Shape shifting in the battery market
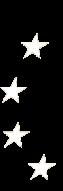
Silicon expands dramatically when charged with lithium, which limits its potential use in lithium-ion batteries. The METABATT project team are building up a new class of metamaterials which change their shape but not their volume when a stimulus is applied, work which holds relevance for a variety of applications, as Professor Ivano E. Castelli and Joonyeob Jeon explain.
The majority of lithium-ion batteries use a graphite anode, while the cathode is typically a metal oxide like NMC (LiNiMnCoO2) , or a phosphate such as LiFePO4 (LFP). Many scientists are looking to develop new materials and improve the performance of these batteries, a topic that Professor Ivano Castelli and his colleagues in the MetaBatt project are addressing. “We want to develop materials that increase the storage capacity of batteries, while at the same time, we also want to improve interfaces, influencing stable battery operation on account of various chemical reactions and ion transportation,” he outlines. This research centres around developing metamaterials using a negative Poisson ratio to control the volume expansion of Si anode during the charging process, the properties of which are derived from their shape rather than their chemistry. “We want to achieve interesting properties not only through the atomic level, so the chemistry and the structure, but also from the way the material is shaped,” explains Professor Castelli. “In our case, it can be rods, materials that change their shape, depending on their response to the environment.”


Nanostructured materials
A major motivating factor behind this work is a recognition of the limitations of silicon as an anode material in lithium-ion batteries. While silicon has a 10 times higher storage capacity than graphite, it expands by a factor of four during charging, which can cause it to crack. “This, in the long run, breaks contacts in the battery and makes it unusable,” says Professor Castelli. The project team is working to address this issue by developing nanostructured metamaterials that change their shape on the application of a stimulus, instead of changing volume. “Imagine that you will still have the same overall volume, but the shape inside will be different. We are developing a methodology to computationally design and experimentally fabricate materials with a pre-programmed response,” continues Professor Castelli. “We want to add a new degree of freedom in rational material design, where we not only change the chemistry when a stimulus is applied, but we also change the shape.”
The challenge is to maximise the storage capacity of the anode while keeping the interface stable, which will help maximise efficiency. Artificial Intelligence, in the framework of Reinforcement Learning (RL) models, is
being used in the project to design silicon nanostructures. Moreover, Professor Castelli and his colleagues are also using physics modelling to assess how these new materials will respond to lithiation. “We are building a combined data and physics modelling framework where we combine RL - to design the shape - with a finite element method that predicts how the material will respond to a stimulus. We want to see the point at which the material breaks for example, or whether some parts of the electrode are at risk of mechanical failure in certain circumstances,” he explains. “My work is about using the finite element method to analyse the structure of metamaterials,” outlines Joonyeob Jeon, a PhD student working on the project.
This can then inform the design of metamaterials and help ensure that the shapes developed using RL techniques are practical and feasible. It is currently very expensive and technologically challenging to make these materials chemically with silicon, so researchers are instead using a 3D-printed polymer that swells in contact with water. “Dr. Xiaoxing Xia from Lawrence Livermore National Laboratory is our collaborator on this, and he fabricates the structures that we predict. When we dip the
polymeric metamaterial in water, we see the shape change; when it is taken out of the water and dried, you essentially see it returning to its earlier shape. So, it’s fully cyclable and mimics what happens at the anode during the charge/ discharge process,” says Professor Castelli. The next step will be to embed the data and physics model within the overall fabrication, which Professor Castelli believes could automate the discovery process and open up a new dimension in materials design. While most materials discovery is currently done at the atomistic level, through changes to the chemistry or the crystal structure, Professor Castelli and his colleagues are following a different approach. “What we want to do here is
The aim is to make silicon with very specific shapes, which may involve the use of different techniques. “It could be 3D printing or lithography for example, and we may need to collaborate with researchers in other disciplines,” says Professor Castelli. Looking ahead, Professor Castelli hopes to collaborate with industry, with a view to translating this research into practical applications; cost is an important consideration in this respect.
“We want to produce something affordable, but currently these kinds of nanostructures are not cheap,” he acknowledges. “This is still a bit of a niche area, it’s not currently something that we can mass produce. We are taking the first steps towards using nanostructured silicon in lithiumion batteries.”
