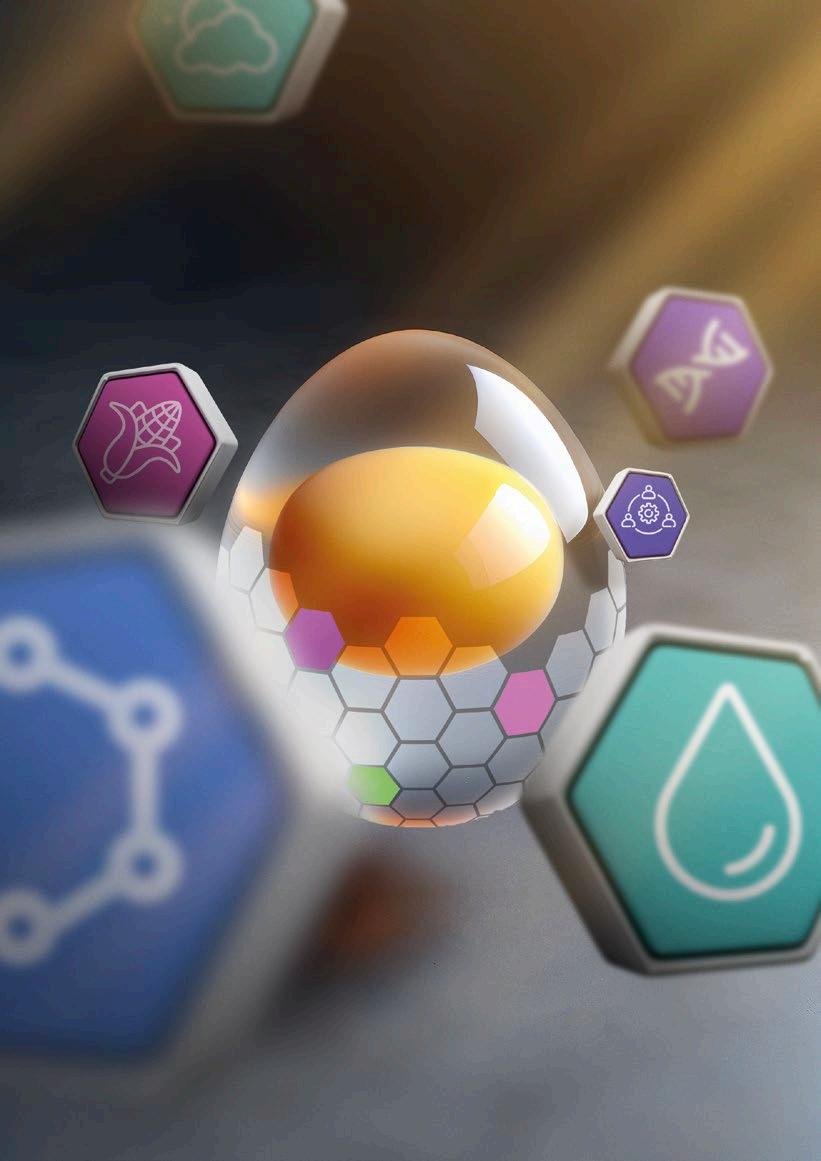
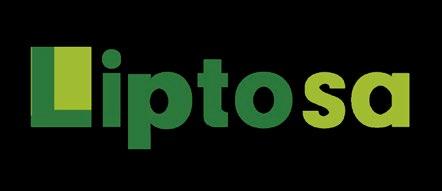
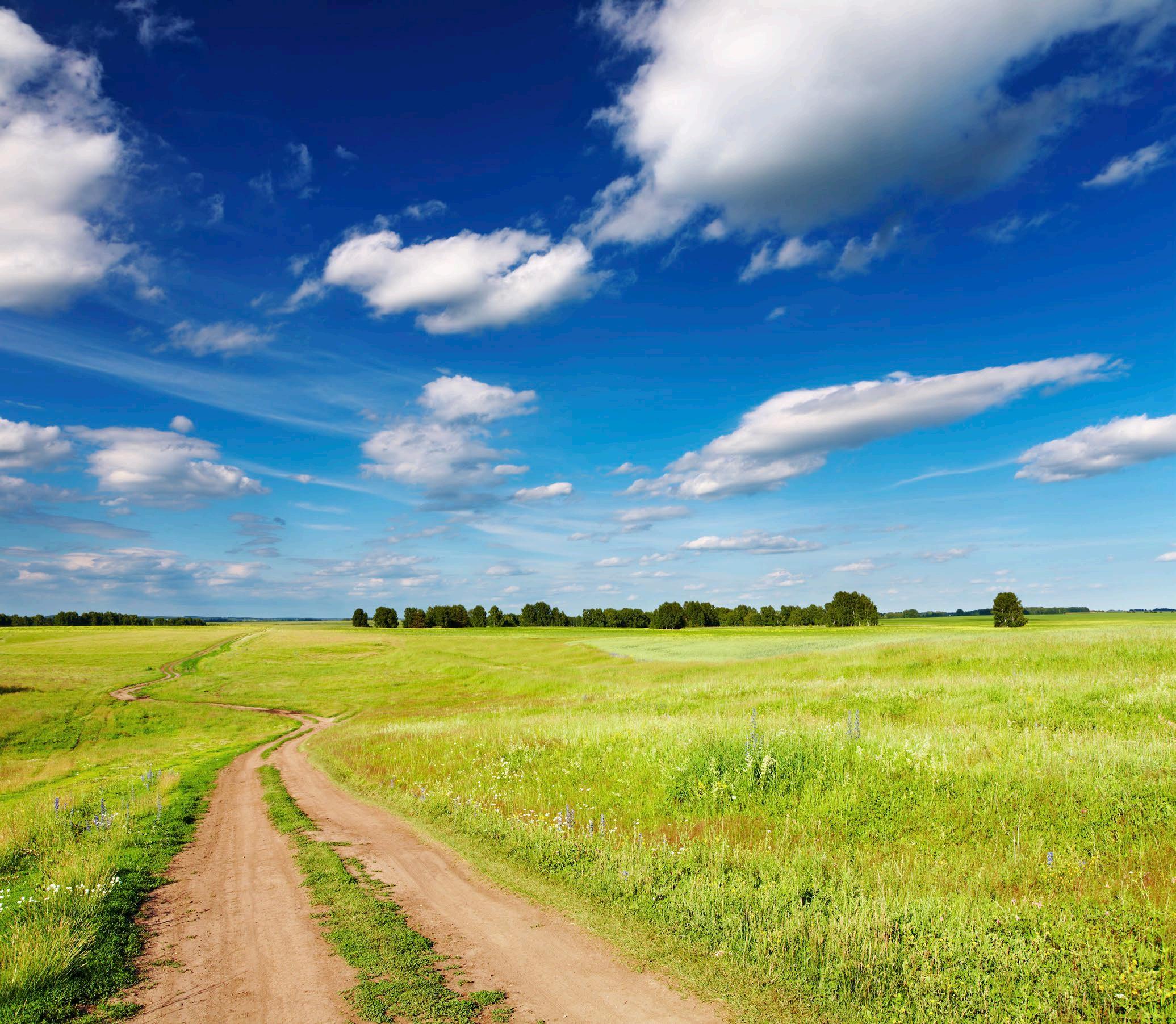


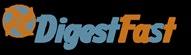
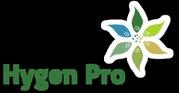
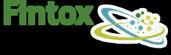
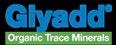


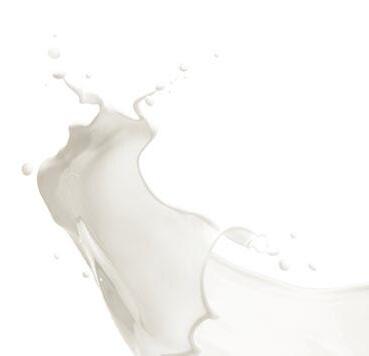
In an era of increasing demand for sustainable and efficient livestock production, phytogenics have emerged as one of the most promising solutions in animal nutrition. These plant-derived additives, encompassing essential oils, herbs, spices, and plant extracts, are reshaping the way we approach feed formulation for poultry and swine. As pressure mounts to reduce antibiotic use, enhance animal performance, and meet consumer expectations for sustainable production practices, phytogenics offer a multifaceted approach to tackling these challenges while fostering better animal health and productivity.
The global livestock industry is at a crossroads, balancing the need for profitability with growing scrutiny over production practices. Antibiotic growth promoters (AGPs) are now under stringent regulation or outright bans in many regions due to concerns over antimicrobial resistance. In this context, phytogenics have garnered attention as a natural, sustainable alternative
One of the most significant contributions of phytogenics lies in their ability to support gut health, a critical determinant of animal performance. The gastrointestinal tract plays a central role in nutrient utilization, immunity, and overall well-being. In addition to their antimicrobial activity, phytogenics have demonstrated antioxidant and anti-inflammatory properties, which are especially relevant in modern production systems.
Beyond animal health and performance, phytogenics align with consumer demands for natural, sustainable food production. Modern consumers are increasingly discerning, seeking products free from synthetic additives and antibiotics. Livestock producers incorporating phytogenics into feed programs can appeal to these preferences while differentiating their products in competitive markets. Moreover, phytogenics contribute to environmental sustainability by enhancing feed efficiency and reducing nitrogen and phosphorus excretion, thus lowering the environmental footprint of animal production systems.
As the livestock industry embraces the paradigm shift toward sustainable production, phytogenics are poised to play a pivotal role in shaping the future of poultry, swine and cattle nutrition.
In conclusion, phytogenics are not merely a trend but a transformative approach to animal nutrition. As science continues to uncover their multifaceted benefits, their role in addressing the challenges of modern animal production becomes increasingly evident. For producers, feed manufacturers, and nutritionists, the message is clear: embracing phytogenics is not just an opportunity but a necessity in the journey toward more sustainable and profitable livestock production.
Enjoy reading about this topic and many others we specially selected for the 4th edition of nutriNews International.
Gustavo Quintana-Ospina Technical Coordinator nutriNews International
EDITOR
COMMUNICATION GROUP
AGRINEWS LLC
ADVERTISING
Luis Carrasco +34 605 09 05 13 lc@agrinews.es
Gustavo Quintana +1 754 207 1272 g.quintana@agrinewsgroup.com
Simone Dias +55 11 9 8585-2436 nutribr@grupoagrinews.com
CUSTOMER SUPPORT
Mercé Soler
EDITORIAL STAFF
Álvaro José Guzman
Gustavo Quintana
TECHNICAL DIRECTION
Dr. Edgar Oviedo (poultry)
info@grupoagrinews.com nutrinews.com grupoagrinews.com
distribution magazine
AT VETERINARIANS AND TECHNICIANS Legal Deposit Nutrinews B11597-2013
Subscription price 125$
Legal Deposit: B15281-2024 ISSN 2938-8929
Images: Noun Project / Freepik/Dreamstime
It is time to update the old system of feedstuff nutrient evaluation to achieve precision nutrition
Dr. Gene Pesti PoultryHub, Australia
Table of Phytogenics Updated edition
Choose your potassium source wisely
Biozyme relies on ao-biotics to accomplish its mission
Bronchovest: The natural solution for respiratory and thermal support in animal production Technical Team Biozyme
Natu-B4: Polyherbal innovation in animal nutrition
M Naeem
Postdoctoral Fellow, Department of Poultry Science, Auburn University
Tainara Ciuffi E. Dornelas Doctor in Animal Production, Technical Coordinator of nutriNews Brazil
Maykelly da Silva Gomes1 , Gabriel Cipriano Rocha1 , Danyel Bueno Dalto2 , Pedro Silva Careli 1 , Jansller Luiz Genova1
1Muscle Biology and Nutrigenomics Laboratory, Department of Animal Science, Universidade Federal de Viçosa, Viçosa, Minas Gerais, 36570-900, Brazil
2Sherbrooke Research and Development Centre, Agriculture and Agri-Food Canada, Sherbrooke, Quebec, J1M 0C8, Canada
of Aberdeen in Scotland, Freelance writer
processing in cattle feeding
Breno Luis Nery Garcia
Dr. Gene Pesti
The PoultryHub, Australia
The primary method for describing the gross nutrient composition of feed ingredients and feeds has changed little since the US Food and Drug Administration adopted the “proximate analysis” method in 1888. As analytical chemistry advanced, the knowledge of feed composition improved, and separate requirements were determined for each new nutrient, mineral, vitamin, amino acid, and some essential fatty acids.
