
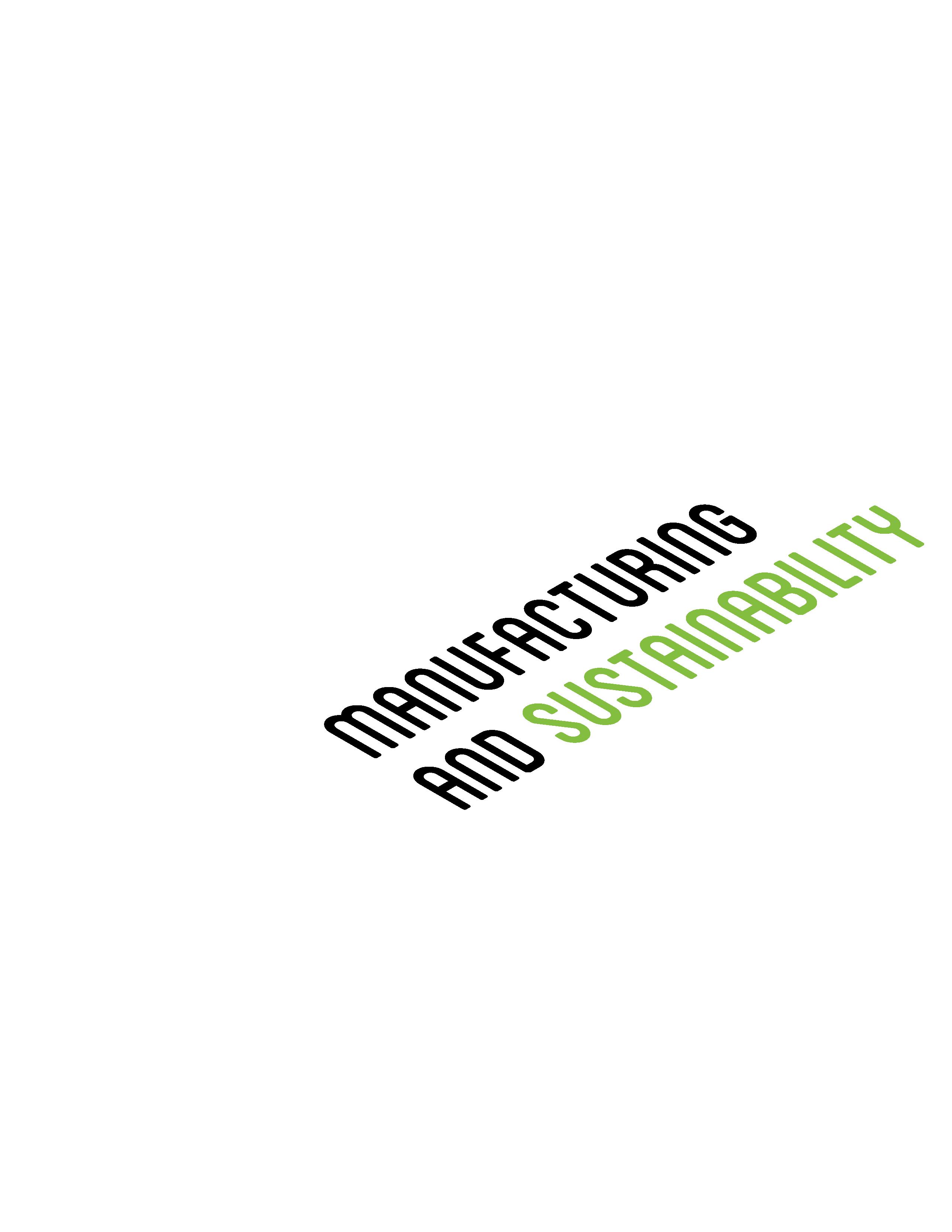
With over two decades of experience, BioLegend has become the leading provider of flow cytometry reagents, bolstered by an extensive antibody clone library, spectrum-spanning fluorophores, and an unwavering commitment to quality. With our continued support and teams of dedicated scientists at every level, you can confidently construct the perfect panel to identify your cells in research areas like immunology, cancer, developmental biology, and neuroscience.
• Our proprietary library of antibody clones can be combined with dozens of fluorophores to yield thousands of combinations.
• Spark Dyes, Fire Dyes, and Brilliant Violet™ antibody conjugates expand panels into the ultraviolet and infrared range.
• Supplement your experiments with buffers optimized for surface, intracellular, and nuclear staining.
• Our new and reliable compensation beads bind to most mouse, human, rabbit, rat, hamster, and donkey immunoglobulin isotypes. They are provided in two separate vials for positive and negative populations.
Discover the difference at biolegend.com/en-us/flow-cytometry
Manufacturing has been around for a long time, from hand production to automated machinery. Regardless of the method, it has undoubtedly improved and continues to improve our quality of life. With advancements in technology, we have witnessed accelerated production and expansion in breadth of goods from items of need to those of want. This convenience is at the expense of the environment, and unfortunately, we have already begun to witness the detrimental side effects of this. Ironically, this ends up impacting our quality of life as we face depletion of resources and a deterioration of habitable land.
The question today is how do we make manufacturing sustainable? There are many approaches with no one-size-fits-all solution. To further complicate the matter, the process of manufacturing is deeply intertwined in many societal factors. Despite the difficulty, all is not doomed. There are valiant efforts made in current manufacture to develop and implement sustainable methods, utilizing science to neutralize or counteract byproducts of manufacturing and cultivating green mindfulness in future innovations.
In the cover of this issue, we depict the bridging of manufacturing and sustainability. Manufacturing is represented by the automated assembly lines. Sustainability efforts can be seen in various stages of the assembly line, from the green colouration of the lightbulb to the insertion of a green sprout in the lightbulb. There are two layers to this design – one being the physical integration of green efforts in manufacturing and second being the green idea generation represented by the lightbulbs. Though canonically observed as two opposing forces, both manufacturing and sustainability are represented harmoniously in the cover, offering the outlook that integration of both is not far from the future.
In this issue, our design team effectively employs a green and blue colour palette with gray mixed in to represent the element of sustainability and manufacturing. By varying the shades of the colour palette, the design of each article sets a different tone. The articles are further decorated with photographs and whimsical illustrations. As you read through this issue, we hope you appreciate the incredible and detailed efforts of our designers in their visual story-telling. One magazine issue after another, our design team continues to impress us with their creativity. As such, we would like to thank all the new and returning designers for their beautiful contributions.
- Kitty Liu-
EDITORS-IN-CHIEF
Philip Barbulescu
Karen Yeung
DESIGN DIRECTOR
Kitty Liu
SOCIAL MEDIA COORDINATOR
Salma Sheikh-Mohamed
SENIOR EDITORS
Philip Barbulescu
Baweleta Isho
Meghan Kates
Manjula Kamath
Diana Nakib
James Pollock
Salma Sheikh-Mohamed
Siu Ling Tai
Deeva Uthayakumar
Matt Wong
Stephanie Wong
Karen Yeung
DESIGN ASSISTANTS
Jennifer Ahn
Philip Barbulescu
Baweleta Isho
Meggie Kuypers
Diana Nakib
Louis Ngai
Deeva Uthayakumar
Karen Yeung
Tianning Yu
Evey Zheng
CONTENT CONTRIBUTORS
Philip Barbulescu
Baweleta Isho
Robbie Jin
Manjula Kamath
Meghan Kates
Steve Lee
Ammarah Naseer
Natalia Ogrodnik
James Pollock
Salma Sheikh-Mohamed
Siu Ling Tai
Alara Tuncer
Deeva Uthayakumar
Matthew Wong
Stephanie Wong
Jason Yang
Tianning Yu
Evey Zheng
FOUNDING EDITORS
Copyright © 2013 IMMpress Magazine. All rights reserved. Reproduction without permission is prohibited. IMMpress Magazine is a student-run initiative. Any opinions expressed by the author(s) do not necessarily reflect the opinions, views or policies of the Department of Immunology or the University of Toronto.
Yuriy Baglaenko
Charles Tran
First
Second
The circular economy of human anatomical waste
Giving a second life to our excess bodily material
Waste management around the world
Local solutions to global sustainability crisis
The era of sustainable investing
ESG and corporate responsibility
Milestones on the road to sustainable manufacturing
Diverting the course for the end of the world as we know it
Investment banking: bridging academia and life sciences industry
Man’s trash is a microbe’s treasure: Bioremediation as a pollution solution
Industry 4.0 and the promises of sustainability - Will it be enough?
Developed by the Dow Chemical Company, Eco-compass is a compilation of data about the eco-efficiency of a product’s life cycle based on 6 “pillars” of sustainability.
Repackaging the future - Sustainable packaging in the pharmaceutical industry
1990s: “Eco-e ciency”
Eco-efficiency describes environmental management strategies that ensure competitively-priced high-quality products which are also progressively environmentally-friendly. Example: The Eco-compass tool models sustainable product innovations and compares them to base methods.
Biodegradable polymers in biomedical applications: A sustainability perspective
Adding “green” to the formula
EV-ening out the costs: A look into the challenges of electric vehicles
Is your “recycling” actually recycled?
Adoption of the “6Rs” to CP and eco-efficiency: reduce, reuse, recycle, recover, redesign, remanufacture
Biodegradable products can break down into non-toxic materials when exposed to naturally-occurring bacteria. Replacement of traditional plastics with biodegradable ones reduces landfill waste and green
Carbon pricing: Putting a price tag on climate change
Where is additive manufacturing today and why isn’t it mainstream?
Thinking about the future
The Earth post-humanity: A book review of Alan Weisman’s The World Without Us
We would love to hear your thoughts! Comment on our articles online or send us an e-mail at editor@immpressmagazine.com
Since the first industrial revolution in the mid-1700’s where breakthroughs in science and engineering led to the unprecedented rise in production output, the abrupt growth that societies experienced allowed for larger population capacities and an increased quality of life. For a large part of history, this endless need to progress has caused a reckless craving for finite resources. Now, a global audience witnesses the consequences of production—pollution and the impact it has on the environment—as we share a growing fear that the current trajectory of progress will be unsustainable for future generations.