“We want to achieve interesting properties not only through the atomic level, so the chemistry and the structure, but also from the way the material is shaped”
to change the shape, “he explains. The project’s research is primarily focused on lithium-ion batteries, yet Professor Castelli believes the methodology could also be relevant to other applications that require a pre-programmed response. “We have developed a method in the project, and we can use it for many different things, from membranes to electrochemically activated mechanical metamaterials. We are essentially looking at building up a new way to design the materials we need rather than use the materials we have,” he says.
Silicon shapes
The methodology itself is still under development, with Professor Castelli and his colleagues working to add further elements, which it is hoped will enable the design of more complex materials.
There are already companies commercializing Li-ion batteries based on silicon nanowires, but their capacity and safety could be drastically improved, while keeping the cost down. For his part, Professor Castelli is keen to build on the progress that has been made in the project. “We are interested in widening our collaborative networks and working with more experimental and industrial partners,” he says. This is not limited purely to research into lithium-ion batteries, as the project’s work holds relevance to a wide range of potential applications.
“Nanostructures represent a new dimension for materials discovery, and we achieve this using reinforcement learning, data, and a physicsdriven model,” outlines Professor Castelli. “Lithium-ion batteries are one example, but we have also been thinking about others.”




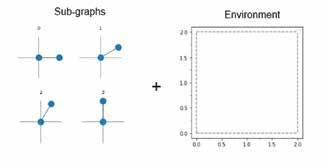
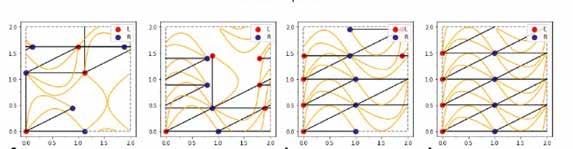
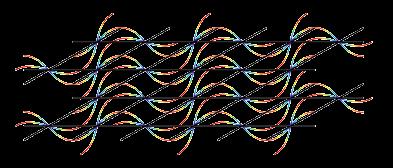
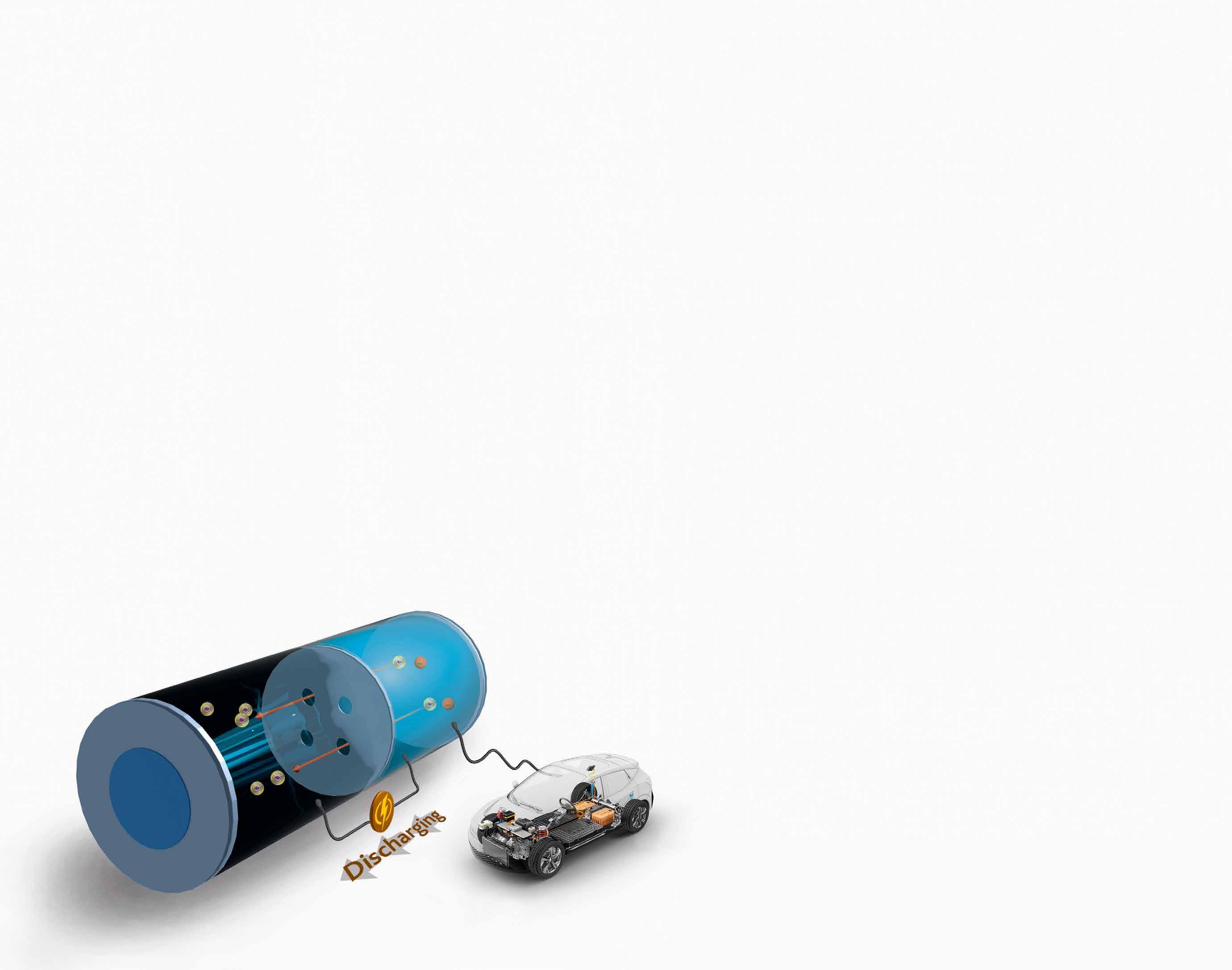
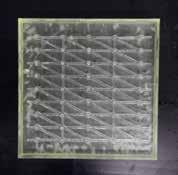