However, the proximate analysis has persisted for the feedstuff trade and for estimating the energetic contributions of feed ingredients.
Feed chemistry was in its’ infancy when the proximate analysis, or Weende Method, was conceived in the city of Weende, in the Hanover Kingdom, in the 1860’s. The true nature of proteins and the other constituents was unknown.
Dividing feed ingredients into the crude albuminous material, or protein, ether extract, crude fiber, and ash fractions, was state of the art then.
Whatever was left over was called the “nitrogen-free extract” despite not being an extract at all. It was supposed to represent soluble or available carbohydrates.
The proximate analysis method has some helpful features.
For instance, the nitrogen-free extract is found by difference, so the analytical results always add up to 1,000.00 g/kg.
In reality, there are no perfect methods for chemically defining feed ingredients.
Using one technique or set of techniques for analyzing all ingredients should not be expected to give perfect answers every time.
Finding and understanding why the composition of ingredients does not sum to 1,000 g/kg should lead to a better understanding of just what is in all the different feed ingredients.
The difficulty in describing feed ingredients is due to the great variety of nutrients and other chemicals across and within plant and animal species
The proteins in each ingredient have different amino acids. Consequently, the proteins’ nitrogen and energy contents are unique for each ingredient
Starch from different strains of even one crop type, like maize, will have different branching among their glucose units and different molecular weights and physical properties.
For instance, dent corns are used for feed, while waxy corns are used to make corn starch for food and corn gluten feed for animals.
The same applies to oligosaccharides, non-starch polysaccharides, pectins, lignin, and hemicellulose. Only cellulose is free of branching glucose chains, but the fibers can be of different lengths in different species and cultivars.
Some of the main lessons learned since the Weende Method (Proximate, meaning close), analysis was introduced in 1864 include:
Protein comprises amino acids, which contain different amounts of nitrogen. However, many other compounds, like nucleotides, resins, nitrates, nitrides, and phospholipids, also contain nitrogen.
Crude fiber contains unabsorbed plant cell wall components, including lignin, but not all undigested and unabsorbed material can be fermented in the animals’ hindgut (true fiber).
True fiber is the sum of the non-starch polysaccharides (pectin, hemicellulose, and cellulose) and lignin.
Plants contain amino acids as protein, free amino acids, and peptides. Animals can use all of them for protein synthesis. From the animal’s perspective, the sum is called “True Protein”.
The oligosaccharides, with 3 to 12 sugar residues, are good animal energy sources and have prebiotic properties, altering the animal’s intestinal microflora.
The choline in lecithin (phosphatidyl choline) and betaine can be converted into glycine for amino acid synthesis by animals.
Pectins can be good sources of energy for animals through fermentation. They also have prebiotic activities and enhance immune responses.
Ether extract contains neutral lipids like the triacyl glycerides but also
Depending on the method used to determine ash, some minerals may be volatilized, and ash residue may contain bound carbon, oxygen, and sulfur.
Advances in analytical chemistry are again making it possible to define feed ingredients further to advance nutritional science. An Australian Feed Ingredient Database (AFiD) has been developed (Moss, 2024).
It includes more detailed information on the carbohydrate contents of typical feed ingredients than earlier databases. The AFiD database includes information on several five and six-carbon sugars that are found in many feed ingredients for poultry and swine.
The Armidale Method of categorizing the chemicals in feed ingredients was first conceived as a teaching tool. It shows students how modern 21st-century chemical knowledge relates to 19thcentury methods still in everyday use.
Data from the AFiD database and Feedipedia.com were combined to create a new feed ingredient database based on the Armidale Method
The Armidale Method has been proposed as a more relevant method to describe feed ingredients based on our understanding of modern knowledge of the actual chemical composition, and not just components that are soluble or insoluble in various solutions, like the Weende Method.
To categorize feed ingredient chemicals by the Armidale Method, the following measurements must be made:
Sugars refers to the free sugars in an ingredient. They include mono and diglycerides like glucose, fructose, sucrose, xylose, and mannose.
Oligosaccharides consist of sugar polymers of 3-12 residues.
Starch refers to sugar polymers of more than 13 glucose residues.
Neutral Lipids are compounds soluble in hexane, ether, and similar solvents. They include triacylglycerols, diacylglycerols, monoacylglycerols, free fatty acids, hydrocarbons such as waxes and steroids, chlorophyll, and pigments.
Polar Lipids, including phospholipids, sphingolipids, and glycolipids, have limited solubility in hexane and ether and are better extracted in solvents like methanol.
Lignin refers to polymers of phenolic residues. Therefore, they are not polysaccharides. Not only is lignin resistant to degradation, it is covalently bonded to cellulose and hemicellulose, which inhibits their degradation.
Cellulose, like starch, consists of polymers of glucose. Cellulose differs from starch in the type of linkage joining the glucose residues. Unlike starch, cellulose contains only linear chains of glucose.
Pectins are heteropolysaccharides found in the cell walls of terrestrial plants. Their primary units are sugar acids, mainly galacturonic acid. Pectins are soluble fibers that bind sugars and steroids in the gut and slow absorption and have immunomodulatory effects.
Ash refers to the residue after burning the ingredient at 550 oC to remove all the carbon. It consists mainly of silica and other minerals.
Hemicellulose refers to polymers that include 5-carbon sugars (like xylose and arabinose) and 6-carbon sugars (like mannose and galactose) and their sugar acids. Hemicellulose can contain branched chains of residues.
There are four types of hemicelluloses found in different plants: xylans, mannans, mixed linkage beta-glucans, and xyloglucans.
True Protein is the sum of all the amino acid residues in a feed ingredient. It can be thought of as representing the potential protein made from the feed ingredient.
It does not distinguish between the amino acids in the ingredient that come from protein or peptide-linked amino acids and free amino acids.
Methods to measure true protein in milk measure the peptide bonds, excluding any free amino acids.
Non-Protein Nitrogenous Compounds include chemicals like DNA, RNA, phospholipids, betaine, nitrites, and nitrates. The choline in phospholipids is metabolized to betaine and then glycine.
While phosphatidylcholine and betaine are not part of the true protein in feed ingredients, they can become parts of animal protein after ingestion due to their conversion to glycine.
And Moisture
Then, the following calculations yield categories 9 – 12:
Cellulose = ADF -Lignin
Hemicellulose = NDF – ADF
True Protein = Sum of the Amino Acid residues
Non-Protein Nitrogenous Compounds = Crude Protein – True Protein
measurements that must be made are:
Amino Acids in the ingredients are determined by standard methods.
Nitrogen in the feed ingredient is determined by either the Kjeldahl or Dumas Combustion procedures.
Neutral Detergent
Fibre measures the total cellulose, hemicellulose, and lignin in the cell walls of feed ingredients.
Acid Detergent
Fibre measures the cellulose and lignin in the cell walls of feed ingredients.
And:
Total Non-starch Polysaccharides
(Total NSP) = Pectin + Cellulose + Hemicellulose
Total Polysaccharides = Starch + Total NSP
True Fibre is the sum of the Pectin, hemicellulose, cellulose, and lignin.
There has been much conversation about “precision nutrition” and how it should be developed in the future.
However, it isn’t easy to make more precise feed formulas when they are based on the feed’s energy content, and this value is primarily based on 1860’s technology.
A new system for describing feed ingredient composition is needed to make more precise feed formulas, especially ones with various proteases and carbohydrases.
The Armidale Method is a potential possibility. Much discussion amongst theoretical and practical nutritionists, analytical chemists, and statisticians is needed to refine and combine 160 years of advancements into a new system to facilitate further progress.
Sorghum Moisture 12%
Pectins 0% Hemi-cellulose 6%
Crude Protein 9% Lignin 1%
3%
0%
3%
0% Moisture 11%
Figure 4. The composition of soybean meal using Weende (Proximate) Analysis (Left) and the Armidale Method (Right).
Figure 5. The composition of canola meal using Weende (Proximate) Analysis (Left) and the Armidale Method (Right).
It is time to update the old system of feedstuff nutrient evaluation to achieve precision nutrition DOWNLOAD ON PDF Bibliography upon request.
DISTRIBUTION
SPECIES & DOSAGE
COMPONENTS (NONPHYTOGENICS)
Pigs and poultry: 100200 g per ton of complete feed.
Poultry chicks: 100 ml Miarom Intest L per 1,000 l drinking water.
Poultry: 100-250 ml Miarom Intest L per 1,000 l drinking water.
Piglets and fattening pigs: 50-100 ml Miarom Intest L per 1,000 drinking water.
Pigs: 200500 g per ton of complete feed.
Worldwide
Poultry: 150350 g per ton of complete feed.
Chicks: 100 ml Miarom Classic L per 1,000 of drinking water.
Poultry: 100-250 ml Miarom Classic L per 1,000 of drinking water.
Piglets: 150 ml Miarom Classic L per 1,000 of drinking water.
Fattening pigs/Sows: 200 ml Miarom Classic L per 1,000 of drinking water.
COMPONENTS (PHYTOGENICS)
Coated essential oils and milled phytogenics.
Complementary feed, which is a special combination of essential oils from caraway, star anise, chamomile, ginger, cinnamon and oregano. It is increasing the daily weight gain as well as improving the feed conversion, by positively influencing the gut health.
Miarom Intest L is a liquid complementary feed that provides a specific combination of diverse phytogenics including the active components of caraway, star anise, chamomile, ginger, cinnamon and oregano. The perfect synergism of beneficial herbs that ensures the health of the intestine through prevention and in case of intestinal health challenges. On farm level, administration is easy and flexible thanks to the drinking water application.
Essential oils.
Complementary feed, which is a synergistic combination of essential oils from thyme, anise, peppermint and eucalyptus, among others. It’s supporting the respiratory health and on top of that improving the air quality in the houses.
Miarom Classic L is a liquid complementary feed supplement which comprises a special combination of various essential oils. Its long-lasting benefits are obtained from a synergistic, standardised combination of essential oils from thyme, anise, peppermint and eucalyptus, among others. It’s supporting respiratory health and alleviating heat stress. On farm level, administration is easy and flexible thanks to the drinking water application.
Electrolytes.
Piglets: Administer orally 2 ml (1 pump). PRODUCT ACTION
Miarom Intest C
Miarom Intest L
Miarom Classic P
Miarom Classic L
Tannins from chestnut extract and essential oils.
MiaFirstAid L A liquid dietary complementary feed for stabilisation of water and electrolyte balance to support suckling piglets during diarrhea incidences. In case of risk and during periods of, or during recovery from digestive disturbance (diarrhea).
DISTRIBUTION
SPECIES & DOSAGE
All species: 1001000g/tn of feed. Consult your Nuproxa technician to establish a personalized dosage.
All species conventional diets: 50% substitution of synthetic methionine. Organic diets: 0.5 -1.5 kg per ton of feed. Consult your Nuproxa technician to establish a personalized dosage.
All species organic diets: Up to 1 kg per ton of feed. Consult your Nuproxa technician to establish a customized dosage.
COMPONENTS (NON- PHYTOGENICS)
COMPONENTS (PHYTOGENICS)
PRODUCT ACTION
Phosphatidicoline, rutin, terpenoid.
Phosphatidylcholine isomer and its conjugates that replace choline chloride in diets, contribute to modulate fatty acid metabolism, liver health and PPARα receptor activation.
Natu-B4™
Organosulfurates.
Optimizer of methionine metabolism. In conventional diets it partially replaces synthetic methionine and in organic diets it acts as a source of methionine.
OptiMethione™
Organosulfurates and flavonoids.
Lysine bioavailability optimizer. In organic diets, it acts as a lysine supplement.
OptiLysin™
Worldwide
All species: 500-1000 g/ton of feed. Consult your Nuproxa technician to establish a customized dosage.
All species: 250g/tn of feed. Consult your Nuproxa technician to establish a customized dosage.
Poultry, Swine, Rabbits and Ruminants: Maximum dosage of 100 g/tn of feed. Consult your Nuproxa technician to establish a customized dosage.
