3D fluorescence microscopy on a sub-nanometer scale
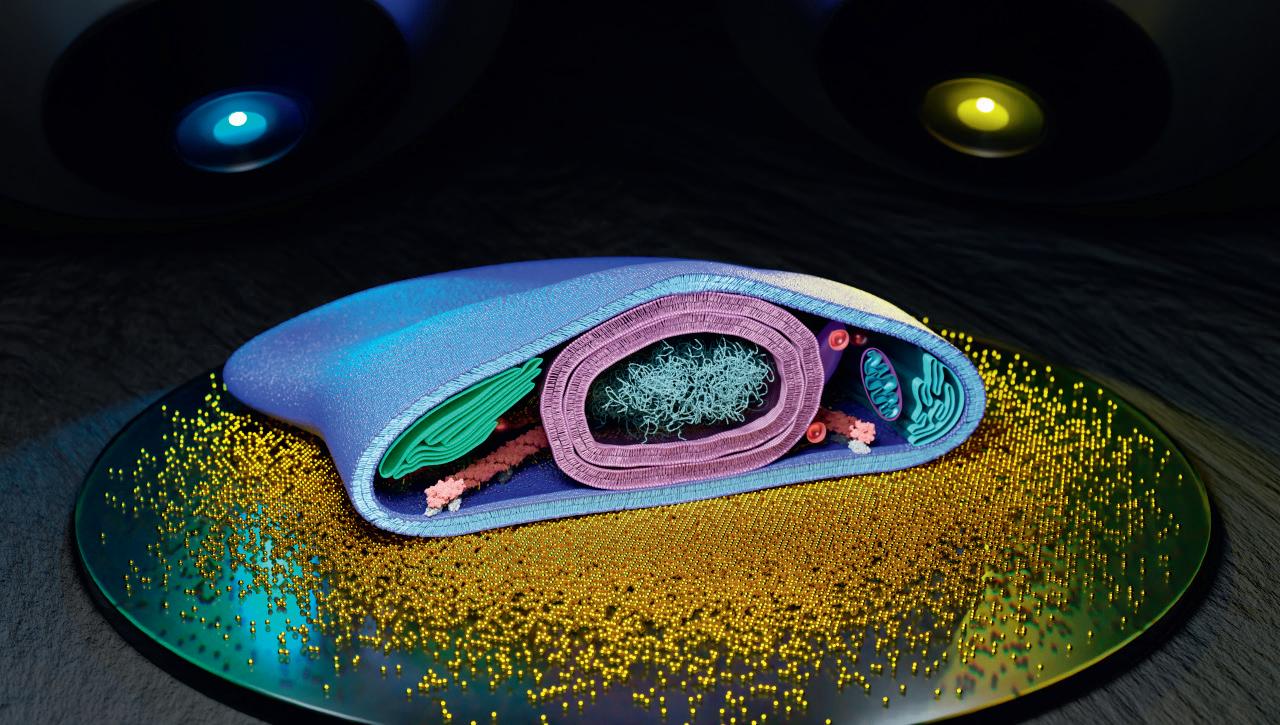
Today, microscopy is at such an advanced level that scientists are able to surpass physical barriers of resolution and visualize dynamic cellular processes occurring on a molecular level. We spoke to Dr. Jörg Enderlein, about his project Single-Molecule Metal-Induced Energy Transfer, a new method of three-dimensional fluorescence microscopy with a resolution on a molecular scale.
The classical diffraction limit is a physical barrier that restricts the resolution of conventional microscopy, making it impossible to resolve details smaller than ca. 250 nanometers with a classical microscope. Superresolution microscopy is a group of techniques in optical microscopy that can bypass this limit, and achieves resolutions ca. 100 times greater than classical microscopy. Super-resolution microscopy allows for the detailed visualization of subcellular structures and allows biologists to witness dynamic cellular processes occurring on a molecular level. Super-resolution microscopy is, therefore, a valuable tool for biological and biomedical research.
