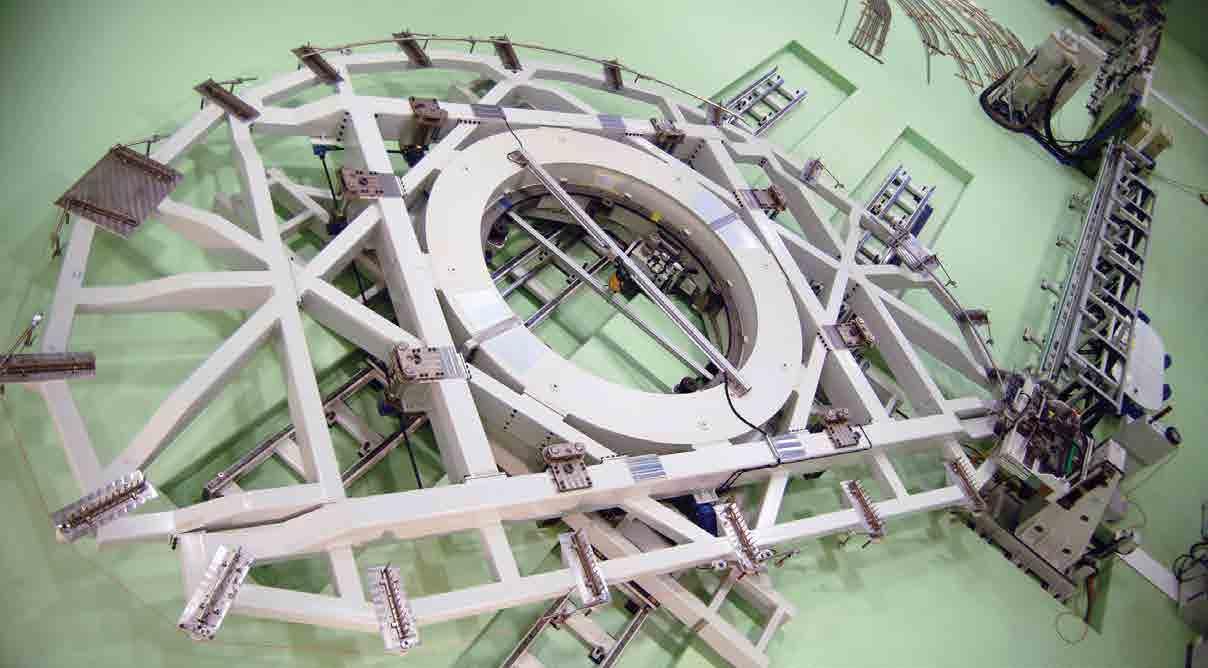
4 minute read
Superconductors make fusion the future for energy
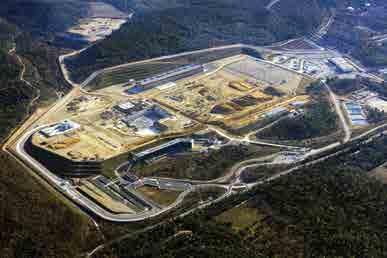
Bruce Meechan reports on work relating to the international development of the ITER fusion reactor and hears from Antonio della Corte, a world authority on super-conductors.
Extremes of temperature are quite literally at the core of the international effort to make fusion reactors a commercial reality in order to alleviate the world’s growing energy crisis and develop a sustainable energy source for the future with zero or low CO2 emission sources.
While debate rages over the relative merits of wind turbines, shale gas and other ‘alternative’ technologies, work is underway to construct an experimental fusion reactor, known as the International Thermonuclear Experimental Reactor – or ITER – at Cadarache, Southern France. Crucial to its success will be the production of large electro-magnets in Italy, featuring the use of super-conducting cable supplied by Tratos Cavi SpA, the leading European manufacturer of Electrical, Electronic and Fibre Optic cables.
Nuclear fusion – as opposed to fission where atoms split – has remained the elusive Holy Grail for physicists over the past half century, offering the promise of virtually unlimited power supply without the production of radioactive waste.