In recent decades, a growing cognizance towards sustainable practices and waste production has been felt at all levels of today’s world. From changes in government policies to tax carbon emission, efforts in favor of cleaner energy production, greener alternatives advertised by companies in all industries, and people trying to reduce their own carbon footprints, there is a noticeable shift in attitude towards reactive and proactive mitigation of our environmental impact.
With this issue of IMMpress Magazine, we dive into the progress humanity has made in the past and present, as well as the plans proposed in favor of a sustainable future. We begin with an infographic detailing the development of sustainable manufacturing throughout the years (p10). We highlight topics that aim to reduce waste production such as the concept of a circular economy (p12), countries around the world that lead by example with their waste management policies (p16), and bioremediation (p20). We discuss policy and government involvement in advocating for a greener future through the promotion of sustainable investing (p15), clean energy (p18), and carbon pricing (p30). We look into the future of manufacturing through topics like sustainable packaging (p24), electric vehicles (p28), and 3D printing (p32). Additionally, we focus into the progress made by the scientific community with the goal of sustainable alternatives such as synthetic polymers (p25) and green chemistry in the pharmaceutical industry (p29). Thank you to our alumnus, Dr. Evelyn Pau for her interview and giving us a closer look into investment banking in the life science industry (p16). Lastly, this issue includes a 2022 Benchmarking infographic highlighting the growth IMMpress Magazine continues to see over the years (p8).
As always, we are incredibly grateful for our immpressive team of talented writers, editors, and designers who worked tirelessly to make yet another wonderful issue possible. Thank you to the Department of Immunology for their continued support and to our sponsors at BioLegend for their generosity.
As we approach the new year, we hope the incoming Fundamental PhD and Applied Masters students in the Department of Immunology are happy with the labs they have joined. We also want to wish all our readers a happy holiday season and a joyful start to the New Year!
The Graduate Peer Support Network (GPSN) Mentorship Program is looking for motivated individuals to join our mentorship team as a peer mentor. Scan to fill out our recruitment form.
Twitter:
GPSN _UofT
Instagram:
gpsn uoft
Website:
uoftgpsn.ca
The Department of Immunology will be celebrating its 40th anniversary in 2024, and for the last 10 years IMMpress Magazine has been an amazing publication from our creative and industrious graduate students, which has transformed how we inform and communicate with each other and our alumni. This issue explores “Manufacturing and Sustainability”, how the process of making products changed humanity, arguably for the better, but how it also continues to affect our lives and the environment.
The relentless IMMpress team has constructed an extraordinary issue, which delves into how things are made, how pollutants or waste can be removed, reduced, reused, and recycled. Additionally, the alumni interview with Dr. Evelyn Pau offers us a view to her work in investment banking, which not only fits well with the theme of this issue, but also highlights the incredible diversity in career tracks that our alumni pursue and excel in after completing their graduate studies at UofT Immunology.
I would like to applaud the outstanding IMMpress editorial and production team and contributors for providing us with the latest Benchmarking for the magazine, which makes it clear that it is having a tremendous impact in Canada and around the world.
Happy to say that we just completed our Department’s cyclical Self-Study, which will be part of the upcoming external review in March 2023. I look forward to the feedback as to how we have progressed over the last 5-years and what remains still to be achieved. I am extremely thankful to all that have contributed in putting together the Self-Study, which was a major undertaking that I will be pleased to share early in the new year.
We are also marking the upcoming retirement of Sherry Kuhn, our Department’s Business Officer, who for the last 22 years has been the backbone of UofT Immunology. Her love and dedication for the Department translated into her ability to have a tremendous impact and enduring legacy for our academic unit. We are ever so thankful and wish her the best in her future endeavors.
I know that we are all looking forward to the end of another pandemic year, with hopeful optimism for a healthier new year in 2023. I extend my best wishes to all for the new year, filled with scientific, educational, and personal achievements.
I hope you enjoy this issue, and importantly whether you are an alumnus of the department, or a prospective student, I really look forward to hearing from you.
With2023 on the horizon, we are quickly approaching a decade of IMMpress Magazine’s continued publication. We want to thank our talented and hardworking team of writers, editors, and designers who have made it all possible, and the Department of Immunology at the University of Toronto for the continuous years of support! IMMpress Magazine has always aimed to start the conversation on interesting and relevant subject matter in the realm of science we believe deserve a spotlight. We hope that our readers from across the globe continue to enjoy our wide range of content and look forward to the many issues we have planned ahead!
This year, the IMMpress website saw:
22,740 Total page views
1,700
Monthly average website Visits from unique users
83% New
17% Returning Visitors
3% Australia
77% Desktop
83%
22% Mobile
17%NewReturning
1% Tablet Devices
Where are our readers ?
8% United Kingdom
3% Philippines
25% Canada
5% China
2% France
2% Germany
22% United States
2% Kenya
4% India
2% Japan
Healthcare in a Technological World Volume 9, Issue 3 - December 2021
Nanotechnology: Small but mighty
By: Meghan KatesDesigner Babies: A potential future of genetic inequality
By: James Pollock
Work to Live, or Live to Work Volume 10, Issue 1 - April 2022
Labor unions: The good, the bad, and the ugly
Vaccine Hesitancty in the Age of COVID-19
By: Rahman Ladak & Aly LadekBy: Baweleta Isho
The Gig EconomyInnovation or exploitation
By: Manjula Kamath
Metabolism and microbiome: Tiny universes Volume 10, Issue 2 - August 2022
Improving food with fermentation: Super-food or super-fad?
By: James Pollock
Pica cravings to the extreme
By: Faustina Lau
Industry-made environmental calamities and pollution led to the rise of envrionmentalism. The resulting global movement led to tighter government-imposed restrictions on industrial waste production. Example: Clean Air Act (USA) and Canada Water Act (Canada) in 1970
North American and European countries were the first to implement these standards, despite not enforcing them in manufacturing endeavors on foreign soil. This emphasizes the need for a globalized effort to reduce pollution.
Pioneer industries adopted Cleaner Production (CP) strategies, to conserve resources, reduce harmful wastes, and reduce the envionmental impact of products throughout their life cycle. Example: Green chemistry is the use of alternative starting materials to reduce toxic waste from chemical synthesis.
The Boots/Hoechst Celanese process replaced excess reagents with the usage of catalysts to produce ibuprofen, significantly reducing the chemical waste produced from maufacturing this drug.
1700s - 1800s
1700s - 1800s: The First and Second Industrial Revolutions
Early 2000s
Resource conservation
Revalorization
Mass intensity
Energy intensity Service extension
PROCESS 1
2
Developed by the Dow Chemical Company, Eco-compass is a compilation of data about the eco-efficiency of a product’s life cycle based on 6 “pillars” of sustainability.
1990s: “Eco-e ciency”
Eco-efficiency describes environmental management strategies that ensure competitively-priced high-quality products which are also progressively environmentally-friendly. Example: The Eco-compass tool models sustainable product innovations and compares them to base methods.
2000s: Closed-loop production
Adoption of the “6Rs” to CP and eco-efficiency: reduce, reuse, recycle, recover, redesign, remanufacture Example: Biodegradable products
Population Pollution Manufacturing Recover
Biodegradable products can break down into non-toxic materials when exposed to naturally-occurring bacteria. Replacement of traditional plastics with biodegradable ones reduces landfill waste and greenhouse gas emissions.
Post-consumerRemanufacture Production Redesign
IMMpress Vol. 10 No. 3 2022 11
Every time I vacuum, it never ceases to amaze me the amount of hair and dust scattered around my apartment. The human body produces considerable amounts of waste, even if we are not always aware of it. Every day, the average adult sheds around 50 to 100 hairs from their head and loses up to 2.5 litres of fluids through urine, feces, and perspiration. Apart from our day-to-day routine, medical procedures generate anatomical waste, such as blood and organs. Furthermore, millions of people who sadly pass away each year are buried or cremated. Just like with plastics or food scraps, human-derived waste has a significant carbon footprint that we should strive to eliminate. We can apply the common principles of “reduce, reuse, recycle”, although it comes with many challenges. For instance, it is wholly unreasonable to ask people to reduce their daily bowel movements. This type of waste can also spread deadly diseases if mishandled. Nevertheless, if performed in a carefully controlled manner, we can find a new purpose to our bodily wastes.
For epidemiologists and public health experts, the sewage system is like a gold mine. Since everyone has bowel movements, the city’s wastewater is a useful resource for monitoring community health trends, like Mycobacterium tuberculosis infections and the prevalence of opiate use. Moreover, an infected person sheds 105 to 1,012 viral particles per gram of fecal matter. This has especially been useful for tracking the waves of COVID-19 and informing dynamic public health policies, such as masking requirements and indoor capacity limits. While this is not a strategy that will significantly reduce sewage, it is an illustration of how our bodies’ waste contains valuable information.
When it comes to deciphering the nuances of human diseases, research requires more than monitoring the general population. People are differentially impacted by illnesses and treatments due to heterogeneity in their genetic makeup and environmental exposures. This usually means studying patients at a cellular and molecular level, particularly at the site of disease. However, asking patients to donate tissues solely for research is unethical, as it would require potentially invasive procedures with minimal to no benefit for the patient. If the patient is already scheduled for a necessary medical procedure, then excess anatomical waste can be utilized by researchers with consent from the patients.
A primary source of human tissue for research comes from surgeries. For example, breast implant removals and revisions of patients with capsular contracture. This is a condition where fibrous scar tissue forms around the implant, which may cause chronic pain in severe cases. Researchers can analyze resected patient specimens under a microscope to determine if the type of implant correlates with scar tissue formation. Knowing this information will help improve the design of implants, and hopefully reduce the incidence of capsular contracture.
In situations where collecting material from the diseased site is not an option, peripheral material can be used instead. People with liver cirrhosis, pancreatitis or ovarian cancer may suffer from build-up of fluid in their abdomen caused by dysfunctional blood vessels. This fluid, also known as ascites, may have to be drained through a needle and discarded. Ascites is comprised of enzymes, immune cells, and
"A safe and effective treatment is perhaps the ultimate goal of biomedical research, and we may not have to look further than ourselves for the answer."
Getting ahead of the COVID-19 sh*t-storm
other factors that partially resemble the inflammatory environment of the diseased organ. Thus, researchers can take advantage of ascites in lieu of the actual organ to better understand the underlying pathology of these diseases.
