iron, manganese, sulfur, and carbon.
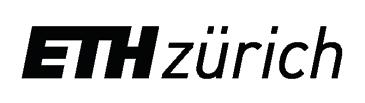
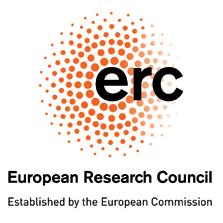
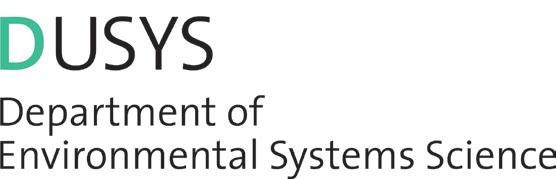
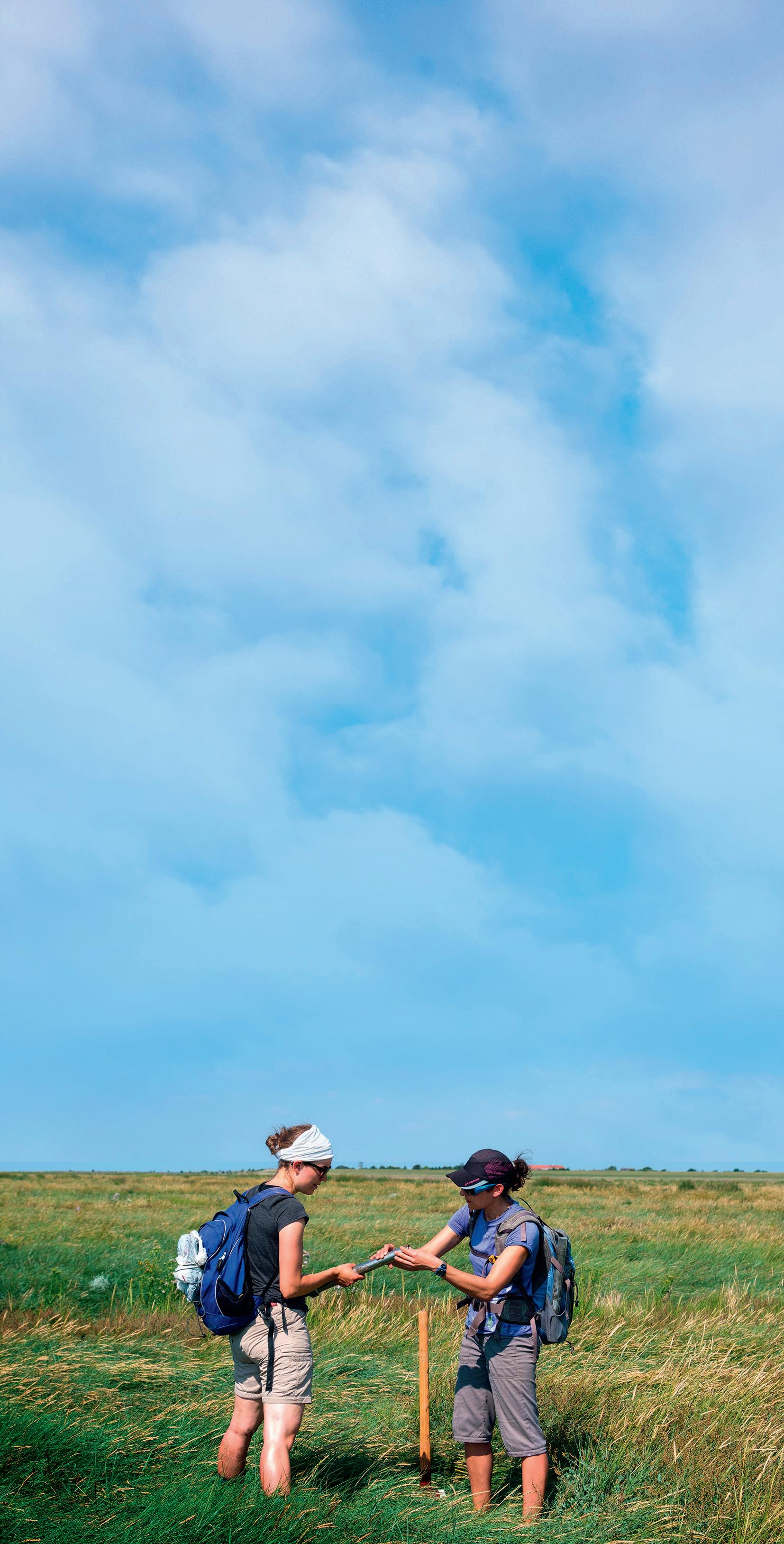
This research contributes to a deeper understanding of nutrient dynamics and the behaviour of contaminants like cadmium, arsenic and zinc in soils, which is a major issue in rice production. Arsenic becomes mobilised under iron-reducing conditions in soil, and it can then be taken up fairly easily by rice plants, to a point where it may be harmful to human health. “The amount of arsenic in rice grains must not exceed certain limits,” stresses Professor Kretzschmar. A lot of attention is currently focused on controlling arsenic levels in rice grains, for example through controlled irrigation and by making certain changes to soil chemistry. “Our work is going to be interesting to researchers seeking to understand the speciation and uptake of arsenic in these rice paddy soils,” continues Professor Kretzschmar. “The project’s research will also contribute to a fuller understanding of the impact of a flood on water quality in the surrounding area.”
The new approach developed in the project could be applied by other researchers studying iron mineral dynamics, while it also holds wider relevance beyond the soil chemistry field. Indeed, Professor Kretzschmar says there is interest in the project’s research from several
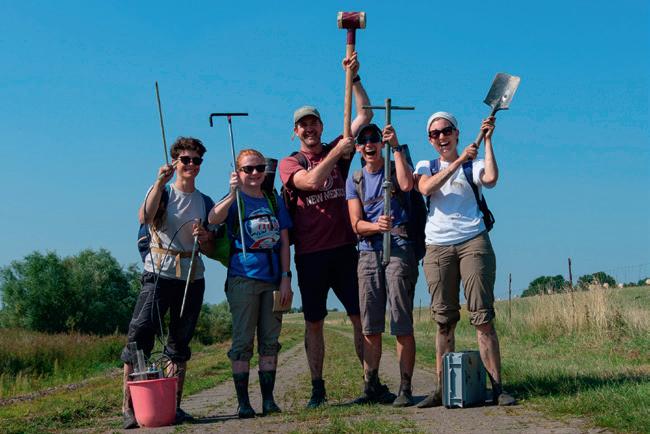
other scientific disciplines. “For example, geologists or geochemists studying how iron minerals formed under certain conditions in the early ocean might want to use our approach. It could also be applied to study iron mineral transformations in completely different settings, like corrosion science, or other fields,” he outlines. Beyond the scope of the IRMIDYN project, Professor Kretzschmar plans to conduct field experiments over longer timescales, as well as looking at the effects on nutrient and contaminant behaviour. “We’re also starting some experiments on soils that are partially wet rather than fully saturated or flooded. Most soils in Switzerland are periodically wet during certain parts of the year, and there are also anoxic zones in these soils,” he continues.