All species: 100-500g/tn of feed. Consult your Nuproxa technician to establish a customized dosage.
Terpenoids such as saponins and tannins among other polyphenols.
Improved animal productive performance by improving intestinal health through modulation of intestinal microbiota, reduction of chronic inflammation and oxidative stress. In addition, it contributes greatly to the control of intestinal protozoa.
NuxaSan 500™
Terpenoids and flavonoids.
Natural additive that improves the productive performance of animals due to its protective effect on liver function, thanks to its antioxidant, detoxifying and bile secretion stimulating activity.
LivoLiv 250™
Polyphenole.
Natural metabolic antioxidant for the protection of animals from oxidative stress contributing to the improvement of productive, reproductive and meat quality parameters. Partial substitute of synthetic Vitamin E reducing the formulation cost.
NuxaFen™
Polyphenols, gallic acid and its derivatives.
Herbal Vitamin C substitute, used with adaptogenic effect, slowing down oxidative stress and heat stress. It is stable in aqueous medium, at environment temperature and at granulation temperatures.
C-Power™
DISTRIBUTION
SPECIES & DOSAGE
Poultry, Swine, Fish, Horses and Pet Animals: 50 to 150 g/ton of feed Cattle400 to 2400 mg/ animal/day.
COMPONENTS (NON- PHYTOGENICS)
COMPONENTS (PHYTOGENICS)
PRODUCT ACTION
Macleaya cordata extract and Macleaya cordata dehydrate.
Worldwide
Poultry, Swine and Ruminants: 50 to 100 g for 1.000L of water.
Poultry and Swine: 100g for 1.000L of water.
Swine, Poultry, Dairy, Beef, Ruminant. See label for recommended dosage levels.
Swine, Poultry, Dairy, Beef, Ruminant. See label for recommended dosage levels.
Swine, Poultry, Dairy, Beef, Ruminant. See label for recommended dosage levels.
Worldwide
Sangrovit Extra
Macleaya cordata extract and Macleaya cordata dehydrate and organic acids.
Additive that improves food intake and intestinal health.
Sangrovit WS
Sangromix AC
Microfused® Essential Oils: Specific Blend of Phytonutrients.
Regano® Dry Most trusted natural feed additive to help animals respond to stress events; contains essential oils.
Microfused® Essential Oils: Specific Blend of Phytonutrients.
Regano® Liquido Most trusted natural water additive to help animals respond to stress events; contains essential oils.
Actifibe® Prebiotic
Swine, Poultry, Dairy, Beef, Ruminant. See label for recommended dosage levels.
Swine. Mix at the rate of 2 lbs. per ton with other ingredients to make a complete feed.
Bioactives From Colostrum, Actifibe® Prebiotic Swine. Give orally 2 ml (1pump) per piglet within the first 24 hours after birth. Repeat in 24 hours as needed. Can also be used for fall behind pigs. Give orally 2 ml (1pump) per pig.
Microfused® Essential Oils: Specific Blend of Phytonutrients.
ProsperEO™ Dry Everyday natural feed additive, containing essential oils, that supports a broad range of health challenges.
Microfused® Essential Oils: Specific Blend of Phytonutrients.
ProsperEO™ Liquido Everyday natural water additive, containing essential oils, that supports a broad range of health challenges.
Microfused® Essential Oils: Specific Blend of Phytonutrients.
Dual Defender™ Natural feed supplement that helps support your feed biosecurity program.
Microfused® Essential Oils: Specific Blend of Phytonutrients.
First Pulse® D Natural oral drench to get baby pigs up and eating quickly.
By Jorge Castro, LATAM Technical Services Manager, Arm & Hammer
Animal Nutrition
Potassium is an essential nutrient needed by animals to maintain productivity and mitigate heat stress. It’s also a mobile nutrient, meaning it doesn’t leave reserves in the body and must be replenished in the daily ration. Without replenishment, animals can become deficient and experience reduced growth, muscle weakness or stiffening, reduced feed intake, nervous disorders, heart palpitations and more.
Adding potassium sources to the ration takes careful management. Potassium is part of a critical electrolytic balance, which must be maintained when supplemental potassium is added to the diet. Certain potassium sources can also cause ration heating and nutrient loss, so it’s important to minimize the risk of heating as much as possible.
Finding the right balance comes down to your potassium source.
There are two common formulas for electrolyte balancing in animal nutrition, which help us understand potassium’s important role in rations.
The dietary electrolytic balance (dEB) formula is:
(Sodium + Potassium) – Chlorine
The dietary cation-anion difference (DCAD) formula is:
(Sodium + Potassium) – (Chlorine + Sulfur)
Both formulas are commonly manipulated to maximize animal health and productivity. Dietary levels of cations (sodium and potassium), which are positively charged ions, and anions (chlorine and sulfur), which are negatively charged ions, can be adjusted to create a balance that’s either positive or negative.
The relationship between these elements is closely linked. That means no single element can be looked at individually, as it takes a balance of all to maintain and optimize animal health.
The animal’s body has a buffering system to maintain near-normal physiologic pH and prevent most imbalances. However, many factors can impact element levels at any given time. For example, daily maintenance needs, production, and environmental factors like heat stress drain potassium, so extra dietary potassium is often required to maintain levels.
Wheat, corn and soybean-based diets are commonly considered “sufficient” in potassium supply. However, animals typically need a higher dEB/DCAD than what’s supplied in the ration without potassium supplementation. To reap the production benefits of a higher dEB/DCAD, supplemental sodium and potassium are needed.
For example, in dairy cattle, pre-calving diets are designed for negative DCAD to prevent hypocalcemia, where lactating diets are designed for positive DCAD to support milk production.
Potassium carbonate, found in Arm & Hammer DCAD Plus™, is the best choice for adding potassium into the ration.
Many nutritionists and farmers use potassium chloride; however, potassium chloride has a distinct drawback when it comes to electrolyte balance.
Potassium’s positive ionic charge and chloride’s negative ionic charge cancel each other out in the electrolytic balance. In other words, dEB or DCAD can’t become more positive to support production needs.
Potassium carbonate works differently because it only supplies potassium. It can be used to create a more positive dEB/DCAD, helping animals maintain production.
Potassium sources typically generate heat when they are exposed to moisture. The heat generated ends up heating the entire ration, which can cause the feed to “cook” and lose essential nutrients. Protein is particularly susceptible.
Feed bins or silos can even generate steam when cooking occurs. A steaming feed pile should be spread out on the ground to allow feed to cool down. Fire is another risk.
Animals eating hot feed also feel the effects. Reducing ration heating is a necessary heat stress abatement strategy.
Choosing the right potassium source can help minimize ration heating risk, along with adding potassium to the ration near the end of feed mixing.
There are three primary sources for potassium in the diet:
Potassium bicarbonate
Anhydrous potassium carbonate
Potassium carbonate sesquihydrate (DCAD Plus™)
Potassium bicarbonate doesn’t cause ration heating. But the bicarbonate form only contains half of the potassium compared to its carbonate counterpart. As a result, potassium bicarbonate costs about twice as much, making it impractical to use.
Anhydrous potassium carbonate generates a lot of heat when exposed to moisture, making ration heating a big concern.
DCAD Plus™ uses potassium carbonate sesquihydrate. This form of potassium does not generate heat because it has been partially hydrated – removing the potential for heating up in a moist ration.
Many species can benefit from potassium carbonate in the diet. Most research has been done in lactating and nonlactating dairy cows, and nutritionists are comfortable managing DCAD levels in dairy rations.
While less widely adopted, poultry operations can apply the same principles to broiler and layer diet management with similar production and heat stress mitigation results.
Low potassium levels are dangerous for birds and can cause high blood pressure, heart disease and sudden death, especially in high-salt diets. Increasing potassium and reducing salt can help lower blood pressure and reduce risk of stroke.
Low potassium levels can also reduce calcium needed for strong bones and shells.
Additionally, electrolyte imbalance can cause metabolic issues like tibial dyschondroplasia and respiratory alkalosis in layers. Too much dietary sodium relative to potassium, paired with high chloride levels, can cause tibial dyschondroplasia. When chloride or sulfur levels are high, overall electrolyte balance is especially important – and cations (sodium and potassium) are needed to offset the negatively charged anions.
Birds are also susceptible to loss of potassium during times of heat stress, and sodium bicarbonate alone may not mitigate this stress.
Potassium carbonate can be added to the diet year-round and adjusted when needed to balance electrolytes and optimize production.
Visit the Arm & Hammer website at ahfoodchain.com to learn more about potassium carbonate and DCAD Plus™, and find your local representative.
https://ahfoodchain.com/en
Choose your potassium source wisely DOWNLOAD ON PDF VISIT WEBSITE
Biochem offers BronchoVest® as part of its product portfolio, an additive composed of essential oils of eucalyptus and mint, along with menthol crystals. BronchoVest® has been carefully designed to support the respiratory functions of animals.
It can be administered via spray or drinking water, with rapid absorption through the mucous membranes, making it ideal for targeted applications during challenging times for the animals.
The composition of BronchoVest® provides support for the animals’ respiratory system, acting preventively to reduce the negative effects of respiratory challenges and heat stress.
Thus, the synergistic action of BronchoVest’s ingredients is evident, as their complementary characteristics support respiratory functions and animal welfare.
Eucalyptus oil, with a high concentration of the active compound 1,8-cineole, helps to improve lung function. It restores the natural activity of the respiratory epithelium and reduces mucus buildup in the bronchioles.
Mint oil regulates excessive mucus production, increasing air ow to the lungs.
Menthol crystals have e ects on speci c sensory receptors, providing a cooling sensation, particularly in situations of heat stress.
Initial studies with BronchoVest® were conducted on poultry. These studies demonstrated key benefits such as reduced negative respiratory effects during challenges, standardization of vaccine titration when used post-vaccination, reduced postvaccination reactions, decreased losses at processing plants, and improved zootechnical performance in broiler chickens, heavy breeders, and commercial layers.
More recently, studies conducted in Brazil with pigs during nursery, growth, and finishing phases showed a reduction in postpartum respiratory rates of sows, decreased need for medication in nursery piglets, reduced negative respiratory effects during challenges for nursery and finishing pigs, lower piglet mortality, and better zootechnical performance across evaluated categories.
Similarly, for calves, the use of BronchoVest led to improved overall health status and reduced need for medication.
In conclusion, BronchoVest®, whether applied through drinking water or spray, has proven efficacy and can contribute to enhanced animal welfare, thereby supporting the expression of genetic potential for performance.
and Thermal Support in Animal Production
ON PDF
Technical Team Biozyme
Digestive health is key to successful animal production. At BioZyme® Inc., we know that good digestive health, as well as nutrition, are the cornerstone of a good health protocol.
Nutrition and health work synergistically. It’s fairly well-known that 70% of the immune system is found within the digestive system, and a healthy immune response leads to a healthy animal. That is one reason that BioZyme places such an emphasis on digestive health in its nutrition products and feed additives.
BioZyme has served the agriculture industry as an innovator in fermentation and animal health for more than 70 years. What started as a small feed store in the Saint Joseph, Missouri Stockyards has flourished. Today, BioZyme is a global company that manufactures and sells its own line of fermentation products, AO-Biotics®, produced from a proprietary strain of the Aspergillus oryzae, a filamentous fungus.
In addition to these products, the BioZyme family of brands manufactures supplements and animal health products for a variety of animals, including cattle, swine, poultry, sheep, goats, horses, dogs and cats.
At BioZyme, our motto is to provide care that comes full circle. That means we want to provide the best possible care to every animal every day. We do that by providing research-proven products to solve animal agriculture’s greatest challenges.
Our mission: an undeniable impact on the health and wellness of your animals and your business.
BioZyme uses fermentation and knowledge to enable passionate solutions that promote healthy animals. In 1968, BioZyme’s founder, Larry Ehlert, purchased a patent and marketing rights to an unheard-of direct-fed microbial produced from Aspergillus oryzae.
Today, that product is now known as a prebiotic and is marketed as AO-Biotics® Amaferm®. More recently BioZyme has leveraged fermentation expertise to release a postbiotic for layers, AO Biotics® EQE, also derived from A. oryzae.
AO-Biotics Amaferm is a prebiotic researchproven to enhance digestibility. It has more than 111 published and/or presented research studies proving its increase in digestibility and, ultimately, its impact on the animal. The result of including Amaferm in the diet can be succinctly summarized as more performance for less cost.