Blood is another type of material that is useful in research, particularly for its serum and immune cell components. Since blood can be collected in large quantities, it is a useful tissue that allows for standardization over multiple experiments. Patients with hemachromatosis have excess iron stored mainly in red blood cells, and therefore undergo blood removals on a regular basis. These patients are a suitable alternative to finding healthy donors for blood, which often requires providing them with monetary incentives.
A safe and effective treatment is perhaps the ultimate goal of biomedical research, and we may not have to look further than ourselves for the answer. For one, organ transplants from deceased donors are undoubtedly lifesaving for many recipients. Sometimes, a cure can be derived from something less pleasant. Fecal microbiota transfer is a therapy approved for patients with recurrent C. difficile bacterial gut infections. Microbes and metabolites isolated from feces of healthy individuals can help re-establish a healthy gut community in these patients. Conceptually, this makes sense – if your bike helmet is split in half, it’s safer and more economical to take an extra one from your friend than to try and fix it.
The circular economy of human-derived waste is unique because it is driven by compassion and selflessness. It is truly remarkable how many patients choose to contribute to research, even though it may not improve their medical prognosis. I hope that their bravery can inspire more healthy individuals to donate to the medically vulnerable, especially in ways that are simple and painless. By registering as an organ donor, you could save one of 1,400 Ontarians on the organ transplant waitlist. It can also be as easy as donating hair for medical wigs. Even if your contribution is more supportive than curative, patients may find comfort in knowing that someone cares for their well-being.
Sustainability may commonly be associated with environmentalism, but it is also an intersection of many other fields. Beyond reducing our carbon footprint, repurposing anatomical and bodily wastes has improved and saved many lives, either indirectly through research or directly as therapeutics. There are also major economical benefits to utilizing this waste instead of spending money on synthesizing drugs. Putting constraints on resources fosters creativity that can lead to revolutionary innovations. For that, sustainability is worth pursuing in any industry.
"Beyond reducing our carbon footprint, repurposing anatomical and bodily wastes has improved and saved many lives, either indirectly through research or directly as therapeutics."
For humans; by humans
Diamonds form under stress
- Stephanie Wong
Underthe streets of Vancouver lies the Great Marpole Midden, an ancient landfill site once used by the Coast Salish people. The 4,000-year-old dump is a reminder that for as long as humans have existed, we have left behind waste. However, with the global population projected to exceed 11 billion by 2100, new strategies in waste management are needed to address present and future sustainability challenges.
In 2015, the United Nations Environment Programme reported that the global population generates 2 billion tonnes of solid household waste annually, with roughly four times as much commercial, industrial, and construction waste added to that pile each year. Since household waste generation per capita is strongly tied to national income, different countries take different approaches to waste management, often depending on their economic output, or Gross Domestic Product (GDP).
Low-income countries (LICs) tend to rely on informal waste collection and community initiatives with lower community coverage than high-income countries (HICs), which often subsidize formal municipal waste collection. LICs also tend to produce more organic waste and less paper waste than HICs, though HICs notably produce more “avoidable” organic waste than LICs. Often lacking formal municipal waste collection, LICs are compelled to innovate new strategies for disposing of hazardous electronic waste, the fastest growing waste stream in the world. For example, entrepreneurs in Nairobi, Kenya, have partnered with American tech companies to build “e-waste” disposal businesses to safely convert used electronics into raw materials that can be re-used locally or traded globally.
Among the countries with formal waste collection practices, some currently have a limited capacity for technological innovation and instead turn to legislative action to maintain waste management standards. The city of Bogotá, Colombia, uses a formal waste collection system but has worked with informal waste collectors since 1992. That year, Bogotá introduced organizations that protect the legal status of the 20,000+ waste collectors that use hand-pulled and animal-pulled carts to collect the waste that the formal system misses. By establishing standardized wages and improving health and safety conditions for their recyclers, the city has become a success story for improving waste management capacity through inclusivity.
On the other hand, some HICs are able to push the limit of technological innovation by creating a circular economy that reverts waste back into energy and raw materials. A waste management company partially owned by Malmö, Sweden, uses incineration, composting, and biogasification (which uses microbes to create methane from waste) to recover energy from trash that cannot be reused or recycled. This company, Sysav, uses waste to meet 60% of the heating demand in Malmö, create 25,000 tonnes of fertilizer annually, and produce the equivalent of 2 million litres of gasoline in methane.
While national or municipal waste management strategy is usually tied to a country’s GDP, innovative communities have come up with new ideas that expand their ability to manage waste beyond their financial means. It remains to be seen, however, if new ideas for waste management can keep up with the rapidly growing global population. If not, we may be in store for a future where everyone is down in the dumps.
- James PollockWhen it comes to investing, there are a multitude of factors that come into play - everything from the company’s reputation to tax rates to international conflicts. The latest trend in responsible investing is the incorporation of environmental, social and governance (ESG) factors. ESG factors can include things like a company’s view on climate change, their employee health and safety policies and their corporate culture, and is used by “socially conscious” investors to help them in their financial decision making.
SinceESG investing involves screening companies based on social factors in addition to financial valuation, it encourages companies to act responsibly. As a result, additional investors become interested in investing in companies that are viewed as socially responsible, which promotes increased responsibility, which attracts more investors and you can see where this is going. In the end, the company’s valuation as well as their capital options increases. Furthermore, not only are ESG practices beneficial to shareholders, but to company employees as well. A company with high ESG performance means that their employees are receiving salaries above a living wage, that the company’s culture is safe and non-toxic, and that there is an emphasis placed on employee wellness.
Speaking of employees, not all ESG practices are equal. For example, some companies focus on the environmental aspect of ESG and employ sustainable water and waste management policies. However, this is relatively simple and can be employed by any company. It won’t necessarily provide the company with an edge in terms of their ESG score. However, a social factor like incorporating ESG policies into the recruitment and retainment of employees is a bit more difficult to do. For example, skincare company Neutrogena has been praised for their “thoughtful” company culture. According to the company, the average tenure at Neutrogena is approximately 10.2 years.
This impressive employee retention rate has been attributed to things like the company’s emphasis on work-life balance, health benefits and creating a collaborative culture. These factors place companies like Neutrogena, Google, Airbnb and others on a much higher level when it comes to ESG scores.
ESG practices are also extremely helpful when it comes to financial valuation in the global market. Studies by financial analysts have found that companies with strong ESG disclosures benefited greatly in the stock market when the European Union announced stronger disclosure requirements. This was especially beneficial for countries with strong ESG disclosures that were considered emerging markets such as South Africa and Brazil.
Overall, ESG practises serve great benefits to companies, but the ultimate question is - do companies actually implement these practices? One report from earlier this year stated that 90% of the companies included in the S&P 500 published some form of ESG reporting in 2022. The impact of placing importance on ESG can also be seen in studies that track the flow of investments. For example, sustainable funding inflows went up from $5 billion in 2018 to $50 billion in 2020. Lastly, with increases in awareness of social justice issues, financial analysts also found that socially-conscious investment proposals went up 37% from 2021 to 2022.
ESG-based investing is still relatively new in the financial world. On one hand, despite data showing the benefits of ESG practices, experts agree that most companies treat ESG as extra padding. Something that is nice for extra protection, but not necessary. On the other hand, it may still be too early to make conclusions about the integrity and longevity of ESG practices. As ESG becomes more popular, the already substantial influence that corporations have on the global political and social framework has the potential to be a real force of change in the world. Whether that change will be good or bad is yet to be determined.
Climate change, driven by human activities such as burning fossil fuels, threatens the natural balance of our home on Earth. Already affecting the vast majority of regions around the globe, we have observed long-term shifts in weather and temperatures outside the range of natural variations of the solar cycle. From rising sea levels to intense droughts, we must adequately and urgently address this crisis – and one quick and effective way we can reduce greenhouse gas emissions is through clean energy.
Clean energy and renewable energy are often used interchangeably, however, they have distinct meanings.
Importantly, this wording has huge implications in developing and following government policies.
Clean energy is the generation of power without negatively impacting the environment (i.e. zero-carbon energy sources) and encompasses renewable energy, nuclear power, and carbon neutralizing technologies. The definition of renewable energy is more specific; it is power
production through natural sources that are continually replenished at a faster or equal rate to its consumption. Sources of renewable energy include solar, water, wind, biomass, and geothermal power.
Yet, some forms of clean energy pose some issues. For example, abundantly used in Toronto, Ontario, nuclear power requires additional safety measures and special infrastructure for storage of spent fuel. Furthermore, many skeptics argue that many forms of renewable energy (ie. nuclear, wind, and solar) are intermittent. However, this can be mitigated through diversifying the sources of energy, using gas turbines or batteries to store energy, and expanding across geographies to capture varying weather conditions. Although the infrastructure of renewables requires financial support, raw materials, and land space, the indirect emissions are still much less carbon intensive than using fossil fuels.
Some countries are already paving the way in reducing emissions, using renewable energy and effective policies. For example, 50% of Swe-
den’s energy needs are fueled by renewable resources such as hydropower and bioenergy. For the last 7 years, Costa Rica has generated 98% of their electricity through hydro, wind, geothermal, solar, and biomass energy. Norway has been using hydropower since the 1800s and currently provide 98% of their electricity through a combination of hydro, thermal, and wind energy. Compared to these inspirational climate leaders, where does Canada fall on the list?
Canada currently utilizes renewables to produce 18.9% of our power. Hydroelectricity is the most important form of renewable energy in Canada, generating 59.3% of our electricity, followed by wind (3.5%) and biomass (1.4%). Quebec currently produces the highest amount of hydroelectricity out of all the provinces, with the second being British Columbia. Given Canada’s vast land space and varying geographies, there is huge opportunity to harness many sources of renewable energy with proper investment and establishment of infrastructures. Canada has implemented a new federal policy to prioritize the purchase of lower-carbon materials for construction projects, which aims to reduce half of the emissions from public and private infrastructure construction in the next decade. If provincial and municipal policies can follow, this will build a solid national foundation for continuing and enhancing these policies in the future. Moreover, the latest statement from the Canadian federal government has introduced clean energy tax credits, encouraging sustainable practices and infrastructure for clean energy sources.