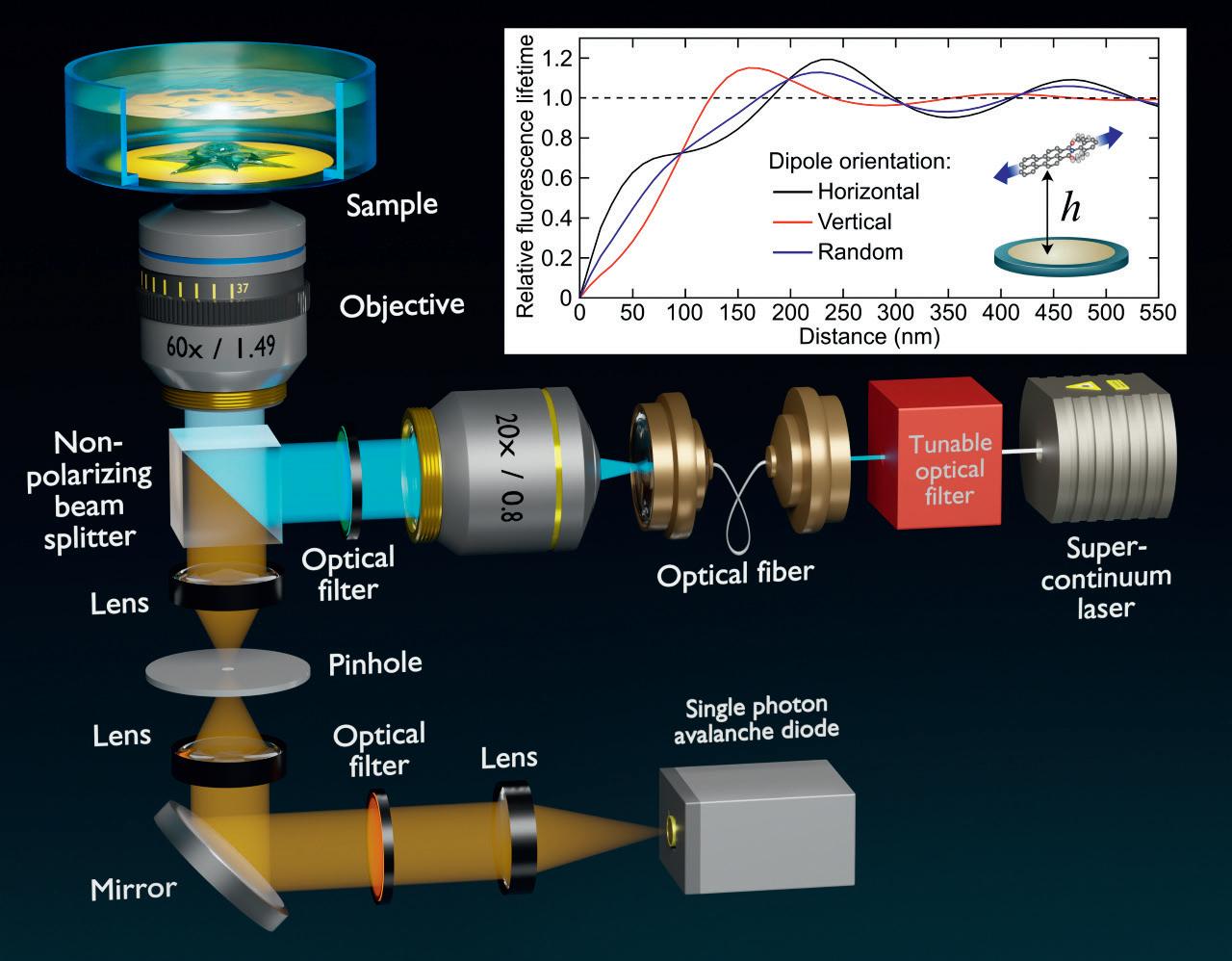
The first super-resolution method was Stimulated Emission Depletion (STED) microscopy, developed by Stefan Hell in the nineties of the last century. For his development, he shared the Nobel Prize in Chemistry in 2014, for “bringing optical microscopy to the nano dimension”. A few years later after the development of STED microscopy, alternative super-resolution methods that are based on the imaging and detection of single molecules in wide-field images were developed independently from each other by Eric Betzig and Xiaowei Zhuang in the US. The detectors of these microscopes are so sensitive that they allow the visualization of single fluorescent molecules in a sample, given that the molecules are sufficiently far apart from each other.
A few years ago, Dr. Enderlein and his team developed the technology of Single-Molecule Metal-Induced Energy transfer (smMIET)
which can be combined with the previously mentioned single-molecule localization superresolution microscopy to achieve “resolving of three-dimensional structures with nanometer isotropic resolution.” Even more, by combining smMIET with fluorescence correlation spectroscopy, it allows even to observe “structural dynamics on the nanometer length scale with nanosecond temporal resolution”.