“Amaferm stimulates the growth of energy-harvesting bacteria, resulting in greater volatile fatty acid production (VFA) in the rumen. Volatile fatty acids serve as the primary energy source for ruminants, and an improvement in VFA production by 16% from feeding Amaferm is nutritionally the same as feeding a pound of DDGs per head per day,”
said Chris Cassady, Ph.D., BioZyme Director of Beef Technical Sales.
“Unlocking more energy from within affords the animal additional energy available for growth, improved body condition and an earlier onset of puberty. Elevated energy levels have also been shown to improve the production of follicle stimulating hormone (FSH) and luteinizing hormone (LH), which are crucial to the effective onset of ovulation in heifers. Obviously, these are essential for reproductive success, meaning that the prebiotic Amaferm can help support breed-ups without any risks of grain-fed, overweight heifers.”
In 2023, BioZyme introduced AO-Biotics EQE, the first-and-only AO postbiotic developed specifically for layers. Unlike many other postbiotics, AO-Biotics EQE starts with BioZyme’s proprietary strain of AO and is tailored to address specific production challenges.
“Through the AO-Biotics brand, BioZyme aims to offer a line of prebiotics and postbiotics matched to specific animal health challenges, well-being and productivity. The way we have developed these products is truly innovative and a game-changer for the industry,”
said Cesar Ocasio, Ph.D., Senior Manager of Business Development and Innovation.
BioZyme manufacturers innovative products for all species of animals at our locations in Saint Joseph, Missouri and Lexington, Kentucky. Our products are research-proven and powered by Amaferm or AO-Biotics EQE
AO-Biotics EQE is research-proven to:
Produce more sellable eggs.
Improve egg mass.
Increase productive life span.
In addition, we provide various services and resources to producers and partners to ensure the success of their businesses. This includes hay testing for customers and easyto-use gestation calculators for your cattle, sheep and goats.
BioZyme® Relies on AO-Biotics® to Accomplish its Mission DOWNLOAD ON PDF
The growing demand for improved efficiency in animal nutrition without compromising health, welfare and environmental balance has increasingly driven the search for natural solutions.
Natu-B4™, present in the global market since 2003, is today a tool that goes far beyond replacing synthetic choline chloride, promoting improvements in performance and reduction of carcass fat deposition through changes in energy partitioning.
In nearly 2 decades of application development and product validation under the most diverse conditions, Nuproxa Switzerland has conducted several studies with Natu-B4 on 10 different species, resulting in more than 25 scientific publications that make up the most complete, solid, diversified and reliable scientific dossier of its product range.
Natu-B4™ is composed exclusively of plants, including Solanum nigrum and Achyranthes aspera, among others, and is rich in phospholipids, phosphatidylcholine, phosphatidylethanolamine and phosphatidilinositol, as well as other bioactive molecules such as Rutin.
The study by White et al. (2019) objectively demonstrated that its main mechanism of action focuses on the activation of peroxisome proliferatoractivated receptors (PPAR-α), which play a key role in fatty acid metabolism and nutrient utilization.
Unlike choline chloride, which has a more limited impact on this function, Natu-B4™ activates several metabolic pathways, improving energy homeostasis and promoting better fat utilization. To this day, Natu-B4™ remains the only product on the market that has scientifically demonstrated its mechanism of action.
Acetil COA Carboxilase (ACC) +12,18%
Use of Fatty Acids and Gluconeogenesis
Fatty Acid Synthase (FAS) +11,41% Fatty Acid Binding Protein (FABP) +18,39% Fatty Acid Transport
Peroxisome proliferator activated receptor (PPAR) +39,03% Adiponectin +14,61% Perilipin (PLIN) +24,97%
Enzymes Involved in Fatty Acid Synthesis
Regulation of Glucose Levels and Fatty Acid Breakdown
Pyruvate Kinase: +159,51% Cholesterol Acyltransferase (ACAT): +16,15%
Phosphoenolpyruvate Carboxykinase (PEPCK):+47,69%
An interesting detail is that the active compounds are presented in Natu-B4™ in the form they are found in nature, within plant matrices, which confers them a high stability and capacity to be integrated into various feed and premix manufacturing processes, being inert in the interaction with vitamins and pigments, even under extreme conditions of temperature and humidity.
These attributes make Natu-B4™ a safer and more sustainable alternative in the formulation of diets and premixtures.
In poultry production, Natu-B4™ is the only one that not only replaces choline chloride and is thus free of all its weaknesses, but also has a unique effect on energy metabolism, allowing maximum use to be made of the energy compounds after absorption, thus not competing for substrates with enzymes and emulsifiers, for example.
With Natu-B4™ it is possible to decrease their formulation costs without loss of performance, which was demonstrated by Calderano et al. (2015), Alleno et al. (2015), Farina et al. (2017) and Goulart et al. (2021), among others.
Alternatively, through specific protocol, it is also possible to control the prevalence of fatty liver syndrome and decrease hepatic and abdominal fat deposition, as demonstrated by Agrawal et al. (2012) and reduce the relative and/or absolute weight of the livers (Le Roux, 2015 and Atkinson, 2016).
Liver weight at 35 days
France 2015
France 2015 (g) (%)
Liver weight (g) Relative liver weight (%)
Relative liver weight at 35 days
In pig production, one of the main challenges in much of the world is to maximize productivity, maximize yields of the main cuts and at the same time maintain low carcass fat.
For many years we have had advances in genetic improvement, nutritional programs and in the combined use of additives which for many years was the only tool capable of significantly reducing fat deposition in the final stages of pig production, however, its use has now been banned in several countries.
In this context, in studies conducted by Calderón et al. (2016), a higher daily lean tissue gain and higher energy efficiency for lean tissue gain was obtained when using Natu-B4™ compared to Choline Chloride and a negative control.
With the evolution of its development, Natu-B4™ has been shown to be a natural alternative for leaner carcasses.
Back fat Pigs Brazil 2018
16,0 15,5 15,0 14,5 14,0 13,5 13,0 millimetres
In ruminants, Natu-B4™ presents itself as a viable alternative to protected choline products, due to its resistance to degradation in the rumen.
Studies in sheep, goats and dairy cows have shown improvements in milk production, milk quality and reproductive parameters, introducing a transformative approach to dairy farming (Gutierrez et al., 2019; Roque-Jimenez et al., 2020; Alba et al., 2020 and 2021; Mendoza et al., 2021; Nunes et al., 2022; Mendoza et al., 2024).
Control Natu-B4 250g Natu-B4 500g
% Meat in carcasses Pigs Brazil 2018
57,5
57,0
56,5
56,0
55,5
54,5
54,0 %
In pets, Natu-B4™ has been shown to be an effective alternative to synthetic choline chloride, offering superior metabolic benefits. Nutrigenomics studies suggest that Natu-B4™ provides broader health benefits, from cardiovascular health to cognitive function, positioning it as a holistic solution for pet nutrition (Mallo and Paolella, 2017; Mendoza et al., 2021; Nascimento et al., 2022).
Control Natu-B4 250g Natu-B4 500g
Herbal products in general are susceptible to various forms of microbiological contamination throughout their chain and processing.
An efficient sanitization process is the only definitive alternative to guarantee low levels of microbiological load, especially those belonging to the classes of micro-organisms of major concern for animal and human health.
Gamma irradiation applied after packaging or, alternatively, organic acid treatments when irradiation is not allowed, emerge as ideal sanitization methods, balancing efficiency, absence of residues and compliance with quality standards and legal regulations.
The Nuproxa Group ensures strict quality control and is FAMI-QS certified company for its entire product line, including Natu-B4™. In addition to this, Natu-B4™ production is also ISO 9000, 14000 and 45000 certified, guaranteeing superior quality, 100% traceability and commitment to sustainability, and is also permitted for use in organic production in Europe through FiBL.
In polyherbal formulations, the supplier's expertise in raw material selection and processing is critical. Products derived from Ayurvedic medicine require precise selection of plant parts and processing to achieve the desired results. Experienced suppliers offer invaluable insights, optimizing product efficacy in diverse environments.
With over two decades of experience and a dedicated team, Nuproxa Switzerland and its business partners offer technical and after-sales support unmatched in the market. Leveraging extensive documentation and high talent retention, they stand out as pioneers in providing technical consultancy, ensuring optimal utilization of Natu-B4™.
The reliance on choline chloride for supplementation is outdated, considering the sound scientific basis of alternatives such as Natu-B4™. In addition to offering a safer and more consistent option, Natu-B4™ aligns with sustainability goals, reducing reliance on petroleum derivatives in favor of plant-based, environmentally friendly alternatives.
Among the various options available on the market, Natu-B4™ emerges as the most reliable, complete and economical solution, transcending its role as a choline substitute to become a potent metabolic modulator.
Eggshell quality is a crucial aspect of the poultry industry, particularly for layer hens that are bred for egg production. Poor eggshell quality can result in economic losses, as weaker eggs are more likely to break during handling, transportation, and storage, leading to reduced marketability and wastage.
M. Naeem
Postdoctoral Fellow Department of Poultry Science, Auburn University, Auburn, AL., 36849
Factors contributing to poor eggshell quality are multifaceted and range from environmental conditions to nutrition and genetic factors. This article delves into the primary causes of poor eggshell quality in layer hens, exploring each to offer insights into how poultry managers and farmers can mitigate these issues and improve production outcomes.
The most common cause of poor eggshell quality is nutritional imbalance, particularly deficiencies in key minerals and vitamins essential for shell formation.
Calcium is the primary mineral responsible for forming the eggshell, which is mainly composed of calcium carbonate.
A lack of adequate calcium in the diet results in thinner, weaker eggshells, as the body prioritizes calcium use for other vital functions, such as maintaining bone structure. Layer hens require around 3-4 grams of calcium per day, and failure to meet this requirement often leads to poor shell quality. Limestone or oyster shells are commonly used to supplement calcium in the diet.
During the growing period, a pullet’s calcium needs are pretty low. However, once she begins laying eggs, her calcium requirement surges to at least four times the
Interestingly, a hen’s calcium requirement fluctuates throughout the day. Eggshell formation generally occurs around midnight, so if you notice thin shells, particularly common in older hens, consider supplementing their diet with calcium-rich oyster shell. Due to its larger particle size, oyster shell is absorbed slowly, allowing calcium to be available overnight when the shell is forming.
Additionally, it’s a good practice to switch to a laying feed about 10 days before hens begin laying, rather than waiting until eggs start forming.
Vitamin D, particularly in its active form (D3), is crucial for calcium absorption and metabolism. Without sufficient vitamin D3, even with ample dietary calcium, the hen’s body cannot absorb and utilize calcium efficiently, leading to poor eggshell formation. A deficiency in vitamin D can thus indirectly cause shell quality issues, underscoring the importance of a well-balanced diet.
Phosphorus, in coordination with calcium, contributes to eggshell integrity. An imbalance between calcium and phosphorus (often in a Ca:P ratio of about 10:1) can affect shell formation. While phosphorus is essential, excessive amounts can hinder calcium absorption, further weakening the eggshell.
While a calcium-to-non-phytate phosphorus ratio of around 2:1 is ideal for most poultry diets, this ratio should rise to between 8:1 and 12:1 once the birds start laying. Accordingly, the dietary calcium percentage should increase from 2.5% to 5%.
Proteins, especially those rich in amino acids like methionine and lysine, are necessary for the synthesis of the eggshell membrane, a key component in eggshell strength. Protein deficiency can result in fragile membranes and shells, increasing the likelihood of breakage.
Zinc, manganese, and copper are trace minerals essential for shell quality. These elements are part of various enzymes responsible for eggshell formation, and deficiencies can negatively affect shell thickness and strength.
Environmental factors, particularly temperature, humidity, and light exposure, can significantly impact eggshell quality.
Heat stress in layer hens reduces their calcium absorption, leading to poor shell quality. When exposed to high temperatures (above 30°C or 86°F), hens tend to eat less, resulting in decreased nutrient intake, including calcium.
Additionally, heat stress can cause respiratory alkalosis, a condition that reduces blood calcium levels and, consequently, eggshell formation.