Not only are our ecosystems, water supply, and food availability impacted, climate change also impacts human health. According to the World Health Organization, climate change is predicted to cause increases in deaths due to zoonoses (eg. malaria), malnutrition, heat stress, and natural disasters. Importantly, these crises will disproportionally affect low income and disadvantaged countries with less healthcare resources. Even worse, these communities are the ones that contribute the least to the driving forces of climate change, thus further widening the gap in long-standing socioeconomic and health inequalities. How-
ever, the current trajectory of climate change can still be diverted with targeted efforts to reduce the impact of human activities. Despite the concerns regarding certain clean energy technologies, research continues to advance and innovate new solutions. Coal, oil, and gas still account for over 80% of electricity around the world. Given that climate change poses a huge threat to humanity, we must continue to advocate for meaningful policies and infrastructure that can reduce harmful emissions to zero as soon as possible.
Hydroelectricity harnesses the power of flowing water to spin turbines and drive an electrical generator.
Biomass refers to the generation of energy from a solid, liquid, or gaseous state of a plant or other biological materials, such as solid landfill waste, sewage, and wood waste from industrial activities.
To produce energy from the wind, the power of wind speed is used to spin turbines.
The energy from the sun can be converted to electricity as well as provide light and heat.
The heat beneath the earth’s surface can also be captured to produce geothermal energy.
Research has also begun on developing technologies to utilize ocean waves to produce power.
Investmentbanksplayan indispensablerolein transformingacademic discoveriesintoinnovation.
Dr.EvelynPau isaPhD alumnafromtheDepartmentof ImmunologyattheUniversityof Toronto(Classof2013;theWither lab).Dr.PauiscurrentlyVice Presidentatahealthcare-focused investmentbank,BloomBurton SecuritiesInc.,andpreviouslyserved ontheBoardofDirectorsofthe McMasterInnovationPark.
CouldyoupleaseexplainwhatBloom Burtondoesanddescribeyourrolein thecompany?
BloomBurtonisCanada’sleadinglife science-focusedinvestmentbanking firm.Weworkwithtwomaintypesof clients.Thefirstisinvestorslookingfor investmentopportunitiesinhealthcare organizations.Theotheris organizationsthatarelookingfor capital.BloomBurton&Co.works acrossawidespectrumofhealthcare organizations,includingdifferent stagesofdevelopment(start-upto publiclylistedcompanies)anddifferent typesoforganizations,suchasbiotech, pharmaceuticals,digitalhealth, hospitals,anduniversities.Myroleis focusedonconsulting.Isupportmy clientswithinourscientificbanking teamtoprovidestrategicadvice, launchnewbusinessdevelopment initiatives,andengageinM&A transactionalwork.Whetherweare lookingforanewdrugtargetor diseaseindicationtopursue,oridentify newproductsorcompaniestoacquire, weassistourclientsbasedonour insightsfromhealthcareinvestorsto ultimatelygetourclientstoachievea monetizationevent.
Whatdoyouthinkisthecurrent relationshipbetweentheinvestment sectorandacademiainbiotech?
Thereisastrongandlongrelationship betweenthebiotechinvestmentsector andacademia.Usually,academiais wheretheideastarts.However,initial academicdiscoveriesaddressvery specificproblems,andrarelyemphasize keyinformationforcommercialization. Thisincludesunderstandingthe market,competitors,andclinical impact.Academicdiscoverieswith goodpotentialforcommercialization arefundedbyinvestors.Oftentimes, investorsattendacademicconferences tocommunicatewithacademia,andto learnnewideasanddiscoveries.
Duringyourgraduatestudies,what motivatedyoutopursueconsultingin thefirstplace,andultimately investmentbanking?
Iwasactuallyinterestedinthebusiness ofscienceevenbeforeenteringthePhD program.Iembarkedonmydoctorate tobuildmyscientificfoundationand trainproblem-solvingskills,whichin myopinionmaybemuchmoredifficult thanbuildingbusinessacumen.
DuringmyPhD,Ididvariousextracurricularactivitiesto learnbusinessskills:businesscasecompetitions,healthcare consultingprojects,andworkinginamed-techstartup company.Throughouttheseexperiences,Iimprovedmy analyticalandcommunicationskillsthatareimportantfor clientengagement.
IchoseinvestmentbankingbecauseIcanparticipatein shapingandfundingcompaniestogrowwhileremainingin contactwithcutting-edgescientificdiscoveries.Ifinditvery impactfultobringnewproductsanddrugstoCanadiansin atimelymanner.Asaninvestmentbanker,Iseethefull spectrumofthevaluecreationprocesscoveringthestrategy, execution,andimplementation.
Whatwasyourperceptionofconsultingandinvestment bankingbeforeyoustartedyourposition?Havetheychanged sofar?
Communicationsskillsalsomatter,especiallywhenengaging withclients.Thisincludeswritingprofessionalemails, speakingconciselyaswellasconfidently,andaskingcritical questionsinrealtimeregardingfieldsofwhichyouarenot necessarilyanexpert.Suchlevelofconfidenceoftenrequires trainingovertime,butisveryimportantforconsultantsand investmentbankers.
Iamanimmigrantmyself,andmypartnerisasmallAsian woman.Weoftendiscussdifficultiesthatarefacedbypeople invisibleminoritygroups.Ifyouhaveanyadvicefor graduatestudentsseekingindustrypositionswhoarein visibleminorities,couldyoupleasesharewithus?
IcanspeakfrommyexperienceasanAsianwomanwhois petiteandintroverted.Thefinancialindustryisstillmostly maledominatedbutischanging.Iusuallyconnectwith femaleprofessionalsinnetworkingevents.Forashort womanlikeme,itismorechallengingtospeakwithtall malesespeciallyinloudenvironments.And,becausemany ladieswearheelsitismorenaturalformetoinvitethemto sitdownatatable.Thismakesiteasiertoengagein conversationwitheyecontactinamorerelaxingatmosphere.
Initially,Ithoughttherolewouldrequiremorefinancialand accountingskills.However,nowIlearnedthatitismuch morescientificthanwhatIanticipatedatleastwithinmy role.IreadmoreacademicpapersinthefirstmonthatBloom Burtonthanduringmygraduatestudies.Understanding academicdiscoveriesandpresentingkeyinformationtothe audienceinapreciseandtimelymannerisveryimportant. Forexample,readingapieceofscientificliteraturetakesonly secondsforme.
Readingpapersmustbecriticalasyousaid.Whataresome additionalskillsthatwouldbeimportantbutwasnot extensivelycoveredduringyourgraduatetraining?
Learninginformationbeyondyouracademicexpertiseisa goodskill.Forexample,Ireadscientificpapersfromvarious fieldsotherthanimmunologyallthetime.Ifindthatjournal clubpresentationisagreatmethodtobuildthisskillset.
Pleasesharewithusanycareeradviceforgraduatestudents.
Iwouldrecommendtoalwaysparticipateinextracurricular activitiestofindyourcareerpassionandtolearnwhatisnot coveredingraduatetraining,likeinterviewskills.Ifyoustay humbleandkeepnetworking,opportunitieswillcomeup. ForPhDstudents,yourthirdyearisagreattimetostartthis process.
-SteveLee“Ifyou stayhumble andkeep networking, opportunities willcomeup.”
Pollution is a growing problem around the globe and only increasing as industries rise to face the challenge of a booming population. Pollution from factories, oil spills, and heavy metal contamination can linger in the environment, leading to potentially catastrophic effects. These contaminants can leach into ground water and contaminate agricultural soil. Degradation of agricultural soil in turn can lead to a reduced crop yield, which can exacerbate existing social problems of food insecurity and poverty that are rising with our growing population. However, there is a potential solution to the growing epidemic of man’s trash: bioremediation.
Bioremediation involves the use of organisms to clean contaminated soil and groundwater. These organisms are often microbes that have a remarkable ability to utilize the most improbable of resources in their environment to synthesize components essential to their survival. Bioremediation takes advantage of indigenous (naturally-occurring) or engineered microbes that are capable of consuming and processing environmental contaminants into a form that is no longer toxic to humans, animals, or agriculture. However, to effectively metabolize environmental contaminants, microbes require additional nutrients that are often scarce in the polluted area. In this scenario, a technique called biostimulation is used, where limiting nutrients such as nitrogen and phosphorus, are added to the environment to stimulate the metabolic pathways in microbes that are needed for decontamination. Biostimulation is sufficient for bioremediation if indigenous microbes are capable of processing the contaminant. However, if indigenous microbes are not present in the contaminated environment, the alternative is bioaugmentation, where microorganisms capable of degrading the contaminant are introduced to the site.
It is important to understand the metabolic pathway used by a microbe to transform the contaminant to ensure this microbe (engineered or indigenous) will produce a less harmful by-product than the original contaminant. Additionally, a better understanding of the metabolic pathway will shed light on what environmental conditions will favour degradation, which can then allow for manipulation of this environment to favour bioremediation. Such factors include temperature, water potential, pH, and other nutrients.
Bioremediation has been used in several practical field tests to great effect. A notable example was after the Exxon Valdez oil tanker accident in 1989, where 40.8 million litres of crude oil were released into the Gulf of Alaska. A portion of the contaminated shore line was used in a biostimulation test to determine if bioremediation could successfully clean up the area. Since petroleum is high in carbon but low in nitrogen and phosphorus, fertilizer containing these components was sprayed along the contaminated shoreline to increase the activity of indigenous hydrocarbon-degrading bacteria. This process had great effect on the four beaches that the fertilizer was applied to from 2011 to 2012. Biostimulation increased the natural degradation rate by 12- to 60fold. However, more than 30 years after the oil spill, harmful hydrocarbons still remain along the shoreline and maintain their long-term negative effects on the ecosystem.
Although this case study shows the power of bioremediation, it also demonstrates some potential drawbacks. Microbes take time to perform the degradation process. It is not an ideal technique if an immediate solution to the problem is required. Unfortunately, the faster alternative is to haul the contaminated material away to be incinerated or dumped into a landfill. Comparatively, bioremediation is a publicly-accepted, greener technique that involves directly treating the contaminated site. While promising, more work needs to be done to improve this technique for wider field applications.