What is more, they will use abundant sources of fuel, and will not leak radiation above normal background levels; so health risks diminish while the end-of-life disposal process for the reactor itself becomes far simpler.
Therefore, although no one has an operational reactor, the day is coming and fusion reactors are now in experimental stages at several laboratories across the United States and around the world. It is anticipated that ITER will demonstrate the feasibility of using sustained fusion reactions to generate electricity.
Employing nuclear fusion generates energy when two atoms join to form one; so in a fusion reactor, hydrogen atoms come together to form helium atoms, neutrons and vast amounts of energy or heat. In order to control and contain the collision process, very large and powerful magnets are required to propel the hydrogen particles. Critical is the fact that unlike conventional magnets wound with copper, those featuring superconducting wire are far more compact and offer 99 per cent or higher efficiency.
Pushing the boundaries
Creating the ITER reactor and the Japanese JT60SA unit are therefore massive undertakings, with international collaboration throughout. The Italian National Agency for New Technologies (ENEA) is responsible for putting together the tender for assembling very large quantities of super-conducting wire in conjunction with Tratos and Criotec; the latter being a specialist in ultra-low temperature work.
Tratos became involved in the first stage of the contract, processing the specialised superconducting material, worth €90 million and supplied by Fusion for Energy (F4E), at its plant in Pieve Santo Stefano in Italy, after which it was transported to Criotec near Turin for final assembly.
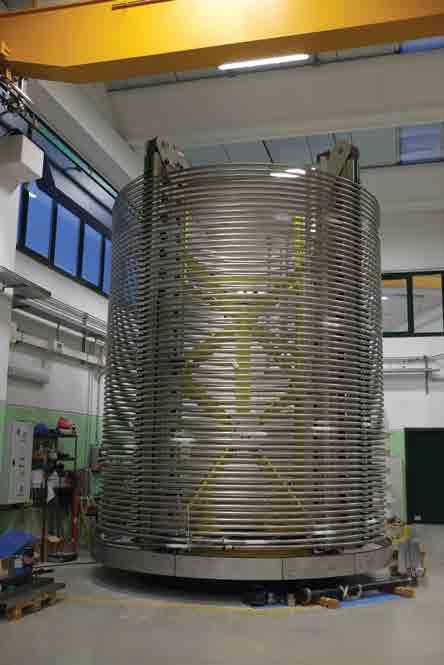
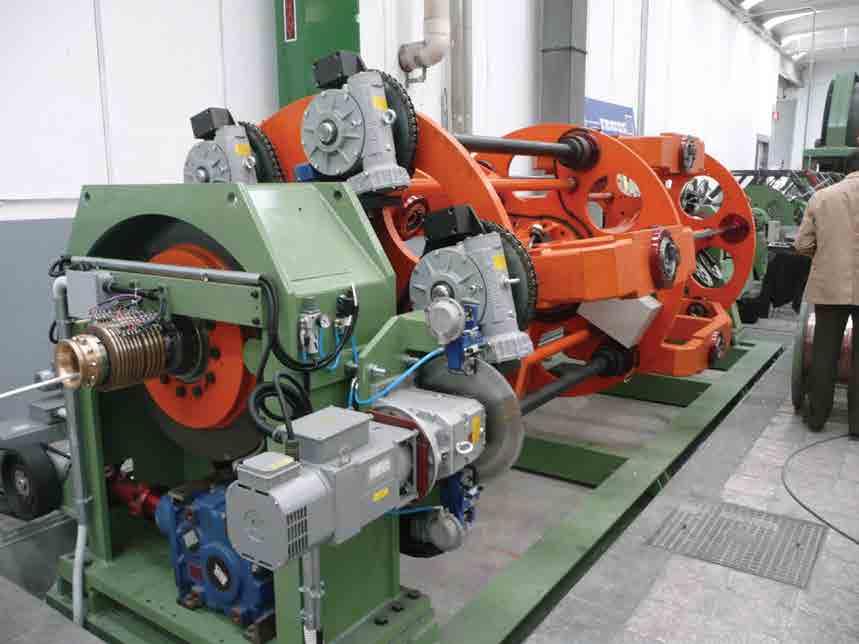
As well as the cutting edge complexity of the science being developed, the quantities involved are also impressive – while the specification boundaries have been pushed higher than ever before in pursuit of the ultimate goal.