High humidity can exacerbate heat stress, further affecting the hen’s calcium metabolism and intake. On the other hand, very low humidity can cause rapid moisture loss from the egg, potentially leading to shell cracks and brittleness. Optimal humidity levels (about 50-60%) help maintain shell strength.
Light exposure regulates laying cycles, and inadequate lighting can lead to reduced calcium utilization, thereby impacting shell quality. Providing appropriate light intensity and duration (around 16 hours per day for layers) helps hens maintain a steady egg-laying pattern and supports optimal calcium utilization.
Genetics plays an undeniable role in eggshell quality. Some breeds of chickens are predisposed to laying eggs with stronger shells, while others may produce thinner or more brittle shells.
Certain breeds, like Leghorns, are known for laying eggs with excellent shell quality, whereas other breeds may produce weaker shells. Selecting breeds with desirable eggshell traits can help improve shell strength.
As hens age, they generally produce eggs with thinner shells. Older hens often experience a natural decrease in calcium absorption efficiency, coupled with a longer interval between eggs, which can reduce eggshell thickness.
Infectious diseases and certain health conditions can impair the ability of hens to form strong eggshells.
IBV is a viral disease that affects the respiratory and reproductive systems of poultry. Infected hens often produce eggs with malformed, weak, or thin shells. This virus damages the shell gland in the reproductive tract, impairing its function.
Newcastle disease, another viral infection, can also impact eggshell quality. It
EDS is caused by a viral infection that affects the reproductive tract, resulting in soft-shelled or shell-less eggs. The virus interferes with calcium metabolism and shell formation, compromising eggshell integrity.
Parasites and Bacterial Infections: Internal parasites like coccidia or bacterial infections can lead to poor nutrient absorption, impacting shell quality.
Additionally, bacterial infections in the reproductive tract, such as those caused by Escherichia coli, can affect the shell gland, causing irregularities in eggshell formation.
Hormones regulate many of the physiological processes involved in egg production, including eggshell formation. Imbalances in reproductive hormones can disrupt the process, resulting in weaker shells.
Estrogen is a key hormone responsible for the mobilization of calcium to form eggshells. Fluctuations in estrogen levels can alter calcium distribution and eggshell quality. Reduced estrogen can lead to inadequate calcium mobilization, weakening the shell.
The parathyroid hormone helps maintain calcium homeostasis by mobilizing calcium reserves from bones. An imbalance in this hormone can result in insufficient calcium availability for eggshell formation, leading to weaker shells.
The way hens are housed and managed also significantly influences eggshell quality. Certain toxins or contaminants, either from feed or the environment, can interfere with eggshell formation.
In systems where hens are confined to small cages for long periods, they may develop cage fatigue, a condition that affects bone density and strength. This condition leads to weak bones, resulting in insufficient calcium reserves for eggshell formation
Overcrowded environments increase stress levels in hens, impacting nutrient absorption and utilization. Stress from overcrowding can lead to hormonal imbalances and reduced calcium metabolism, weakening eggshell quality.
Hard or abrasive bedding can cause minor shell damage as the eggs are laid. Soft, appropriate bedding reduces the risk of damage to the eggshell, helping maintain its integrity during handling and transportation.
Poor water quality, especially if it is contaminated or contains excessive minerals, can interfere with calcium absorption. Maintaining clean and accessible water sources is essential for optimal shell quality.
Mycotoxins are toxins produced by fungi in moldy feed. They can disrupt hormone levels and calcium absorption in hens, leading to poor shell quality. Regular monitoring and proper storage of feed are essential to prevent mycotoxin contamination.
Exposure to heavy metals such as lead, cadmium, and mercury can interfere with calcium metabolism. These metals are toxic and disrupt normal physiological processes, including shell formation.
Some medications or chemical residues in feed or water can inadvertently affect shell quality.
While certain medications are necessary to treat infections, overuse or improper use of antibiotics can interfere with nutrient absorption and metabolism. For example, antibiotics may disrupt gut flora, leading to poor calcium absorption and weaker shells.
Residues from pesticides or herbicides in feed can harm hens and impact eggshell quality. Strict regulation and careful sourcing of feed ingredients can reduce the likelihood of such contamination.
Eggshell quality in layer hens is influenced by a complex interplay of factors, ranging from nutrition and genetics to environmental and management practices.
Addressing poor eggshell quality requires a multifaceted approach that includes providing balanced diets rich in calcium and essential vitamins, maintaining proper environmental conditions, preventing and controlling diseases, and implementing good management practices.
By understanding the underlying causes of poor eggshell quality, poultry farmers and managers can make informed decisions to enhance production efficiency, reduce losses, and improve the overall health and well-being of their flocks.
From Calcium to Care: Key Factors Influencing Eggshell Quality in Layers DOWNLOAD ON PDF
YELA PROSECURE is a specifically designed hydrolyzed yeast. Produced with a controlled hydrolysis process ensuring a consistent product composition, YELA PROSECURE offers highly digestible and functional nutrients that support animal performance, digestive care and feed palatability while contributing to the feed protein balance.
Tainara Ciuffi E. Dornelas Doctor in Animal Production Technical Coordinator of nutriNews Brasil
In the last edition of the nutriNews International magazine, we reviewed information to better understand the necessity and the reason why methionine is so important in poultry diets. Recalling key points:
It promotes the mobilization of lipids to provide energy for growth and protein deposition, regulating nutrient metabolism.
It increases antioxidant capacity and immunity.
It participates in protein synthesis.
It is also a precursor of cystine, so as long as methionine supplementation is adequate, its supplementation becomes unnecessary.
It is also used in the formation of skin and feathers, which explains the high demand for these amino acids by poultry.
Now we will discuss its role in the body’s antioxidant metabolism.
The control of stress, which may result from management, nutrition, technology, and environmental factors, as well as internal stress, is one of the inevitable challenges in poultry production.
There can be various stressors, such as:
Temperature - heat or cold
Humidity
Stocking density
Immunization
Transportation.
Most of these stressors can induce acute stress responses and further reduce production performance, health and welfare, as well as the quality and quantity of meat products in the broiler population.
Some stressors can increase their susceptibility to diseases, resulting in low daily gains and feed conversion rates. A typical source of stress is the excessive generation of free radicals, which causes oxidative stress at the molecular level.
Methionine residues can be used as endogenous antioxidants to alleviate damage caused by the excess of Reactive Oxygen Species (ROS).
Similarly, dietary methionine can upregulate the expression of heat shock protein mRNA in the intestine to protect against intestinal mucosal damage in broiler chickens caused by heat stress. Therefore, dietary methionine can help alleviate adverse reactions caused by high-temperature stress.
Methionine is a precursor of succinylCoA, homocysteine, cysteine, creatine, and carnitine. It is a precursor for cellular methylation and the synthesis of cysteine, and thus can reduce the dietary requirement for cysteine.
It can act against oxidative stress by being a precursor to antioxidants or through the incorporation of residues into proteins.
Reactive oxygen species (ROS) are produced by a variety of physiological and non-physiological events, including the Fenton reaction, cellular respiration, mitochondrial dysfunction, pathologies, phagocytes, neutrophils, and stress.
Methionine plays an essential role in the immune system through its metabolites. It directly influences the functioning of the immune system because its catabolism can lead to an increase in the production of glutathione, taurine, and other metabolites. Methionine is also easily used by hepatocytes for the direct synthesis of glutathione.
The free radical has an unpaired electron. The antioxidant is responsible for donating this electron.
The most common and studied antioxidants in different bird tissues regarding methionine supplementation include:
Total antioxidant capacity (TAC)
Superoxide dismutase (SOD)
Catalase (CAT)
Glutathione peroxidase (GPX)
Glutathione (GSH).
Among the enzymatic substances, the superoxide dismutase (SOD) is the enzyme responsible for catalyzing the dismutation of the superoxide anion (O2-) into less reactive species such as hydrogen peroxide (H2O2). The formation of the superoxide anion involves oxygen with two unpaired electrons that bind to another electronsharing molecule:
Dismutation is the process by which this oxide radical, upon receiving hydrogen ions, results in the compound hydrogen peroxide, and this compound will be reduced by the enzymes catalase (CAT) and glutathione peroxidase (GPx):
This way, these enzymes act as the first line of antioxidant defense in the body, assisting in the control of free radical production. The final result of the action of these enzymes will be harmless molecules such as oxygen and water.
Methionine protects against oxidative stress in two ways: first, by indirectly producing antioxidants (precursors to cysteine and glutathione), and second, through Met residues in proteins (Figure 2). The latter pathway occurs during repair, where various ROS products attack Met residues in proteins to form methionine sulfoxide, leading to the elimination of reactive species by cells.
Methionine sulfoxide reductases (MSRs) are enzymes that reduce reactive oxygen species (ROS) in all organisms, from bacteria to mammals. This is achieved by the oxidation of eight Met residues per protein molecule, maintaining the enzymatic integrity of the individual protein.
Thiodoxin (TRx) prepares the enzyme for another catalytic cycle by reducing the oxidized MSR. NADPH is also a scavenger of reactive nitrogen and oxygen species.
The antioxidant potential through this pathway is influenced by thermal stress and methionine supplementation, where the TRxR1 and MsrA genes were highly expressed in broiler chickens under thermal stress and supplemented with DL-HMTBA.
Methionine remains an essential nutrient in the bodies of birds, and no matter how many studies are conducted, there will always be a new and relevant role for it to be elucidated.
Figure 2. Overview of the effects of stressors, the function of the antioxidant system, and the influences of Met on the antioxidant system/status of birds. Adapted from Lugata, 2022.
in Poultry Diets and Its Role in Antioxidant Prevention in the Body - Part II DOWNLOAD PDF Agentes
Gwendolyn Jones, PhD in Animal Nutrition at the University of Aberdeen in Scotland, Freelance writer
Many layer flocks are operating below their performance potential, because producers are unable to control stressors impacting the potential length of productivity in birds.
This calls for solutions that can help control the response of the bird to a variety of stressors in such a way that high productivity, egg quality and wellbeing can be maintained for longer.
Research from the University of Athens is helping to increase the understanding of cellular adaptive pathways in the gut and ovary and how they can be regulated by nutritional means with positive effects on layer performance.
The reason why many layer units perform below their potential in terms of productivity and productive life span in hens is often the fact that under commercial conditions the birds are exposed to a variety of stressors that are difficult to control by producers.
These stressors include: changes in diet, a rise in ambient temperatures, xenobiotics (including mycotoxins) in the feed and the stress of high productivity.
All of these factors have implications for long-term performance and well-being, as well as the quality of the eggs produced.
Oxidative stress is a well-known factor that affects the long-term health and productivity of key organs for egg production in the laying hen, i.e. the ovary, gut and liver. It is the result of an increased accumulation of reactive oxygen species (ROS) in response to stressors that the body’s own defence mechanisms are no longer able to counterbalance.
Maintaining high levels of production of good quality eggs in an extended production cycle requires hens to develop and maintain healthy and functional ovaries. It is well known that the decline in ovarian functions over the egg production cycle is linked to ovarian ageing.
This means there is a performance gap that can only be closed if there are ways to support the bird to respond to stressors in more efficient ways or if the bird itself becomes more resilient through genetic selection.
In a commercial environment that is increasingly relying on reducing the cost and environmental footprint of production, laying hens that maintain productivity for longer represent a competitive advantage for the profitability and sustainability of egg production.
Oxidative stress is one of the main causes of ovarian ageing, which is linked to a decline in ovarian function and affects egg production and quality as laying hens get older.
Highly productive laying hens are more vulnerable to oxidative stress and ovarian ageing due to rapid daily ovulations. Loss of tissue and organ function is frequently caused by an excessive buildup of reactive oxygen species.
Growing evidence highlights the crucial balance between ROS and antioxidant levels within the ovary, which is essential for preserving female reproductive health.
Excessive ROS accumulation causes oxidative damage, resulting in damage to oocytes in the ovaries, a pivotal contributor to fertility decline.
A better understanding of the mechanisms underlying ovarian ageing and possibilities to mitigate oxidative stress is helping to find ways to prolong ovarian lifespans and thus potentially increase the length of productivity for egg production in laying hens.
ROS accumulate during metabolic activity, which can be exacerbated in periods of high productivity and when the bird is challenged by stressors in its environment or feed. For example, the exposure to heat stress is known to increase the production of ROS at the cellular level and so does the exposure to mycotoxins in poultry feed.