Recently, much interest has gone toward the use of bioremediation to decontaminate agricultural soil from heavy metal and metalloid contamination. Build up of metals (such as cadmium, arsenic, copper, mercury, lead or chromium) in the soil can be due to factory run off and industrial pollution. Heavy metal contamination in the soil can lead to loss of crop yield which poses a threat to global food security. While some plants, such as most crops, fare poorly in soil contaminated with heavy metals, other plants are specially adapted to grow in the presence of these contaminants. Such plants make up another type of bioremediation, referred to as phytoremediation, which can be employed to protect crop yields.
Phytoremediation takes advantage of plant species that have adaptations to neutralize the toxicity of heavy metals through a variety of means. One neutralization method used by plants is restricting heavy metals only to their roots by
converting them to a non-toxic form that will not move to the rest of the plant. Additionally, some plants can trap metals in their cell wall to prevent these contaminants from affecting the health inside the cell. Finally, certain plants can detoxify metals within the cell by modifying their structure. When metals are moved above the root system, plants that are repulsive to herbivores should be selected to prevent the metals from entering the food chain. In any of these cases, metals are taken out of the soil and groundwater where they are stored in a form that should not harm the environment. Plant species need to be carefully selected for optimal growth in the region of interest, where they can often be grown alongside crops to increase the productivity of the agricultural region.
As a phytoremediation trial, two plant species, Pteris vittata and Sedum alfredii , were planted in southwestern China in an area contaminated with lead, cadmium and arsenic. Over the course of two years, the cultivation of these plants in the area led to a significant reduction of metal contamination. Therefore, using plants for bioremediation is both cost-effective and feasible in cleaning contaminated soils.
Microbial bioremediation can also be performed in soils contaminated by heavy metals. Some bacteria can release small molecules called siderophores which bind heavy metals and change them into a form that is not harmful to the environment. Additionally, some bacteria can take up and metabolize heavy metals into a non-toxic form. Microbial bioremediation and phytoremediation can be used in conjunction or to replace one another where the environment is more suited to a particular technique.
Overall, bioremediation is a slow but growing solution to help eliminate pollution from contaminated soils and groundwater. It takes advantage of the ability of plants and microbes to accumulate or break down contaminants to eliminate pollutants. Ongoing research into improving growth, survival, and ability to break down contaminants will help to improve the practicality of bioremediation in the field. Understanding these organisms and harnessing their potential to reduce pollutants can help to create a cleaner and greener world.
- Meghan KatesIn 1764, James Watts sat by a Newcomen Steam Engine and wondered—why waste all that steam with no direction? That question sparked the idea and the eventual invention of the separate condenser which fueled the first industrial revolution, creating the blueprint to the modern steam engines we use today.
The first Industrial revolution began with the mechanization of England’s textile mills and a transition from farms into factories. On the one hand, the industrial revolution brought a boom of productivity with great economic and social change and development, but on the other hand, as lamented by Dickens in Hard Times, brought suffering and misery for the working poor. At the time, it was too soon for Dickens to comment on the effects of the industrial boom on the environment, as the impact unraveled in the later decades.
With the arrival of electricity, the first industrial revolution transformed into a second period marked by the development of transportation networks. The newfound availability of steamships, airplanes, and automobiles greatly improved resource accessibility throughout England. In1952 industrialization began to be considered a health hazard when a smog covered London, killing over 4000 people in a week due to excessive coal burning. Still, by the end of the twentieth century, most countries had
become dependent on using fossil fuels.
With the invention and implementation of computers, large-scale manufacturing was realized, and the next period arrived with the speed of automated systems. This normalized the daily use of electronics and information technology. Today, we have something emerging and carrying—not just the advancements made by its ancestors—but also the accumulated burdens. Industry 4.0 is all about connectivity—it promises the use of smart factories defined by interconnected machines and intelligent robots.
Industry 4.0 will also incorporate big data, Internet of Things, and Artificial Intelligence. Companies and operations will be run by a network of computers, allowing predictive maintenance and processing of large data sets to meet immediate manufacturing needs. Successful businesses will also likely have to rethink their business models to leverage more technology. Industry 4.0 has remarkable potential to strive for sustainability, however, emerging in a society with cumulated burdens like climate change, this will likely remain challenging.
According to the World Economic Forum, some of the recent concerning global trends are growing socio-economic inequality, increasing environmental decline and climate change with regional risks to social instability, unemployment, resource depletion and natural catastrophes. For Industry 4.0 to be worth the “hype”, it must leverage
its capability along with novel technologies to alleviate some of these trends.
The United Nations has already come up with the Sustainable Development Goals which are intended to help countries transform their economies while calling “action to end poverty and inequality, protect the planet” and “ensure that everyone enjoys the same access to health, justice and prosperity”. To ensure sustainability, Industry 4.0 will likely have to play part by changing the meaning of economic value creation—value will not be created in the expense of the environment and its people but rather conscientiously considering the needs and longevity of the planet and its inhabitants. Still, there are concerns about data security, loss of jobs due to robots and AI that will come with this age of technological advancement. But one of the greatest challenges that remains in the spotlight is the sustainability and how we are going to reverts the effects of climate change.
In 2015, world leaders formed the Paris Climate Agreement, having a common understanding that a 2.0°C global rise in temperatures would be devastating, they settled to stay under 1.5C. However, the global rise in temperatures has already reached 1.3°C and when 200 nations revisited the issue in Egypt, they remained committed to their 1.5°C promise despite the harsh critics. As we enter a new era, we will need more energy and material resources to sustain the level of connectivity that Industry
4.0 is all about. With the recent fall of the crypto currencies, one does wonder if we will ever need more energy to mine that too? Still, we do not have the same luxury as previous generations to add to the burden of climate change as the consequences have become near irreversible. Extreme weather conditions such as droughts and wildfires with devastating outcomes have increased in frequency. As glaciers melt, time is running out for many places that will be left defenseless against the rising sea waters.
Fortunately, big data and advanced computing promises the appropriate collection and relocation of resources based on immediate needs with opportunity to personalize manufacturing, however, what does this mean for declining resources and greenhouse emissions? Digitization of supply chains is expected to reduce waste levels but there is still a need for solid replacement of fossil fuels. Fortunately, global trends show that the price of certain renewable energy resources such as solar power have significantly declined from where it started out. With fossil fuels prices on a steady incline, Industry 4.0 technologies may even supplement the accessibility to these resources in the coming years.
Undoubtedly, the issue of greenhouse gas emissions is more than the availability of different renewable energy resources, there is an underlying provision instigated by large corporations and oil companies because the use of renewable resources doesn’t fit their business model.
Furthermore, the Russia-Ukraine conflict has forced some European countries to return to coal burning, the dirtier fossil fuel. The United States’ short departure from efforts to reverse climate change during the Trump era also hasn’t helped the global state of environmental affairs. China may remain to its commitment to reduce emissions as the world’s largest emitter, but this is hanging on a thin thread depending on how the US is willing to keep its promises to reduce emissions. We remain confident in our scientists and engineers, and with the increasing connectivity, technologies will advance even more to address climate change and it may be just enough to alleviate the symptoms of a disease that is burning our planet to its core.
The best action taxpayers can take is to support collective efforts that strive for sustainability and fund research efforts to reverse climate change. Voters must hold governments accountable regarding decisions they make for the collective. Unarguably, this is no longer a moral issue but an existential crisis, that has been unfortunately oversaturated with politicians that have treated the issue as a debate rather than a matter-of-fact that we are on the verge of environmental calamity. It is becoming overwhelmingly clear that if we don’t reach an agreement to take collective action, the damage will become irreversible. Fortunately, Industry 4.0 can help with connectivity as it allows for the congregation of people and ideas across the globe to act
in favor of reverting climate change.
Another concern in the era of Industry 4.0 is the loss of jobs and the increasing skill gap. Undoubtedly, as AI and robots are implemented to replace those in low skill jobs, the rates of unemployment and the demand for highly technical skills is expected to skyrocket. Fortunately, the same digital technologies that are limiting the availability of low skill jobs can also help teach highly technical skills such as programming and problem solving using different modalities. With the recent pandemic having already set the baseline for online education and remote work, Industry 4.0 must continue carrying on the torch.
So, is this era going to fall short to address the current global trends and meet the needs of the environment? Or will it be sufficient to improve our current state of global burdens in a sustainable way? Many have argued that the current literature has analyzed Industry 4.0 from a technological perspective overlooking challenges regarding sustainability. But like James Watts, hopefully we will recognize that neglecting these global problems is parallel to wasting the steam of this era with no direction. This is no longer an issue of economics or politics but of self-preservation.
The large quantities of consumable plastics used in day-to-day life science research does not go unseen. It is estimated that scientific research consumes approximately 5.5 million metric tonnes of plastic globally per year. Additionally, it is estimated that the pharmaceutical industry generates nearly 55% more toxic carbon emissions than the automotive industry, of which the generation of plastic packaging is a driving force. The pharmaceutical and life sciences sectors are driven by a need to improve the welfare of society, and as such, environmental sustainability in the life sciences and pharmaceutical industry must be a focal point for current and future STEM professionals.
Moving towards sustainable packaging in science:
As governments move towards reducing the usage of single-use plastics, the life science and pharmaceutical industries must also take heed and consider sustainable alternatives for plastic packaging. Over the last decade, the pharmaceutical industry has begun to pursue several strategies to improve the sustainability of their products, including “light-weighting” and changing packaging formats.
“Light-weighting” in the pharmaceutical industry involves the generation of thinner, lighter, plastic containers for reagents and medication. In 2018, jARDEN Plastic Solutions worked with manufacturers SACMI group and Milliken & Company to reveal new sustainable pharmaceutical bottles. These
bottles are made of high-density polyethylene using a sustainable plastic production method known as “compression blow forming.” This resulted in the generation of bottles that maintain necessary barrier protection but reduced plastic consumption for manufacturing by up to 28%. However, many of these specialized packages are still single-use and often not made of recyclable materials, which compounds on the issue of disposal.
Changing packaging formats to more sustainable materials is also a strategy being employed by the pharmaceutical and scientific research industries. A notable example is the transition of drug blister packaging from the traditional polyvinyl chloride (PVC) material to recycled polyethylene terephthalate (RPET), or even specialty paper, which is an entirely plastic-free alternative to traditional blister packaging. In addition to alternative plastics, other “green” biodegradable materials have been explored in the pharmaceutical industry. In 2020, Natupharma developed fully recyclable plastic from sugarcane, which has the benefit of degrading in 10 years time as opposed to traditional plastics that take hundreds of years to degrade. As of October 2021, companies such as Astellas Pharma have been using sugarcane-extracted plastics to comprise 50% of their material for developing blister packaging. More recently, Huhtamaki, a notable pioneer of sustainable packaging, developed a blister package made up of an aluminum-free, mono-material PET, significantly increasing the recyclability of the product.