Single-molecule localization microscopy
Dr. Enderlein explains the mechanisms behind single-molecule localization microscopy, which is the starting point of his project: “when you want to see the spatial distribution of certain proteins in a cell, you would normally label them with a fluorescent dye and visualize them with fluorescence microscopy. However, if you have fluorescent dyes which you can switch off into a dark state, you can switch them off and make them invisible. If at any point in time, you switch on very few of these molecules back into a fluorescent state, the image will look like a starry sky. So, you
see a dark background with very few fluorescent (light) molecules. This allows you to determine each of their positions with very high accuracy.” Having only one single molecule in a field of view permits the determination of the center position with very high accuracy, much better than the classical resolution limit that determines the size of a single-molecule image. “The idea is: I have very few molecules, I localize them, I find their center position, then I switch them off and I switch on another subset of my molecules in my sample. As I do this a thousand and thousand times, I localize the position of all the molecules in my sample, and with these coordinates, can reconstruct a very high-resolution image. This is the state of the art” explains Dr. Enderlein. This method is relatively simple to realize with a standard widefield microscope, and it can determine the position of molecules in two dimensions. However, since in reality, everything is three-dimensional, the challenge is localizing the positions of molecules along the third dimension, along the optical axis of the microscope.
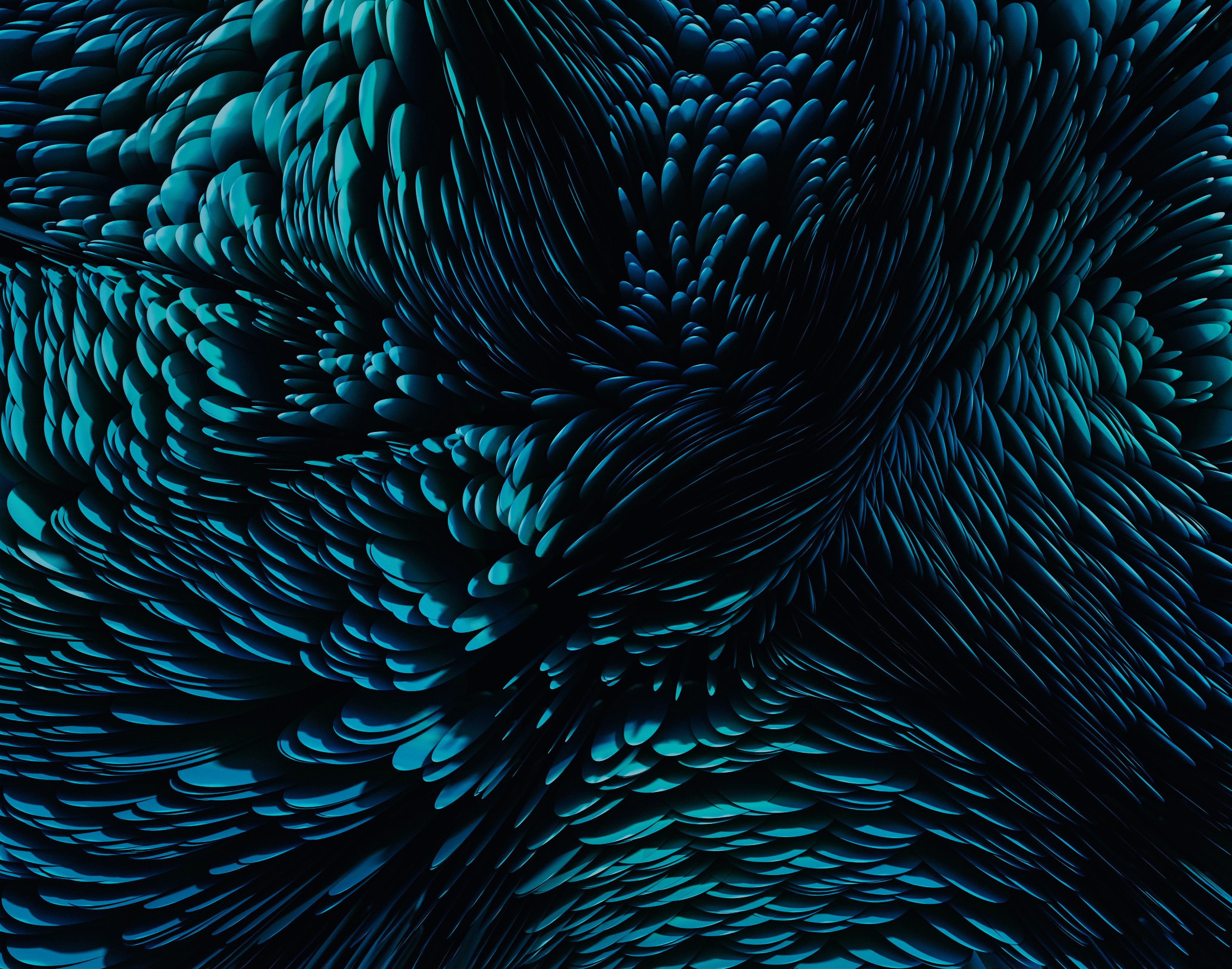
Metal-Induced Energy transfer
For this purpose, back in 2012, Dr. Enderlein and his group invented MIET, an idea based on a principle from physics called the Purcell effect. “When you take a fluorescent molecule and bring it very close to a metal, e.g a gold or silver surface, then what you will observe is that as the distance between the molecule and the surface becomes small, in the range below 200 nanometers, the brightness of the molecule becomes increasingly dimmer. Moreover, when you excite a molecule with light, it does not instantly jump back to its ground state by emitting a fluorescent photon, but it resides for a very short time in the excited state - a few nanoseconds. The average of this residence time in the excited state is called fluorescence lifetime, and this lifetime does also become shorter when the molecule comes close to a metal surface. We can measure lifetimes easily with a pulsed laser and a fast single photon detector. Since you