Hence a lack of support to increase the birds’ antioxidative defence against ROS particularly during more challenging periods of the production cycle can lead to an increased rate of decline of the ovaries.
This limits the ability to extend laying cycles, reduces egg production and decreases the commercial value of laying hens.
The underlying mechanisms for the decline in the antioxidative capacity of ovaries of laying hens during the ageing process have been linked to the down-regulation of the nuclear factor erythroid 2-related factor 2 (Nrf2) gene expression in the Nrf2-KEAP1 pathway at the cellular level.
This signalling pathway is involved in the mobilisation of cellular antioxidant defences to alleviate oxidative stress in tissues. Nrf2 upregulates antioxidant response elements (AREs)-mediated expression of antioxidant enzyme and cytoprotective proteins. Classical antioxidant enzymes inactivate ROS and prevent ROS-initiated reactions.
These antioxidant enzymes include superoxide dismutases (SODs) 1, 2, and 3, catalase, glutathione peroxidase (GPx), and GSH reductase.
Going one step beyond in animal nutrition means ceaseless research and development. Going one step beyond means anticipating developments in the livestock industry and offering the highest quality products and services. Going one step beyond means total commitment to overcoming all our customers’ challenges.
Egg quality parameters such as albumen height, Haugh Unit (HU), eggshell thickness and eggshell strength have been shown to be depressed when the Nrf2-Keap-1 pathway was impaired.
A decrease in the expression of Nrf2-related genes in response to stress factors such as heat stress and mycotoxins has also been associated with a reduction in productivity and intestinal health in chickens.
Peer-reviewed laying hen nutrition research carried out at the University of Athens suggests that the response of important cellular adaptive pathways in the gut and ovary can be modulated in favour of positive outcomes for productivity.
In a 12-week study, Hy-Line brown laying hens were given a gut agility activator in their feed to assess its effects. This gut agility activator, a mix of plant compounds, was tested at various doses and compared to a standard diet.
It was found to enhance egg-laying rates and positively influence gene expression related to detoxification and antioxidant defence in the hens’ gut and ovaries, suggesting a reduced need for detoxification and a boost in antioxidant capacity.
Critical genes for cell protection against oxidation from the Nrf2 pathway were upregulated at week 12 of the experiment in the gut and ovary.
The activation of the Nrf2 pathway not only significantly upregulated superoxide dismutase (SOD1) but also gene expression of other important antioxidant enzymes such as GPX2, GSR and PRDX1 in both the gut and ovaries of the laying hens.
The researchers interpreted the results at the molecular level as an increased antioxidant capacity in the bird in response to the treated diet.
Advances in the understanding of cellular adaptive pathways in the laying hen in combination with nutritional strategies to support longlife ovarian function can enhance the control egg producers have over sustaining longer laying cycles in their flocks. In addition, it is paving the way for increased capabilities to further improve the economics and sustainability of egg production.
INTRODUCTION
CELMANAX™ is a multicomponent, all-natural feed supplement containing Refined Functional Carbohydrates™ (RFC™) that has Generally Recognized as Safe (GRAS) status as a feed ingredient.
STUDY OVERVIEW
Two independent studies1,2 were done to evaluate the effect of CELMANAX supplementation in broiler breeder and broiler diets on Salmonella prevalence.
Study 11
A total of 1,040 one-day-old broiler breeders were reared in 16 pens (60 – 65 females per pen and 8 – 18 males per pen; 8 pens per treatment)
Broiler breeders were fed 0 or 50 g/MT of CELMANAX SCP
Eggs from 51-week-old breeder hens were collected and hatched, and male progeny broiler chicks were fed diets supplemented with 0 or 50 g/MT of CELMANAX SCP
All broilers (n=192) were tested for cecal Salmonella spp. at 34 days of age
Ceca were collected from breeder hens (n=28 – 30) from each treatment group at 23 and 64 weeks of age and tested for Salmonella prevalence
Breeder performance was measured
Study 22
One-day-old Ross Broiler chicks sorted by sex were allotted to 24 replicate pens of 12 broilers each per diet per sex and fed either 0 or 50 g/MT of CELMANAX SCP
Broilers were fed a standard starter, grower and finisher diet
Broiler pens were tested for Salmonella prevalence in the litter
Salmonella prevalence was also tested in broiler ceca
Broiler performance was measured
1 Walker et al. Poult Sci 2017;96(8):2684-2690.
2 Walker et al. Poultry Science 2018;97:1412–1419.
In study 1, control broiler breeder hens had 71.43% and 40.00% prevalence of Salmonella in the ceca, while hens fed CELMANAX had zero prevalence of Salmonella (P<0.01) (Figura 1).
Breeders at 23 weeks
Breeders at 64 weeks
1. Treatment effects on % prevalence of Salmonella in breeder hen ceca in Study 1.
In study 1, broiler progeny from hens fed the control diet and receiving control broiler diets had 12.5% prevalence of Salmonella in the ceca whereas broilers from CELMANAX-fed hens and receiving 0 or 50 g/MT of CELMANAX in the broiler diets had zero prevalence of Salmonella at 34-day sampling (Figure 2).
breeders
Figure 2. Breeder and broiler treatment effects on % prevalence of Salmonella in broiler ceca in Study 1.
In study 2, Salmonella spp. was isolated from litter from 7 of 48 (14.58%) control-fed broiler pens, but none (0%) were isolated from CELMANAX-fed pens (P≤0.05) (Figure 3).
Figure 3. Broiler treatment effects on % incidence of Salmonella presence in litter in Study 2
Broiler cecal sampling for Salmonella at 44 days of age and 55 days of age confirmed 45.83% and 29.17% prevalence respectively in control-fed broilers, but none from pens fed CELMANAX (Figure 4) CELMANAX-fed female broilers had a tendency for improved body weight and feed-conversion ratio (data not presented).
Figure 4. Treatment effects on % prevalence of Salmonella in ceca of broilers in Study 2
CELMANAX supplementation in broiler breeder and broiler diets significantly reduced prevalence of Salmonella in the litter and ceca, thus supporting its benefit in a multifactorial Salmonella mitigation strategy in poultry production.
CELMANAX supplementation in broiler breeder and broiler diets reduced prevalence of Salmonella
Maykelly da Silva Gomes1, Gabriel Cipriano Rocha1, Danyel Bueno Dalto2, Pedro Silva Careli1, Jansller Luiz Genova1
1Muscle Biology and Nutrigenomics Laboratory, Department of Animal Science, Universidade Federal de Viçosa, Viçosa, Minas Gerais, 36570-900, Brazil 2Sherbrooke Research and Development Centre, Agriculture and Agri-Food Canada, Sherbrooke, Quebec, J1M 0C8, Canada
Pig production frequently face significant challenges (e.g. infectious diseases, environmental stressors, and management practices) that negatively affect pigs health and productivity, causing exacerbated immune reactivations and oxidative stress (Le Floc’h et al., 2021).
Oxidative stress caused by zearalenone-contaminated feed (Su et al., 2018), lipid peroxidation (Silva-Guillen et al., 2020), heat stress (Feng et al., 2017), rotavirus (Tian et al., 2016), and porcine epidemic diarrhea virus (Yang et al., 2019)
can be used as challenge models that simulate stressors at the farm level. These challenge models aim to understand their effects on the physiological and immunological responses of pigs, and help to develop mitigation strategies such as vitamin supplementation in diets.
Vitamins D, E, and C play vital roles in supporting pig health through their contributions to immune response, antioxidant defense, and metabolic processes (Hao et al., 2021; Mainardi et al., 2024)
Although isolated studies have addressed the effects of these vitamins under normal and challenging conditions, there are still significant gaps regarding their specific requirements and mechanisms of action.
Maintaining optimal dietary levels of these vitamins is essential, particularly when pigs face various stressors that require additional nutritional support. Research shows that increasing dietary levels of these vitamins beyond current recommendations can boost immune responses and reduce oxidative stress, enhancing intestinal health and mitigating adverse effects of stressors (Liu et al., 2016; Feng et al., 2017).
Thus, this review aimed to summarize information on the roles of vitamins D, E, and C in immune regulation and antioxidant capacity, emphasizing their importance in supporting the intestinal health of pigs in challenging conditions (e.g. infections, oxidative stress, and heat stress)
Vitamin D regulates mineral homeostasis (Ca, P, Mg) and bone function, and influences innate and adaptive immune responses (Wu et al., 2019; Calder et al., 2020), for example:
Supports intestinal barrier function by upregulating tight and adherens junctions proteins, increasing transepithelial resistance in intestinal epithelial cells, thus enhancing barrier integrity (Yang and Ma, 2021)
It stimulates antimicrobial peptide production, enhancing immune defense (Wang et al., 2004)
It promotes monocyte proliferation and differentiation into macrophages, enhancing chemotactic and phagocytic functions (Wu et al., 2019)
It reduces pro-inflammatory cytokine expression by inhibiting p38 activation (Zhang et al., 2012)
It inhibits dendritic cell differentiation and maturation (Gombart et al., 2020).
Influences adaptive immunity by inhibiting T and B cell proliferation and modulating CD4+ T cell differentiation, promoting anti-inflammatory Treg cells and suppressing pro-inflammatory Th1 and Th17 cells (Wu et al., 2019; Pecora et al., 2020).
Studies (see Table 1) using porcine rotavirus models have shown that dietary vitamin D3 (5000 IU/kg) reduces the diarrhea prevalence and maintains weight gain and feed intake in infected pigs (Zhao et al., 2014; Tian et al., 2016). Liao et al. (2011) showed that supplementing weaned pigs with 2200 IU of 25-OH-D3/kg increased antiinflammatory and reduced proinflammatory cytokines, while lowering serum IgA and IgM concentrations in pigs challenged with rotavirus.
It regulates endothelial function and enhances antioxidant by increasing superoxide dismutase activity (Kim et al., 2020). Dietary supplementation with 2000 IU of 25-hydroxyvitamin D3/kg improved antioxidant capacity in weaned piglets (Zhou et al., 2022).
Table 1. Summary of studies about the use of vitamin D (Vit D) in pig diets under challenging conditions.
Vit
25(OH)D3
Vit D3
25(OH)D3
Weaning piglets (7.4 kg) 220 IU/kg 2200 IU/kg
Growing pigs (21.8 ± 2.1 kg) 200 IU/kg 5000 IU/kg = 125 mg of cholecalciferol
Growing pigs (26.2 ± 2.02 kg)
25(OH)D3
200 IU/kg
5000 IU/kg
Weaning piglets (6.6 ± 0.4 kg) 5.5 μg/kg = 220 IU/kg 155.5 μg/kg = 6220 IU/kg
Vitamin E, encompassing tocopherols and tocotrienols, plays essential roles in immunity and antioxidant defense (Wu et al., 2019), as shown in Table 2.
It enhances T cell activation and promotes immune synapse formation between antigenpresenting cells and CD4+ T cells (Lewis et al., 2019).
It reduces prostaglandin E2 production, probably by inhibiting cyclooxygenase activity, thereby supporting T-cell proliferation (Wu et al., 2019).
As a lipid-soluble antioxidant, vitamin E scavenges reactive oxygen species (ROS), protects cell membranes, and preserves immune cell function (Calder, 2013).
It prevents lipid peroxidation in membranes and participates in “vitamin E recycling”, improving antioxidant defenses (Traber and Stevens, 2011).
Rotavirus
: IgG, IgM, IgA, IL-2, IL-6 serum concentrations; IFN-γ intestinal contents concentrations. : I L-4 serum and intestinal contents concentrations.
: diarrhea incidence. : RIG-I, IPS-1, IFN-β, ISG15 mRNA in the intestine; ADG and ADFI.
: Beclin1 and PR-39 mRNA in the RVinfected IPEC-J2 cells, PMAP23, PG1-5, PR-39 mRNA in the RV-infected cells.
Liao et al. (2011)
Porcine epidemic diarrhea virus
: diarrhea scores; SI, PRR, IFN-λ, STAT1, MxA, IL6, IL8 mRNA in the jejunal mucosa.
: claudin2 mRNA in the jejunal mucosa.