While these alternatives provide a positive outlook, their implementation poses some difficulty. When new eco-friendly materials are being researched, scientists must assess their environmental impact as well as meet stringent requirements for pharmaceutical packaging. This is often easier to overcome with secondary or tertiary packaging that do not come into direct contact with the product, but less so with primary packaging.
Despite these challenges, the future looks promising for alternative packaging in the pharmaceutical and life sciences sectors. Although we are continuing to further the development of eco-materials for packaging, environmentalists and researchers agree that the one of the best strategies to combat increases in single-use plastic is reduction
Biodegradable synthetic polymers have been widely used in biomedical applications, such as in gene delivery, tissue engineering, diagnosis, medical devices, antibacterial/antifouling biomaterials, and as drug carriers. Their utility can be attributed to several factors, including their chemical and mechanical properties, smart responsiveness to diverse external stimuli and their improved processibility, functionality and degradability over other materials. In this article, we will discuss the key features of biodegradable polymers in biomedical applications from a sustainability perspective.
Biodegradable polymers are petrochemical or bio-based compounds that can be degraded by microorganisms through the process of biodeterioration, bio-fragmentation, and assimilation. Due to their excellent biodegradability and biocompatibility, these polymers can be exploited for a number of biomedical applications. An example of this is tissue engineering. In the tissue engineering process, a biodegradable porous scaffold plays a crucial role. Scaffolds not only provide a tissue template, but also support cell attachment, proliferation and differentiation. One promising candidate for tissue engineering applications is aliphatic polyester, which can form stable porous structures that do not dissolve or melt in vitro and can undergo degradation rapidly through hydrolysis of the ester groups in their backbones in aqueous solutions or in vivo. Biodegradable polymers are also of great interest in the field of drug delivery. A biodegradable drug carrier can bring its payload to a specific site in the body and then degrade to release the drug in a controllable manner. Meanwhile, by-products of the biodegradation process are eliminated from the body via natural metabolic pathways. In addition, releasing the drug at a specific target site decreases the toxicity of the drug to healthy cells due to less off-target non-specificity. Synthetic biodegradable polymers also have great potential in gene delivery applications. Of non-viral gene-delivery vectors, cationic synthetic biodegradable polymers have gained the largest interest for use as an alternative to viral vectors due to their high chemical versatility, biocompatibility and low immunogenicity. The biodegradable features of synthetic biodegradable polymers can be utilized to unpack and release therapeutic genes into target cells as well as lower their accumulation in treated cells.
Traditional synthetic polymers have become a serious environmental hazard as they are typically disposed of through landfilling, recycling and incineration. The long degradation period of plastic waste, the increasing scarcity of landfill sites and the air pollution contributed by incineration have made people turn to biodegradable polymers due to their degradable nature, which is critical for global waste management. The biodegradation process is typically carried out by the enzymatic work of microorganisms, thereby forming metabolic products such as methane, water, biomass and carbon dioxide. This occurs through several steps. Firstly, extracellular enzymes and abiotic agents depolymerize long-chain
polymers into oligomers through hydrolysis, oxidation and photo-degradation. Next, oligomers are bio-assimilated by microorganisms and then mineralized, followed by either aerobic degradation or anaerobic degradation. Aerobic degradation occurs in the presence of oxygen, producing CO2, H2O, biomass and residue, while anaerobic degradation takes place in the absence of oxygen, producing the above components along with methane. The non-toxic end products from biodegradable polymers minimize the stress from shrinking landfill availability and plastic pollution. Additionally, many biodegradable polymers, such as polylactic acid (PLA) and polyhydroxy alkanoates (PHA), are synthesized from renewable resources, thereby reducing the dependency on petroleum supply during manufacturing and decreasing greenhouse gas emission into the atmosphere.
One category of biodegradable polymers whose degradation mechanisms have been thoroughly characterized are hydrogels, which are three-dimensional crosslinked polymeric networks with high water absorbability that can be used for diverse drug delivery applications. Hydrogels can be degraded in the body via several mechanisms, such as hydrolytic degradation, pH change, enzymatic degradation and photodegradation. Upon desirable physiological environmental changes, hydrogels are degraded into biocompatible, non-toxic materials that are eliminated through the body’s metabolic system. Another well-characterized synthetic biodegradable polymer is PLA. These polymers possess hydrolytically labile chemical bonds in their polymer backbone, which can be degraded via hydrolysis into lactic acid, which can be rapidly eliminated from the body without toxicity.
The future aspect of biodegradable polymers seems promising. However, there are still several challenges to overcome in order to achieve widespread use of biodegradable polymers in the fields of biomedicine and waste management, including the design and development of diverse and more sophisticated polymers to suit various applications, high manufacturing costs and economic burden for patients. Nevertheless, it is clear that biodegradable polymers will open up new possibilities in the effort to establish a better environment devoid of hazardous substances and products, as well as push the envelope of the development and applications of polymer-based biomedical products.
- Tianning Yu
Without realizing it, artificial chemicals have become the backbone of modern society. From plastic to cosmetics to pharmaceutical industries, chemical-producing sectors are making significant impacts on the current world, culturally and physically. However, with the boom of these industries, the daily manufacturing processes and the waste produced from them have also caused major pollution and resource depletion issues.
Around the 1990s, scientists and industries worldwide started implementing efforts to advance research and develop strategies to address these problems, leading to the first
wave of green chemistry. Green chemistry, also known as sustainable chemistry, is an approach focusing on designing and implementing chemical processes that minimize the use and generation of hazardous substances.
Two pioneer chemists in the field, Paul Anastas and John Warner co-authored the book “Green Chemistry: Theory and Practice” and published the 12 principles of green chemistry. This book is considered the foundation of green chemistry and is still highly relevant to current-day chemical practices.
Prevention
It it better to prevent waste than to clean up waste after it has been created.
Safer Solvents and Auxiliaries
Minimize the total amount of solvents used and, if necessary, choose the safest solvent available.
Atom Economy
Product synthesis should maxmize incorporation of all materials used.
Design for Energy Efficiency
Choose the least energy-intensive chemical route.
Less Hazardous chemical syntheses
Chemical reactions should be designed to be as safe as possible, to both human health and environment.
Use of Renewable Feedstocks
When practical, use chemicals that are made from renewable sources.
Designing Safer Chemicals
The chemical products should be designed to preserve efficacy while reducing toxicity.
Reduce Derivatives
Minimize the use of temporary derivatives (e.g. protecting groups).
Use catalytic reagents to help increase selectivity and minimize waste.
Design for Degradation
Chemical products should be designed to be degraded easily.
Real-time analysis for Pollution Prevention
Real-time monitoring of the process should be developed to prevent formation of hazardous substances.
Inherently Safer Chemistry for Accident Prevention
Chemical process chosen should minimize the potential of chemical accidents.
In the United States, the Environmental Protection Agency (EPA) played an integral role in promoting green chemistry in its early days, by introducing funding and pollution prevention programs. The passing of the Pollution Prevention Act in 1990 boosted the growth of green chemistry, as it declared the need to lower pollution by improving the designs of products instead of their disposal. To this day, the EPA awards multiple “Green Chemistry Challenge” winners year-
830 million pounds of hazardous chemicals and solvents reduced each year
Chemicals to fill a train 47 miles long
Reduce use of 21 billion gallons of water each year
ly to incentivize innovation for “greener” chemical processes.
According to the EPA, as of 2022, the 133 technologies that the agency has awarded for the “Green Chemistry Challenge” have eliminated significant amount of chemical waste. Their progress and the equivalent presentations are listed below:
Water used by 980,000 people annually
7.8 billion pounds of CO2 equivalent emission to air
In 2002, Pfizer was one of the “Green Chemistry Challenge” winners for its alternative manufacturing process for Sertraline Hydrochloride, the active ingredient in Zoloft. This drug is one of the most commonly prescribed anti-depression medications in the US, with over 38 million prescriptions.
Merck recently has also developed a “greener” way to produce Molnupiravir, an anti-viral medication for COVID-19, shortening the manufacturing steps, cutting liquid waste, and increasing the yield by more than 1.5-fold.
Getting 770,000 automobiles off the roads
Merck and Codexis innovated the production of Sitagliptin Phosphate, which is the active ingredient in Januvia, a leading drug for type 2 diabetes. Compared to the 1st generation process, the new manufacturing workflow reduced waste by 5 times, estimated to translate to at least 150 million kg of waste reduction over the lifetime of the drug.
Green chemistry strives to lower the carbon footprint and hazardous by-products of industrial chemical processes without compromising productivity by reducing waste at the source rather than at the disposal. Adopting green chemistry is beneficial from a business standpoint where products can
be created with higher efficiency, or lower costs. More importantly, it directly reduces hazardous chemicals released into the environment, creating a cleaner environment for everyone.
Fossil fuels have long been primary contributors to global warming and environmental destruction since the industrial revolution. One of the largest consumers of fossil fuels remains the automobile industry, with drivers releasing a whopping 4.6 metric tons of carbon dioxide into the air per vehicle annually. Metrics like this are what have demanded for the bold innovation of new types of vehicles that rely on more sustainable energy sources. Electric vehicles (EV) represent a step in this direction, with companies like Tesla and Rivian leading the pack in the introduction of EVs onto the road.
EVs are cars that rely on electricity to power their motors. They work by using electrical energy to spin similarly polarized magnets contained inside their electric motors, which works to subsequently rotate wheel axes and push the car forward. Electricity is stored in large lithium-ion batteries located at the bottom of the vehicle. These batteries are rechargeable, allowing for energy refueling through charging stations that effectively replace traditional gas pumps for these vehicles. It is estimated that EVs generate one-third of the amount of CO2 compared to gasoline vehicles even when considering electricity emissions, which – given mass adoption – represents a sizable impact on global greenhouse gas emissions.