The Head of Super-conductive Laboratories for Tratos, Antonio della Corte, reports: “500 tonnes of NbSn and 240 tonnes of NbTi wire are being produced for the ITER project and a further 35 tonnes of NbTi for the JT60SA in Japan. On the ITER project, each Toroidal Field (TF) conductor is composed of 900 super-conducting strands, plus a further 522 copper strands, with each one having an outside diameter of 0.82mm. The final conductor has a diameter of 40.5mm, plus an outer steel jacket that is 2.0mm thick.
“There will be 18 TF magnets built for the ITER project in France measuring 15 metres by nine metres, while the seven PF magnets are a colossal 24 metres across. The JT60 project employs a similar number of magnets.”
Referring to the application of the superconducting magnets to the fusion process, Antonio della Corte continues: “In operation, the plasma confined by the magnetic field generated will be at a temperature of 60 million degrees, while the super conducting strands of the magnets must be maintained at a super-cooled 4.5 Kelvin – equating to minus 268 degree Celsius.
“The function then of the conduit manufactured in South Korea and Italy is to carry the refrigerant liquid, in the form of supercooled helium which enables the resistance of the conducting wires to be reduced to the lowest and most efficient possible levels. The conduits also function to provide shielding against electro-magnetic loads.”
Mega magnets
Super-conducting magnets are, of course, already used successfully in the power industry as well as other fields such as medicine. Some experimental power stations employ Super-Conductor Magnetic Storage units to regulate current, while in hospitals Magnetic Resonance Image (MRI) scanners assist doctors with precise sections through the bodies of patients suffering anything from cancer to cartilage problems. Large diameter super-conducting magnets were also key to the success of the Large Hadron Collider particle accelerator.
The specification of the ITER and JT60SA magnets and the super-conductor wire for them is, however, of the most exacting standards to help crack one of the greatest and potentially most rewarding challenges for mankind.
With Government ministers in the UK revealing that they are making contingency plans to pay industrial users to shut down to avoid black-outs during periods of high demand, and ‘renewable technologies’ proving unreliable as well as expensive, we have to hope that fusion power becomes a reality very soon.
All life on Earth is directly or indirectly reliant on the Sun’s energy – one giant fusion reactor. Now contemporary super-conductor technology is helping us reach for the science of the stars. n
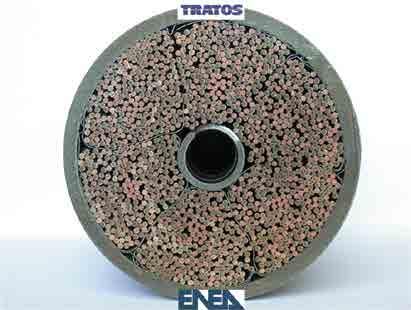
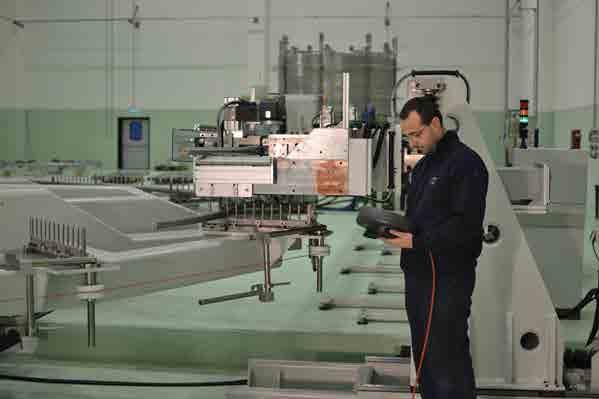