Zhao et al. (2014)
Tian et al. (2016)
Yang et al. (2019)
BW: Body weight; IgG, IgM, IgA: Immunoglobulin G, M, A; IL-2, IL-6, IL-4, IL-8: Interleukin 2, 6, 4, 8; IFN: Interferon; RIG-I: Retinoic acid-inducible gene I; IPS-1: interferon-b promoter stimulator 1; ISG15: Interferon-stimulated gene 15; ADG: Average daily gain; ADFI: Average daily feed intake; Beclin 1: protein involved in the regulation of autophagy; PR-39: proline-arginine rich 39-amino-acid peptide (an antimicrobial peptide); PMAP23: Porcine myeloid antimicrobial peptide 23; PG1-5: cysteine-rich PG1 (PG-1) to PG-5; RV: Rotavirus; SI: Sucrase-isomaltase; PRR: Pattern recognition receptors; STAT1: signal transducers and activators of transcription; MxA: Myxovirus resistance A.
2. Summary of studies about the use of vitamin E (Vit E) in pig diets under challenging conditions
DL-αtocopherylacetate
Vit E
DL-αtocopherylacetate
α-tocopheryl acetate
α-tocopherol + Se yeast
Weaned piglets (6.6 ± 1.0 kg) 33 IU/kg 200 IU/kg
Sows (day 107 of gestation until weaning) 44 IU/kg 250 IU/kg
Oxidative stress (lipid peroxidation) : G:F. : TAC (serum).
: ADG and weaning weight of piglets; IgG, IgA in plasma of sows and piglets, colostrum and milk; TAC, CAT in piglets’ plasma.
Silva-Guillen et al. (2020)
Sows (day 80 of gestation) 50 IU/kg 200 IU/kg : GPx in serum of sow. Wang et al. (2019)
Sows (from day 85 of gestation until weaning) 30 IU/kg 100 IU/kg -tocopherylacetate
Growing pigs (20,0 ± 3,0 kg)
Oxidative stress (gestation, farrowing, and lactation)
0.2 mg Se and 17 IU/ kg Vit E 1.0 p.p.m. Se + 200 IU/kg Vit E Heat stress
: Plasma MDA of the sow. : Birth weight, measures related to the head and trunk, ADG until weaning.
: Bicarbonate (blood), TER, intestinal GPx-2 mRNA. : intestinal GSSG:GSH ratio, intestinal FD4 permeability.
Liu et al. (2016)
BW: Body weight; G:F: Gain-to-feed ratio; ADG: Average daily gain; IgG, IgA: Immunoglobulin G, A; IL-10: Interleukin 10; TAC: Total antioxidant capacity; CAT: Catalase; GPx: Glutathione peroxidase; TER: Transepithelial resistance; MDA: Malondialdehyde; GSSG: Oxidized glutathione; GSH: Glutathione; FD4: fluorescein isothiocyanate–dextran.
The addition of peroxidized oil to pigs’ diets induces oxidative stress, negatively affecting growth performance. Vitamin E supplementation (200 IU of DL-αtocopheryl acetate/kg diet) improved total antioxidant capacity (TAC) in weaned pigs, but did not enhance TAC in those fed peroxidized oil, indicating effective antioxidant activity against oxidation (SilvaGuillen et al., 2020).
Oxidative stress can impair sow performance and piglet development (Gómez et al., 2023). Wang et al. (2017) found that 250 IU of vitamin E/kg from day 107 of gestation improved the piglets’ average daily gain, weaning weight, immune function, and antioxidant status.
Gómez et al. (2023) reported that 100 mg of vitamin E/kg from day 85 of gestation enhanced birth weight and early growth, highlighting the importance of maternal oxidative status in supporting piglet development.
Vitamin C supports skin integrity by stabilizing collagen, enhancing collagen gene expression, and aiding tissue remodeling (Carr and Maggini, 2017; Pecora et al., 2020). This is relevant for group-housed sows, where restricted feeding can lead to aggressive behaviors and increased skin and hoof lesions (Bench et al., 2013).
As a cofactor for hydroxylases, vitamin C limits neutrophil survival and neutrophil extracellular trap formation, reducing tissue damage (Carr and Maggini, 2017).
It increases T and B cell differentiation, proliferation, and antibody production, while regulating T-cell maturation (Pecora et al., 2020).
It boosts NK cell cytotoxicity and acts as a potent immunostimulant (Pecora et al., 2020).
by scavenging ROS, protecting biomolecules from oxidative damage (Hao et al., 2021).
Vitamin C supplementation (150 mg/kg) mitigates zearalenone-induced oxidative stress, restoring serum concentrations of estradiol, progesterone, luteinizing hormone, and follicle-stimulating hormone in weaning piglets, and reducing vulva size (Shi et al., 2017; Su et al., 2018).
C (1.0 g/day) increased interferon-γ synthesis, suppressing IL-4 and serum IgE (Sun et al., 2009). Additionally (see Table 3), vitamin C supplementation (500 mg of vitamin C/kg + 500 mg of N-carbamylglutamate/kg) in sows diet improved piglet birth and weaning weights and reduced oxidative stress markers during heat stress (Feng et al., 2017).
Table 3. Summary of studies about the application of vitamin C (Vit C) in pig diets under challenging conditions. Vit
Weaned piglets
Vit C
Vit C
Vit C
(4.3 ± 0.3 kg) - 1.0 g/day
Oxidative stress (soybean glycinin-induced hypersensitivity)
Vit C
Weaned piglets (12.3 ± 0.3 kg) - 150 mg/kg
Weaned piglets (12.27 ± 0.30 kg)- 150 mg/kg
Sows (from day 85 of gestation to weaning) -
500 mg of Vit C/ kg + 500 mg of N-carbamylglutamate/ kg
Oxidative stress (zearalenonecontaminated feed)
Heat stress
: F:G, diarrhea occurrence, IL-4 in the cultured splenocyte supernatant, serum IgE.
: IFN-γ in the cultured splenocyte supernatant; Histamine content in the small intestine.
: PXR, CAR, CYP1A1, CYP1A2, CYP1A6 UGT1A1, UGT1A3, UGT1A6 mRNA in liver; GPx, TAC, SOD levels in liver. : MDA (liver).
: BUN, CRE, AST, TBIL, IgA, IgG, IgM (serum).
: TG, HDL, GLB, E2, LH, FSH, PROG (serum).
: Cortisol, MDAin the maternal circulation; respiration rate.
: IgG in maternal circulation, FI; litter weights, liveweight of piglets at birth, liveweights of piglets at weaning.
et al. (2009)
Shi et al. (2017)
Su et al. (2018)
Feng et al. (2017)
BW: Body weight; F:G: Feed-to-gain ratio; FI: Feed intake; IgG, IgM, IgA, IgE: Immunoglobulin G, M, A, E; IL-4: Interleukin 4; IFN: Interferon; PXR: Progesterone receptor; CAR: Constitutive androstane receptor; CYP: Cytochrome P450, phase I metabolic enzyme genes (1A1, 1A2, 1A6); UGT: Uridine diphosphate glucuronosyltransferase, phase II metabolic enzyme genes (1A1; 1A3; 1A6); GPx: Glutathione peroxidase; TAC: Total antioxidant capacity; SOD: Superoxide dismutase; MDA: Malondialdehyde; BUN: Blood urea nitrogen; CRE: Creatinine; AST: Aspartate aminotransferase; TBIL: Total bilirubin; TG: Triglycerides; HDL: High-density lipoprotein; GLB: Globulin; E2: Estradiol; LH: Luteinizing hormone; FSH: Follicle stimulating hormone; PROG: Progesterone
The recommended and practical levels of these vitamins for pigs and sows are shown in Tables 4 and 5, respectively. NRC (2012) recommends 150–220 IU of vitamin D3/kg for pigs and 800 IU/kg for gestating and lactating sows.
However, commercial diets often exceed these recommendations by over ten times (Dalto and Silva, 2020; Yang et al., 2021; Faccin et al., 2023). The Brazilian Tables (Rostagno et al., 2024) provide recommendations of 1108 to 2468 IU/kg for pigs, and 1660 IU/kg for gestating and lactating sows.
Nursery diets (± 5.5 - 30 kg | ± 21 - 70 days of age)
Studies with nursery and grower pigs (Liao et al., 2011; Zhao et al., 2014; Tian et al., 2016; Yang et al., 2019) following NRC’s 220 IU/kg baseline showed that higher vitamin D3 levels (2200–6220 IU/kg) in immunologically challenged pigs improved performance, fecal scores, immune status, and antioxidant responses.
Table 4. Recommended and practical levels of vitamins (D, E, and C) in nursery, growing, and finishing diets for pigs.
Recommendations (health status) 2468
Practical industry levels
Recommendations (health status)
Growing diets (± 30 - 70 kg | ± 71 - 110 days of age)
Practical industry levels
- Rostagno et al. (2017)
(2012)
- Flohr et al. (2016) – US
Dalto and Silva (2020) – Brazil 2697
- Yang et al. (2021) – China
57.4 - Faccin et al. (2023) – US
1603 42.8 - Rostagno et al. (2024) 150 11 - NRC (2012) 1850 50 - Genetic A
40.6 - Genetic B 1750 70 Genetic C
- Flohr et al. (2016) – US
Dalto and Silva (2020) – Brazil
- Yang et al. (2021) – China
27.6 - Faccin et al. (2023) – US
Recommendations (health status) 1108 29.6 - Rostagno et al. (2024)
(2012)
Finishing diets (± 70 - 125 kg | ± 111175 days of age)
Practical industry levels
21.7 - Flohr et al. (2016) – US
26.9 122.9 Dalto and Silva (2020) - Brazil 2032 23.5 - Yang et al. (2021) – China
969 23.8 - Faccin et al. (2023) – US
Rostagno et al. (2024)
NRC (2012)
Gestation diets
Recommendations (health status)
Practical industry levels
44
Recommendations (health status)
Lactation diets
Practical industry levels
Rostagno et al. (2024)
NRC (2012)
Flohr et al. (2016) – US
Dalto and Silva (2020) – Brazil
Yang et al. (2021) – China
Faccin et al. (2023) – US
Table 5. Recommended and practical levels of vitamins (D, E, and C) in gestation, and lactation diets for sows.
NRC (2012) recommends 11–14.3 IU of vitamin E/ kg for pigs and 44 IU/kg for gestating and lactating sows. In contrast, Rostagno et al. (2024) suggest such levels: 65.9 IU/kg for nursery pigs,
IU/kg for growing pigs,
IU/kg for finishing pigs, and
for sows during gestation and lactation.
However, the beneficial effects of dietary supplementation with vitamin E at 200 IU/kg, have been evidenced in the immune response, intestinal health, and antioxidant potential of weaned and growing pigs challenged with heat stress and oxidative stress (Liu et al., 2016; Silva-Guillen et al., 2020).
Additionally, 200–250 IU of vitamin E/ kg during late gestation and lactation improves the sows’ health and the performance and immune status of their offspring (Wang et al., 2017; Wang et al., 2019).
NRC (2012) and Rostagno et al. (2024) do not estimate and there is no recommendation for vitamin C requirements for pigs.
However, research has shown that supplementing vitamin C to the diets of newborn (75 mg/kg) and weaning piglets (150 mg/kg) under stress (e.g. LPS or zearalenonecontaminated feed) positively affects various aspects of the immune system (Shi et al., 2017; Su et al., 2018).
Additionally, supplementation of 500 mg of vitamin C/kg of diet during gestation and lactation of sows under heat stress improved reproductive performance (Feng et al., 2017).
Vitamins D, E and C supplementation in pig diets above current recommendations has demonstrated the capacity to enhance immune responses and alleviate oxidative stress damage, improving overall health and performance.
Further investigation into the supplementation of these vitamins at supranutritional levels under specific challenging conditions reflecting commercial production systems may provide valuable insights for the formulation of more effective and precise diets for pigs.
Breno Luis Nery Garcia
Adequate nutrition is a crucial factor for the productive performance, health, and well-being of dairy cattle (NRC, 2001). Meeting the nutritional demands of lactating cows is fundamental and simultaneously challenging for dairy herds, especially high-production cows. In this regard, providing highly digestible feed is essential, and for this reason, the use of processed grains is an alternative (Faria júnior et al., 2009).