Although the benefits of EVs are tangible and appealing, a gasless future does not come without its challenges. For widespread adoption to occur, first there must be appropriate infrastructure in place for the charging of EVs, as well as sufficient electrical grid output to accommodate for increased energy consumption. Many consumers point towards anxiety over running out of electricity on the road or the inability to find a charging station as primary deterrents towards purchasing an EV. Issues with battery fires have also plagued EVs throughout their history. Fires from lithium-ion batteries, which can sometimes occur spontaneously and are notorious for being difficult to extinguish, have required recalls
of thousands of vehicles over the years. Additionally, an increase in demand for EVs would necessitate upscaling the manufacturing of EV-specific parts and batteries, which may be difficult with predicted reagent shortages in the future. Lithium-ion batteries, for example, require lithium and cobalt as main constituents. These products are mined in energy-intensive or worker-endangering conditions, neither of which are sustainable with an increase in production.
However, efforts are currently underway to account for each of these issues. More charging stations, although expensive at up to $35,000 a pop, are being setup around the world and are being paid for or subsidized by companies and governments. Charging times are getting faster with improved technology and charging fees are slowly getting standardized. Researchers are finding new ways to extract lithium, cobalt, and other important battery constituents that are more efficient and less hazardous. Solid-state batteries are being developed that reduce the carbon footprint of battery production and decrease the chances of battery fires compared to current liquid lithium-ion models. Furthermore, some legislations are pushing for the cessation of gas-powered car sales in the next 15 years, providing incentive for automobile manufacturers to get onboard the zero-emission train.
Despite these efforts, the zero-emission dream seems distant. Most vehicles on the road remain gas-powered and EVs themselves still generate a carbon footprint from battery manufacturing and electricity emissions. However, progress is being made with society finding itself in a transition phase in dealing with automobile emissions. The availability of public transit and hybrid vehicles, which use both electricity and traditional gas fuel in an efficient, lower emission manner, has eased the transition for some consumers looking to go greener. All these efforts together point towards a cleaner and emission-less future, which is looking more and more achiEV-able with time.
- Matthew WongWhen we think of recycling, we imagine the blue bins that line the hallways and are tucked away in a corner of our home. We dump our cardboard boxes, junk mail, and plastic bottles in these bins, feeling assured that we have played our part in reducing waste and saving the environment. But seldom do we wonder - what happens to the recycling that leaves our homes?
According to the US Environment Protection Agency, in 2017, only 35% of the total 267.8 million tons of municipal solid waste was recycled. Recycling is not some magical process that turns a waste item into a useful product. All the recyclables that you discard are commodities that have a market. Paper is the most recycled waste. Most paper and cardboard can be recycled five to seven times until it becomes so degraded that it can no longer be used – a process known as “downcycling”. Other materials such as glass or metal are also highly recyclable. However, it is the plastics that are a major issue. According to the National Geographic, 91% of plastics do not get recycled. They end up in landfills where they get converted to dangerous microplastics or incinerated which produces toxic chemicals and pollutes the air. The 9% that does get recycled are always downcycled to flimsy plastics that cannot be reused. In Canada, 8% of plastic waste is recycled, 6% is incinerated and 86% goes to landfills.
Big corporations that produce plastics, such as Dow, Imperial Oil and Nova Chemicals, have successfully sold us the idea that their plastic bottles are not causing any harm just because they have the precious “recyclable” symbol printed on them. This is far from the truth. Plastic recyclables have different grades assigned to them from 1 through 7. Grade 1 and 2 plastics are the ones that have the greatest potential to be recycled. Hence, they also have the highest price in the market. However, plastics marked as numbers 3 to 7 are not as recyclable and often have a negative value which means you would have to pay money for someone to take them away to be recycled.
Contamination of plastics is another pressing issue being faced by the recyclables industry. Often plastics that are used to package degradable items, such are food products, render the plastics unrecyclable irrespective of their grade.
Ignorance of proper waste separation methods means that a lot of garbage enters the recycling bins. Rigorous separation of such waste is needed and requires money, infrastructure, as well as labor. While single-stream recycling, where all recyclables including paper, plastic or metal are combined in one bin, has made it easier to recycle on our end, it has made the downstream recycling processes extremely challenging. For instance, paper contaminated with oil from food or containing shards of glass is no longer recyclable.
Since 1992, almost half of the world’s recycling is shipped to China. This practice seemed feasible as it fueled China’s booming manufacturing industries. But there was an issue. Plastics from Grades 1 and 2 were mixed with the lower grade plastics when large shipments of recyclables were sent to buyers in China. This was polluting the Chinese countryside and doing more harm than good. In 2018, China imposed regulations curbing the import of low grade and contaminated plastics. This has forced the world to rethink their recycling infrastructure and become self-reliant in processing their recyclable waste.
The world at large is working towards a greener and cleaner future, building innovative technologies to tackle the plastic recycling problem at the forefront. Pyrofuel, a Jordan based start-up has used pyrolysis to develop a plastic-to-fuel system. Scindo and Depoly are companies that work to convert plastic polymers into monomers pure enough to repolymerize into high quality plastic. Ecoplasteam, an Italian start-up has developed an infinitely recyclable plastic. From waste sorting to degradation and creating reusability, every aspect of plastic recycling is being refined and upgraded by scientists and innovators around the world. Ultimately, the onus lies upon each one of us to seek out information, reduce consumption of plastics and to incorporate good recycling practices in our lives. Afterall, it all starts from our blue bins!
- Manjula KamathSince the beginning of the industrial revolution, over 35 billion tons of carbon dioxide (CO2) have been emitted, while atmospheric CO2 has increased by approximately 50% over the same period. CO2 comprises over 70% of greenhouse gases emitted through human activity which are directly responsible for climate change and its associated detriments. Therefore, to blunt and potentially reverse the damaging impacts of climate change, strategies such as carbon pricing have come to the forefront of reducing CO2 emissions.
The costs associated with running a business are generally composed of expenses that contribute to manufacturing or selling a final product and/or service. However, these costs often do not incorporate the potential damages associated with climate change through CO2 emissions from carbon intensive businesses. To account for the detriments associated with CO2 emissions, carbon pricing—an economic instrument—has been introduced to incorporate these potential costs by setting a price for emitted CO2 . By putting a price on CO2 emissions and steadily increasing this price over time, this aims to disincentivize carbon intensive practices while providing incentive for future investments in ventures that are more sustainable.
Carbon pricing can be implemented either through a carbon tax, or an emission trade system (ETS). A carbon tax places a specified price on the CO2 emissions produced by companies. However, since the price of CO2 is pre-determined, the predicted emission reduction outcome is uncertain. This contrasts with the second type of carbon pricing, the ETS. An ETS is a type of cap-and-trade system where the “cap” is a limit placed on total CO2 emissions produced for a
given industry. The “trade” aspect comes in the form of CO2 emission permits given to companies within the industry, where companies can freely buy and sell these permits. Therefore, rather than a pre-determined price for CO2 emissions, the supply and demand of permits dictates the price for CO2 emissions. In Canada, we have implemented several carbon pricing strategies such as the Canada Federal Output-Based Pricing System and the Canada Federal Fuel Charge which are an ETS and carbon tax system respectively.
But how effective is carbon pricing in reducing carbon emissions in the real world? In a 2020 publication, “Carbon Pricing Efficacy: Cross-Country Evidence”, economist Dr. Rohan Best and colleagues looked at CO2 emissions across 142 countries from 1997 to 2017, with 43 countries implementing some form of carbon pricing by the end of their study period. Their findings show that countries that have implemented carbon pricing have a 5% reduction in the growth of CO2 emissions per year compared to countries that have not implemented carbon pricing. After accounting for other factors that may have contributed to this reduction, they estimate that the implementation of carbon pricing was responsible for about a 2% reduction. Although 2% does not seem substantial at first, these annual differences will compound to drastic reductions in CO2 emissions over decades.
Although the idea of carbon pricing seems ideal, it is not without issues. The price of CO2 and the regulation of carbon pricing practices are unfortunately not uniform across different regions around the world. As a result, this leads to carbon leakage where businesses
will shift carbon-intensive operations to regions where the price of carbon is less expensive, and the regulations and associated enforcement are less stringent. To this end, there have been endless debates on determining the correct price of carbon and the feasibility of implementing this price uniformly across carbon markets around the world. Another issue is that while the implementation of cap-andtrade systems generate a market that favours reduction of carbon emissions, this very market opens the potential for market manipulation and speculation in addition to providing companies with significant windfall profits by providing permits free of charge. A relatively recent example can be highlighted in the 2021 annual filing for Tesla, the largest electric vehicle maker in the world by market cap. Tesla was able to generate 1.46 billion dollars from the sale of their carbon permits, which constituted approximately 26% of their total profits for that year.
The costs of carbon pricing have also been shown to disproportionately impact different demographics, raising concerns regarding equitability. Several carbon intensive industries are essential to everyday life such as generating electricity, manufacturing essential goods, and agriculture. Increases in the costs of these essential services due to carbon pricing could result in increased expenses that are ultimately passed down to the consumer. While this increase may not have a noticeable impact to affluent groups, this has a significant impact on households with lower socioeconomic status where these expenses would take up a more meaningful portion of household budget. However, there are methods to overcome these undesired side effects. For example, the implementation of a carbon tax can generate revenue. In the U.S., some
of this revenue has been returned to the public in the form of dividends where the amount given is based on socioeconomic status, offsetting some of the inequities that result from carbon pricing.
Carbon pricing is currently recognized as the most efficient method in reducing CO2 emissions. This has been echoed by over 3000 U.S economists including 28 Nobel Prize laureates in the “Economists Statement on Carbon Dividends”, the largest public statement from
economists in history. Although the implementation of carbon pricing has been well-received by nations around the world, there are still many areas for improvement. Resolving the issue of carbon leakage and ensuring that carbon pricing strategies are carried out in an equitable manner is of utmost importance. If these issues can be resolved however, then carbon pricing is a powerful strategy to meet our climate change goals.
- Robbie JinAdditive manufacturing is the industrial production name for what’s more widely known as “3D printing”. While 3D printing may sound like some cutting-edge technology that only geeks or niched industry professionals will use, the idea behind it is quite simple. Essentially, 3D printing involves using a computer-aided-design (CAD) software to virtually slice a design into thousands of horizontal layers. Then, a nozzle or laser deposits materials to construct each layer from bottom to top.