have a dependency of the fluorescence lifetime on the distance of the molecules from a metal surface, you can use the lifetime measurement for localizing the molecule and thus determine the distance between the molecule and the metal surface. This works with an accuracy of a few nanometers” explains Dr. Enderlein. With this method, it is possible to localize one molecule along the optical axis with nanometer resolution, and by combining it with single-molecule localization it becomes possible to reconstruct three-dimensional images of a sample. “This is what we do, we can reconstruct three-dimensional images of a sample with nanometer isotropic resolution” concludes Dr. Enderlein.
The implications of this idea are very wide. This method can be used to resolve the global structure of macromolecular complexes and their dynamics. For example, reconstructing a three-dimensional image of the nuclear pore complex in the nuclear envelope of the cell. It can also be used to observe the organization and dynamics of lipid bilayers on a nanometer scale. Lipid bilayers are highly dynamic. A lipid membrane is composed of two leaflets of lipid molecules. Using this method, the researchers can distinguish if a protein is sitting in the upper leaflet or the lower leaflet. They can also observe protein-membrane interactions on a nanometer scale. The team is now exploring all the possible new applications of this general principle, not only for structural imaging but also for membrane biophysics, and for fast conformational dynamics of proteins, DNA, or RNA.
Dr. Enderlein believes smMIET is a tool that is comparable in its usefulness to conventional Förster Resonance Energy Transfer (FRET). One of the aims of Dr. Enderlein is to make his research as widely available as possible. He believes his method is easily reproducible, applicable, and transferable to other labs, which makes it practical for use in everyday research. “We are developing new optical microscopy technology for fundamental biomedical research. The idea is that we want to have three-dimensional fluorescence microscopy with a resolution on a molecular scale. We want to see the organization of protein complexes or the organization of proteins in membranes. This is a super-important method which is simple to implement so that everybody can use it, which is very important for its wide adoption by the community.”
smMIET
Single-Molecule
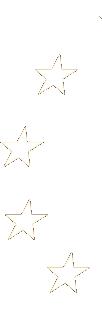
Metal-Induced Energy Transfer

Project Objectives
The core aim of the project is to develop the technology of Single-Molecule MetalInduced Energy Transfer (smMIET) for resolving macromolecular structure and dynamics with sub-nanometer spatial and nanosecond temporal resolution.