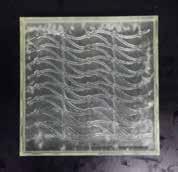
MetaBatt
Reconfigurable Metamaterials for Next Generation High-capacity Batteries
Project Objectives
The aim in the MetaBatt project is to develop reconfigurable metamaterials that change their shape in response to the environment, with a view to their potential application in the next generation of batteries. These nanostructures represent a new dimension in materials discovery, with potential applications in membranes and mechanical metamaterials.
Project Funding
Funded by the Independent Research Fund Denmark, Grant No: 0217-00111B.
Project Partners
Lawrence Livermore National Laboratory (USA), Dr. Xiaoxing Xia
Contact Details
Project Coordinator, Professor Ivano Eligio Castelli DTU ENERGY
Department of Energy Conversion and Storage Danmarks Tekniske Universitet Anker Engelunds Vej Building 301 , Room 108 2800 Kgs. Lyngby
T: +45 45258206
E: ivca@dtu.dk
W: https://www.dtu.dk/english/person/ ivano-eligio?id=62570&cpid=Castelli&enti ty=profile
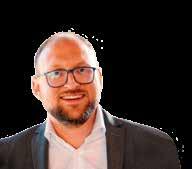
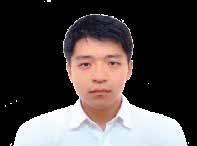
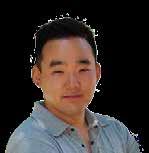
Ivano E. Castelli is a Professor in the Department of Energy Conversion and Storage at the Technical University of Denmark. His group focuses on discovering new materials using computer simulations to accelerate the green transition.
Joonyeob Jeon is a Ph.D. student in the department of Energy conversion and storage at the Technical University of Denmark, focusing on SEI formation, batteries, and nano-/micro-scale material coupling simulation using advanced numerical modeling techniques.
Jin Hyun Chang is an associate professor in the department of Energy conversion and storage at the Technical University of Denmark, expert in multiscale materials modelling and development of self-driving laboratories.
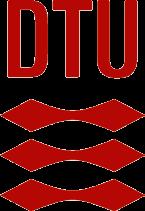
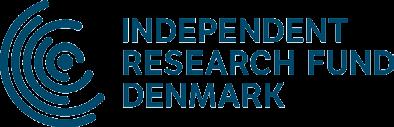