In the more recent years, media buzz surrounding 3D printing and the possibilities that this new technology promises have flooded the news. According to a 2014 CNBC article, “3D printing will make life as we know it today barely recognizable”. With other claims stating that the average lifespan would reach 110 years old with the accessibility of 3D printed organs. Now, looking back there doesn’t seem to be any follow through on these grand visions. Is 3D printing going to change our lives or is it just
another hyped up topic by the media?
There is no doubt that 3D printing is a revolutionary manufacturing method. In the engineering process, designs are often limited by the manufacturing options available. Designs are practically worthless if engineers cannot figure out how to effectively manufacture their products. The limitations of manufacturing have often stalled integration of emerging technologies and confined creative potential. However, what 3D printing offers to the manufacturing process opens up many new doors. It has allowed for the creation of complex structural designs once considered impossible to manufacture, such as complicated hollow tubes with integrated cooling ducts. Thus, it is incredibly useful for generating parts used in high temperature environments such as turbine blades and rocket nozzles. Another incredible benefit that 3D printing brings to the table is high efficiency. In traditional manufacturing methods, giant blocks of raw materials are machined down to final forms, often losing 90% of the raw material
during processing. This low efficiency refinement is especially detrimental when using expensive material such as titanium alloy, where a majority of it ends up in the waste during the manufacturing process. This raises costs for manufacturers which is then passed down to the consumer. Not to mention, these wasteful methods further damage the environment since larger quantities of raw materials need to be mined or harvested. 3D printing solves these issues by only depositing material exactly where they are needed when generating a product which dramatically decreases waste production. All of this supports the fact that 3D printing is indeed revolutionary, but where is 3D printing now and why hasn’t it flooded the mainstream market?
3D printing has also played a major role in the medical devices industry, especially in the area of prosthetics by allowing medical device designers to innovate faster. Prototyping has remained one of the greatest hurdles in bringing a new invention to the market. This is because designers and engineers have
to carefully navigate around the limitations of the traditional manufacturing methods. If engineers cannot manufacture the design, then the design is not worth the paper it is printed on. 3D printing almost fully negates this problem as it allows designers and engineers to directly produce their prototype in a CAD software such as SolidWorks™. This software can also perform topology optimization which is a type of stress analysis to aid designers in figuring out exactly where material is needed. This helps to optimize a design for its particular application. All of this has allowed designers and engineers to bring their inventions to life incredibly fast which rapidly increases the innovation pipeline. 3D printing has also brought the price of prosthetic down for amputees by increasing their availability and accessibility. Following traditional methods of manufacturing, an expensive mold of the amputee’s limb has to be made. The diverse morphology of all amputee’s limb makes this very difficult because what might work for one person, likely won’t work for another. This creates a price barrier for amputees to obtain prosthetics. With 3D printing, all that has to be done is making a fast 3D scan of the amputee’s limb and saving it as a CAD file. Then the file can be sent to a 3D printer and a custom prosthesis can be made relatively cheaply compared to traditional methods. This workflow completely avoids the expensive mold manufacturing process and can increase access to prosthesis for amputees in need. An example of a well-known 3D printed prosthesis is the futuristic looking Exo by William Root. This design uses minimum material and is 100% custom fitted to its wearer. Due to the fact that it is 3D printed, it also allows the wearer to implement specific designs to give the prosthesis character.
Although a majority of the population can’t relate to prosthesis, most people don’t know that the field of or-
thodontics is also shifting towards 3D printing. Many are required to wear retainers or dental aligners which have to be created to fit unique oral anatomy. Traditionally, a gel is casted in the patients’ mouth, then an experienced technician creates a mold from that cast, lastly a vacuum fits a plastic sheet over the mold to make the final product. This method is prone to inaccuracies and often require trained professionals to make many adjustments. 3D printing can dramatically improve this lengthy and expensive process. All that’s required is a 3D scan of the patient’s mouth and within a few hours an exact replica of that mouth can be generated through a 3D printer. This skips over the need for a technician to manually create the 3D model, dramatically decreasing the cost of making retainers/aligners. From creating a replacement leg to enable amputees to walk again, to printing retainers for teenagers after taking off their braces. 3D printing has been a larger part of our lives than we often think.
From looking at the medical device industry, we can see that 3D printing seems to be moving into the mainstream, but why hasn’t 3D printing moved into other fields? Why aren’t consumers seeing the shelves of stores filled with 3D printed products? The answer is the economics. If we plot the per unit cost of a part as a function of total number of parts created, we can see that for traditional manufacturing it looks like this:
The initial cost of manufacturing is high due to the machines and tools required, but as the number of parts created increase the price of each unit dramatically decreases. Most consumer products follow this form of economics such as iPhones and cars etc. This is because as long as there is continuous demand for the product the same tooling, machinery, and mold can be reused again and again.
If we were to plot the per unit cost of a part as a function of total number of parts created for 3D printing, the graph would look completely different like below:
The initial cost of manufacturing is significantly lower than traditional manufacturing methods, however, as the number of parts produced increase, the price per part doesn’t significantly decrease. This is because each part must be 3D printed from scratch and the only reusable parts are the 3D printers and the CAD files which are very cheap to make in the first place. This is why manufacturers often stick with traditional manufacturing processes because the products are simply cheaper to make due to the sheer scale that is required.
Due to the economics of scale, as of today, manufacturers are sticking with traditional manufacturing methods for mass produced products while only using 3D printing for products that are made in small amounts such as prototypes or customized products. Only when the price of 3D printing can find its way around this problem can it become mainstream in everyday products.
- Jason YangIn the 1980s, the United Nations defined sustainability as “attempting to meet the existing needs without comprising the ability of future generations to meet their needs.” We collectively as a society need to make large-scale changes in how we live to ensure that our planet remains healthy. Understandably, it can be a daunting task to consider how we can reduce our carbon footprints in a way that protects the environment for future generations. There are, however, steps that we can take in our daily lives to safeguard the planet.
Each year the world produces 400 million metric tons of plastic and 10 million tons of this plastic ends up in the ocean. Plastic pollution can be particularly detrimental to aquatic animals, who may ingest synthetic material or become trapped in it. One thing that we can do to live more sustainably is limit the amount of plastic that we use. Instead of purchasing single-use plastic bags at our local grocery stores, we can opt for reusable fabric bags instead. When it comes to water bottles, we should consider switching to one that is more environmentally friendly. In the current day and age, it seems near impossible to avoid plastic as it’s quite literally everywhere. If we are using plastic, we should ensure that we recycle it correctly.
A major producer of CO2 emissions and other air pollutants is the transport sector. Every year the world produces 67 million cars, with 2 vehicles entering our roads every second. Commuting is a necessary part of our lives for many of us. One thing that we can do to reduce our carbon footprint is taking public transport to work or even riding a bike during the warmer seasons. Carpooling is also another way that we can reduce the number of vehicles that are on our roads at any given time. Finally, when thinking about purchasing a car, we should consider buying a car that is environmentally friendly and fuel-efficient.
In recent years there has been a rapid shift in how we make and even think about clothing. Buying trendy but cheap attire is now easier than ever, with the products arriving at our doorstep following a few simple clicks. Due to their poor quality, we are more likely to toss out such items after using them a handful of times. Fast fashion has created an epidemic of sorts, where discarded clothing items end up polluting the planet. One thing that we can do is cut down our consumption of cheaply produced clothing items, choosing instead to buy from sustainable brands that prioritize the Earth’s health. Another alternative to purchasing from fast fashion brands is buying locally or buying from thrift shops.
Thinking about the needs of future generations may feel overwhelming. There will likely be a need to implement stricter legislation that cracks down on companies that produce high carbon emissions. Nonetheless, even minor lifestyle changes on an individual level that take the environment into consideration will ensure that the next generation prospers.
Alan Weisman’s The World Without Us is a nonfiction book that explores the question of what Earth would look like if we were to suddenly disappear and leave behind everything as it stood in the early 2000s. Clues from the past, such as the disappearance of the Mayan civilization, and present, such as nature reclaiming the Demilitarized Zone (DMZ) between North and South Korea, may give us insight into how such a future may come to be. Each chapter focuses on different areas of how man-made creations will come undone and the rewilding of such niches to a pre-Homo sapiens ecosystem, with some likely changes that are to last far beyond our existence.
Weisman uses beautiful imagery to paint a picture of how structures would fall apart and what would appear to replace them. One specific chapter that comes to mind is ‘ The City Without Us’, which focuses on New York City and how nature would reclaim such a vast city over time. Something that seems unimaginable to many of us would become a certainty without humans present to keep the city afloat (quite literally).
Weisman illustrates the terrifying and breathtaking undoing of civilization with frighteningly realistic research, backed up by 30 pages worth of references. As a journalist, Weisman did a fantastic job of interviewing experts across diverse disciplines, along with visiting most places he wrote about, highlighting the thoroughness with which he wrote this book. The content is both condensed and accessible for the lay public, but would not be out of place in an academic textbook.
Although the book was hopeful with regards to how nature will find a way to thrive after our disappearance, it conveys a sense of helplessness about our current situation. Weisman concludes his haunting tour of a world without us by suggesting population control, a dystopian and draconian choice for many. Although I am a believer in being honest with uncomfortable news, I am also a hopeful optimist. I believe that for this book appeal to a larger audience, an empowering message of change could have been made throughout the book. Educating others on our impacts, emphasizing the urgency with which we
must change our lifestyles and providing potential solutions to minimize our impact may be more meaningful than fixating on the overwhelming magnitude of the problem.
Overall, I enjoyed the book. It was well researched and thoroughly informative, but may be hard to reach to certain audiences without some understanding in various scientific disciplines. Nonetheless, I recommend this book to all those who want to imagine what a world without us may look like, and understand how the magnitude of our impact on the environment has led us down the eery path we are heading in. To finish with a quote from the book that I believe summarizes this beautifully:
“Without us, Earth will abide and endure; without her, however, we could not even be”.
- Natalia OgrodnikDepartment of Immunology
University of Toronto
1 King’s College Circle
Toronto, ON M5S 1A8 Canada
Construction of the Medical Sciences Building at the University of Toronto (1969) Photo Credits: University of Toronto Archives.