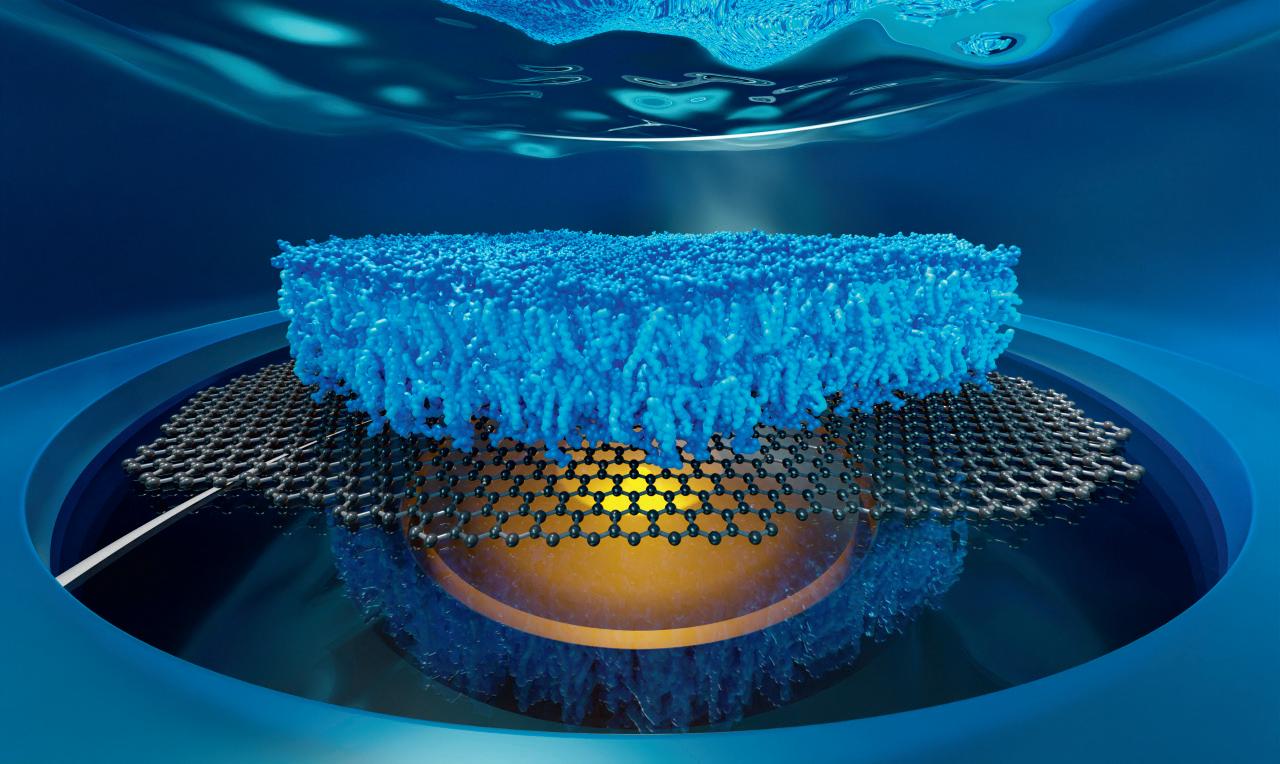
Project Funding
This project has received funding from the European Union’s Horizon 2020 research and innovation programme under grant agreement No 884488.

Project Partners
Prof. Dr. Stefan Jakobs (University Medicine, Göttingen, Germany) • Prof. Dr. Silvio Rizzoli (University Medicine, Göttingen, Germany) • Prof. Dr. Ralph Kehlenbach (University Medicine, Göttingen, Germany) • Prof. Dr. Kai Tittmann (Biology Department, Göttingen University, Germany) • Prof. Dr. Markus Sauer (Würzburg University, Germany) • Prof. Dr. Christoph Schmidt (Duke University, Durham, NC, USA) • Prof. Dr. Jordan Chill (Bar Ilan University, Raman Gat, Israel) • Prof. Dr. Gaby Frank (BenGurion University, Beer Sheva, Israel)
Contact Details
Project Coordinator, Dr. Jörg Enderlein
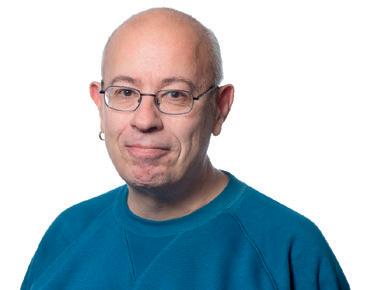
Third Institute of Physics - Biophysics Georg August University
Friedrich-Hund-Platz 1
37077 Göttingen
T: +49-551-39 26908
E: jenderl@gwdg.de : @JoergEnderlein
W: www.joerg-enderlein.de
W: https://cordis.europa.eu/project/id/884488
The idea is that we want to have threedimensional fluorescence microscopy with a resolution on a molecular scale.
Jörg Enderlein is a physics professor at the Third Institute of Physics (Biophysics) of the Faculty of Physics of the Georg August University in Göttingen. He is also founding Editor-in-Chief of the journal Biophysical Reports of the Biophysical Society.
We are developing new optical microscopy technology for fundamental biomedical research.Dr. Jörg Enderlein Artistic visualization of a biological cell with its various organelles (plasma membrane, nucleus with chromatin, Golgi apparatus, mitochondria, endoplasmic reticulum, cytoskeleton etc.) sitting on a gold covered glass slide observed by two microscopes at two different wavelengths. Artistic visualization of a lipid bilayer consisting of two leaflets of lipid molecules sitting on top of a single sheet of graphene and observed from below by a microscope objective. Principle of Metal-Induced Energy Transfer (MIET) Imaging.