Grain processing refers to preparation methods aimed at achieving physical and/ or chemical changes in the grain structure, improving digestibility, palatability, and/ or inactivating antinutritional factors in feed (Faria júnior et al., 2009)) Grain processing for feeding dairy cattle aims to increase the availability and utilization of grains in the gastrointestinal tract, improving digestibility (Mathison, 1996), and can affect the site of digestion (Huntington, 1997).
During processing, the protein matrix of the endosperm is broken, allowing easy access to starch granules by microbial and/or intestinal enzymes. Moreover, starch gelatinization occurs during processing, making it more available to rumen microorganisms (Hale, 1973).
Effects on the digestion site are observed due to processing, with more processed and smaller particle grains tending to show higher ruminal starch digestibility compared to the small intestine (Rémond et al., 2004).
Various physical and chemical processing methods can be employed to increase grain digestibility in ruminants. Among the available methods, the main types of processing can be classified into:
dry processes and wet processes, involving highpressure steam.
Dry methods include grinding and rolling, while wet methods include steam rolling, flaking, and extrusion (Mourão et al., 2012).
This work aims to present, characterize, and summarize the main processing methods used in grains for feeding dairy cattle.
Grinding involves reducing the size of grain particles through mechanical impact, cutting, or friction (Lage et al., 2017; Mourão et al., 2012). Hammer mills are commonly used for grinding, where the grain is reduced in size through mechanical impact. The particles formed, beyond a certain size, can pass through a sieve, which can vary in calibration, resulting in a fine or coarse appearance depending on desired specifications (Silva, 2011).
Expected changes in the physical structure and, consequently, the availability of grains results from the grinding process, causing significant changes in starch and protein digestibility (Silva, 2011).
In addition to reducing particle size, grinding alters the internal structure of grains, eliminating the pericarp and breaking the protein matrix of the endosperm (Hale, 1973).
Grinding is the simplest and most costeffective method of grain processing, and it is also the most widely used. The main factors that can influence the grinding process are:
sieve size; mill capacity and speed; type of grain used, and grain moisture content (Silva, 2011).
The reduction of the physical barrier (endosperm) and the breaking of the endosperm increase the surface area of the grains, facilitating and intensifying the action of ruminal microorganisms and digestive enzymes, enhancing ruminal starch digestibility (Faria Júnior et al., 2009; Lage et al., 2017).
The cost of acquiring a hammer mill is around US$15,000.00, with a production capacity of 15 tons/hour (MFRural; internet).
The dry rolling process involves compressing the grain between a series of metal rollers (steel cylinders) that flatten and break the grain into smaller particles. The particle size generated after the dry rolling process varies depending on the spacing and pressure adjustment between the cylinders. Other factors influencing particle size include the grain flow rate in the equipment and the moisture content of the grain to be rolled (Silva, 2011).
Similar to grinding, the modifications undergone by the grain are only in the physical structure, and more gently. Dry rolling can increase ruminal digestibility compared to whole grain but has lower ruminal digestibility compared to grinding due to reduced exposure surface to enzymes (Huntington, 1997).
In addition to changes in the physical structure of grains, chemical alterations result from the treatment of grains with steam pressure. Grains undergo partial gelatinization of starch granules in the steam rolling process. Gelatinization involves breaking the hydrogen bonds that hold glucose polymers together, allowing water absorption by starch granules, facilitating enzymatic digestion (Plasencia; Zinn, 1996). 2 3
To carry out the steam rolling process, before grains pass through the roller, a preliminary stage is performed to increase grain moisture to concentrations between 17 and 20%. In a condenser, grains receive steam at 90 – 95°C for 15 to 20 minutes and are then subjected to rolling by a process similar to dry rolling (Lage et al., 2017). After the rolling process, partially gelatinized grains are dried (Mourão et al., 2012).
Additionally, since it does not undergo gelatinization, starch from dry-rolled grains may have lower availability to ruminal microorganisms than in other processing methods (Faria Júnior et al., 2009). In this sense, the use of dry-rolled grains increases the amount of starch reaching the small intestine compared to grinding. The acquisition cost of a dry roller is around US$5,000.00, with a production capacity of 2400 to 3400 kg/hour (MFRural; internet).
Flaking presents itself as a modification of the steam rolling process. Similar to steam rolling, before undergoing compression, the grain is subjected to steam application to increase internal moisture.
In the flaking process, the grain is held in a condenser at a temperature of 90 to 105°C for 30 to 60 minutes, raising the moisture to 20 to 24% (Faria Júnior et al., 2009).
After the condenser, grains undergo compression by rolling cylinders and then pass through a second set of calibrated cylinders with smaller spacing that compress the grain more intensely. Grains after the second set of cylinders have a thickness between 0.9 to 1.1 mm (Faria Júnior et al., 2009; Lage et al., 2017).
Water vapor increases the temperature of the grains to approximately 100°C, causing hydration and breaking the molecular complex of starch granules, leading to gelatinization (Silva, 2011).
Due to greater gelatinization and increased availability of starch granules, higher ruminal degradation of grains processed by flaking compared to dry and steam rolling is observed.
Additionally, flaking can provide greater palatability to the grain and consequently increase dry matter intake (Plasencia; Zinn, 1996; Silva, 2011). The flaking process increases starch digestibility by 10 to 20%, but it causes a decrease in NDF digestibility, possibly associated with increased facilitated starch fermentation (Plasencia; Zinn, 1996)
The equipment for grain flaking costs an average of US$40,000.00 and can produce 30 tons/hour (Abramilho contact).
Extrusion is a thermomechanical and continuous processing similar to steam rolling but performed with pre-ground grains. The feed is converted into a fluid mass through the combination of moisture, heat, and compression applied to the ground grains in an endless screw (Mourão et al., 2012). The mass formed in the process is forced to pass through holes where the feed expands in the direction and is expelled (Faria Júnior et al., 2009)
The expansion undergone by the grains leads to gelatinization, rupture of starch granules, and/or denaturation of protein (Lage et al., 2017). Furthermore, the extrusion process reduces the specific density of particles (e.g., dry-rolled sorghum: 450-644g/L, wet-rolled: 438-540g/L, and flaked: 360-438g/L), influencing rumen passage rate and consequently digestibility al., 2009)
Different grain processing methods for cattle feeding aim to promote physical and/or chemical changes in the grain structure that can influence the digestion site (rumen/small intestine) and digestibility, especially starch (Mourão et al., 2012).
In a systematic review conducted by Huntington (1997), the influence of processing methods on different grains was observed in the percentage of ruminal starch degradation and changes in the
The acquisition cost of an extruder is around US$45,000.00, with a production capacity of 400kg/hour (MFRural; internet).
Table 1. Digestibility Coefficient in the Rumen, Post-rumen, and Total Gastrointestinal Tract (GIT) of Grains subjected to Different Processing Methods.
Adapted from (Huntington, 1997)
The effect of corn processing and particle size on starch digestibility and digestion site was evaluated in two experiments conducted by Rémond et al. (2004).
In the first experiment, using semi-flint corn processed by:
grinding; medium rolling, and coarse rolling with particle sizes ranging from 730 to 3668 μm mm.
Ruminal starch digestibility was linearly related to particle size, being higher in grinding (59%) and decreasing linearly between processing methods based on particle size up to coarse rolling (36%). Concurrently, small intestine digestibility decreased linearly with particle size increase, and despite higher ileal starch flow, there was no increase in large intestine starch digestion based on particle size.
In the second study, ground and coarsely rolled dent corn (568 and 3458 μm) were used. Similar to the first study, ruminal starch digestibility was influenced by particle size, being higher for ground corn (70%) and lower for rolled corn (54%). Small intestine starch digestibility was not affected by particle size, but the amount of starch digested in the small intestine tended to be higher for rolled corn than ground corn.
In both studies, small intestine digestibility decreased linearly with ruminal starch escape, associated with digesta transit time.
In addition to increasing ruminal starch digestibility, grain processing can enhance propionic acid production and influence microbial protein synthesis in the rumen, as well as the flow of metabolizable protein to the small intestine (Mourão et al., 2012; Martins et al., 2019).
To assess the influence of different starch sources and processing forms on productive performance and nitrogen metabolism in lactating cows, (Vaz Pires et al., 2008) conducted a Latin square study with five cows canulated in the rumen and duodenum. Starch sources used were:
coarsely ground corn; finely ground corn; corn flocculated at 310 g/L; corn flocculated at 360 g/L, and cassava shavings.
Cassava shavings showed higher in vitro hydrolysis rate (p<0.05) than other starch sources tested, and both flocculated corn treatments had higher in vitro starch hydrolysis compared to coarsely ground corn.
In vivo evaluations showed more active ruminal fermentation (indicated by lower rumen ammonia concentration) for flocculated starch sources and cassava shavings.
There was no effect of starch sources on nitrogen consumption or the flow of nitrogen fractions to the duodenum, but there was a tendency (p<0.10) for higher microbial nitrogen flow to the duodenum in flocculated corn and finely ground corn diets compared to cassava shavings and coarsely ground corn (Table 2).
The data indicates that increased ruminal starch degradability, provided by grain processing, improved protein metabolism, allowing better synchronization in ruminal fermentation of energy and protein sources. This effect was also observed in plasma urea concentration, which was higher (P<0.05) for cows receiving cassava shavings compared to those receiving flocculated corn.
The relationship between ruminal starch availability and microbial protein synthesis is associated with milk production and composition. Nutritional deficiency can reduce milk stability in the alcohol test (Martins et al., 2019).
Ruminal degradability of starch and protein influences the expression of casein subunits, associated with microbial protein production and the supply of ruminal undegradable protein available for milk synthesis (Li et al., 2014).
A decrease in the kappa casein percentage is associated with the alcoholic instability of milk, and the degradability of crude protein, along with starch in the rumen, this needs to be adjusted to meet the nitrogen demand for microbial synthesis and the supply of amino acids to the cow.
To evaluate the association between increased starch degradability, N utilization efficiency, and milk composition and stability concerning corn processing, Martins et al. (2019) conducted a balanced Latin square study in five contemporary periods, in a 2x2 factorial arrangement with 20 dairy cows.
1 2
The first factor considered was corn processing, with treatments:
ground corn (average of 952 ± 1.86 μm particle size), or
flocculated corn (density of 280 g/L).
The second factor considered was crude protein degradability:
high: 107 g rumen-degradable protein/kg dry matter and 51 g rumen-undegradable protein/kg dry matter, or
low: 95 g rumen-degradable protein/kg DM and 63 g rumenundegradable protein/kg DM.
There was an interaction effect between corn processing and crude protein degradability on dry matter intake, with cows fed ground corn and low degradability protein showing increased dry matter intake (1.73 kg/d), increased milk production by 2.3 kg/d, and increased heat coagulation time (a measure of milk stability) by 4.8 min compared to cows fed ground corn and high degradability protein.
Cows fed flocculated corn had higher digestibility of dry matter, starch, crude protein, and ether extract, and the diet with ground corn and low crude protein digestibility had lower apparent NDF digestibility than the others (p = 0.041).
There was no interaction between corn processing, protein degradability, and casein subunit expression, nor milk protein content.
However, cows fed low degradability crude protein diets had higher kappa casein content, as well as milk protein content, and nitrogen use efficiency for milk production than cows fed high degradability crude protein diets.
Although flocculated corn reduced dry matter intake (which may be related to increased hepatic oxidation of propionic acid, facilitated by increased ruminal starch digestibility; MARTINS et al., 2019), no effect was observed on milk production compared to groups fed ground corn, indicating higher productivity in cows fed flocculated corn.
The results suggest that recommendations for crude protein degradability in lactating cow diets should correlate with the source and ruminal starch availability, associated with grain processing.
Overall, in response to the flow and availability of amino acids for milk protein synthesis, crude protein degradability is correlated with milk stability.
Grain processing techniques can increase the digestibility of feed, influencing the starch digestion site, favoring ruminal degradability, and influencing ruminal microbial protein synthesis.
Physical changes associated with breaking the grain pericarp protein matrix and decreasing particle size are associated with increased ruminal degradability and total digestibility of grains.
Additionally, gelatinization promoted by wet processing involving steam, temperature, and pressure facilitates enzymatic action on starch granules.
In this sense, the use of processed grains constitutes an alternative to meet the nutritional demands of ruminants and increase the digestibility of feeds used in cattle nutrition.
References upon request.
Grain processing in cattle feeding DOWNLOAD ON PDF